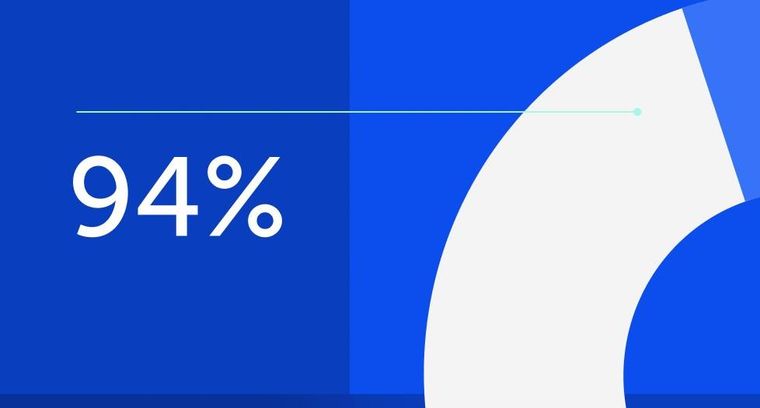
94% of researchers rate our articles as excellent or good
Learn more about the work of our research integrity team to safeguard the quality of each article we publish.
Find out more
MINI REVIEW article
Front. Allergy, 16 June 2022
Sec. Allergen Immunotherapy
Volume 3 - 2022 | https://doi.org/10.3389/falgy.2022.898494
This article is part of the Research TopicInsights in Allergen Immunotherapy: 2021/22View all 4 articles
Allergen immunotherapy (AIT) is the only treatment with disease-transforming potential for allergic disorders. The immunological mechanisms associated with AIT can be divided along time in two phases: short-term, involving mast cell (MC) desensitization; and long-term, with a regulatory T cell (Treg) response with significant reduction of eosinophilia. This regulatory response is induced in about 70% of patients and lasts up to 3 years after AIT cessation. MC desensitization is characteristic of the initial phase of AIT and it is often related to its success. Yet, the molecular mechanisms involved in allergen-specific MC desensitization, or the connection between MC desensitization and the development of a Treg arm, are poorly understood. The major AIT challenges are its long duration, the development of allergic reactions during AIT, and the lack of efficacy in a considerable proportion of patients. Therefore, reaching a better understanding of the immunology of AIT will help to tackle these short-comings and, particularly, to predict responder-patients. In this regard, omics strategies are empowering the identification of predictive and follow-up biomarkers in AIT. Here, we review the immunological mechanisms underlying AIT with a focus on MC desensitization and AIT-induced adverse reactions. Also, we discuss the identification of novel biomarkers with predictive potential that could improve the rational use of AIT.
Allergic diseases are a heterogeneous group of immunological disorders characterized by a detrimental reaction to a given allergen. The onset of allergy occurs at the sensitization phase, which entails induction of a T helper (Th) 2 response and production of interleukin (IL)-4, IL-13 or IL-5, and immunoglobulin (Ig) E. Following sensitization, the effector phase is triggered by allergen re-exposure (1, 2). Effector allergic reactions are complex and often classified -according to the timing of the reaction- in acute and late phase (3, 4). The former is largely (but not exclusively) mediated by IgE (5–8) and its binding to the high-affinity IgE receptor (FcεRI), which is expressed on eosinophils (9, 10), monocytes (11), dendritic cells (12, 13) platelets (14), and specifically on basophils (15, 16) and mast cells (MCs) (17–19). IgE-FcεRI cross-linking leads to MC degranulation and the rapid release of vasoactive and pro-inflammatory mediators (e.g., histamine, tryptase or prostaglandins), which underlies clinical manifestations associated with acute allergic reactions, such as angioedema, hypotension or even anaphylaxis (1, 2, 5, 20, 21). Of these, anaphylaxis is defined as a life-threatening condition that compromises patient's airway, breathing, and/or circulation, and may occur without typical skin features or the presence of cardiovascular collapse (22).
The standard of care is allergen avoidance, when possible, together with the urgent treatment of an allergic reaction upon accidental allergen exposure (23). Allergen immunotherapy (AIT) is the most promising therapeutic approach as it is the only clinical intervention with disease-transforming capacity. AIT has been proven to confer long-term protection and to prevent disease progression and exacerbation. AIT operates in two phases: an early or escalation phase, headed by MC hypo-responsiveness on allergen provocation and an increase of Th2 cells and IgE; and a late or consolidation phase that takes 2–3 years of treatment and is dominated by regulatory T cells (Treg) (24–26). Nevertheless, effector cell activation and adverse side effects can happen at any time during AIT, compromising patients' safety and compliance. Thus, it is essential to discover reliable biomarkers to monitor immunological changes, to prevent side effects and to identify AIT-responder patients that can benefit from intervention.
Recent studies support that AIT efficacy relies on MC desensitization during the initial phase (24, 27, 28). However, the molecular mechanisms underlying AIT-induced MC hypo-responsiveness are controversial (29). Given that MC degranulation is a common driver of anaphylaxis (30), understanding MC desensitization is key, not only for preventing these life-threating reactions, but also for improving current intervention strategies, including AIT. Here, we review the immunological mechanisms underlying AIT with a focus on MC desensitization and AIT-induced adverse reactions. Also, we discuss the identification of novel biomarkers with predictive potential that could improve the rational use of AIT.
AIT constitutes a pivotal pharmacological intervention aiming to control allergic diseases such as allergic rhinitis, allergic asthma, atopic dermatitis, insect venom hypersensitivity (31) or food allergy (29, 32). It consists of the administration of subsequent increasing doses of allergen until an adequate dose is reached, which induces immunological tolerance (31). The efficacy of AIT relies on changes in both innate and adaptive immune cells and is associated with a shift from a Th2 toward a Th1 and Treg phenotype. However, despite being in use for 110 years, the immunological mechanisms of AIT remain poorly understood (33).
A 3-year-follow-up study demonstrated that the immunological changes induced by sublingual AIT come about in two sequential phases (Figure 1). First, an early desensitization phase which takes place in the first 4 months. This stage is accompanied by an initial but short invigoration of Th2 immunity, with an increase in both allergen-specific Ig (sIg) E and IL4+ cells (24). In addition, AIT has been demonstrated to impair MC degranulation in this early stage (29, 32, 34). Finally, there is a later augmentation in sIgG/sIgG4 levels, which compete with sIgE and inhibit sIgE, thus preventing MC and basophil activation and their production of Th2-related cytokines (29).
Figure 1. Schematic representation of early and late immune changes associated to AIT. The early phase of AIT (1–4 months) is dominated by a decrease in MC activation, known as desensitization, and an initial invigoration of Th2 immunity, with increasing levels of allergen sIgE and IL4+ cells. Then, sIgE decreases while sIgG4 increases at the end of the early phase. Consolidation of AIT needs 2–3 years of treatment and is defined by an increase in Treg responses and a decrease in Th2 immune responses as well as in peripheral eosinophilia. amTreg, active memory Treg; MC, mast cell.
Next comes the consolidation of the regulatory response, which needs at least 3 years of AIT (24). During this period there is a contraction of IL4+ cells, a downregulation of sIgE levels and a decrease in blood eosinophilia (24). In parallel, there is an increase in Treg responses, particularly activated memory Treg cells (24). Recent studies have pointed out that there is a regulatory network between MCs and Tregs. On the one hand, Treg suppresses MC activation by different mechanisms (i.e., IL-10 secretion, MC anergy via OX40L engagement) (29). On the other hand, in a food allergy model, desensitized MCs facilitated a Treg cell expansion in a IL-2-dependent manner (32).
Altogether, MCs appear to play key roles in both early and late phase AIT. As previous reports demonstrated strong benefits only few months after starting treatment (24), MC desensitization seems to be a key mechanism in keeping AIT efficacy.
Local and systemic adverse reactions have been observed during AIT (22, 35). Of these, systemic reactions are described in ~1–4% of patients and can be mild to severe, anaphylaxis being the gravest (31). Over the years, diverse definitions of anaphylaxis have appeared in the literature with the purpose of improving its diagnosis and patients' management. Lately, the World Allergy Organization (WAO) depicted anaphylaxis as a potential life-threatening compromise of airways, breathing, and/or circulation, which may occur without typical skin symptoms or the presence of circulatory shock (22). These symptoms are usually developed within the first 30 min after AIT administration (31).
While the occurrence of adverse reactions in AIT is influenced by factors such as viral infections, fever, physical activity, non-steroidal anti-inflammatory drug use, hormonal changes, etc., the route of administration and allergen type are determinant. AIT with aeroallergens is usually administered subcutaneously and is less likely to induce anaphylactic reactions (36, 37). On the other hand, adverse allergic reactions including anaphylaxis are more common in AIT with food allergens (38). In terms of the route, subcutaneous AIT in peanut allergy is highly associated with anaphylaxis (39), but oral and sublingual AIT for peanut (and other food allergens) are clearly safer (40–44). Despite being safer, a recent systematic review and meta-analysis showed that the risk of anaphylaxis was significantly higher in peanut-allergic patients undergoing oral AIT than in those following allergen avoidance (45).
The classical pathway of anaphylaxis is IgE-mediated and involves MCs and basophils (5–8) and recently, omalizumab in combination with AIT has been proven to improve patients' outcome (46). However, IgE-independent mechanisms have also been described in murine models, and there is growing evidence of their importance in humans (5, 8, 47). These mechanisms involve IgG and platelet activating factor (PAF) release by neutrophils, basophils and macrophages (5), or complement activation. Non-immunological anaphylaxis can also occur through the direct stimulation of MC degranulation (48, 49) or by Mas-related G protein-coupled receptor X2 (MRGPRX2) expressed in MCs. In addition, the differential contribution of the endothelium to the pathophysiology of the anaphylaxis is being increasingly recognized, which adds another layer of complexity to this clinical manifestation (50).
Anaphylaxis severity is correlated to MC degranulation and the release of pro-inflammatory mediators (50–52). Intriguingly, anaphylactic mediators such as histamine are released during AIT without induction of anaphylaxis (53, 54), which insinuates that a certain level of MC activation may be required to achieve desensitization. MC desensitization is accomplished during the early-phase of AIT, and studies in murine models support that this process directs the immunological outcome of AIT (30). However, the molecular mechanisms of AIT involve several effector cell types (55, 56). Therefore, it is likely that different cellular and molecular microenvironments created between immune and non-immune cells modify the threshold of a detrimental inflammatory MC response during AIT.
MCs are key effector cells in allergic disease for different reasons. They are immune sentinels located in mucosal and epithelial tissues, close to the vascular and lymphatic endothelium (57, 58). Because of this strategic distribution, MCs sense and respond promptly to allergens or pathogens (59, 60). Furthermore, MCs have a long lifespan as compared to its mobile analog, the basophil (61); retain surface IgE for months (62, 63); and can react to minute amounts of allergen (64). On activation, MCs degranulate rapidly because they are equipped with cytoplasmic granules (50–200 per MC) that contain preformed allergic mediators (60, 65).
In sensitized individuals, IgE-FcεRI complex clustering causes MC activation and degranulation. FcεRI activates several pathways through the immunoreceptor tyrosine-based activation motif in its cytoplasmic domain, e.g., Syk, PI3K/Akt, ERK and STAT6 (66, 67). These routes increase the intracellular calcium flux, which is crucial for exocytosis of preformed inflammatory mediators such as histamine or tryptase. Also, they activate the de novo synthesis of late-phase inflammatory cytokines (e.g., IL-6, TNF-α), prostaglandins, leukotrienes, and PAF, among others (29). The rapid release of these vasoactive and inflammatory mediators underlies clinical manifestations associated with acute allergic reactions (i.e., angioedema, hypotension or cardiovascular collapse and anaphylaxis) (5, 20, 21).
The IgE-MC pathway has long been a target for therapeutic intervention, and some drugs and biologicals have been developed to interfere with it (20, 29, 68). In this regard, AIT has been shown to dampen this axis (24, 26). Several in vitro and in vivo studies in mice have demonstrated that MC become hypo-responsive to allergen exposures after desensitization (34). MC desensitization appears to be allergen-specific (69, 70) and reversible (71, 72). Yet, the molecular mechanisms underlying AIT-induced MC desensitization remain controversial (29) (Figure 2).
Figure 2. Putative mechanisms of MC desensitization in AIT. (A) Internalization of the IgE-FcεRI complex. (B) Actin-cytoskeleton remodeling and impaired calcium flux in MC after. (C) Dysregulation of STAT6 pathway. MCs, mast cells.
Different in vitro studies support that increasing doses of allergen induce IgE-FcεRI complex internalization, rendering MCs unresponsive to allergen challenge (70, 73). In contrast, others report a partial IgE reduction in desensitized MCs (69, 72, 74, 75). In these studies, primary MCs of different origins were assayed, including murine and rat peritoneal MCs (70, 73), murine bone marrow-derived MCs (69, 74), and human lung MCs (72), which may explain this inconsistency. Moreover, MC sensitization was performed with different IgE clones such as SPE-7 (69, 74) and ε-26 (70). However, experiments with the same clone yielded divergent results (69, 70). Other experimental variables may have contributed to the discrepancy in IgE internalization. For instance, Oka et al. (70) used lower MC cellularity and a higher target dose of allergen than Sancho-Serra et al. (69), which may have facilitated IgE saturation and internalization in the former. Despite the variable results on IgE internalization, all these experimental approaches induced MC desensitization. In other words, MC desensitization is accomplished whether the loss of IgE is total or partial. Hence, mechanisms other than IgE-FcεRI internalization might be at play during MC desensitization. The recent research of Nagata et al. (75) report that the size of the IgE-FcεRI internalization complexes are responsible of MC desensitization success.
Another line of inquiry on the mechanisms of MC desensitization focused on the STAT6 pathway. Morales et al. reported that murine STAT6-deficient bone marrow-derived MCs failed to get desensitized (76), although they also showed that MC desensitization did not induce STAT6 phosphorylation (69). Additional experiments in peritoneal MCs from STAT6-null mice demonstrated that STAT6 was redundant for desensitization (70). STAT6 affects different aspects of MC biology, and its deficiency may cause unspecific effects depending on the maturity of MCs. For example, STAT6 is required for IL4-dependent responses (77), which increase FcεRI expression on MCs (18). Besides STAT6, recent studies are shedding light on the cytoskeletal dynamics that drive MC activation and desensitization (66, 78, 79). Gladys Ang et al. (74) showed that desensitized MCs had an atypical but stable redistribution of the actin cytoskeleton, which precluded calcium flux from intracellular stores and abrogated exocytosis of inflammatory granules.
There are some questions remaining on the mechanisms of MC desensitization. The notion that IgE-FcεRI complex internalization occurs to some degree during MC desensitization is well established, but how this process is regulated is largely unknown. In this regard, recent studies in murine MCs suggested that sIgG binding to FcγRIIB is required for IgE downregulation (80), and other MC inhibitory signaling pathways such as gp49B1/LILRB4 (81) may be also involved in MC desensitization. Nevertheless, in vitro experimentation supporting IgE-FcεRI complex internalization was conducted in the absence of IgG (69, 70, 74). Moreover, the role of STAT6 in MC desensitization is controversial (69, 76) and the kinetics of STAT6 phosphorylation during desensitization are not clearly defined.
From a broader perspective, the current mechanistic knowledge on MC desensitization can explain how it occurs at the cellular or local level. However, the fact that minute amounts of allergen can desensitize systemically in AIT, even by sublingual route (82–84), is certainly intriguing and points toward the participation of widespread, fast-acting systems. Further studies are necessary to understand how allergen desensitization operates at the level of an entire organism, as well as to identify biomarkers to monitor/predict successful MC desensitization in AIT.
A biomarker is any substance objectively measured that can be used as an indicator of biological/pathological processes, or pharmacologic responses to a therapeutic intervention. There is a lack of reliable biomarkers that can accurately reflect the clinical course or predict a positive response to AIT (85–87). Despite this dearth, there are some in vivo and in vitro biomarkers applied to monitor AIT safety and efficacy.
In the clinical practice, in vivo biomarkers such as skin prick test (SPT), intradermoreaction, nasal provocation and controlled exposure tests in chambers evaluate allergen-specific reactivity, which is expected to decrease after AIT (88). In vitro biomarkers are based on the cellular and humoral events that take place during AIT (89). Some widespread biomarkers are the determination of total IgE (tIgE) and sIgE. The latter is the gold-standard test for AIT patient selection. A high sIgE/tIgE ratio is predictive of positive responses to AIT (90, 91), although it has not been properly validated. AIT-induced desensitization correlates with a CD4+ T cell shift from Th2 towards a Th1 and Treg phenotype (Table 1). Also, sIgE increases during up-dosing but decreases during the maintenance phase, in parallel with a higher production of sIgG4, which suggests the development of a Treg response (24). AIT has also been shown to increase sIgA (113) and IL-10-producing innate-like lymphoid cells 2 (98).
Other biomarkers for AIT efficacy are the assessment of the serum inhibitory activity of IgE, which can be measured by IgE-facilitated allergen binding (IgE-FAB) (85) or enzyme-linked immunosorbent-facilitated antigen binding assay (ELIFAB). IgE-FAB determines the binding of allergen-IgE complexes to B cells via the low-affinity IgE receptor (FcεRII or CD23). The decrease of IgE-FAB correlates with a positive clinical response to AIT (87). It has been reported that serum IgE-inhibitory activity persists for several years and is associated with long-term clinical efficacy (114). Moreover, in vitro assays, like the basophil activation test (BAT) (115), which measures lysosomal-associated proteins indicative of degranulation (e.g., CD63, CD203c) have been used to evaluate basophil suppression in AIT (85, 91, 116). Also, cytokines, chemokines and cellular markers have been applied for the study of AIT (Table 1).
During the last several years, omics have been applied in AIT research. Omics are techniques that use high-throughput approaches, each one correlating with a specific level of the system biology. Genomics, epigenomics, transcriptomics, proteomics, metabolomics (including lipidomics) and microbiomics could empower the identification of new diagnostic strategies for AIT (117) (Table 1). Genomics has been applied for the discovery of genetic variants that predispose to atopy (118) or affect asthma severity (119). Genetic variants that associate with good AIT outcomes could be used as biomarkers moving forward to stratify patients prior to treatment (28). Epigenomics studies have suggested that DNA methylation patterns, specifically in gene promoter regions associated with Forkhead box protein 3 (FoxP3), could inform of AIT progress (120, 121). Additionally, it has been proposed that the microbiota composition could influence AIT efficacy (111), which is another potential source of AIT biomarkers. Furthermore, transcriptomics and proteomics have been used to improve AIT patient selection through the characterization of allergen extracts, along with a profiling of IgE reactivity (113, 122, 123). Regarding metabolomics, a recent study demonstrated that the type of the patient's sensitization (mono- or poli-sensitized) is key in the clinical response to AIT (26). A different study focused on eicosanoid profiles showed that they increased at the beginning of AIT and then decreased after 1 to 3 years of AIT, decreasing at year 3 to levels below than baseline (110). Finally, techniques such as immunophenotyping using flow cytometry and mass spectrometry have allowed the parallel analysis of all cell subpopulations in a sample during AIT (124).
Despite the widespread use of AIT for more than 110 years, MC desensitization has just recently been identified as a key mechanism during the first 2 years of AIT. Yet, fundamental mechanisms associated with desensitization remain obscure. How a MC gets desensitized in an allergen-specific manner and how the desensitization pattern is transmitted throughout all barrier systems is certainly intriguing. MCs have a broad repertoire of signaling pathways. Due to the potential for inducing life-threatening reactions, research focus has always been on MC degranulation, perhaps overlooking their role as lipid-secreting mediators such as prostaglandins or leukotrienes. Moreover, MCs hypo-responsiveness, even without dampening Th2 responses, is effective not only in anaphylaxis prevention, but also for the control of allergic symptoms and reduction of medication usage (83, 125). This supports the key role of MC activation in allergic inflammation.
The sustained and disease-modifying effect of AIT is linked to the acquisition and epigenetic fixation of a regulatory phenotype. However, how the initial MC control predates the Treg response is unclear. Understanding this link is pivotal for the design of new AIT strategies aiming to avoid IgE-mediated reactivity. To date, no study with strict focus on Treg induction has proven to be effective. If effector cell desensitization governs AIT during the first 2 years of intervention, studies aiming to bypass effector cell activation should be planned for at least 3 years of intervention.
Different inflammatory routes have been described in anaphylaxis. AIT reduces IgE and likely impairs the classical pathway of anaphylaxis, but its effect on allergic reactions mediated by alternative pathways is debatable. Alternative routes could be activated during allergic sensitization (126), and might be relevant in pediatric anaphylaxis and AIT to foods. Should this be the case, AIT patient selection may benefit from novel biomarkers that classify patients according to the dominant inflammatory routes (127).
MME and DB designed the manuscript structure and participated in the writing and discussion. CLS, VE, and RJS participated in the design, writing, and discussion of the manuscript. CLS, RJS, CPC, AV, and MIDD collaborated in the writing, correction, and discussion of the manuscript. AV and CPC prepared the table. MME and MIDD participated in the design and preparations of the figures.
This work was supported by ISCIII (PI18/01467 and PI19/00044), cofounded by FEDER Investing in your future for the thematic network and co-operative research centers and the thematic network RICORS “Red de Enfermedades Inflamatorias” (REI) RD21 0002 0008. This work was also supported by the Ministry of Science, Innovation and Universities in Spain (PCI2018-092930) co-funded by the European program ERA HDHL – Nutrition & the Epigenome, project Dietary Intervention in Food Allergy: Microbiome, Epigenetic and Metabolomic interactions DIFAMEM. MIDD is supported by FPI-CEU predoctoral fellowships. RJS's laboratory acknowledges the support received by the Severo Ochoa Program (AEI/SEV-2017-0712), FSE/FEDER through the Instituto de Salud Carlos III (ISCIII; CP20/00043), The Nutricia Research Foundation (NRF-2021-13), New Frontiers in Research Fund (NFRFE- 2019-00083), and SEAIC (BECA20A9). VE laboratory was supported by ISCIII (PI21/00158) cofounded by FEDER Investing in your future for the thematic network and co-operative research centres ARADyAL RD16/0006/0013 and SEAIC (19_A08).
The authors declare that the research was conducted in the absence of any commercial or financial relationships that could be construed as a potential conflict of interest.
All claims expressed in this article are solely those of the authors and do not necessarily represent those of their affiliated organizations, or those of the publisher, the editors and the reviewers. Any product that may be evaluated in this article, or claim that may be made by its manufacturer, is not guaranteed or endorsed by the publisher.
AIT, allergen immunotherapy; BAT, basophil activation test; ELIFAB, enzyme-linked immunosorbent-facilitated antigen binding assay; FcεRI, high-affinity IgE receptor; FcεRII, low-affinity IgE receptor; FoxP3, Forkhead box protein 3; IgE-FAB, IgE-facilitated allergen; Ig, immunoglobulin; IL, interleukin; ITAM, immunoreceptor tyrosine-based activation motif; MC, mast cell; MoAb, monoclonal antibodies; MRGPRX2, Mas-related G protein-coupled receptor X2; PAF, platelet activating factor; sIg, specific immunoglobulin; SPT, skin prick test; STAT6, signal transducer and activator of transcription 6; Th, T helper cells; tIgE, total IgE; TNF-α, tumor necrosis factor alfa; Treg, regulatory T cells.
1. Breiteneder H, Diamant Z, Eiwegger T, Fokkens WJ, Traidl-Hoffmann C, Nadeau K et al. Future research trends in understanding the mechanisms underlying allergic diseases for improved patient care. Allergy. (2019) 74:2293–311. doi: 10.1111/all.13851
2. Escribese MM, Gómez-Casado C, Barber D, Diaz-Perales A. Immune polarization in allergic patients: role of the innate immune system. J Investig Allergol Clin Immunol. (2015) 25:251–8.
3. Jiménez-Saiz R, Chu DK, Waserman S, Jordana M. Initiation, Persistence and Exacerbation of Food Allergy. In: Schmidt-Weber C, editor. Allergy Prevention and Exacerbation. Birkhäuser Advances in Infectious Diseases. Springer, Cham (2017): 121–144. doi: 10.1007/978-3-319-69968-4_7
4. Valenta R, Hochwallner H, Linhart B, Pahr S. Food allergies: the basics. Gastroenterology. (2015) 148:1120–31.e4. doi: 10.1053/j.gastro.2015.02.006
5. Finkelman FD, Khodoun MV, Strait R. Human IgE-independent systemic anaphylaxis. J Allergy Clin Immunol. (2016) 137:1674–80. doi: 10.1016/j.jaci.2016.02.015
6. Godon O, Hechler B, Jönsson F. The role of IgG subclasses and platelets in experimental anaphylaxis. J Allergy Clin Immunol. (2021) 147:1209–11. doi: 10.1016/j.jaci.2021.01.009
7. Elieh Ali Komi D, Wöhrl S, Bielory L. Mast cell biology at molecular level: a comprehensive review. Clin Rev Allergy Immunol. (2020) 58:342–65. doi: 10.1007/s12016-019-08769-2
8. Jönsson F, De Chaisemartin L, Granger V, Gouel-Chéron A, Gillis CM, Zhu Q et al. An IgG-induced neutrophil activation pathway contributes to human drug-induced anaphylaxis. Sci Transl Med. (2019) 11:1479. doi: 10.1126/scitranslmed.aat1479
9. Rosenberg HF, Dyer KD, Foster PS. Eosinophils: changing perspectives in health and disease. Nat Rev Immunol. (2013) 13:9–22. doi: 10.1038/nri3341
10. Lee JJ, Jacobsen EA, Ochkur SI, McGarry MP, Condjella RM, Doyle AD et al. Human vs. mouse eosinophils: ‘that which we call an eosinophil, by any other name would stain as red'. J Allergy Clin Immunol. (2012) 130:572–84. doi: 10.1016/j.jaci.2012.07.025
11. Shin JS, Greer AM. The role of FcεRI expressed in dendritic cells and monocytes. Cell Mol Life Sci. (2015) 72:2349–60. doi: 10.1007/s00018-015-1870-x
12. Wang B, Rieger A, Kilgus O, Ochiai K, Maurer D, Födinger D et al. Epidermal Langerhans cells from normal human skin bind monomeric IgE via Fc epsilon RI. J Exp Med. (1992) 175:1353–65. doi: 10.1084/jem.175.5.1353
13. Bieber T, Salle HD, La Wollenberg A, Hakimi J, Chizzonite R, Ring J, et al. Human epidermal Langerhans cells express the high affinity receptor for immunoglobulin E (Fc epsilon RI). J Exp Med. (1992) 175:1285–90. doi: 10.1084/jem.175.5.1285
14. Hasegawa S, Pawankar R, Suzuki K, Nakahata T, Furukawa S, Okumura K, et al. Functional expression of the high affinity receptor for IgE (FcεRI) in Human platelets and its' intracellular expression in human megakaryocytes. Blood. (1999) 93:2543–51. doi: 10.1182/blood.V93.8.2543
15. Malveaux FJ, Conroy MC, Adkinson NF. IgE receptors on human basophils. Relationship to serum IgE concentration. J Clin Invest. (1978) 62:176–81. doi: 10.1172/JCI109103
16. Maclashan D, McKenzie-White J, Chichester K, Bochner BS, Davis FM, Schroeder JT, et al. In vitro regulation of FcRIα expression on human basophils by IgE antibody. Blood. (1998) 91:1633–43. doi: 10.1182/blood.V91.5.1633
17. Matsuda A, Okayama Y, Ebihara N, Yokoi N, Gao P, Hamuro J et al. High-affinity IgE receptor-β chain expression in human mast cells. J Immunol Methods. (2008) 336:229–34. doi: 10.1016/j.jim.2008.05.006
18. Ra Hano Toru C, Nonoyama S, Suzuki K, Yata J, Nakahata T, et al. Induction of the high-affinity IgE receptor (FcεRI) on human mast cells by IL-4. Int Immunol. (1996) 8:1367–73. doi: 10.1093/intimm/8.9.1367
19. Bax HJ, Bowen H, Dodev TS, Sutton BJ, Gould HJ. Mechanism of the antigen-independent cytokinergic SPE-7 IgE activation of human mast cells in vitro. Sci Rep. (2015) 5:9538. doi: 10.1038/srep09538
20. Dahlin JS, Maurer M, Metcalfe DD, Pejler G, Sagi-Eisenberg R, Nilsson G. The ingenious mast cell: contemporary insights into mast cell behavior and function. Allergy. (2022) 77:83–99. doi: 10.1111/all.14881
21. Galli SJ, Tsai M. IgE and mast cells in allergic disease. Nat Med. (2012) 18:693–704. doi: 10.1038/nm.2755
22. Cardona V, Ansotegui IJ, Ebisawa M, El-Gamal Y, Fernandez Rivas M, Fineman S, et al. World allergy organization anaphylaxis guidance 2020. World Allergy Organ J. (2020) 13:100472. doi: 10.1016/j.waojou.2020.100472
23. Yu W, Freeland DMH, Nadeau KC. Food allergy: immune mechanisms, diagnosis and immunotherapy. Nat Rev Immunol. (2016) 16:751–65. doi: 10.1038/nri.2016.111
24. Varona R, Ramos T, Escribese MM, Jimeno L, Galán A, Würtzen PA, et al. Persistent regulatory T-cell response 2 years after 3 years of grass tablet SLIT: links to reduced eosinophil counts, sIgE levels, and clinical benefit. Allergy. (2019) 74:349–60. doi: 10.1111/all.13553
25. Suárez-Fueyo A, Ramos T, Galán A, Jimeno L, Wurtzen PA, Marin A, et al. Grass tablet sublingual immunotherapy downregulates the TH2 cytokine response followed by regulatory T-cell generation. J Allergy Clin Immunol. (2014) 133:43. doi: 10.1016/j.jaci.2013.09.043
26. Barker-Tejeda TC, Bazire R, Obeso D, Mera-Berriatua L, Rosace D, Vazquez-Cortes S et al. Exploring novel systemic biomarker approaches in grass-pollen sublingual immunotherapy using omics. Allergy. (2021) 76:1199–212. doi: 10.1111/all.14565
27. Drazdauskaite G, Layhadi JA, Shamji MH. Mechanisms of allergen immunotherapy in allergic Rhinitis. Curr Allergy Asthma Rep. (2021) 21:2. doi: 10.1007/s11882-020-00977-7
28. Zelm MC, McKenzie CI, Varese N, Rolland JM, O'Hehir RE. Recent developments and highlights in immune monitoring of allergen immunotherapy. Allergy. (2019) 74:2342–54. doi: 10.1111/all.14078
29. Tontini C, Bulfone-Paus S. Novel approaches in the inhibition of IgE-induced mast cell reactivity in food allergy. Front Immunol. (2021) 12:2725. doi: 10.3389/fimmu.2021.613461
30. Woo HY, Kim YS, Kang NI, Chung WC, Song CH, Choi IW et al. Mechanism for acute oral desensitization to antibiotics. Allergy. (2006) 61:954–8. doi: 10.1111/j.1398-9995.2006.01147.x
31. Moote W, Kim H, Ellis AK. Allergen-specific immunotherapy. Allergy, Asthma Clin Immunol. (2018) 14:53. doi: 10.1186/s13223-018-0282-5
32. Takasato Y, Kurashima Y, Kiuchi M, Hirahara K, Murasaki S, Arai F et al. Orally desensitized mast cells form a regulatory network with Treg cells for the control of food allergy. Mucosal Immunol. (2020) 143:14:640–51. doi: 10.1038/s41385-020-00358-3
33. Pfaar O, Bousquet J, Durham SR, Kleine-Tebbe J, Larché M, Roberts G et al. 110 years of Allergen immunotherapy: a journey from empiric observation to evidence. Allergy. (2022) 77:454–68. doi: 10.1111/all.15023
34. Zhao W, Gomez G, Macey M, Kepley CL, Schwartz LB. In vitro desensitization of human skin mast cells. J Clin Immunol. (2012) 32:150–60. doi: 10.1007/s10875-011-9605-8
35. Simons FER, Ardusso LRF, Bilò MB, El-Gamal YM, Ledford DK, Ring J, et al. World allergy organization guidelines for the assessment and management of anaphylaxis. World Allergy Organ J. (2011) 4:13–37. doi: 10.1097/WOX.0b013e318211496c
36. Incorvaia C, Ridolo E, Mauro M, Pucciarini F, Heffler E, Canonica GW. Venom immunotherapy and aeroallergen immunotherapy: how do their outcomes differ? Front Allergy. (2022) 0:14. doi: 10.3389/falgy.2022.854080
37. Nelson HS. Allergy immunotherapy for inhalant allergens: strategies to minimize adverse reactions. Allergy Asthma Proc. (2020) 41:38–44. doi: 10.2500/aap.2020.41.190014
38. Mori F, Giovannini M, Barni S, Jiménez-Saiz R, Munblit D, Biagioni B, et al. Oral immunotherapy for food-allergic children: a pro-con debate. Front Immunol. (2021) 12:3861. doi: 10.3389/fimmu.2021.636612
39. NELSON H, LAHR J, RULE R, BOCK A, LEUNG D. Treatment of anaphylactic sensitivity to peanuts by immunotherapy with injections of aqueous peanut extract1. J Allergy Clin Immunol. (1997) 99:744–51. doi: 10.1016/S0091-6749(97)80006-1
40. Staden U, Rolinck-Werninghaus C, Brewe F, Wahn U, Niggemann B, Beyer K. Specific oral tolerance induction in food allergy in children: efficacy and clinical patterns of reaction. Allergy. (2007) 62:1261–9. doi: 10.1111/j.1398-9995.2007.01501.x
41. Longo G, Barbi E, Berti I, Meneghetti R, Pittalis A, Ronfani L et al. Specific oral tolerance induction in children with very severe cow's milk–induced reactions. J Allergy Clin Immunol. (2008) 121:343–7. doi: 10.1016/j.jaci.2007.10.029
42. O'B Hourihane J, Beyer K, Abbas A, Fernández-Rivas M, Turner PJ, Blumchen K et al. Efficacy and safety of oral immunotherapy with AR101 in European children with a peanut allergy (ARTEMIS): a multicentre, double-blind, randomised, placebo-controlled phase 3 trial. Lancet Child Adolesc Heal. (2020) 4:728–39. doi: 10.1016/S2352-4642(20)30234-0
43. Anagnostou K, Islam S, King Y, Foley L, Pasea L, Bond S et al. Assessing the efficacy of oral immunotherapy for the desensitisation of peanut allergy in children (STOP II): a phase 2 randomised controlled trial. Lancet. (2014) 383:1297–304. doi: 10.1016/S0140-6736(13)62301-6
44. AR101 Oral immunotherapy for peanut allergy. N Engl J Med. (2018) 379:1991–2001. doi: 10.1056/NEJMoa1812856
45. Chu DK, Wood RA, French S, Fiocchi A, Jordana M, Waserman S, et al. Oral immunotherapy for peanut allergy (PACE): a systematic review and meta-analysis of efficacy and safety. Lancet. (2019) 393:2222–32. doi: 10.1016/S0140-6736(19)30420-9
46. Giannetti M, Silver J, Hufdhi R, Castells M. One-day ultrarush desensitization for Hymenoptera venom anaphylaxis in patients with and without mast cell disorders with adjuvant omalizumab. J allergy Clin Immunol Pract. (2020) 8:1431–5. doi: 10.1016/j.jaip.2019.10.022
47. Jiménez-Saiz R. Drug-induced IgG-neutrophil-mediated anaphylaxis in humans: uncovered! Allergy. (2020) 75:484–5. doi: 10.1111/all.14118
48. Bruhns P, Chollet-Martin S. Mechanisms of human drug-induced anaphylaxis. J Allergy Clin Immunol. (2021) 147:1133–42. doi: 10.1016/j.jaci.2021.02.013
49. Montañez MI, Mayorga C, Bogas G, Barrionuevo E, Fernandez-Santamaria R, Martin-Serrano A, et al. Epidemiology, mechanisms, and diagnosis of drug-induced anaphylaxis. Front Immunol. (2017) 8:614. doi: 10.3389/fimmu.2017.00614
50. Galli SJ. The mast cell-IgE paradox. Am J Pathol. (2016) 186:212–24. doi: 10.1016/j.ajpath.2015.07.025
51. van der Linden PWG, Hack CE, Poortman J, Vivié-Kipp YC, Struyvenberg A, van der Zwan JK. Insect-sting challenge in 138 patients: relation between clinical severity of anaphylaxis and mast cell activation. J Allergy Clin Immunol. (1992) 90:110–8. doi: 10.1016/S0091-6749(06)80017-5
52. Vadas P, Perelman B, Liss G. Platelet-activating factor, histamine, and tryptase levels in human anaphylaxis. J Allergy Clin Immunol. (2013) 131:144–9. doi: 10.1016/j.jaci.2012.08.016
53. Eberlein-Konig B, Ullmann S, Thomas P, Przybilla B. Tryptase and histamine release due to a sting challenge in bee venom allergic patients treated successfully or unsuccessfully with hyposensitization *. Clin Exp Allergy. (1995) 25:704–12. doi: 10.1111/j.1365-2222.1995.tb00007.x
54. Nuñez-Borque E, Fernandez-Bravo S, Yuste-Montalvo A, Esteban V. Pathophysiological, cellular, and molecular events of the vascular system in anaphylaxis. Front Immunol. (2022) 0:700. doi: 10.3389/fimmu.2022.836222
55. Akdis CA, Akdis M. Mechanisms of allergen-specific immunotherapy and immune tolerance to allergens. World Allergy Organ J. (2015) 8:17. doi: 10.1186/s40413-015-0063-2
56. Wachholz PA, Durham SR. Mechanisms of immunotherapy: IgG revisited. Curr Opin Allergy Clin Immunol. (2004) 4:313–8. doi: 10.1097/01.all.0000136753.35948.c0
57. Cheng LE, Hartmann K, Roers A, Krummel MF, Locksley RM. Perivascular mast cells dynamically probe cutaneous blood vessels to capture immunoglobulin E. Immunity. (2013) 38:166–75. doi: 10.1016/j.immuni.2012.09.022
58. Kunder CA, St John AL, Abraham SN. Mast cell modulation of the vascular and lymphatic endothelium. Blood. (2011) 118:5383–93. doi: 10.1182/blood-2011-07-358432
59. Abraham SN. St. John AL Mast cell-orchestrated immunity to pathogens. Nat Rev Immunol. (2010) 10:440–52. doi: 10.1038/nri2782
60. Krystel-Whittemore M, Dileepan KN, Wood JG. Mast cell: a multi-functional master cell. Front Immunol. (2016) 6:620. doi: 10.3389/fimmu.2015.00620
61. Yamanishi Y, Karasuyama H. Basophils and mast cells in immunity and inflammation. Semin Immunopathol. (2016) 38:535–7. doi: 10.1007/s00281-016-0582-0
62. Jiménez-Saiz R, Chu DK, Mandur TS, Walker TD, Gordon ME, Chaudhary R, et al. Lifelong memory responses perpetuate humoral TH2 immunity and anaphylaxis in food allergy. J Allergy Clin Immunol. (2017) 140:1604–15. doi: 10.1016/j.jaci.2017.01.018
63. Kubo S, Nakayama T, Matsuoka K, Yonekawa H, Karasuyama H. Long term maintenance of IgE-mediated memory in mast cells in the absence of detectable serum IgE. J Immunol. (2003) 170:775–80. doi: 10.4049/jimmunol.170.2.775
64. Khodoun MV, Strait R, Armstrong L, Yanase N, Finkelman FD. Identification of markers that distinguish IgE- from IgG-mediated anaphylaxis. Proc Natl Acad Sci. (2011) 108:12413–8. doi: 10.1073/pnas.1105695108
65. da Silva EZM, Jamur MC, Oliver C. Mast cell function: a new vision of an old cell. J Histochem Cytochem. (2014) 62:698–738. doi: 10.1369/0022155414545334
66. Ménasché G, Longé C, Bratti M, Blank U. Cytoskeletal transport, reorganization, and fusion regulation in mast cell-stimulus secretion coupling. Front Cell Dev Biol. (2021) 9:316. doi: 10.3389/fcell.2021.652077
67. Malaviya R, Uckun FM. Role of STAT6 in IgE receptor/FcεRI-mediated late phase allergic responses of mast cells. J Immunol. (2002) 168:421–6. doi: 10.4049/jimmunol.168.1.421
68. Khodoun MV, Morris SC, Angerman E, Potter C, Schuman R, Wunderlich M, et al. Rapid desensitization of humanized mice with anti-human FcεRIα monoclonal antibodies. J Allergy Clin Immunol. (2020) 145:907–21. doi: 10.1016/j.jaci.2019.12.003
69. Sancho-Serra M. del C, Simarro M, Castells M. Rapid IgE desensitization is antigen specific and impairs early and late mast cell responses targeting FcεRI internalization. Eur J Immunol. (2011) 41:1004–13. doi: 10.1002/eji.201040810
70. Oka T, Rios EJ, Tsai M, Kalesnikoff J, Galli SJ. Rapid desensitization induces internalization of antigen-specific IgE on mouse mast cells. J Allergy Clin Immunol. (2013) 132:922–32. doi: 10.1016/j.jaci.2013.05.004
71. Mendoza GR, Orner FB. Reversibility of IgE-mediated rat mast cell desensitization. Int Arch Allergy Immunol. (1982) 69:50–5. doi: 10.1159/000233145
72. Lewis A, MacGlashan DW, Suvarna SK, Peachell PT. Recovery from desensitization of IgE-dependent responses in human lung mast cells. Clin Exp Allergy. (2017) 47:1022–31. doi: 10.1111/cea.12912
73. Shalit M, Levi-Schaffer F. Challenge of mast cells with increasing amounts of antigen induces desensitization. Clin Exp Allergy. (1995) 25:896–902. doi: 10.1111/j.1365-2222.1995.tb00033.x
74. Gladys Ang WX, Church AM, Kulis M, Choi HW, Wesley Burks A, Abraham SN. Mast cell desensitization inhibits calcium flux and aberrantly remodels actin. J Clin Invest. (2016) 126:4103–18. doi: 10.1172/JCI87492
75. Nagata Y, Suzuki R. FcεRI cluster size determines effective mast cell desensitization without effector responses in vitro. Int Arch Allergy Immunol. (2022) 183:453–61. doi: 10.1159/000520132
76. Morales AR, Shah N, Castells M. Antigen-IgE desensitization in signal transducer and activator of transcription 6-deficient mast cells by suboptimal doses of antigen. Ann Allergy, Asthma Immunol. (2005) 94:575–80. doi: 10.1016/S1081-1206(10)61136-2
77. Sherman MA, Secor VH, Brown MA. IL-4 preferentially activates a novel STAT6 isoform in mast cells. J Immunol. (1999) 162:2703–8.
78. Navinés-Ferrer A, Ainsua-Enrich E, Serrano-Candelas E, Proaño-Pérez E, Muñoz-Cano R, Gastaminza G, et al. MYO1F regulates IgE and MRGPRX2-dependent mast cell exocytosis. J Immunol. (2021) 206:2277–89. doi: 10.4049/jimmunol.2001211
79. Dispenza MC, Krier-Burris RA, Chhiba KD, Undem BJ, Robida PA, Bochner BS. Bruton's tyrosine kinase inhibition effectively protects against human IgE-mediated anaphylaxis. J Clin Invest. (2020) 130:4759–70. doi: 10.1172/JCI138448
80. Uermösi C, Zabel F, Manolova V, Bauer M, Beerli RR, Senti G et al. IgG-mediated down-regulation of IgE bound to mast cells: a potential novel mechanism of allergen-specific desensitization. Allergy. (2014) 69:338–47. doi: 10.1111/all.12327
81. Castells MC, Klickstein LB, Hassani K, Cumplido JA, Lacouture ME, Austen KF et al. gp49B1-αvβ3 interaction inhibits antigen-induced mast cell activation. Nat Immunol. (2001) 2:436–42. doi: 10.1038/87749
82. Jin JJ Li JT, Klimek L, Pfaar O. Sublingual Immunotherapy Dosing Regimens: What Is Ideal? J Allergy Clin Immunol Pract. (2017) 5:1–10. doi: 10.1016/j.jaip.2016.09.027
83. Barber D, Rico P, Blanco C, Fernandez-Rivas M, Ibañez MD, Escribese MM. GRAZAX®: a sublingual immunotherapy vaccine for Hay fever treatment: from concept to commercialization. Hum Vaccin Immunother. (2019) 15:2887–95. doi: 10.1080/21645515.2019.1622976
84. Hoover H, Leatherman B, Ryan M, McMains K, Veling M. Evidence-based dosing of maintenance subcutaneous immunotherapy: a contemporary review of state-of-the-art practice. Int Forum Allergy Rhinol. (2018) 8:806–16. doi: 10.1002/alr.22118
85. Pitsios C. Allergen immunotherapy: biomarkers and clinical outcome measures. J Asthma Allergy. (2021) 14:141–8. doi: 10.2147/JAA.S267522
86. Moingeon P. Biomarkers for allergen immunotherapy: a ‘panoramic' view. Immunol Allergy Clin North Am. (2016) 36:161–79. doi: 10.1016/j.iac.2015.08.004
87. Kouser L, Kappen J, Walton RP, Shamji MH. Update on biomarkers to monitor clinical efficacy response during and post treatment in allergen immunotherapy. Curr Treat Options Allergy. (2017) 4:43–53. doi: 10.1007/s40521-017-0117-5
88. Breiteneder H, Peng YQ, Agache I, Diamant Z, Eiwegger T, Fokkens WJ et al. Biomarkers for diagnosis and prediction of therapy responses in allergic diseases and asthma. Allergy. (2020) 75:3039–68. doi: 10.1111/all.14582
89. Hardy LC, Smeekens JM, Kulis MD. Biomarkers in food allergy immunotherapy. Curr Allergy Asthma Rep. (2019) 19:61. doi: 10.1007/s11882-019-0894-y
90. Pfaar O, Bonini S, Cardona V, Demoly P, Jakob T, Jutel M et al. Perspectives in allergen immunotherapy: 2017 and beyond. Allergy. (2018) 73:5–23. doi: 10.1111/all.13355
91. Shamji MH, Kappen JH, Akdis M, Jensen-Jarolim E, Knol EF, Kleine-Tebbe J, et al. Biomarkers for monitoring clinical efficacy of allergen immunotherapy for allergic rhinoconjunctivitis and allergic asthma: an EAACI position paper. Allergy. (2017) 72:1156–73. doi: 10.1111/all.13138
92. Agache I, Bilò M, Braunstahl GJ, Delgado L, Demoly P, Eigenmann P, et al. In vivo diagnosis of allergic diseases–allergen provocation tests. Allergy. (2015) 70:355–65. doi: 10.1111/all.12586
93. Nolte H, Maloney J, Nelson HS, Bernstein DI, Lu S, Li Z, et al. Onset and dose-related efficacy of house dust mite sublingual immunotherapy tablets in an environmental exposure chamber. J Allergy Clin Immunol. (2015) 135:1494–501. doi: 10.1016/j.jaci.2014.12.1911
94. Horak F, Zieglmayer P, Zieglmayer R, Lemell P, Devillier P, Montagut A, et al. Early onset of action of a 5-grass-pollen 300-IR sublingual immunotherapy tablet evaluated in an allergen challenge chamber. J Allergy Clin Immunol. (2009) 124:471–7. doi: 10.1016/j.jaci.2009.06.006
95. Shamji MH, Ljørring C, Francis JNA, Calderon M, Larché M, Kimber I, et al. Functional rather than immunoreactive levels of IgG4 correlate closely with clinical response to grass pollen immunotherapy. Allergy. (2012) 67:217–26. doi: 10.1111/j.1398-9995.2011.02745.x
96. Pilette C, Nouri-Aria KT, Jacobson MR, Wilcock LK, Detry B, Walker SM, et al. Grass pollen immunotherapy induces an allergen-specific IgA2 antibody response associated with mucosal TGF-β expression. J Immunol. (2007) 178:4658–66. doi: 10.4049/jimmunol.178.7.4658
97. Gueguen C, Bouley J, Moussu H, Luce S, Duchateau M, Chamot-Rooke J, et al. Changes in markers associated with dendritic cells driving the differentiation of either TH2 cells or regulatory T cells correlate with clinical benefit during allergen immunotherapy. J Allergy Clin Immunol. (2016) 137:545–58. doi: 10.1016/j.jaci.2015.09.015
98. Golebski K, Layhadi JA, Sahiner U, Steveling-Klein EH, Lenormand MM, Li RCY et al. Induction of IL-10-producing type 2 innate lymphoid cells by allergen immunotherapy is associated with clinical response. Immunity. (2021) 54:291–307. doi: 10.1016/j.immuni.2020.12.013
99. Shamji MH, Layhadi JA, Scadding GW, Cheung DKM, Calderon MA, Turka LA, et al. Basophil expression of diamine oxidase: a novel biomarker of allergen immunotherapy response. J Allergy Clin Immunol. (2015) 135:913–21. doi: 10.1016/j.jaci.2014.09.049
100. Caruso M, Cibella F, Emma R, Campagna D, Tringali G, Amaradio MD et al. Basophil biomarkers as useful predictors for sublingual immunotherapy in allergic rhinitis. Int Immunopharmacol. (2018) 60:50–8. doi: 10.1016/j.intimp.2018.04.034
101. Tsai M, Mukai K, Chinthrajah RS, Nadeau KC, Galli SJ. Sustained successful peanut oral immunotherapy associated with low basophil activation and peanut-specific IgE. J Allergy Clin Immunol. (2020) 145:885–96. doi: 10.1016/j.jaci.2019.10.038
102. Palaniyandi S, Watanabe K, Ma M, Tachikawa H, Kodama M, Aizawa Y. Inhibition of mast cells by interleukin-10 gene transfer contributes to protection against acute myocarditis in rats. Eur J Immunol. (2004) 34:3508–15. doi: 10.1002/eji.200425147
103. Cosmi L, Santarlasci V, Angeli R, Liotta F, Maggi L, Frosali F, et al. Sublingual immunotherapy with Dermatophagoides monomeric allergoid down-regulates allergen-specific immunoglobulin E and increases both interferon-γ- and interleukin-10-production. Clin Exp Allergy. (2006) 36:261–72. doi: 10.1111/j.1365-2222.2006.02429.x
104. Ebner C, Siemann U, Bohle B, Willheim M, Wiedermann U, Schenk S, et al. Immunological changes during specific immunotherapy of grass pollen allergy: reduced lymphoproliferative responses to allergen and shift from TH2 to TH1 in T-cell clones specific for Phi p 1, a major grass pollen allergen. Clin Exp Allergy. (1997) 27:1007–15. doi: 10.1111/j.1365-2222.1997.tb01252.x
105. Bohle B, Kinaciyan T, Gerstmayr M, Radakovics A, Jahn-Schmid B, Ebner C. Sublingual immunotherapy induces IL-10-producing T regulatory cells, allergen-specific T-cell tolerance, and immune deviation. J Allergy Clin Immunol. (2007) 120:707–13. doi: 10.1016/j.jaci.2007.06.013
106. Zhu Z, Lee PH, Chaffin MD, Chung W, Loh P-R, Lu Q, et al. A genome-wide cross-trait analysis from UK Biobank highlights the shared genetic architecture of asthma and allergic diseases. Nat Genet. (2018) 50:857–64. doi: 10.1038/s41588-018-0121-0
107. Mondoulet L, Dioszeghy V, Busato F, Plaquet C, Dhelft V, Bethune K, et al. Gata3 hypermethylation and Foxp3 hypomethylation are associated with sustained protection and bystander effect following epicutaneous immunotherapy in peanut-sensitized mice. Allergy. (2019) 74:152–64. doi: 10.1111/all.13479
108. Hwang SS, Jang SW, Lee KO, Kim HS, Lee GR. RHS6 coordinately regulates the Th2 cytokine genes by recruiting GATA3, SATB1, and IRF4. Allergy. (2017) 72:772–82. doi: 10.1111/all.13078
109. Croote D, Darmanis S, Nadeau KC, Quake SR. High-affinity allergen-specific human antibodies cloned from single IgE B cell transcriptomes. Science (80-). (2018) 362:1306–9. doi: 10.1126/science.aau2599
110. Zheng P, Bian X, Zhai Y, Li C, Li N, Hao C et al. Metabolomics reveals a correlation between hydroxyeicosatetraenoic acids and allergic asthma: evidence from 3 years' immunotherapy. Pediatr Allergy Immunol. (2021) 32:1654–62. doi: 10.1111/pai.13569
111. Ohnmacht C, Park JH, Cording S, Wing JB, Atarashi K, Obata Y, et al. The microbiota regulates type 2 immunity through RORγt+ T cells. Science (80-). (2015) 349:989–93. doi: 10.1126/science.aac4263
112. Tsai YG, Yang KD, Niu DM, Chien JW, Lin CY. TLR2 Agonists enhance CD8 + Foxp3 + regulatory T cells and suppress Th2 immune responses during allergen immunotherapy. J Immunol. (2010) 184:7229–37. doi: 10.4049/jimmunol.1000083
113. Lam HY, Tergaonkar V, Ahn KS. Mechanisms of allergen-specific immunotherapy for allergic rhinitis and food allergies. Biosci Rep. (2020) 40:256. doi: 10.1042/BSR20200256
114. James LK, Shamji MH, Walker SM, Wilson DR, Wachholz PA, Francis JN, et al. Long-term tolerance after allergen immunotherapy is accompanied by selective persistence of blocking antibodies. J Allergy Clin Immunol. (2011) 127:509–16. doi: 10.1016/j.jaci.2010.12.1080
115. Knol EF, Mul FPJ, Jansen H, Calafat J, Roos D. Monitoring human basophil activation via CD63 monoclonal antibody 435. J Allergy Clin Immunol. (1991) 88:328–38. doi: 10.1016/0091-6749(91)90094-5
116. Patil SU, Steinbrecher J, Calatroni A, Smith N, Ma A, Ruiter B, et al. Early decrease in basophil sensitivity to Ara h 2 precedes sustained unresponsiveness after peanut oral immunotherapy. J Allergy Clin Immunol. (2019) 144:1310–9. doi: 10.1016/j.jaci.2019.07.028
117. Barber D, Escribese MM. Predictive biomarkers in allergen specific immunotherapy. Allergol Immunopathol. (2017) 45:12–4. doi: 10.1016/j.aller.2017.09.003
118. Irvine AD, McLean WHI, Leung DYM. Filaggrin mutations associated with skin and allergic diseases. N Engl J Med. (2011) 365:1315–27. doi: 10.1056/NEJMra1011040
119. Kim KW, Ober C. Lessons learned from GWAS of asthma. Allergy Asthma Immunol Res. (2019) 11:170. doi: 10.4168/aair.2019.11.2.170
120. Bunning BJ, DeKruyff RH, Nadeau KC. Epigenetic changes during food-specific immunotherapy. Curr Allergy Asthma Rep. (2016) 16:87. doi: 10.1007/s11882-016-0665-y
121. Sindher SB, Long A, Acharya S, Sampath V, Nadeau KC. The use of biomarkers to predict aero-allergen and food immunotherapy responses. Clin Rev Allergy Immunol. (2018) 55:190–204. doi: 10.1007/s12016-018-8678-z
122. Waldron R, McGowan J, Gordon N, McCarthy C, Mitchell EB, Fitzpatrick DA. Proteome and allergenome of the European house dust mite dermatophagoides pteronyssinus. PLoS ONE. (2019) 14:e0216171. doi: 10.1371/journal.pone.0216171
123. Glesner J, Filep S, Vailes LD, Wünschmann S, Chapman MD, Birrueta G, et al. Allergen content in German cockroach extracts and sensitization profiles to a new expanded set of cockroach allergens determine in vitro extract potency for IgE reactivity. J Allergy Clin Immunol. (2019) 143:1474–81. doi: 10.1016/j.jaci.2018.07.036
124. Ihara F, Sakurai D, Yonekura S, Iinuma T, Yagi R, Sakurai T, et al. Identification of specifically reduced Th2 cell subsets in allergic rhinitis patients after sublingual immunotherapy. Allergy. (2018) 73:1823–32. doi: 10.1111/all.13436
125. Durham SR, Emminger W, Kapp A, de Monchy JGR, Rak S, Scadding GK et al. SQ-standardized sublingual grass immunotherapy: confirmation of disease modification 2 years after 3 years of treatment in a randomized trial. J Allergy Clin Immunol. (2012) 129:717–25.e5. doi: 10.1016/j.jaci.2011.12.973
126. Jiménez-Saiz R, Ellenbogen Y, Koenig JFE, Gordon ME, Walker TD, Rosace D, et al. IgG1 + B-cell immunity predates IgE responses in epicutaneous sensitization to foods. Allergy. (2019) 74:165–75. doi: 10.1111/all.13481
Keywords: allergen immunotherapy, mast cell, desensitization, Treg, anaphylaxis, IgE
Citation: López-Sanz C, Jiménez-Saiz R, Esteban V, Delgado-Dolset MI, Perales-Chorda C, Villaseñor A, Barber D and Escribese MM (2022) Mast Cell Desensitization in Allergen Immunotherapy. Front. Allergy 3:898494. doi: 10.3389/falgy.2022.898494
Received: 17 March 2022; Accepted: 16 May 2022;
Published: 16 June 2022.
Edited by:
Linda Cox, Wyoming, United StatesReviewed by:
Mariana C. Castells, Brigham and Women's Hospital and Harvard Medical School, United StatesCopyright © 2022 López-Sanz, Jiménez-Saiz, Esteban, Delgado-Dolset, Perales-Chorda, Villaseñor, Barber and Escribese. This is an open-access article distributed under the terms of the Creative Commons Attribution License (CC BY). The use, distribution or reproduction in other forums is permitted, provided the original author(s) and the copyright owner(s) are credited and that the original publication in this journal is cited, in accordance with accepted academic practice. No use, distribution or reproduction is permitted which does not comply with these terms.
*Correspondence: María M. Escribese, bWFyaWFtYXJ0YS5lc2NyaWJlc2VhbG9uc29AY2V1LmVz
Disclaimer: All claims expressed in this article are solely those of the authors and do not necessarily represent those of their affiliated organizations, or those of the publisher, the editors and the reviewers. Any product that may be evaluated in this article or claim that may be made by its manufacturer is not guaranteed or endorsed by the publisher.
Research integrity at Frontiers
Learn more about the work of our research integrity team to safeguard the quality of each article we publish.