- 1Respiratory Department, Children's Hospital, Córdoba, Argentina
- 2School of Health Sciences, Catholic University of Salta, Salta, Argentina
Bronchiolitis is a virus-associated infection of the lower respiratory tract exhibiting signs and symptoms of airway obstruction. Respiratory Syncytial Virus (RSV) is responsible in most cases; however, different rhinoviruses have also been implicated. Specific viruses and time until the first infection, severity of the respiratory condition, and atopic status have a determinant role in the recurrence of wheezing and asthma development. Genetics, lung function, atopic condition, the role of microbiota and environment, pollution, and obesity are considered in the present review. Emergency room visits and hospitalizations because of severe wheezing and smoking during pregnancy among others were identified as risk factors for significant morbidity in our population. Approaching determinant conditions like genetics, allergy, antiviral immunity, and environmental exposures such as farm vs. urban and viral virulence provides an opportunity to minimize morbidity of viral illness and asthma in children.
Introduction
Bronchiolitis is described as the first phase of low respiratory tract infection (LRTI) in children under the age of 2 years with viral etiology and is clinically expressed by peripheral airway obstruction (cough, rales, and/or wheezing) (1). It predominates in autumn-winter months, with Human Respiratory Syncytial Virus (HRSV) being the most constant etiological agent, behind 60–80% of bronchiolitis (2). Data from Argentina reported that HRSV accounted for 81.3% in patients hospitalized for LRI, with a fatality rate of 1.7% (3, 4). New molecular techniques have amplified the knowledge of human rhinoviruses (HRV) A, B, and C which have joined the list of agents responsible for lower respiratory infection, including bronchiolitis (5) and pneumonia (6) besides their role of inducers of wheezing and asthma in childhood (7). Bronchiolitis is much more common between 3 and 5 months of age. Less than 3% of infants without risk factors require hospitalization and among them, mortality is <1%. In Argentina, lower respiratory infections (LRIs) are the third most frequent cause of death in infants within their first year (8), and recent data evidenced its growing incidence in emergency room visits in this population (9).
Recurrent wheezing corresponds to three or more incidents within twelve consecutive months. Mostly, the first event happens within the first year (10). In consequence, identification of risk factors for recurrent and/or persistent wheezing in infants is mandatory.
Significant association between LRTI and wheezing in infants has been reported in the EISL study (11), but also with asthma development (12). We identified the need for emergency room visits because of the perception of severe wheezing by parents, hospitalizations, and frequent night time awakening because of wheezing are variables significantly associated with recurrent wheezing (RW); and smoking during pregnancy, emergency room visits because of severe wheezing, and inhaled corticosteroids prescription were associated with severe wheezing (SW) more than six episodes in the first year of life (13). The presence of bronchiolitis in the first 3 months of life was found to be related to both RW and SW, and having had pneumonia was associated with occasional wheezing (OW) and RW.
Around half of infants hospitalized because of bronchiolitis will develop childhood asthma (14, 15). Asthma is a chronic bronchopulmonary disease characterized by bronchoconstriction and airway inflammation, and is among the top five pediatric conditions in the USA (16). In our population, the prevalence of current asthma symptoms was 16% in 6–7-year-old children (17), and recurrent wheezing was 18.9% (13). While diagnosing asthma in the first year of life is difficult, we have to highlight the need of risk factor identification particularly on those patients suffering bronchiolitis.
New evidence hints that environmental factors may alter genetic background within the first 3 months of life (18, 19). Compared to children born vaginally, those born by C-section have less diverse intestinal microbiota in their first 2 years of life. The development of the immunological system is deeply influenced by intestinal microbiota bacteria, and its alteration has been linked to the development of asthma (20). Not only microbiota but several variables have a role for developing asthma in childhood, such as first degree familiar history of asthma, premature birth, low birth weight, early weaning, few siblings in the household, attending kindergarten, exposure to cigarette smoke during pregnancy, low socioeconomic status, and low parental level of education (21, 22) (Figure 1).
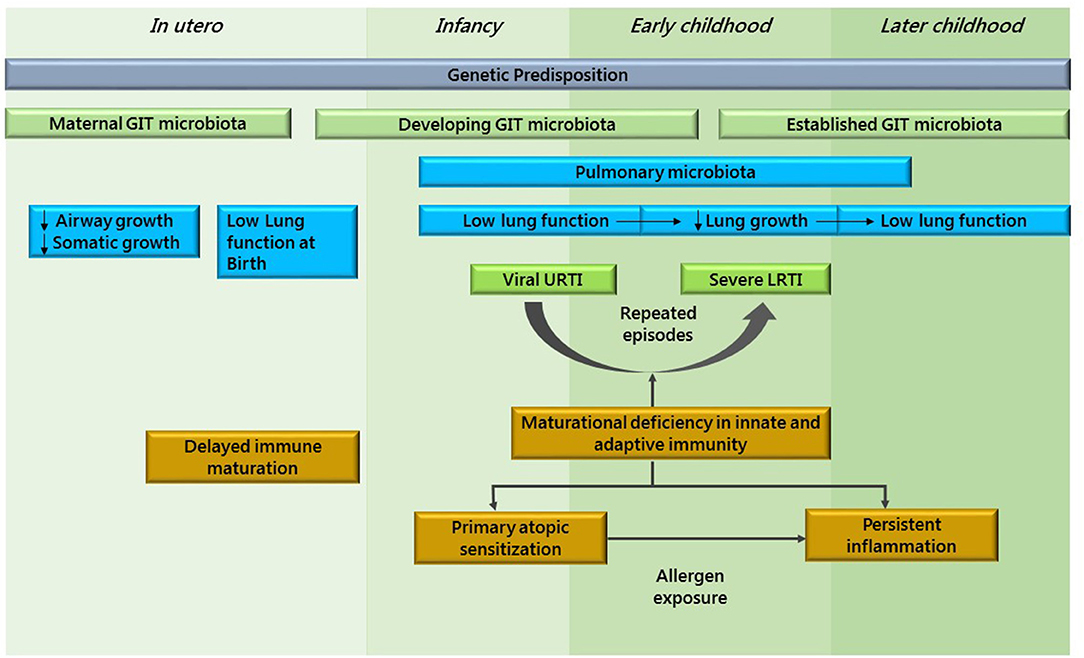
Figure 1. This figure shows asthma as a developmental disease. Adapted from Holgate et al. (23).
Taking into account that pre-commitment of the airways may underlie infection, different lines of research aiming to identify risk factors in preschool children who develop asthma are considered.
Viral Infection (RSV and RV) and Atopy
Specific viruses and time until the first infection, severity of the respiratory condition and atopic condition of child are determinants. An early onset wheezing episode induced by RV, RSV, or other viruses increases the risk for asthma at the age of 17–20 years compared to controls without wheezing history (24).
Then, is the bronchiolitis and asthma relationship cause or coincidence? The COAST study linked allergic sensitization within the first year of life with virus-associated wheezing, but the opposite is not true (25). It seems that the two assumptions of bronchiolitis as a cause or as a marker of asthma are not mutually exclusive. RSV infections usually occur in epidemic outbreaks around winter, producing a cytopathic effect on the epithelial airway in children younger than 3 months who characteristically require hospitalization (26). On the opposite side, rhinovirus infections are present throughout the year, involving older outpatient children with a frequent family history of asthma and/or atopy (27, 28). While rhinovirus bronchiolitis appears as a risk marker for asthma and atopy, RSV bronchiolitis may act as a cause, mostly in severe cases requiring hospitalization (29, 30).
The RSV Bronchiolitis in Early Life (RBEL) study reinforces the association between severe RSV bronchiolitis and incidental asthma: in 206 hospitalized infants, half received an asthma diagnosis by the age of 7 years (31).
Rhinovirus A is the most prevalent etiologic virus in acute bronchiolitis; and respiratory viruses such as the rhinovirus family are also associated with both bronchiolitis and the potential development of asthma (32, 33). Other studies show that preschoolers with early viral wheezing who have been referred to a pediatric hospital due to severe symptoms will have a higher risk of asthma between the ages of 5 and 10 (34).
Other studies found that rhinovirus C infection was related to the highest risk of RW, particularly on those with IgE sensitization during infancy. Additionally, these data suggest that RW resulted in asthma at the age of 4 years, while another study showed that rhinovirus infections significantly predicted RW in a group of infants with bronchiolitis under study for 3 years (35).
Bronchial epithelial cells are pre-committed to a type-2 (E2)-like phenotype in atopic patients. Once activated, their epithelial alarmins TSLP, IL-31, CCL-26, IL-25, and IL-33 induce a local inflammatory type-2 response with the characteristic cytokines IL-4, IL-5, IL-9, IL-13, IL-25, IL-33, and the increase of eosinophils (36). Allergen-specific immunotherapy, the most personalized treatment for allergic conditions, induces some kind of local gene expression footprint in the lower airways, which can be explained by multiple regulatory pathways that go beyond the scope of this review (37).
Rhinoviruses affect both airway epithelial cells and macrophages and activate them, with differences in the innate immune response between infants who develop asthma compared to non-atopic children (38). Deficiencies in innate cytokine production (e.g., interferons) were associated with the subsequent risk of wheezing (39). Several reports evidence recurrent wheezing following RSV bronchiolitis, and a significant number of infants with preschool RW will maintain their wheezing condition into adolescence (40).
Additional recent findings describe the role of RV infection in children with acute bronchiolitis, its impact on subsequent asthma development, and the implication in clinical practice (41), while familial history of atopy and RSV-hRV co-infection are risk factors for recurrent bronchial obstruction and sensitization (42).
A different phenotype compared to those who transiently wheeze seems evident. There are several reports correlating viral infection, atopy, and genetic burden. Then, we might consider all these variables.
Asthma Predictive Index
It is widely proclaimed that ~80% of asthmatic children present with symptoms in the first few years of life, whereas about 30% of early wheezers will develop physician's diagnosed asthma (43). Among the leading representative index is the Asthma Predictive Index (API), which was derived from the Tucson's Children's Respiratory Study (TCRS) (44). According to their established criteria, diagnosis of asthma is predicted by recurrent wheezing episodes more than four times previously plus one of the two major criteria (physician-diagnosed eczema or parental asthma), or two of the three minor criteria (physician-diagnosed allergic rhinitis, wheezing without cold, or peripheral eosinophilia concentration not <4%). Positive API values were highly predictive of current asthma between the ages of 6 and 13 years (as high as 77%), and negative values discarded asthma as a cause in 68% of cases. Specificity is as high as 97%, but its sensitivity was low (15–22%), which is consistent with new asthma in adulthood having had minimal wheezing during childhood. Adopting the “loose index” (at least one wheezing episode during the first 3 years, without changing other criteria), sensitivity would increase up to 57%, although several patients who would not become asthmatic later in life (i.e., transient-early wheezers) may be misclassified as positive, potentially receiving unnecessary treatment (45). Then, API was modified according to the Prevention of Early Asthma in Kids study: more than one inhalant allergen sensitization was added as a major criterion, and food allergen sensitization (milk, eggs, and peanuts) were substituted for physician-diagnosed allergic rhinitis (46). Only the API derived from the TCRS has a supporting publication to date; when it was applied to the Colombian population, it showed a sensitivity of 43% and a specificity of 79% after a 6-year follow up period (47). In consequence, guidelines like the Global Initiative for Asthma recommend consideration of API when controller medication is prescribed in preschool wheezers (48).
Perhaps the greatest risk factor is familiar asthma; then, the role of 17q21 variants in the development of HRV wheezing illnesses during early childhood was evaluated in a study published in 2013, showing the effects of (17q21 with ORMDL3) genotype on having asthma in a subgroup of children with early onset HRV wheezing. These results emphasize the importance of the joint effects of genetic and environmental conditions in the mechanisms underlying complex diseases (49).
Relationship Between Infection and Lung Microbiota
Colonization of the upper respiratory airways occurs directly after child birth, and quickly develops particular profiles during their first weeks (50–53). Various cross-sectional and case-control studies described differences in respiratory microbial profiles between children with mild, moderate, and severe respiratory syncytial virus infection (54). Early colonization at birth and the development of URT microbiota during the first months of life may directly influence respiratory health (55). Hence this study (56) provided data that quick microbiota maturation is associated with microbiota instability and also with the number of RTIs along the first year. These changing processes could be seen as early as within the first month of age (i.e., before the first RTI episodes). Then, we could link the impact of known important factors, such as birth mode, feeding regimes, siblings in family, early day care attendance, and microbiota alteration because of antibiotics with susceptibility to RTIs.
Searching for which specific microbes may provide protection has allowed us to identify wide families of species within microbial taxa that may explain the effect of the farming environment. Badellino et al. observed that among Argentinean teenagers, current rural residence and rural residence for over 5 years resulted in diminished odds of current wheezing and allergic rhino-conjunctivitis. These potential protective effects on the presence of atopy and asthma may be explained by “the farm protective effect” (57). While exposure to cowsheds and consumption of unprocessed cow's milk seems to be the main pillars for protection, the diversity of exposure (across gut, airways, and/or skin) and across multiple populations, altogether seems to contribute to the whole effect (58).
Relationship of Wheezing With Lung Function and Airway Hyper-Responsiveness
Few studies have evaluated lung function in young children (maximal forced expiratory flow at functional residual capacity [V'max FRC]) by rapid thoracic compression in the TCRS (59) and sRaw by the forced oscillation technique in the MAAS (60), while many studies have not evaluated lung function until children reached school-age. V'max FRC was found to be reduced in recurrent wheezers who started wheezing within the first year of life, and when measured before 6 months of age wheezing was reduced only in transient-early but not in persistent wheezers. A progressive decrease in lung function seems to precede the onset of wheezing, and may not be entirely attributable to a sequelae of respiratory diseases. However, this needs to be confirmed by prospective cohort studies.
While transient-early wheezers (but not late-onset wheezers) display decreased lung function early in age, late-onset wheezers (but not transient-early wheezers) have increased airway hyper-responsiveness. And in persistent wheezers, lung function tends to decrease as measured after the age of 6 years, which is indicative of airway remodeling, a long-term unwanted complication in asthmatic patients. In utero and early life, conditions must be addressed in order to gain confidence around these statements (61, 62).
Gender and Familiar Influence
Having maternal asthma history evidenced a risk factor for wheezing in boys (OR = 1.79, 1.29–2.48, p = 0.0004) and girls (OR = 2.05, 95% CI 1.44–2.91, p < 0.0001), with a similar effect on wheezing throughout the years. Paternal asthma (OR = 1.83, 1.38–2.57, p = 0.0005) and infant bronchiolitis (OR = 2.15, 1.47–3.14, p < 0.0001) have been associated with boys only, with no differences at all ages (63). Another Canadian study coordinated by Tse et al. described that maternal asthma, infant bronchiolitis, and atopic dermatitis were all significantly linked with persistent wheezing in both genders, however paternal asthma was a risk factor for persistent wheezing in boys only (OR = 4.27, 95% CI 2.33, 7.83, p for sex by paternal asthma interaction = 0.02), while being Black or Hispanic was associated with wheezing only in girls (64).
Air Pollution
Indoor and outdoor pollution has increased side by side with urbanization and population growth and has become an important contributing factor for the incidence and exacerbation of asthma, with particular emphasis in the developing world (65, 66). Pollutants associated with asthma exacerbations are ozone, nitrogen dioxide, volatile organic compounds, particulate matter (PM10 and PM2.5), and traffic-related air pollution, containing vehicles exhaust fumes and non-combustion particles. These pollutants provoke epithelial damage, activate oxidative stress with consequent airway inflammation (67, 68), and reduce inhibitory T-Reg function (69). These immune inflammatory mechanisms have been proposed to influence asthma development and contribute to augmented respiratory sensitization to inhalant allergens (70) and enhanced airway hyper-responsiveness and remodeling (71). It has also been described that exposure to pollutants prenatally might confer risk to development of postnatal asthma (72), interacting with glutathione S-transferases, and promoting TNF at a genetic level as well (73), influencing gene function through the promotion of methylation and histone acetylation (74).
Tobacco Smoke
As a result of mixed compounds including nitrogen dioxide and several volatile organic elements, tobacco smoke has been implicated as a remarkable independent risk factor for asthma incidence (75, 76) partially acting as a T2-type response enhancer (77). Also, grand-maternal smoking during pregnancy elevated the risk for asthma development over the second generation (78) through intrauterine epigenetic mechanisms involved in asthma causation. In our study we found that SHS was significantly related to more frequent wheezing, particularly when the mother was a smoker (OR = 2.7; IC 95%: 1.4–5.18; p = 0.0009) and if she smoked during pregnancy (OR = 4; IC 95%: 1.8–8.5; p = 0.0001). In our children, second-hand tobacco smoke was related to the need for emergency room visits because of wheezing (40.87%, p = 0.0056), representing a significant risk factor for the presence and severity of it (79).
Obesity
Obesity is strongly associated with the incidence and severity of asthma (80) but also with maternal obesity history, and elevated gestational weight gain was associated with an increased risk of asthma in children, particularly in non-asthmatic mothers (81). Two cohort studies, Tucson and ALSPAC, have found an association between obesity and asthma in adults when they had asthma at 8 years (82, 83). A combination of minimal persistent inflammation, mechanical, metabolic, and hormonal factors might explain these associations. A chronic inflammatory pathway was linked with obesity, presenting increased levels of inflammatory cytokines, like TNF, IL-1, and IL-6 (produced by macrophages) and adipokines like leptin, chemerin, and adiponectin (produced by adipocytes), altogether inhibiting cell proliferation and causing tissue damage. Leptin has a key role on the differentiation of lipo-fibroblasts and controls pulmonary surfactant synthesis in fetal lungs (84). In mouse models, leptin infusion increased airway hyper-responsiveness, enhanced IgE levels as a result of allergen challenge (85), and elevated levels of IL-6 in the airways (86). Elevated maternal levels of leptin favor a low-grade inflammatory status, contributing to the postnatal risk of asthma (87).
However, the exact mechanism linking obesity and asthma still remains to be fully defined.
Virome
Nowadays, multiple omic and molecular techniques such as metagenomic DNA sequencing is unveiling the virome in human airways. In asthmatic airways, particular microbial colonization may condition not only asthma development but also exacerbations (88).
Anelloviruses (AVs) are present during early life and seemingly do not cause harm to the host. Even though their role in the development of asthma is elusive, an increase in viral load has been implicated in acute respiratory disease, exacerbated chronic lung disease, lower CD3+ and CD4+ T cell numbers, and higher B cell and eosinophilic counts (89, 90).
Besides AVs, Caudovirales and Picornaviridae have been described in asthmatic children. Nevertheless, equilibrium of this core virome and bacteriophages seems to be necessary against asthma symptoms (91). More research in the field is mandatory.
Conclusions and Future Perspectives
Several interventional options have emerged to control the recurrence and severity of viral respiratory diseases, and hopefully the incidence and exacerbations of asthma (Figure 2).
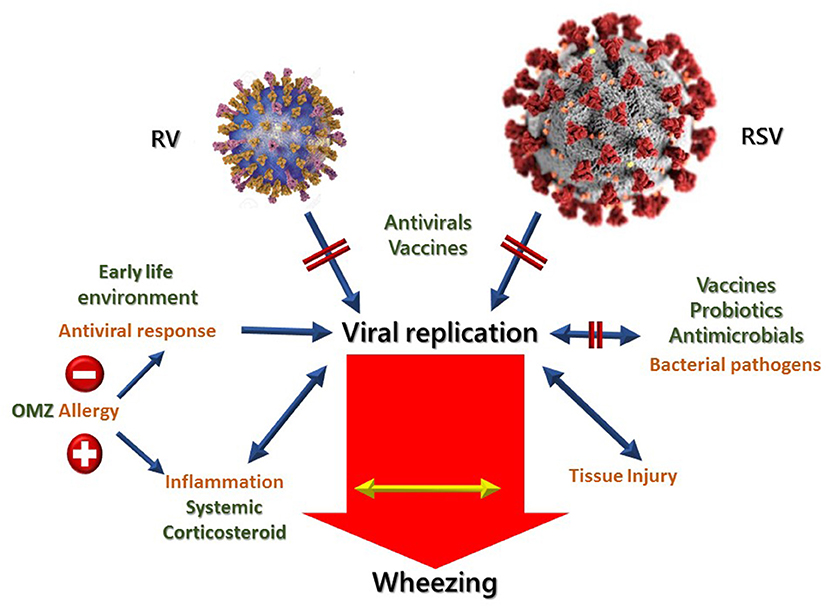
Figure 2. Possibilities of treating and preventing viral induced wheezing diseases. Opportunities for intervention are marked in red. OMZ, Omalizumab. Adapted from Jartti et al. (92).
The search should focus on effective and safe antivirals and vaccines for RV and RSV, where candidates are currently being investigated in clinical trials (93). Atopic condition has been described both as a frequent condition and also as a risk factor for asthma and wheezing. Through blocking lgE with monoclonal antibody omalizumab, it is possible to ablate antigen signaling through IgE-related mechanisms and re-establish IFN-a response to rhinovirus, reducing asthma exacerbations in viral season as well. In this regard, the PARK study is an ongoing double-blind, placebo-controlled trial with omalizumab in 2–3-year-old infants with a high risk of asthma (94).
Another strategy under evaluation in high-risk young children, in order to prevent wheezing condition and asthma incidence, is the oral administration of bacterial lysates (95).
Finally, therapeutical interventions able to reduce or stop allergies may strengthen antiviral pathways with consequent diminished inflammatory mechanisms responsible for airway obstruction and the remodeling process. It is mandatory to establish a global approach involving personal risk factors like genetics, allergic sensitization, and innate immune system status, together with environmental pollutants and microbiota, in order to lessen viral respiratory morbidity and childhood asthma.
Author Contributions
All authors listed have made a substantial, direct and intellectual contribution to the work, and approved it for publication.
Conflict of Interest
The authors declare that the research was conducted in the absence of any commercial or financial relationships that could be construed as a potential conflict of interest.
Publisher's Note
All claims expressed in this article are solely those of the authors and do not necessarily represent those of their affiliated organizations, or those of the publisher, the editors and the reviewers. Any product that may be evaluated in this article, or claim that may be made by its manufacturer, is not guaranteed or endorsed by the publisher.
References
1. Megalaa R, Perez GF, Kilaikode-Cheruveettara S, Kotwal N, Rodriguez-Martinez CE, Nino G. Clinical definition of respiratory viral infections in young children and potential bronchiolitis misclassification. J Investig Med. (2018) 66:46–51. doi: 10.1136/jim-2017-000491
2. Giubergia V, Martinchuk G, Moreno N, Colombres G, Parra L, Viale D, Murtagh P, et al. Gravedad en la infección por virus sincicial respiratorio en pacientes con factores de riesgo y sin ellos. Arch Argent Pediatr. (2004) 102:330–4.
3. Weissenbacher M, Carballal G, Avila M, Salomón H, Harisiadi J, Catalano M, et al. Etiologic and clinical evaluation of acute lower respiratory tract infections in young Argentinian children: an overview. Rev Infect Dis. (1990) 12:s889–98. doi: 10.1093/clinids/12.Supplement_8.S889
4. Charlton CL, Babady E, Ginocchio CC, Hatchette TF, Jerris RC, Li Y, et al. Practical guidance for clinical microbiology laboratories: viruses causing acute respiratory tract infections. Clin Microbiol Rev. (2018) 32:e00042–18. doi: 10.1128/CMR.00042-18
5. Lukkarinen M, Koistinen A, Turunen R, Lehtinen P, Vuorinen T, Jartti T. Rhinovirus-induced first wheezing episode predicts atopic but not nonatopic asthma at school age. J Allergy Clin Immunol. (2017) 140:988–95. doi: 10.1016/j.jaci.2016.12.991
6. Greenberg SB. Update on human rhinovirus and coronavirus infections. Semin Respir Crit Care Med. (2016) 37:555–71. doi: 10.1055/s-0036-1584797
7. Turunen R, Jartti T, Bochkov YA, Gern JE, Vuorinen T. Rhinovirus species and clinical characteristics in the first wheezing episode in children. J Med Virol. (2016) 88:2059– 68. doi: 10.1002/jmv.24587
8. Ministerio de Salud. Boletín Integrado de Vigilancia. (2020) (481) SEO2. Available online at: https://www.argentina.gob.ar/sites/default/files/biv_481_edicion_ampliada.pdf
9. Vinelli NF, Mannucci C, Laba NI, del Vecchio L, Valerio A, Lago MI, et al. Consultas no urgentes al Departamento de Urgencias de un Hospital pediátrico. Arch Argent Pediatr. (2011) 109:8–13. doi: 10.1590/S0325-00752011000100004
10. Oostenbrink R, Jansingh-Piepers EM, Raat H, Nuijsink M, Landgraf JM, Essink-Bot ML, et al. Health-related quality of life of pre-school children with wheezing illness. Pediatr Pulmonol. (2006) 41:993–1000. doi: 10.1002/ppul.20486
11. Garcia-Marcos L, Mallol J, Solé D, Brand PL; EISL Study Group. International study of wheezing in infants: risk factors in affluent and non-affluent countries during the first year of life. Pediatr Allergy Immunol. (2010) 21:878–88. doi: 10.1111/j.1399-3038.2010.01035.x
12. Holt PG, Rowe J, Kusel M, Parsons F, Hollams EM, Bosco A, et al. Toward improved prediction of risk for atopy and asthma among preschoolers: a prospective cohort study. J Allergy Clin Immunol. (2010) 125:653–9:659.e1–659.e7. doi: 10.1016/j.jaci.2009.12.018
13. Neffen H, Teijeiro A. Available online at: http://globalasthmareport.org/resources/Global_Asthma_Report_2018.pdf (accessed June 12, 2021)
14. Teijeiro A, Badellino B MG. Raiden, MN Cuello. Risk factors for recurrent wheezing in the first year of life in the city of Córdoba, Argentina. Allergol Immunopathol. (2017) 45:234–9. doi: 10.1016/j.aller.2016.08.009
15. Balekian DS, Linnemann RW, Hasegawa K, Thadhani R, Camargo CA Jr. Cohort study of severe bronchiolitis during infancy and risk of asthma by age5 years. J Allergy Clin Immunol Pract. (2017) 5:92–6. doi: 10.1016/j.jaip.2016.07.004
16. Carroll KN, Wu P, Gebretsadik T, Griffin MR, Dupont WD, Mitchel EF, et al. The severity-dependent relationship of infant bronchiolitis on the risk and morbidity of early childhood asthma. J Allergy Clin Immunol. (2009) 123:1055–61, (1061) e1. doi: 10.1016/j.jaci.2009.02.021
17. Soni A. The Five Most Costly Children's Conditions, 2011: Estimates for U.S. Civilian Noninstitutionalized Children, Ages 0–17. (2014) Apr. In: Statistical Brief (Medical Expenditure Panel Survey (US)) [Internet]. Rockville (MD): Agency for Healthcare Research and Quality (US); 2001–. STATISTICAL BRIEF #434.
18. Chong Neto HJ, Rosário NA; Grupo EISL Curitiba (Estudio Internacional de Sibilancias en Lactantes). Risk factors for wheezing in the first year of life. J Pediatr. (2008) 84:495–502. doi: 10.2223/JPED.1835
19. Mallol J, Andrade R, Auger F, Rodríguez J, Alvarado R, Figueroa L. Wheezing during the first year of life in infants from low-income population: a descriptive study. Allergol Immunopathol. (2005) 33:257–63. doi: 10.1157/13080928
20. Bisgaard H, Szefler S. Prevalence of asthma-like symptoms in young children. Pediatr Pulmonol. (2007) 42:723–8. doi: 10.1002/ppul.20644
21. Juntti H, Osterlund P, Kokkonen J, Dunder T, Renko M, Pokka T, et al. Cytokine responses in cord blood predict the severity of later respiratory syncytial virus infection. J Allergy Clin Immunol. (2009) 124:52–58.e1–2. doi: 10.1016/j.jaci.2009.04.014
22. Nuolivirta K, Helminen M. Mannose-binding lectin gene polymorphisms in infants with bronchiolitis and post-bronchiolitis wheezing. Allergol Int. (2012) 61:305–9. doi: 10.2332/allergolint.11-OA-0385
23. Holgate S, Wenzel S, Postma D, Weiss ST, Renz H, Sly PD. Asthma. Nat Rev Dis Primers. (2015) 1:15025. doi: 10.1038/nrdp.2015.25
24. Backman K, Piippo-Savolainen E, Ollikainen H, Koskela H, Korppi M. Increased asthma risk and impaired quality of life after bronchiolitis or pneumonia in infancy. Pediatr Pulmonol. (2014) 49:318–25. doi: 10.1002/ppul.22842
25. Jackson DJ, Evans MD, Gangnon RE, Tisler CJ, Pappas TE, Lee WM, et al. Evidence for a causal relationship between allergic sensitization and rhinovirus wheezing in early life. Am J Respir Crit Care Med. (2012) 185:281–5. doi: 10.1164/rccm.201104-0660OC
26. Calvo C, Pozo F, García-García ML, Sanchez M, Lopez-Valero M, Pérez-Breña P, et al. Detection of new respiratory viruses in hospitalized infants with bronchiolitis: a three-year prospective study. Acta Paediatr. (2010) 99:883–7. doi: 10.1111/j.1651-2227.2010.01714.x
27. Jackson DJ, Lemanske RF. The role of respiratory virus infections in childhood asthma inception. Immunol Allergy Clin North Am. (2010) 30:513–22. doi: 10.1016/j.iac.2010.08.004
28. Carroll KN, Gebretsadik T, Minton P, Woodward K, Liu Z, Miller EK, et al. Influence of maternal asthma on the cause and severity of infant acute respiratory tract infections. J Allergy Clin Immunol. (2012) 129:1236–42. doi: 10.1016/j.jaci.2012.01.045
29. Beigelman A, Bacharier LB. The role of early life viral bronchiolitis in the inception of asthma. Curr Opin Allergy Clin Immunol. (2013) 13:211–6. doi: 10.1097/ACI.0b013e32835eb6ef
30. Saglani S. Viral infections and the development of asthma in children. Ther Adv Infect Dis. (2013) 1:139–50. doi: 10.1177/2049936113497202
31. Bacharier LB, Cohen R, Schweiger T, Yin-Declue H, Christie C, Zheng J, et al. Determinants of asthma after severe respiratory syncytial virus bronchiolitis. J Allergy Clin Immunol. (2012) 130:91–100.e3. doi: 10.1016/j.jaci.2012.02.010
32. Calvo C, García-García ML, Blanco C, Frías ME, Casas I, Pérez-Brena~ P. Role of rhinovirus in hospitalized infants with respiratory tract infections in Spain. Pediatr Infect Dis J. (2007) 26:904–8. doi: 10.1097/INF.0b013e31812e52e6
33. O'Callaghan-Gordo C, Bassat Q, Díez-Padrisa N, Morais L, Machevo S, Nhampossa T, et al. Lower respiratory tract infections associated with rhinovirus during infancy and increased risk of wheezing during childhood. A cohort study. PLOS ONE. (2013) 8:e69370. doi: 10.1371/journal.pone.0069370
34. Kappelle L, Brand PLP. Severe episodic viral wheeze in preschool children: High risk of asthma at age 5–10 years. Eur J Pediatr. (2012) 171:947–54. doi: 10.1007/s00431-011-1663-7
35. Hasegawa K; Mansbach JM, Bochkov YU, Camargo CA Jr. Association of Rhinovirus C Bronchiolitis and Immunoglobulin E Sensitization during Infancy with Development of Recurrent Wheeze. JAMA Pediatr. (2019) 173:544–52. doi: 10.1001/jamapediatrics.2019.0384
36. Frey A, Lunding LP, Ehlers JC. More than just a barrier: the immune functions of the airway epithelium in asthma pathogenesis. Front Immunol. (2020) 11:761. doi: 10.3389/fimmu.2020.00761
37. Zissler UM, Jakwerth CA, Guerth F. Allergen-specific immunotherapy induces the suppressive secretoglobin 1A1 in cells of the lower airways. Allergy. (2021) 76:2461–74. doi: 10.1111/all.14756
38. Pierangeli A, Gentile M, Di Marco P, Pagnotti P, Scagnolari C, Trombetti, et al. Detection and typing of molecular techniques of respiratory viruses in children hospitalised for acute respiratory infection in Rome, Italy. J Med Virol. (2007) 79:463–8. doi: 10.1002/jmv.20832
39. Semple MG, Cowell A, Dove W, Greensill J, McNamara PS, Halfhide C, et al. Dual infection of infants with human metapneumovirus and human respiratory syncytial virus is s strongly associated with severe bronchiolitis. J Infect Dis. (2005) 191:382–6. doi: 10.1086/426457
40. Halfhide C, Smyth RL. Innate immune response and bronchiolitis and preschool recurrent wheeze. Paediatr Respir Rev. (2008) 9:251–62. doi: 10.1016/j.prrv.2008.05.005
41. Biagi C, Rocca A, Poletti G, Fabi M, Lanari M. Rhinovirus infection in children with acute bronchiolitis and its impact on recurrent wheezing and asthma development. Microorganisms. (2020) 8:1620. doi: 10.3390/microorganisms8101620
42. Amat F, Plantard C, Mulliez A. RSV-hRV co-infection is a risk factor for recurrent bronchial obstruction and early sensitization 3 years after bronchiolitis. Med Virol. (2018) 90:867–72. doi: 10.1002/jmv.25037
43. Taussig LM, Wright AL, Holberg CJ, Halonen M, Morgan WJ, Martinez FD. Tucson children's respiratory study: 1980 to present. J Allergy Clin Immunol. (2003) 111:661–75. doi: 10.1067/mai.2003.162
44. Castro-Rodriguez JA, Holberg CJ, Wright AL, Martinez FD. A clinical index to define risk of asthma in young children with recurrent wheezing. Am J Respir Crit Care Med. (2000) 162:1403–6. doi: 10.1164/ajrccm.162.4.9912111
45. Castro-Rodriguez JA. The asthma predictive index: a very useful tool for predicting asthma in young children. J Allergy Clin Immunol. (2010) 126:212–6. doi: 10.1016/j.jaci.2010.06.032
46. Guilbert TW, Morgan WJ, Zeiger RS, Bacharier LB, Boehmer SJ, Krawiec M, et al. Atopic characteristics of children with recurrent wheezing at high risk for the development of childhood asthma. J Allergy Clin Immunol. (2004) 114:1282–7. doi: 10.1016/j.jaci.2004.09.020
47. Castro-Rodriguez JA, Cifuentes L, Rodriguez-Martinez CE. The asthma predictive index remains a useful tool to predict asthma in young children with recurrent wheeze in clinical practice. J Allergy Clin Immunol. (2011) 127:1082–3. doi: 10.1016/j.jaci.2011.01.024
48. Pedersen SE, Hurd SS, Lemanske RF Jr, Becker A, Zar HJ, Sly PD, et al. Global initiative for asthma. Global strategy for the diagnosis and management of asthma in children 5 years and younger. Pediatr Pulmonol. (2011) 46:1–17. doi: 10.1002/ppul.21321
49. Calişkan M, Bochkov YA, Kreiner-Møller E, Bønnelykke K, Stein MM, Du G, et al. Rhinovirus wheezing illness and genetic risk of childhood-onset asthma. N Engl J Med. (2013) 368:1398–407. doi: 10.1056/NEJMoa1211592
50. Bosch AATM, Levin E, van Houten MA, Hasrat R, Kalkman G, Biesbroek G, et al. Development of upper respiratory tract microbiota in infancy is affected by mode of delivery. EBioMedicine. (2016) 9:336–45. doi: 10.1016/j.ebiom.2016.05.031
51. Chu DM, Ma J, Prince AL, Antony KM, Seferovic MD, Aagaard KM. Maturation of the infant microbiome community structure and function across multiple body sites and in relation to mode of delivery. Nat Med. (2017) 23:314–26. doi: 10.1038/nm.4272
52. Biesbroek G, Tsivtsivadze E, Sanders EAM, Montijn R, Veenhoven RH, Keijser BJF, et al. Early respiratory microbiota composition determines bacterial succession patterns and respiratory health in children. Am J Respir Crit Care Med. (2014) 190:1283–92. doi: 10.1164/rccm.201407-1240OC
53. Laufer AS, Metlay JP, Gent JF, Fennie KP, Kong Y, Pettigrew MM. Microbial communities of the upper respiratory tract and otitis media in children. mBio. (2011) 2:e00245–10. doi: 10.1128/mBio.00245-10
54. de Steenhuijsen Piters WA, Heinonen S, Hasrat R, Bunsow E, Smith B, Suarez-Arrabal MC, et al. Nasopharyngeal microbiota, host transcriptome, and disease severity in children with respiratory syncytial virus infection. Am J Respir Crit Care Med. (2016) 194:1104–15. doi: 10.1164/rccm.201602-0220OC
55. Teo SM, Mok D, Pham K, Kusel M, Serralha M, Troy N, et al. The infant nasopharyngeal microbiome impacts severity of lower respiratory infection and risk of asthma development. Cell Host Microbe. (2015) 17:704–15. doi: 10.1016/j.chom.2015.03.008
56. Bosch AT, de Steenhuijsen Piters WA, van Houten MA, Chu ML. Maturation of the infant respiratory microbiota, environmental drivers, and health consequences. A prospective cohort study. Am J Respir Crit Care Med. (2017) 196:1582–90. doi: 10.1164/rccm.201703-0554OC
57. Han YY, Badellino HA, Forno E, Celedón JC. Rural residence, farming environment, and allergic diseases in Argentinean adolescents. Pediatr Pulmonol. (2017) 52:21–8. doi: 10.1002/ppul.23511
58. von Mutius E. The “Hygiene Hypothesis” and the lessons learnt from farm studies. Front Immunol. (2021) 12:635522. doi: 10.3389/fimmu.2021.635522
59. Martinez FD, Morgan WJ, Wright AL, Holberg CJ, Taussig LM. Diminished lung function as a predisposing factor for wheezing respiratory illness in infants. N Engl J Med. (1988) 319:1112–7. doi: 10.1056/NEJM198810273191702
60. Tepper RS, Morgan WJ, Cota K, Wright A, Taussig LM. Physiologic growth and development of the lung during the first year of life. Am Rev Respir Dis. (1986) 134:513–9.
61. Owens L, Laing IA, Zhang G, Le Souëf PN. Infant lung function predicts asthma persistence and remission in young adults. Respirology. (2017) 22:289–94. doi: 10.1111/resp.12901
62. Owens L, Laing IA, Zhang G, Turner S, Le Souëf PN. Airway function in infancy is linked to airflow measurements and respiratory symptoms from childhood into adulthood. Pediatr Pulmonol. (2018) 53:1082–8. doi: 10.1002/ppul.24062
63. Tse SM, Coull BA, Sordillo JE, Datta S. Gender- and age-specific risk factors for wheeze from birth through adolescence. Pediatr Pulmonol. (2015) 50:955–62. doi: 10.1002/ppul.23113
64. Tse SM, Rifas-Shiman SL, Coull BA, Litonjua AA, Oken E, Gold DR. Sex-specific risk factors for childhood wheeze and longitudinal phenotypes of wheeze. J Allergy Clin Immunol. (2016) 138:1561–8. doi: 10.1016/j.jaci.2016.04.005
65. Huang SK, Zhang Q, Qiu Z, Chung KF. Mechanistic impact of outdoor air pollution on asthma and allergic diseases. J Thorac Dis. (2015) 7:23–33. doi: 10.3978/j.issn.2072-1439.2014.12.13
66. Guarnieri M, Balmes JR. Outdoor air pollution and asthma. Lancet. (2014) 383:1581–92. doi: 10.1016/S0140-6736(14)60617-6
67. Chan JK, Charrier JG, Kodani SD, Vogel CF, Kado SY, Anderson DS, et al. Combustion-derived flame generated ultrafine soot generates reactive oxygen species and activates Nrf2 antioxidants differently in neonatal and adult rat lungs. Part Fibre Toxicol. (2013) 10:34. doi: 10.1186/1743-8977-10-34
68. Wang P, Thevenot P, Saravia J, Ahlert T, Cormier SA. Radical-containing particles activate dendritic cells and enhance Th17 inflammation in a mouse model of asthma. Am J Respir Cell Mol Biol. (2011) 45:977–83. doi: 10.1165/rcmb.2011-0001OC
69. Nadeau K, McDonald-Hyman C, Noth EM, Pratt B, Hammond SK, Balmes J, et al. Ambient air pollution impairs regulatory T-cell function in asthma. J Allergy Clin Immunol. (2010) 126:845–852.e10. doi: 10.1016/j.jaci.2010.08.008
70. Gowers AM, Cullinan P, Ayres JG, Anderson HR, Strachan DP, Holgate ST, et al. Does outdoor air pollution induce new cases of asthma? Biological plausibility and evidence; a review. Respirology. (2012) 17:887–98. doi: 10.1111/j.1440-1843.2012.02195.x
71. Goodman JE, Chandalia JK, Thakali S, Seeley M. Meta-analysis of nitrogen dioxide exposure and airway hyper-responsiveness in asthmatics. Crit Rev Toxicol. (2009) 39:719–42. doi: 10.3109/10408440903283641
72. Gauderman WJ, Urman R, Avol E, Berhane K, McConnell R, Rappaport E, et al. Association of improved air quality with lung development in children. N Engl J Med. (2015) 372:905–13. doi: 10.1056/NEJMoa1414123
73. Yang IA, Fong KM, Zimmerman PV, Holgate ST, Holloway JW. Genetic susceptibility to the respiratory effects of air pollution. Postgrad Med J. (2009) 85:428–36. doi: 10.1136/thx.2007.079426
74. Esposito S, Tenconi R, Lelii M, Preti V, Nazzari E, Consolo S, et al. Possible molecular mechanisms linking air pollution and asthma in children. BMC Pulm Med. (2014) 14:31. doi: 10.1186/1471-2466-14-31
75. Vork KL, Broadwin RL, Blaisdell RJ. Developing asthma in childhood from exposure to secondhand tobacco smoke: insights from a meta-regression. Environ Health Perspect. (2007) 115:1394–400. doi: 10.1289/ehp.10155
76. Burke H, Leonardi-Bee J, Hashim A, Pine-Abata H, Chen Y, Cook DG, et al. Prenatal and passive smoke exposure and incidence of asthma and wheeze: systematic review and meta-analysis. Pediatrics. (2012) 129:735–44. doi: 10.1542/peds.2011-2196
77. Feleszko W, Zawadzka-Krajewska A, Matysiak K, Lewandowska D, Peradzyńska J, Dinh QT, et al. Parental tobacco smoking is associated with augmented IL-13 secretion in children with allergic asthma. J Allergy Clin Immunol. (2006) 117:97–102. doi: 10.1016/j.jaci.2005.09.008
78. Li YF, Langholz B, Salam MT, Gilliland FD. Maternal and grand maternal smoking patterns are associated with early childhood asthma. Chest. (2005) 127:1232–41. doi: 10.1016/S0012-3692(15)34472-X
79. Teijeiro A, Cuello MN, Raiden MG, Vieyra RE, Solé D, Ellwood P, et al. The relationship between second-hand smoke and wheezing in infants from Córdoba, Argentina. Allergol Immunopathol. (2020) 48:42–7. doi: 10.1016/j.aller.2019.06.010
80. Mebrahtu TF, Feltbower RG, Greenwood DC, Parslow RC. Childhood body mass index and wheezing disorders: a systematic review and metaanalysis. Pediatr Allergy Immunol. (2015) 26:62–72. doi: 10.1111/pai.12321
81. Forno E, Young OM, Kumar R, Simhan H, Celedón JC. Maternal obesity in pregnancy, gestational weight gain, and risk of childhood asthma. Pediatrics. (2014) 134:e535–46. doi: 10.1542/peds.2014-0439
82. Castro-Rodríguez JA, Holberg CJ, Morgan WJ, Wright AL, Martinez FD. Increased incidence of asthma-like symptoms in girls who become overweight or obese during the school years. Am J Respir Crit Care Med. (2001) 163:1344–9. doi: 10.1164/ajrccm.163.6.2006140
83. Granell R, Henderson AJ, Evans DA, Smith GD. Effects of BMI, fat mass, and lean mass on asthma in childhood: a mendelian randomization study. PLoS Med. (2014) 11:e1001669. doi: 10.1371/journal.pmed.1001669
84. Torday JS, Sun H, Wang L, Torres E, Sunday ME, Rubin LP. Leptin mediates the parathyroid hormone-related protein paracrine stimulation of fetal lung maturation. Am J Physiol Lung Cell Mol Physiol. (2002) 282:L405–10. doi: 10.1152/ajplung.2002.282.3.L405
85. Shore SA, Schwartzman IN, Mellema MS, Flynt L, Imrich A, Johnston RA. Effect of leptin on allergic airway responses in mice. J Allergy Clin Immunol. (2005) 115:103–9. doi: 10.1016/j.jaci.2004.10.007
86. Mc Lachlan CR, Poulton R, Car G, Cowan J, Filsell S, Greene JM, et al. Adiposity, asthma, and airway inflammation. J Allergy Clin Immunol. (2007) 119:634–9. doi: 10.1016/j.jaci.2006.10.029
87. Kattan M, Kumar R, Bloomberg GR, Mitchell HE, Calatroni A, Gergen PJ, et al. Asthma control, adiposity, and adipokines among inner-city adolescents. J Allergy Clin Immunol. (2010) 125:584–92. doi: 10.1016/j.jaci.2010.01.053
88. Alamri A. Diversity of microbial signatures in asthmatic airways. Int J Gen Med. (2021) 14:1367–78. doi: 10.2147/IJGM.S304339
89. Dodi G, Attanasi M, Di Filippo P, Di Pillo S, Chiarelli F. Virome in the lungs: the role of anelloviruses in childhood respiratory diseases. Microorganisms. (2021) 9:1357. doi: 10.3390/microorganisms9071357
90. Freer G, Maggi F, Pifferi M, Di Cicco ME, Peroni DG, Pistello M. The virome and its major component, anellovirus, a convoluted system molding human immune defenses and possibly affecting the development of asthma and respiratory diseases in childhood. Front Microbiol. (2018) 9:686. doi: 10.3389/fmicb.2018.00686
91. Tay CJX, Ta LDH, Ow Yeong YX, Yap GC, Chu JJH, Lee BW, et al. Role of upper respiratory microbiota and virome in childhood rhinitis and wheeze: collegium internationale allergologicum update 2021. Int Arch Allergy Immunol. (2021) 182:265–76. doi: 10.1159/000513325
92. Jartti T, Gern JE. Role of viral infections in the development and exacerbation of asthma in children. J Allergy Clin Immunol. (2017) 140, 895–906. doi: 10.1016/j.jaci.2017.08.003
93. Edwards MR, Walton RP, Jackson DJ, Feleszko W, Skevaki C, Jartti T. The potential of anti-infectives and immunomodulators as therapies for asthma and asthma exacerbations. Allergy. (2018) 73:50–63. doi: 10.1111/all.13257
94. Phipatanakul W. Preventing Asthma in High Risk Kids (PARK). Available online at: https://clinicaltrials.gov/ct2/show/NCT02570984 (accessed June 11, 2021)
Keywords: bronchiolitis, wheeze, asthma, childhood, recurrence, risk
Citation: Teijeiro A and Gómez RM (2021) Wheezing-Related Relevant Factors and the Role of Viral Bronchiolitis. Front. Allergy 2:726972. doi: 10.3389/falgy.2021.726972
Received: 17 June 2021; Accepted: 01 September 2021;
Published: 05 October 2021.
Edited by:
Paraskevi Xepapadaki, National and Kapodistrian University of Athens, GreeceReviewed by:
Yuzaburo Inoue, Chiba Children's Hospital, JapanUlrich Matthias Zissler, Technical University of Munich, Germany
Copyright © 2021 Teijeiro and Gómez. This is an open-access article distributed under the terms of the Creative Commons Attribution License (CC BY). The use, distribution or reproduction in other forums is permitted, provided the original author(s) and the copyright owner(s) are credited and that the original publication in this journal is cited, in accordance with accepted academic practice. No use, distribution or reproduction is permitted which does not comply with these terms.
*Correspondence: R. Maximiliano Gómez, gomezmaximiliano@hotmail.com; orcid.org/0000-0001-6898-186X
†These authors have contributed equally to this work