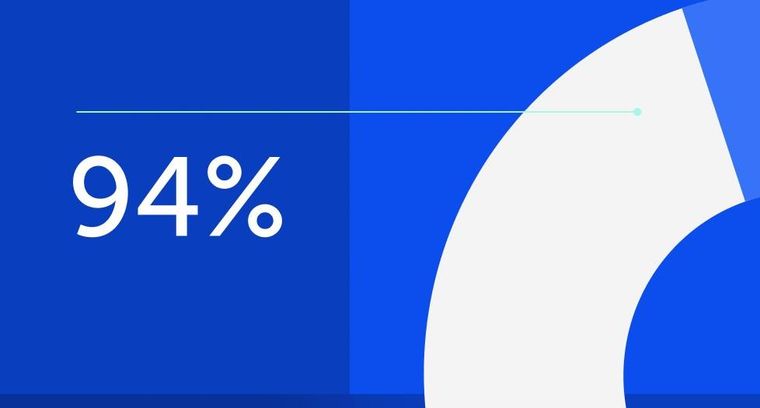
94% of researchers rate our articles as excellent or good
Learn more about the work of our research integrity team to safeguard the quality of each article we publish.
Find out more
REVIEW article
Front. Allergy, 14 June 2021
Sec. Allergens
Volume 2 - 2021 | https://doi.org/10.3389/falgy.2021.695262
This article is part of the Research TopicAllergens and allergic sensitization in Asia and the TropicsView all 10 articles
Glutathione-S transferases (GSTs) are part of a ubiquitous family of dimeric proteins that participate in detoxification reactions. It has been demonstrated that various GSTs induce allergic reactions in humans: those originating from house dust mites (HDM), cockroaches, and helminths being the best characterized. Evaluation of their allergenic activity suggests that they have a clinical impact. GST allergens belong to different classes: mu (Blo t 8, Der p 8, Der f 8, and Tyr p 8), sigma (Bla g 5 and Asc s 13), or delta (Per a 5). Also, IgE-binding molecules belonging to the pi-class have been discovered in helminths, but they are not officially recognized as allergens. In this review, we describe some aspects of the biology of GST, analyze their allergenic activity, and explore the structural aspects and clinical impact of their cross-reactivity.
Glutathione-S transferases (GSTs) are a part of a ubiquitous family of dimeric proteins that have triggered the interest of different biology-related disciplines due to their involvement in drug resistance, carcinogenesis, and allergy. Most of these isoenzymes participate in detoxification reactions, coupling reduced glutathione (GSH) to xenobiotics and/or endogenous substances, such as bilirubin and steroids. They have allergological importance for multiple reasons: several of them are epidemiologically relevant as environmental sensitizers and due to their sequence and structural conservation even among phylogenetically distant species, they may mediate cross-reactivity among invertebrate allergen sources, such as cockroach, house dust mites (HDM), and helminths. Also, probably because of their abundance and immunogenicity, some helminth GSTs have been evaluated in pre-clinical studies and clinical trials as vaccines to prevent helminthiasis (1, 2). Altogether, it means that it is necessary to determine the allergenic activity of these IgE-binding molecules and the clinical impact of their cross-reactivity, including potential allergic reactions after anti-helminth vaccination. Although it is still an unexplored field, immunomodulatory properties have been detected in certain parasite GSTs, of which some of them interfere with type 2 responses or strengthen immunosuppression (3–5). In this review, we describe some aspects of the biology of GST, analyze their allergenic activity, and explore the structural reasons and clinical impact of their cross-reactivity.
The main enzymatic reaction of GST consists of the conjugation of the thiol group of GSH to chemical electrophilic centers. The resulting conjugates are less reactive or more water-soluble, facilitating its excretion (6–8). Other less common functions of GST have been described in invertebrates. Meyer et al. purified a sigma-GST from the helminth Ascaridia galli with homology to a GSH-dependent prostaglandin-H D-isomerase and high activity and specificity in the GSH-dependent isomerization of prostaglandin H to prostaglandin E, a lipid mediator associated with suppression of host immunity (9). Two kappa-GSTs from Caenorhabditis elegans, were located in peroxisomes and mitochondria, and, by RNA interference experiments, were found to be involved in oxygen consumption and lipid metabolism (10). Some GSTs also catalyze the selenium-independent reduction of peroxide-containing compounds (11). Arthropod GSTs may confer resistance via direct metabolism, sequestration of chemicals, or metabolizing secondary products. To date, GST activity has been associated with resistance to all main classes of insecticides (12). In helminths, the main detoxifying system is mediated by GSTs; CYP450, another important system found in animals, is not present in most parasites (13). GST content and activity are usually investigated through affinity chromatography and using 1-chloro-2,4-dinitrobenzene (CDNB) substrate, since most of these enzymes may catalyze reactions. However, due to their importance in insecticide and worm chemotherapy resistance, it is common to use high-throughput methods, such as quantitative transcriptomics, functional genomics, and structural studies, for their characterization (12).
Members of this family are classified according to their cellular localizations into three major families, i.e., cytosolic, mitochondrial/peroxisomal, and microsomal GSTs (14). Cytosolic GSTs are the most abundant and best characterized. In general, they are 23–28 kDa molecules that are functionally active as dimers. They contain the fundamental signature of an N-terminal domain that binds GSH and an alpha-helix conformation in the C-terminal domain, also known as the substrate-binding site. Among cytosolic GSTs, several classes (mu, alpha, pi, theta, sigma, zeta, and omega) are widely distributed in nature. Others are more specific to certain kingdoms or phyla. Epsilon GSTs, for example, have been identified only in insects and delta GSTs in certain arthropod classes (15). All the known allergens belong to mu, sigma, or delta classes. Also, IgE-binding molecules belonging to the pi-class have been discovered in helminths (16), but have not been officially recognized as allergens by the IUIS. Although most GSTs conserved a general structural pattern, isoforms from different classes present low sequence similarity (14, 17). It is expected that GSTs from different classes are not cross-reactive (18), but this is not a rule; for example, cross-reactivity between the sigma Bla g 5 and a pi-GST from the nematode Wuchereria bancrofti was demonstrated (16). Knowledge about the class properties of GSTs may guide the research on its allergenic aspects: (a) confirmation of enzymatic activity of a recombinant GST supports a well-folded product, similar to the natural molecule; (b) it must be considered in the evaluation of intrinsic features of allergens that may promote Th2 responses far more than its IgE-binding properties; (c) the natural abundance of GST isoenzymes according to the species is helpful to evaluate the potential cross-reactivity with other sources and understand its clinical impact. For example, a delta-GST from a cockroach may cross-react with an ortholog in HDM (19), but in this last organism, the isoform may not be abundant (20).
Members of this class have been described in mammalians, chickens, insects, helminths, and mollusks (21). The cockroach allergen Bla g 5 (22) and the nematode GST Asc l 3 (23) belong to this class. Their X-ray crystal structures confirm their similarity with other sigma GSTs (24). The first mammalian sigma-class determined structure was from the rat hematopoietic prostaglandin D synthase with GSH bound (25). Human sigma GST is involved in the biosynthesis of prostaglandin D2 (PGD2) from prostaglandin H2 (PGH2) (21). Also, the Schistosoma hematobium antigen has been found to retain this enzymatic activity, and murine models have supported the concept that PGD2 production inhibits host immune response and favors parasite survival (26).
Its members have a particular loop between the β2 sheet and the α2 helix, and they typically attach GSH to highly electrophilic compounds (27). All described mite GSTs (Der p 8, Der f 8, Blo t 8, and Tyr p 8) correspond to the mu-class (24). Even though GSTs from this class have been reported in different helminth species, there is no description of a mu-GST allergen in helminths (28, 29). In Fasciola hepatica, the common liver fluke, mu-GST expression is highly reactive to chemotherapy, and its activity is involved in protection against xenobiotics, even in a more important way than its sigma member (29). Vaccination with a DNA construct coding for this GST induced humoral response of different isotypes, including IgE but was dominated by the IgG1 isotype (30).
Together with epsilon, these GST classes are monophilic for arthropods. Delta is the major cytosolic GST class in insects and their genes are organized in large clusters (31). Allergenic delta-GSTs have been identified in cockroaches (32, 33). Their presence in other arthropods different from insects has not been fully investigated, although there are reports of delta-GSTs in Arachnida, including the Acari subclass (34, 35). Dougall et al. identified a delta-like GST in Dermatophagoides pteronyssinus, but this sequence also showed similarity with epsilon-GSTs (20). Classification of GST may be imprecise since certain proteins may have homology to more than one class of GST. In this case, studies of the gene locus, substrate-specificity experiments, and evidence of cross-reactivity with a specific class may help to better define its classification. Insects tend to have numerous delta-GST paralogous genes (34). For example, delta class represents 39% of all GST genes in A. gambiae (31). On the contrary, in Acari, mu-GSTs tend to be relatively more abundant (35). Delta-GST activity has been linked to resistance to insecticides and acaricides. Their expression may also be raised by exposure to toxic compounds (36). This has also raised the concern of higher allergenic activity (37) of HDM in cities with poor air quality associated with diesel and other environmental contaminants, as described for Der p 8 (38).
It has been demonstrated that several GSTs induce allergic reactions in humans, those originating from HDM, cockroaches, and helminths being the best characterized (Figure 1). After a systematic search in MEDLINE using the words “glutathione transferase,” “allergen,” and “IgE,” we identified 24 out of 229 references reporting information about IgE-binding properties in 12 GSTs. When searching in Allergome.org, we identified 30 entries with this biochemical function belonging to different sources: cockroaches (32, 33), HDM (37, 42, 43), scabies (20), fungi (44), plants (45, 46), and helminths (23). Only 10 of them are officially recognized as allergens by the WHO/IUIS Allergen Nomenclature Committee and eight are derived from invertebrates. Two GST from helminths (Asc s 13 and Asc l 13) are officially recognized allergens, but in total, eight molecules are listed, of which three are predicted in silico as allergenic without any experimental evidence of at least IgE binding. Table 1 shows the most important features of the eight invertebrate GSTs officially reported as allergens, showing that most of them have positive provocation assays, confirming their allergenic activity. Mueller et al. solved the 3D structure of four of these allergenic GSTs and confirmed the global structural similarity among them; however, a relatively low level of conservation of surface-exposed residues was observed (24). Except for Der p 8 and Der f 8, the allergenic activity (47) of most of them have been evaluated by in vivo or in vitro provocation tests (Table 1), suggesting that they may have a clinical impact. However, their involvement in disease presentation has not been assessed (i.e., case-control studies or avoidance studies). It would be interesting to explore other non–IgE-mediated effects of GST that may predispose to allergic inflammation. Evidence of this was obtained for Der f 8 as explained below (48). Also, immunomodulatory properties influencing type 2 responses have been described for a helminth GST from Schistosoma that reduces intestinal inflammation through eosinophil-dependent modulation of pathological Th1 responses (49).
Figure 1. (A) Multiple sequence alignments of reported GST Allergens. The most conserved columns have the most intense color, and the least conserved are the palest. A threshold score of 6 was used (conserved amino acids are those with six or more shared properties out of 10) (39). Columns with the same amino acids are marked with “*”, and columns with mutations where all properties are conserved are marked with “+”. AMAS method of multiple sequence alignment analysis was used to calculate the physicochemical properties conserved for each column (B) Ribbon diagrams of the structures of allergenic GSTs were generated in UCSF Chimera (40) using data from the PDB database (41), Der p 8 (PDB: 4Q5Q), Blo t 8 (PDB: 4Q5N), Bla g 5 (PDB: 4Q5R) and Asc l 13 (PDB: 4Q5F).
Table 1. The allergenic activity of glutathione-S transferase (GST) from invertebrates officially named as allergens.
Described by O'Neill et al. (50), Der p 8 was the first reported GST allergen (Der p 8.0101). In this review, an IgE-binding frequency of 40% in 193 D. pteronyssinus allergic patients was observed (43). Another isoform was isolated and produced by research group of Dr. YK Chua; interestingly, due to only six amino acid replacements, this isoform had an isoelectric point (pI) of 8.5, which is significantly different from the 6.1 predicted pI of Der p 8.0101. Characterization of the native GST fraction by 2D-gel electrophoresis and western blot analysis (with anti-Der p 8 polyclonal antibodies) indicated that there were at least eight GST isoforms and confirmed the existence of the cloned allergen. Taiwanese patients showed 96 and 84% of IgE reactivity with native and recombinant Der p 8, respectively. Results from Malaysia and Singapore showed lower sensitization rates: 65 and 75%, respectively. Huang et al. also reported moderate cross-reactivity between Der p 8 and the American cockroach native GST isolated by affinity chromatography (42). We also performed data mining of the D. pteronyssinus published genome, finding eight entries sharing different degrees of sequence identity with Der p 8.0101 (41–99%) and were annotated as mu-like GSTs. Characterization of their biochemical features by ProtParam indicated that their pIs also ranged from five to nine as the results previously observed at the proteomic level (42). It is expected that, as in most arthropods, HDM harbor genes coding for other GST classes. Dougall et al. (20) by a random screening of clones from a cDNA library, found a delta-like GST sequence (Dp7018E11) with only 26% identity to Der p 8.0101. Furthermore, Liu et al. identified an IgE-binding spot compatible with this sequence after 2D separation of the whole D. pteronyssinus extract that was also identified in the genome sequence of this mite (51). There are no reports about the evaluation of its allergenic activity, in neither in vivo nor in vitro tests.
Der f 8 was reported by An et al. (52). Serology studies indicate IgE-binding frequencies to Der f 8 in the range of 6–41% (53, 54), but there is no information about its allergenic activity, neither in vivo nor in vitro tests. An interesting data about Der f 8 is its capacity to induce T cell immunoglobulin mucin domain 4 (TIM4) RNA expression in bone marrow-derived dendritic cells at even higher levels than Der f 1 or Der f 2 (48). The interaction of TIM4 with its cognate ligands, TIM1, can promote Th2-cell proliferation, and the blockade of these molecules remarkably dampened Th2 differentiation and allergic reactions (55, 56). Mice sensitized and challenged with Der f 8 developed a strong pulmonary inflammation and increased local and systemic production of Th2 cytokines. Depletion of GST from HDM extracts abrogated the inflammatory potential normally observed with the complete extract and induced regulatory T cells (48).
Blo t 8 is a 23 kDa allergen with two different sequence entries (sharing 99% identity) in UniProt, and two reported IgE-binding frequencies of 10 and 80% in Singapore and Colombia, respectively (57, 58). The tertiary structure of Blo t 8.0101 was experimentally defined by Mueller et al. and matches with a typically mu-GST (24). Blo t 8 can induce positive skin test reactions (23) and also induce positive passive cutaneous anaphylaxis tests (59). Acevedo et al. described in the natural extract of Ascaris suum, a 23 kDa band inhibited by the mite extract. Further MALDI-TOF experiments identified this band as a GST. Blo t 8 and Der p 8.0101 have 35.5% identity. The cross-reactivity between Blo t 8, Der p 8, and Asc l 13 has not been experimentally demonstrated. In silico analysis of the 3D structures of these molecules suggest that cross-reactivity between them is low (24). In summary, the allergenic activity of Blo t 8 is confirmed, but more information about its importance on allergic sensitization in tropical populations is needed due to the high variations in seroprevalence between Asia and Latin America. There is evidence that helminth infections boost IgE response to cross-reactive environmental allergens, such as HDM and cockroaches (16, 60) and, possibly, the national prevalence of helminthiasis plays a role in this difference on Blo t 8 sensitization rates. In Singapore, a high-income country, ascariasis is infrequent, in contrast to Colombia where this infection occurs in >20% of the population (61, 62).
Tyr p 8 was isolated from Tyrophagus putrescentiae by affinity chromatography. It is a 26 kDa protein sharing 83% of amino acid sequence identity with Der p 8. IgE seroprevalence against Tyr p 8 was 45.3% (48/106) among Taiwanese allergic patients who were sensitized to the mite extract. Pre-adsorption of all sera with D. pteronyssinus extract reduced the rate of IgE recognition to 18%, which suggests cross-reactivity among GSTs from these sources. There is evidence of basophil histamine release sensitized with sera from Tyr p 8-allergic patients, confirming its allergenic activity (63).
Bla g 5, the GST from B. germanica, is one of the most common causes of sensitization among cockroach-allergic patients. Arruda et al. first isolated Bla g 5 by affinity chromatography from the natural extract. Serology analysis revealed 67.5% of sensitization among 40 asthmatic patients reactive to this cockroach (22). This group also cloned the sigma GST (Bla g 5.0101), which is experimentally confirmed as a GST and is officially recognized as an allergen. Interestingly, Bla g 5 is the only allergen with a high correlation between specific IgE and wheal size in the skin test (64). Satinover et al. measured using the CAP assay IgE reactivity to different cockroach components, demonstrating in 118 patients that, together with Bla g 2, Bla g 5 dominates IgE responses (65). In summary, this allergen is an epidemiologically relevant component, whose allergenic activity has been confirmed in vivo by skin tests.
Jeong et al. isolated a delta-Bla g 5 isoform with just 15% identity with the sigma class and limited cross-reactivity. This isoform showed higher enzymatic activity when CDNB and 1,1,1-trichloro-2,2-bis(p-chlorophenyl) ethane and 4-hydroxynonenal were used as substrates, but its IgE-binding frequency was lower (17.9%) compared to the sigma class (20.5%) in cockroach-sensitized patients from Seoul, Korea. There is no evidence of its allergenic activity (33).
It would be interesting to explore whether there is a relationship between low socioeconomic background and higher sensitization to Bla g 5, as it has been observed for the complete German cockroach extract (66). Possibly, due to lower hygienic conditions, higher environmental levels of B. germanica allergens are found in low-income dwellings, as detected with Bla g 2 in the United States (67). Since helminth infections are linked to poverty, it may be hypothesized that underdeveloped countries may have higher sensitization to allergens with cross-reactive homologs present in helminths. Santiago et al. demonstrated that filarial-infected patients had higher IgE levels to Bla g 5 than non-infected donors living in the same endemic regions (16); however, this is not easy to explore because of variations in its geographical distribution. B. germanica is one of the most common cockroach species found in Canada, Europe, and the United States (68). Despite being a cosmopolitan species and its origin being tropical Asia (69), it is present but not abundant in the tropics. Meanwhile, in tropical and sub-tropical regions, Periplaneta americana is the most prevalent indoor species (70). Barbosa et al. found only 7% of sensitization to sigma Bla g 5.0101 in 53 subjects in Brazil (71).
A GST-like sequence from this cockroach was reported in 2006 in GenBank by Chew et al., but without data about its allergenicity. PCR primers based on this sequence led to the identification of the two isoforms with 99% homology by different groups, officially named as Per a 5.0101 and Per a 5.0102. Wei et al. produced the 0.0102 isoform as a recombinant protein in Baculovirus and E. coli systems. Chinese P. americana-allergic patients showed 25% (4/16) of IgE reactivity to this isoform. Both recombinant versions induced basophil activation as measured by the CD63-based flow cytometry assay (19). Per a 5 was described as a delta-GST with 81% homology to BGGSTD1 and low similarity to sigma GST allergens (15 and 13% to Bla g 5 and Der p 8, respectively). Sookrung et al. isolated Per a 5.0101 from P. americana collected in a dwelling in Thailand and characterized its whole repertoire of GST isoenzymes. They found that native and recombinant P. americana-GSTs were enzymatically active and 100% of IgE reactivity in sera of all P. americana-allergic patients living in Thailand (32). Proteomic analysis indicated that the native GST comprises three isoforms of delta and sigma classes. Interestingly, all isoforms interacted with serum IgE of the cockroach-allergic subjects. IgE reactivity to the original sequence published by Chew et al., (98–99% similar to the other two official isoforms) was 47% in Indian allergic patients. In this same review, it is reported that Per a 5 inhibited IgE binding to cockroach and HDM extracts (72). Per a 5 was also tested in Taiwan with no differences in IgE reactivity between allergic rhinitis (66%) and asthmatic (55%) patients (73). In summary, P. americana GST is an allergen with two isoforms with confirmed allergenic activity and epidemiological importance in tropical Asia. Data from Latin America is missing.
Different helminth GSTs (S. haematobium, S. mansoni, and W. bancrofti) can induce IgE antibodies in infected individuals (16, 74, 75), but only A. lumbricoides and A. suum GSTs have been reported as allergens. In the tropics, A. lumbricoides is of great relevance; first, it causes the most common soil-transmitted helminthiasis (61); and second, several epidemiological surveys have found that ascariasis is a risk factor for asthma and atopy (76). We cannot rule out the importance of other helminths GSTs as allergens since this has not been systematically explored.
The native Ascaris lumbricoides GSTs (nGSTA) purified by Acevedo et al. contained six isoforms that bind IgE (23). To date, the best-studied of these isoforms is the sigma class GST, currently reported as the Asc l 13 allergen by the WHO/IUIS Allergen Nomenclature Sub-Committee. Its sequence is completely identical to Asc s 13. The allergenic activity of the recombinant isoform (rAsc s 13) and nGSTA have been evaluated in asthmatic patients exposed to A. lumbricoides. In this population, there was a sensitization frequency of 19.5% in asthmatic patients and 13.2% in controls to rAsc l 13; and the strength of IgE levels to rAsc l 13 among asthmatic patients was significantly higher compared to mite and cockroach GSTs. Additionally, four out of 10 asthmatics had a positive skin test to nGSTA, proving the allergenic activity of this molecule and its possible clinical relevance for some patients (23).
Results from the birth cohort study FRAAT (Risk Factors for Asthma and Atopy in the Tropics) conducted in a deprived community living in Cartagena, Colombia, indicated that ~20% of children were sensitized to rAsc l 13 at a young age (6 months old), increasing to 45% at 3 years of age. A positive IgE response to Ascaris GST was associated with housing features related to poor hygienic conditions and Ascaris infection (77). Also, a de-sensitization pattern to rAsc l 13 was evident with a 25% lower rate at 6 years of age. This process of desensitization may continue to older age, according to the results of Acevedo et al. in asthmatic patients living in the same city. We found that the frequency of IgE response to rAsc l 13 was significantly greater in children (7–13 years) compared to adolescents and adults (14 years and older) (23). In that sense, IgE sensitization to rAsc l 13 may be associated with exposure to Ascaris, which tends to be more frequent during infancy (77). It is still necessary to further characterize the allergenic activity of Ascaris GSTs and their clinical relevance.
To characterize other allergenic isoforms of Ascaris ssp. in our laboratory, we identified the GST genes predicted by the Davis laboratory at the University of Colorado and expressed them as recombinant proteins. We have analyzed the enzymatic activity and humoral response of an omega-class isoform. The recombinant GST omega-class (rGSTO) has dehydroascorbate reductase and thiol transferase activity reported as well for other omega-class GSTs (51). More than 30% of people from a rural area and endemic for A. lumbricoides had IgE against GSTO with a similar frequency to rAsc l 13 (78). Despite corroborating that this sequence codes for a biologically active product, in the correction of the A. suum genome assembly submitted by the authors in 2018, the current sequence coding for one omega-GST (F1LF49) has only 90.3% of identity to rGSTO.
In 2018, the Parasite Genomic group at the Wellcome Trust Sanger Institute made the draft genome assembly and gene predictions of A. lumbricoides using a strain from the Republic of Ecuador. Based on this work, we identified 15 GST sequences of A. lumbricoides reported in the UniProt database. The sequence and tertiary structure were verified to confirm that these proteins belonged to this protein superfamily. Most predicted products from these sequences had features of the cytosolic subfamily, as the thioredoxin-like fold domain and the GST N-terminal and C-terminal domains. There was only one sequence identified as kappa subfamily GSTs, due to the presence of the DSBA-like thioredoxin domain, and one as microsomal subfamily GST (79). In A. suum, according to the genome published by the Davis Laboratory at the University of Colorado in 2018 (80), we identified 14 GSTs in the UniProt database. Most of the proteins have features related to the cytosolic subfamily, two have features of the kappa subfamily and one has features of the microsomal subfamily. Cytosolic GSTs from A. lumbricoides and A. suum were classified by their homology with different GST classes (Figure 2). At the transcriptional level, we found expression data for 10 out of the 11 cytosolic GSTs (Figure 3). It is generally observed that one pi- and one sigma-isoform (which we also confirmed in the cDNA library) are the most abundant (FILV84 and P46436, respectively). The transcriptional expression also differed between tissues and developmental stages of the parasite. A. suum and A. lumbricoides are identical at the morphological level, but not in their genomes. DNA sequences coding for GST isoforms in A. lumbricoides were in the range of 77.5 and 99.5% identity with another GST from A. suum. Only one GST (A0A0M3I3X4) of A. lumbricoides did not have a similar sequence in A. suum and two sequences in A. suum (F1LBW9 and F1LCY4) were not identified in A. lumbricoides. The A. suum kappa-GST (F1LDD6) had an identity of 68.3% with an A. lumbricoides DSBA domain-containing protein with a match length of 182 of 254 amino acids.
Figure 2. Phylogenetic tree views of cytosolic helminth GSTs. The workflow was created in Phylogeny.fr (81). The amino acid sequences of the identified molecules were aligned using MUSCLE. The phylogenetic tree was constructed using PhyML. The editing was performed in the iTol platform (82).
Figure 3. Gene expression profile of GSTs from Ascaris suum based on public RNAseq data from experiments SRP013609 (left), SRP005511 (middle), and SRP010159 (left). RNA expression levels in transcripts per million (TPM) in different tissues and lifecycle stages are showed (data from public RNA sequencing studies downloaded from parasite.wormbase.org).
Several investigations have pointed out different parasite GSTs as immunomodulatory proteins, including molecules derived from helminths and protists (83). The participation of GST on immunoregulation in mammalian species has also been documented (21). As the focus of this review is invertebrate GSTs, we describe only the evidence on helminth GSTs showing any effect on the immune responses of exposed hosts.
Immunomodulatory properties of sigma-GST (fhGST-si) from F. hepatica have been found over mouse BMDCs, reducing their inflammatory response to LPS and inhibiting its capacity to induce Th17 polarization (3). Stimulation of BMDCs with the fhGST-si induced secretion of pro-inflammatory cytokines, such as IL-6, IL-12p40, and MIP2 e IL-10, but caused a partial cellular activation: CD40 upregulation in the absence of CD80, CD86, and MHC-II overexpression. Priming dendritic cells with fhGST-si before the addition of OVA reduced Th17 responses in DO11.10 mice, a transgene strain reactive to an OVA peptide antigen. This same group also tested the recombinant mu-GST from F. hepatica, with no observable immunomodulatory effect of this isoform. Aguayo et al. tested the native F. hepatica GST (nFhGST), which is majorly comprised of mu-class GST isoforms (96%), in a septic shock mouse model. It was observed that nFhGST significantly suppressed the LPS-induced TNFα and IL1β in vitro production by macrophages and the pro-inflammatory cytokine/chemokine storm within C57BL/6 mice elicited by lethal doses of LPS (84).
P28GST, a GST derived from Schistosoma haematobium has shown to induce a strong mucosal immune response, associated with IL-10 and Th2 cytokine production in animal models and humans (5, 49). Due to this capacity, it has been tested as a vaccine against schistosomiasis and Crohn's disease. From animal models, it has been observed that administration of Sh28GST is as effective as S. cercariae in preventing tissue damage and inflammation (TNF, IL-1β, and IL-6 production) in experimental colitis. It was also found to induce Th2 polarization, characterized by local and systemic production of IL-13 and IL-5. An eosinophilic infiltration in colonic tissue was evidenced in GST-immunized mice, and eosinophil depletion was associated with a loss of the ameliorating effects observed for Sh28GST (49). This molecule was also tested in a curative model, observing therapeutic effects. Administration of P28GST after TNBS-colitis induction decreased gut inflammation, associated with Th1/Th17 response downregulation and the development of alternatively activated macrophages. In this review, the authors also demonstrated that only intact schistosome-derived P28GST was able to induce a significant reduction of the clinical score, in contrast to heat-inactivated P28GST that loses its enzymatic activity (85). Results from a multicenter, open-label, pilot Phase 2a study that evaluated the safety of P28GST administered to patients with mild Crohn's disease (CD) also indicated that this is a safe product and showed a promising effect on reducing disease severity (86).
Data from immunomodulation in humans can be extracted from clinical trials that tested this GST as a vaccine to prevent urinary schistosomiasis. Results from a Phase I study in 24 healthy volunteers who received Sh28GST with alum indicated that immunization with this antigen is mostly safe, with few cases of mild site-infection reactions. In a subset of patients (n = 8), whose humoral and cytokine response to Sh28GST-Alum was investigated, it was observed that immunization induced IgG1, IgG2, IgG3, and IgG4 antibodies, in contrast to IgE whose titers did not significantly raise in comparison with baseline. Production of IL-5 and IL-13 was significantly induced after two administrations of rSh28GST in six out of eight vaccinated adults (5). In the Phase III study of this vaccine, 250 children, living in a hyperendemic area of Senegal, were randomized to receive rSh28GST or placebo. The vaccine did not show efficacy to prevent cases of urinary schistosomiasis. The anti-Sh28GST response was characterized by elevated levels of specific IgG1, IgG2, and IgG4 antibodies. In this population, vaccination did increase the specific IgE antibody response with 36.8% positive children in the active group. No cases of anaphylaxis or another systemic reaction after vaccination were reported; however, it is important to highlight that in contexts of natural exposure to helminths, helminths vaccines inducing IgE may induce anaphylaxis, as it was reported for a hookworm vaccine (87).
Due to their potential clinical impact, GSTs from environmental sources, such as cockroaches and HDM, are allergens that could be included in component-resolved diagnosis platforms; it will then be necessary to define the clinical impact of cross-reactivity among them. However, there are few studies regarding this topic, probably because the complete repertoire of isoenzymes has not been elucidated in the species of interest. Mueller et al. analyzed in silico the potential of IgE cross-reactivity among some of the most studied allergenic GSTs and concluded that the possibility of this phenomenon is low (24). Although Bla g 5 and Asc l 13 are sigma-GSTs, they share scarce surface conservation, not enough to have predicted common epitopes. As expected, less similarity is found among mu- and sigma-GSTs (24). However, results from Santiago et al. suggest that cross-reactivity must be extensively evaluated even when it is not expected. For example, the piGST from W. bancrofti is cross-reactive with Bla g 5 despite only sharing 27% of sequence similarity. IgE, IgG, and IgG4 binding to Bla g 5 was inhibited to a significant extent (50–70%) in individual sera collected from cockroach-allergic patients. Modeling and sequence analysis indicated that both molecules may share a lineal epitope in their N-terminal domain (16).
Cockroach allergy has important clinical implications due to its association with emergency room admissions (35, 36). Besides HDM, sensitization to cockroach allergens is the next common risk factor associated with asthma in the tropics (37). In different tropical countries, cockroach sensitization is high, but this is not in agreement with the surprisingly low allergen levels found in house dust (38, 42), and it has been argued that this is because of cross-reactivity. Tropomyosins and GST families are candidates to mediate important clinical cross-reactivity. In a co-exposed population, we have observed a high correlation in IgE levels against helminth, cockroach, and HDM GSTs (23). However, in sera obtained from Bla g 5-positive patients living in the United States, and who are not expected to be exposed to tropical allergenic sources, such as Ascaris and B. tropicalis, IgE recognition of Asc l 13 or Blo t 8 was not observed. Moreover, IgE binding to Der p 8 was inhibited only by Der p 8, but not by Bla g 5, Blo t 8, or Asc s 13. These results support the idea that, at least in North America, IgE response to Bla g 5.0101 may reflect genuine sensitization to cockroaches and that cross-reactivity is not relevant. However, in the tropics, a deeper evaluation of cross-reactivity among GSTs might be needed. IgE cross-reactivity is present between Der p 8 and native P. americana GST (42) as well as between B. tropicalis and A. suum native GST (88). Identification of the correct isoforms mediating cross-reactivity is a further step to determine the clinical significance of this phenomenon.
Different GSTs from invertebrates have been identified as molecules with allergenic activity and potential clinical relevance. In addition, some of them are also frequent sensitizers in exposed populations. The identified allergens belong to different GST classes (sigma, mu, and delta); although with a similar global structure, determined by key conserved amino acids and positions, there is low to moderate sequence conservation among them (Figure 1).
Several IgE-binding GSTs have been evaluated for their allergenic activity, being Bla g 5 the best characterized at the recombinant level, but more information on the complete repertoire of GSTs from B. germanica, observed in nature, is needed. Defining the allergenic activity will provide better information about the clinical impact of these IgE-binding molecules. In addition, extending the studies about their mechanisms of action for inducing allergic inflammation is necessary to improve our knowledge of allergy pathophysiology and to discover other options of allergy treatment. Their biological activity may be involved in Th2 polarization, which makes it interesting to explore other non-IgE mediated mechanisms that promote allergic responses (48).
Helminth-GSTs can induce different immune responses in the host; while Schistosoma-GSTs seem to induce a protective IgE response, the Ascaris-GSTs may induce allergic reactions (23). A possible explanation for this difference may be the whole context set up by the infection itself: routes of antigen entry, type 2 adjuvants, and immunosuppressive molecular content (89). There is evidence from animal models that ascariasis could increase the IgE/Th2 responses to bystander antigens (90, 91). In humans, different studies have observed that Th2/IgE hyper-responsiveness induced by Ascaris infection can also boost the IgE responses to HDM allergens, besides its antigens (76, 92). In that sense, there is a possibility that the allergenic response against Ascaris-GST is promoted by other components of Ascaris. On the contrary, it is also possible that due to the nature of the infection, Schistosoma spp. has a more immunosuppressive net effect that reduces the chance of potential allergenic molecules, such as GSTs, to elicit IgE-mediated reactions leading to a topic manifestations. Thus far, most of the studies tend to support that schistosome infection reduces skin test responses and allergic responses (93, 94).
The current data suggest that cross-reactivity is not frequent among GST allergens and those detected seem to be non-clinically relevant; however, more research is needed to understand how molecules with a low degree of sequence homology exhibit cross-reactivity. It is expected that GSTs from different classes are not cross-reactive, but an important exception has been described (16). Since living species usually contain genes coding for more than one GST class, it is necessary to evaluate the complete repertoire of GST isoenzymes if cross-reactivity aims to be assessed. It is also important to elucidate the clinical significance of IgE-binding GSTs from helminths due to the risk of allergic reactions to vaccines or immunotherapy strategies that use GST as antigens.
JZ and LC: conceived this manuscript. AL and JZ: performed bioinformatic analysis and developed figures. All authors contributed to manuscript writing, reviewing, and editing.
This work was funded by MinCiencias and the University of Cartagena (Grant Nos. 699-2017 and 803-2018).
The authors declare that the research was conducted in the absence of any commercial or financial relationships that could be construed as a potential conflict of interest.
1. Adegnika AA, de Vries SG, Zinsou FJ, Honkepehedji YJ, Dejon Agobé JC, Vodonou KG, et al. Safety and immunogenicity of co-administered hookworm vaccine candidates Na-GST-1 and Na-APR-1 in gabonese adults: a randomised, controlled, double-blind, phase 1 dose-escalation trial. Lancet Infect Dis. (2021) 21:275–85. doi: 10.1016/S1473-3099(20)30288-7
2. Capron A, Capron M, Dombrowicz D, and Riveau G. Vaccine strategies against schistosomiasis: from concepts to clinical trials. Int Arch Allergy Immunol. (2001) 124:9–15. doi: 10.1159/000053656
3. Dowling DJ, Hamilton CM, Donnelly S, La Course J, Brophy PM, Dalton J, et al. Major secretory antigens of the helminth Fasciola hepatica activate a suppressive dendritic cell phenotype that attenuates Th17 cells but fails to activate Th2 immune responses. Infect Immun. (2010) 78:793–801. doi: 10.1128/IAI.00573-09
4. Boulanger D, Reid GD, Sturrock RF, Wolowczuk I, Balloul JM, Grezel D, et al. Immunization of mice and baboons with the recombinant Sm28GST affects both worm viability and fecundity after experimental infection with Schistosoma mansoni. Parasite Immunol. (1991) 13:473–90. doi: 10.1111/j.1365-3024.1991.tb00545.x
5. Riveau G, Deplanque D, Remoué F, Schacht AM, Vodougnon H, Capron M, et al. Safety and immunogenicity of rSh28GST antigen in humans: phase 1 randomized clinical study of a vaccine candidate against urinary schistosomiasis. PLoS Negl Trop Dis. (2012) 6:e1704. doi: 10.1371/journal.pntd.0001704
6. Armstrong RN. Structure, catalytic mechanism, and evolution of the glutathione transferases. Chem Res Toxicol. (1997) 10:2–18. doi: 10.1021/tx960072x
7. van Bladeren PJ. Glutathione conjugation as a bioactivation reaction. Chem Biol Interact. (2000) 129:61–76. doi: 10.1016/S0009-2797(00)00214-3
8. Wilce MC, and Parker MW. Structure and function of glutathione S-transferases. Biochim Biophys Acta. (1994) 1205:1–18. doi: 10.1016/0167-4838(94)90086-8
9. Meyer DJ, Muimo R, Thomas M, Coates D, and Isaac RE. Purification and characterization of prostaglandin-H E-isomerase, a sigma-class glutathione S-transferase, from Ascaridia galli. Biochem J. (1996) 313 (Pt. 1):223–7. doi: 10.1042/bj3130223
10. Petit E, Michelet X, Rauch C, Bertrand-Michel J, Terce F, Legouis R, et al. Glutathione transferases kappa 1 and kappa 2 localize in peroxisomes and mitochondria, respectively, and are involved in lipid metabolism and respiration in Caenorhabditis elegans. FEBS J. (2009) 276:5030–40. doi: 10.1111/j.1742-4658.2009.07200.x
11. Mannervik B, Helena Danielson U, and Ketterer B. Glutathione transferases—structure and catalytic activit. Crit Rev Biochem. (1988) 23:283–337. doi: 10.3109/10409238809088226
12. Pavlidi N, Vontas J, and Van Leeuwen T. The role of glutathione S-transferases (GSTs) in insecticide resistance in crop pests and disease vectors. Curr Opin Insect Sci. (2018) 27:97–102. doi: 10.1016/j.cois.2018.04.007
13. Torres-Rivera A, and Landa A. Glutathione transferases from parasites: a biochemical view. Acta Tropica. (2008) 105:99–112. doi: 10.1016/j.actatropica.2007.08.005
14. Sheehan D, Meade G, Foley VM, and Dowd CA. Structure, function and evolution of glutathione transferases: implications for classification of non-mammalian members of an ancient enzyme superfamily. Biochem J. (2001) 360 (Pt. 1):1–16. doi: 10.1042/bj3600001
15. Ketterman AJ, Saisawang C, and Wongsantichon J. Insect glutathione transferases. Drug Metab Rev. (2011) 43:253–65. doi: 10.3109/03602532.2011.552911
16. Santiago HC, Leevan E, Bennuru S, Ribeiro-Gomes F, Mueller E, Wilson M, et al. Molecular mimicry between cockroach and helminth glutathione S-transferases promotes cross-reactivity and cross-sensitization. J Allergy Clin Immunol. (2012) 130:248–56.e9. doi: 10.1016/j.jaci.2012.02.045
17. Mannervik B, Awasthi YC, Board PG, Hayes JD, Di Ilio C, Ketterer B, et al. Nomenclature for human glutathione transferases. Biochem J. (1992) 282 (Pt. 1):305–6. doi: 10.1042/bj2820305
18. Fournier D, Bride JM, Poirie M, Berge JB, and Plapp FW Jr. Insect glutathione S-transferases. Biochemical characteristics of the major forms from houseflies susceptible and resistant to insecticides. J Biol Chem. (1992) 267:1840–5. doi: 10.1016/S0021-9258(18)46023-1
19. Wei JF, Yang H, Li D, Gao P, and He S. Preparation and identification of Per a 5 as a novel American cockroach allergen. Mediators Inflamm. (2014) 2014:591468. doi: 10.1155/2014/591468
20. Dougall A, Holt DC, Fischer K, Currie BJ, Kemp DJ, and Walton SF. Identification and characterization of Sarcoptes scabiei and Dermatophagoides pteronyssinus glutathione S-transferases: implication as a potential major allergen in crusted scabies. Am J Trop Med Hyg. (2005) 73:977–84. doi: 10.4269/ajtmh.2005.73.977
21. Flanagan JU, and Smythe ML. Sigma-class glutathione transferases. Drug Metab Rev. (2011) 43:194–214. doi: 10.3109/03602532.2011.560157
22. Arruda LK, Vailes LD, Platts-Mills TA, Hayden ML, and Chapman MD. Induction of IgE antibody responses by glutathione S-transferase from the German cockroach (Blattella germanica). J Biol Chem. (1997) 272:20907–12. doi: 10.1074/jbc.272.33.20907
23. Acevedo N, Mohr J, Zakzuk J, Samonig M, Briza P, Erler A, et al. Proteomic and immunochemical characterization of glutathione transferase as a new allergen of the nematode Ascaris lumbricoides. PLoS ONE. (2013) 8:e78353. doi: 10.1371/journal.pone.0078353
24. Mueller GA, Pedersen LC, Glesner J, Edwards LL, Zakzuk J, London RE, et al. Analysis of glutathione S-transferase allergen cross-reactivity in a North American population: relevance for molecular diagnosis. J Allergy Clin Immunol. (2015) 136:1369–77. doi: 10.1016/j.jaci.2015.03.015
25. Meyer DJ, and Thomas M. Characterization of rat spleen prostaglandin H D-isomerase as a sigma-class GSH transferase. Biochem J. (1995) 311 (Pt. 3):739–42. doi: 10.1042/bj3110739
26. Hervé M, Angeli V, Pinzar E, Wintjens R, Faveeuw C, Narumiya S, et al. Pivotal roles of the parasite PGD2 synthase and of the host D prostanoid receptor 1 in schistosome immune evasion. Eur J Immunol. (2003) 33:2764–72. doi: 10.1002/eji.200324143
27. Ji X, Zhang P, Armstrong RN, and Gilliland GL. The three-dimensional structure of a glutathione S-transferase from the mu gene class. Structural analysis of the binary complex of isoenzyme 3-3 and glutathione at 2.2-A resolution. Biochemistry. (1992) 31:10169–84. doi: 10.1021/bi00157a004
28. Lopez-Gonzalez V, La-Rocca S, Arbildi P, and Fernandez V. Characterization of catalytic and non-catalytic activities of EgGST2-3, a heterodimeric glutathione transferase from Echinococcus granulosus. Acta Trop. (2018) 180:69–75. doi: 10.1016/j.actatropica.2018.01.007
29. Stuart RB, Zwaanswijk S, MacKintosh ND, Witikornkul B, Brophy PM, and Morphew RM. The soluble glutathione transferase superfamily: role of Mu class in triclabendazole sulphoxide challenge in Fasciola hepatica. Parasitol Res. (2021) 120:979–91. doi: 10.1007/s00436-021-07055-5
30. Smooker PM, Steeper KR, Drew DR, Strugnell RA, and Spithill TW. Humoral responses in mice following vaccination with DNA encoding glutathione S-transferase of Fasciola hepatica: effects of mode of vaccination and the cellular compartment of antigen expression. Parasite Immunol. (1999) 21:357–64. doi: 10.1046/j.1365-3024.1999.00235.x
31. Ding Y, Ortelli F, Rossiter LC, Hemingway J, and Ranson H. The Anopheles gambiae glutathione transferase supergene family: annotation, phylogeny and expression profiles. BMC Genomics. (2003) 4:35. doi: 10.1186/1471-2164-4-35
32. Sookrung N, Reamtong O, Poolphol R, Indrawattana N, Seesuay W, Saelim N, et al. Glutathione S-transferase (GST) of American cockroach, periplaneta americana: classes, isoforms, and allergenicity. Sci Rep. (2018) 8:484. doi: 10.1038/s41598-017-18759-z
33. Jeong KY, Jeong KJ, Yi MH, Lee H, Hong CS, and Yong TS. Allergenicity of sigma and delta class glutathione S-transferases from the German cockroach. Int Arch Allergy Immunol. (2009) 148:59–64. doi: 10.1159/000151506
34. Liu W, Tian J, Hou N, Yu N, Zhang Y, and Liu Z. Identification, genomic organization and expression pattern of glutathione transferase in Pardosa pseudoannulata. Comp Biochem Physiol Part D Genomics Proteomics. (2019) 32:100626. doi: 10.1016/j.cbd.2019.100626
35. Bartley K, Wright HW, Bull RS, Huntley JF, and Nisbet AJ. Characterisation of Dermanyssus gallinae glutathione S-transferases and their potential as acaricide detoxification proteins. Parasit Vectors. (2015) 8:350. doi: 10.1186/s13071-015-0960-9
36. Pavlidi N, Khalighi M, Myridakis A, Dermauw W, Wybouw N, Tsakireli D, et al. A glutathione-S-transferase (TuGSTd05) associated with acaricide resistance in Tetranychus urticae directly metabolizes the complex II inhibitor cyflumetofen. Insect Biochem Mol Biol. (2017) 80:101–15. doi: 10.1016/j.ibmb.2016.12.003
37. Acevedo N, Zakzuk J, and Caraballo L. House dust mite allergy under changing environments. Allergy Asthma Immunol Res. (2019) 11:450–69. doi: 10.4168/aair.2019.11.4.450
38. Vidal-Quist JC, Ortego F, Lambrecht BN, Castanera P, and Hernandez-Crespo P. Effects of domestic chemical stressors on expression of allergen genes in the European house dust mite. Med Vet Entomol. (2017) 31:97–101. doi: 10.1111/mve.12200
39. Livingstone CD, and Barton GJ. Protein sequence alignments: a strategy for the hierarchical analysis of residue conservation. Comput Appl Biosci. (1993) 9:745–56. doi: 10.1093/bioinformatics/9.6.745
40. Pettersen EF, Goddard TD, Huang CC, Couch GS, Greenblatt DM, Meng EC, et al. UCSF chimera–a visualization system for exploratory research and analysis. J Comput Chem. (2004) 25:1605–12. doi: 10.1002/jcc.20084
41. Berman HM, Westbrook J, Feng Z, Gilliland G, Bhat TN, Weissig H, et al. The protein data bank. Nucleic Acids Research. (2000) 28:235–42. doi: 10.1093/nar/28.1.235
42. Huang CH, Liew LM, Mah KW, Kuo IC, Lee BW, and Chua KY. Characterization of glutathione S-transferase from dust mite, Der p 8 and its immunoglobulin E cross-reactivity with cockroach glutathione S-transferase. Clin Exp Allergy. (2006) 36:369–76. doi: 10.1111/j.1365-2222.2006.02447.x
43. O'Neill GM, Donovan GR, and Baldo BA. Glutathione S-transferase a major allergen of the house dust mite, Dermatophagoides pteronyssinus. Immunol Lett. (1995) 48:103–7. doi: 10.1016/0165-2478(95)02452-2
44. Shankar J, Gupta PD, Sridhara S, Singh BP, Gaur SN, and Arora N. Immunobiochemical analysis of cross-reactive glutathione-S-transferase allergen from different fungal sources. Immunol Invest. (2005) 34:37–51. doi: 10.1081/IMM-200047383
45. L'Hocine L, Pitre M, and Achouri A. Detection and identification of allergens from Canadian mustard varieties of Sinapis alba and Brassica juncea. Biomolecules. (2019) 9:489. doi: 10.3390/biom9090489
46. Shankar J, Singh BP, Gaur SN, and Arora N. Recombinant glutathione-S-transferase a major allergen from Alternaria alternata for clinical use in allergy patients. Mol Immunol. (2006) 43:1927–32. doi: 10.1016/j.molimm.2005.12.006
47. Caraballo L, Valenta R, Puerta L, Pomes A, Zakzuk J, Fernandez-Caldas E, et al. The allergenic activity and clinical impact of individual IgE-antibody binding molecules from indoor allergen sources. World Allergy Organ J. (2020) 13:100118. doi: 10.1016/j.waojou.2020.100118
48. Mo LH, Yang LT, Zeng L, Xu LZ, Zhang HP, Li LJ, et al. Dust mite allergen, glutathione S-transferase, induces T cell immunoglobulin mucin domain-4 in dendritic cells to facilitate initiation of airway allergy. Clin Exp Allergy. (2017) 47:264–70. doi: 10.1111/cea.12800
49. Driss V, El Nady M, Delbeke M, Rousseaux C, Dubuquoy C, Sarazin A, et al. The schistosome glutathione S-transferase P28GST, a unique helminth protein, prevents intestinal inflammation in experimental colitis through a Th2-type response with mucosal eosinophils. Mucosal Immunol. (2016) 9:322–35. doi: 10.1038/mi.2015.62
50. O'Neill GM, Donovan GR, and Baldo BA. Cloning and characterization of a major allergen of the house dust mite, Dermatophagoides pteronyssinus, homologous with glutathione S-transferase. Biochim Biophys Acta. (1994) 1219:521–8. doi: 10.1016/0167-4781(94)90080-9
51. Liu XY, Yang KY, Wang MQ, Kwok JS, Zeng X, Yang Z, et al. High-quality assembly of Dermatophagoides pteronyssinus genome and transcriptome reveals a wide range of novel allergens. J Allergy Clin Immunol. (2018) 141:2268–71.e8. doi: 10.1016/j.jaci.2017.11.038
52. An S, Chen L, Long C, Liu X, Xu X, Lu X, et al. Dermatophagoides farinae allergens diversity identification by proteomics. Mol Cell Proteomics. (2013) 12:1818–28. doi: 10.1074/mcp.M112.027136
53. Cui YB, Zhou Y, Wang N, Teng FX, Yu LL, Bian YH, et al. Expression, cloning, and IgE-binding of the full-length dust mite allergen Der f 8. Immunol Res. (2014) 60:60–8. doi: 10.1007/s12026-014-8553-9
54. Jeong KY, Lee JY, Son M, Yi MH, Yong TS, Shin JU, et al. Profiles of IgE sensitization to Der f 1, Der f 2, Der f 6, Der f 8, Der f 10, and Der f 20 in Korean house dust mite allergy patients. Allergy Asthma Immunol Res. (2015) 7:483–8. doi: 10.4168/aair.2015.7.5.483
55. Yang PC, Xing Z, Berin CM, Soderholm JD, Feng BS, Wu L, et al. TIM-4 expressed by mucosal dendritic cells plays a critical role in food antigen-specific Th2 differentiation and intestinal allergy. Gastroenterology. (2007) 133:1522–33. doi: 10.1053/j.gastro.2007.08.006
56. Meyers JH, Chakravarti S, Schlesinger D, Illes Z, Waldner H, Umetsu SE, et al. TIM-4 is the ligand for TIM-1, and the TIM-1-TIM-4 interaction regulates T cell proliferation. Nat Immunol. (2005) 6:455–64. doi: 10.1038/ni1185
57. Kidon MI, Chiang WC, Liew WK, Ong TC, Tiong YS, Wong KN, et al. Mite component-specific IgE repertoire and phenotypes of allergic disease in childhood: the tropical perspective. Pediatr Allergy Immunol. (2011) 22:202–10. doi: 10.1111/j.1399-3038.2010.01094.x
58. Zakzuk J, Fernández-Caldas E, and Caraballo L. Evaluation of IgE responses against Blo t 8, a glutathione S transferase (GST) from Blomia tropicalis (Bt) mite. J Allergy Clin Immunol. (2010) 125:AB6. doi: 10.1016/j.jaci.2009.12.055
59. Bustillo J, Regino R, Coronado SM, Benedetti I, Pedersen L, Mueller GA, et al. Evaluation of the allergenic activity of the glutathione transferase from blomia tropicalis (Blo t 8) in a mouse model of airway inflammation. J Allergy Clin Immunol. (2019) 143:AB187. doi: 10.1016/j.jaci.2018.12.574
60. Suzuki M, Hara M, Ichikawa S, Kamijo S, Nakazawa T, Hatanaka H, et al. Presensitization to Ascaris antigens promotes induction of mite-specific IgE upon mite antigen inhalation in mice. Allergol Int. (2016) 65:44–51. doi: 10.1016/j.alit.2015.07.003
61. Pullan RL, Smith JL, Jasrasaria R, and Brooker SJ. Global numbers of infection and disease burden of soil transmitted helminth infections in 2010. Parasit Vectors. (2014) 7:37. doi: 10.1186/1756-3305-7-37
62. Zakzuk J, Casadiego S, Mercado A, Alvis-Guzman N, and Caraballo L. Ascaris lumbricoides infection induces both, reduction and increase of asthma symptoms in a rural community. Acta Trop. (2018) 187:1–4. doi: 10.1016/j.actatropica.2018.07.016
63. Liao EC, Lin YH, Chiu CL, Lin TC, and Tsai JJ. Identification of allergenic component Tyr p 8 from Tyrophagus putrescentiae and cross-reactivity with Der p 8. Clin Vaccine Immunol. (2013) 20:506–12. doi: 10.1128/CVI.00633-12
64. Slater JE, James R, Pongracic JA, Liu AH, Sarpong S, Sampson HA, et al. Biological potency of German cockroach allergen extracts determined in an inner city population. Clin Exp Allergy. (2007) 37:1033–9. doi: 10.1111/j.1365-2222.2007.02751.x
65. Satinover SM, Reefer AJ, Pomes A, Chapman MD, Platts-Mills TA, and Woodfolk JA. Specific IgE and IgG antibody-binding patterns to recombinant cockroach allergens. J Allergy Clin Immunol. (2005) 115:803–9. doi: 10.1016/j.jaci.2005.01.018
66. Beck AF, Huang B, Kercsmar CM, Guilbert TW, McLinden DJ, Lierl MB, et al. Allergen sensitization profiles in a population-based cohort of children hospitalized for asthma. Ann Am Thorac Soc. (2015) 12:376–84. doi: 10.1513/AnnalsATS.201408-376OC
67. Cohn RD, Arbes SJ Jr, Jaramillo R, Reid LH, and Zeldin DC. National prevalence and exposure risk for cockroach allergen in U.S. households. Environ Health Perspect. (2006) 114:522–6. doi: 10.1289/ehp.8561
68. Bonnefoy X, Kampen H, and Sweeney K. Public Health Significance of Urban Pests. Copenhagen: World Health Organization (2008).
69. Roth L. A revision of the cockroach genus parasymploce (Dictyoptera: Blattaria: Blattellidae). J Nat Hist. (1985) 19:431–532. doi: 10.1080/00222938500770321
70. Bell WJ, and Adiyodi K. The American Cockroach. London: Springer Science & Business Media (1982). doi: 10.1007/978-94-009-5827-2
71. Barbosa MC, Santos AB, Ferriani VP, Pomés A, Chapman MD, and Arruda LK. Efficacy of recombinant allergens for diagnosis of cockroach allergy in patients with asthma and/or rhinitis. Int Arch Allergy Immunol. (2013) 161:213–9. doi: 10.1159/000346318
72. Sharma S, Arora B, Gaur SN, and Arora N. Bioinformatic and immunological investigation of Per a 5 (delta class GST) allergen from Periplaneta americana. Mol Immunol. (2021) 132:93–101. doi: 10.1016/j.molimm.2021.01.026
73. Lee MF, Song PP, Hwang GY, Lin SJ, and Chen YH. Sensitization to Per a 2 of the American cockroach correlates with more clinical severity among airway allergic patients in Taiwan. Ann Allergy Asthma Immunol. (2012) 108:243–8. doi: 10.1016/j.anai.2012.01.014
74. Auriault C, Gras-Masse H, Pierce RJ, Butterworth AE, Wolowczuk I, Capron M, et al. Antibody response of Schistosoma mansoni-infected human subjects to the recombinant P28 glutathione-S-transferase and to synthetic peptides. J Clin Microbiol. (1990) 28:1918–24. doi: 10.1128/JCM.28.9.1918-1924.1990
75. Mutapi F, Bourke C, Harcus Y, Midzi N, Mduluza T, Turner CM, et al. Differential recognition patterns of Schistosoma haematobium adult worm antigens by the human antibodies IgA, IgE, IgG1 and IgG4. Parasite Immunol. (2011) 33:181–92. doi: 10.1111/j.1365-3024.2010.01270.x
76. Caraballo L, Acevedo N, and Zakzuk J. Ascariasis as a model to study the helminth/allergy relationships. Parasite Immunol. (2019) 41:e12595. doi: 10.1111/pim.12595
77. Zakzuk J, Mercado D, Bornacelly A, Sanchez J, Ahumada V, Acevedo N, et al. Hygienic conditions influence sensitization to Blomia tropicalis allergenic components: results from the FRAAT birth cohort. Pediatr Allergy Immunol. (2019) 30:172–8. doi: 10.1111/pai.13004
78. Lozano AM, Kong Y, López JF, Bustillo J, Caraballo L, and Zakzuk J. Description of a new allergenic member of the glutathione transferase (GST) family from ascaris with omega-class features. J Allergy Clin Immunol. (2018) 141:AB176. doi: 10.1016/j.jaci.2017.12.560
79. Frova C. Glutathione transferases in the genomics era: new insights and perspectives. Biomol Eng. (2006) 23:149–69. doi: 10.1016/j.bioeng.2006.05.020
80. Wang J, Gao S, Mostovoy Y, Kang Y, Zagoskin M, Sun Y, et al. Comparative genome analysis of programmed DNA elimination in nematodes. Genome Res. (2017) 27:2001–14. doi: 10.1101/gr.225730.117
81. Dereeper A, Guignon V, Blanc G, Audic S, Buffet S, Chevenet F, et al. Phylogeny.fr: robust phylogenetic analysis for the non-specialist. Nucleic Acids Res. (2008) 36 (Web Server issue):W465–9. doi: 10.1093/nar/gkn180
82. Letunic I, and Bork P. Interactive tree of life (iTOL) v3: an online tool for the display and annotation of phylogenetic and other trees. Nucleic Acids Res. (2016) 44:W242–5. doi: 10.1093/nar/gkw290
83. Ouaissi A, Ouaissi M, and Sereno D. Glutathione S-transferases and related proteins from pathogenic human parasites behave as immunomodulatory factors. Immunol Lett. (2002) 81:159–64. doi: 10.1016/S0165-2478(02)00035-4
84. Aguayo V, Valdés Fernandez BN, Rodríguez-Valentín M, Ruiz-Jiménez C, Ramos-Benítez MJ, Méndez LB, et al. Fasciola hepatica GST downregulates NF-κB pathway effectors and inflammatory cytokines while promoting survival in a mouse septic shock model. Sci Rep. (2019) 9:2275. doi: 10.1038/s41598-018-37652-x
85. Sarazin A, Dendooven A, Delbeke M, Gatault S, Pagny A, Standaert A, et al. Treatment with P28GST, a schistosome-derived enzyme, after acute colitis induction in mice: decrease of intestinal inflammation associated with a down regulation of Th1/Th17 responses. PLoS ONE. (2018) 13:e0209681. doi: 10.1371/journal.pone.0209681
86. Capron M, Béghin L, Leclercq C, Labreuche J, Dendooven A, Standaert A, et al. Safety of P28GST, a protein derived from a schistosome helminth parasite, in patients with crohn's disease: a pilot study (ACROHNEM). J Clin Med. (2019) 9:41. doi: 10.3390/jcm9010041
87. Diemert DJ, Pinto AG, Freire J, Jariwala A, Santiago H, Hamilton RG, et al. Generalized urticaria induced by the Na-ASP-2 hookworm vaccine: implications for the development of vaccines against helminths. J Allergy Clin Immunol. (2012) 130:169–76.e6. doi: 10.1016/j.jaci.2012.04.027
88. Acevedo N, Sánchez J, Erler A, Mercado D, Briza P, Kennedy M, et al. IgE cross-reactivity between ascaris and domestic mite allergens: the role of tropomyosin and the nematode polyprotein ABA-1. Allergy. (2009) 64:1635–43. doi: 10.1111/j.1398-9995.2009.02084.x
89. Weatherhead JE, Gazzinelli-Guimaraes P, Knight JM, Fujiwara R, Hotez PJ, Bottazzi ME, et al. Host immunity and inflammation to pulmonary helminth infections. Front Immunol. (2020) 11:594520. doi: 10.3389/fimmu.2020.594520
90. Marretta J, and Casey FB. Effect of Ascaris suum and other adjuvants on the potentiation of the IgE response in guinea-pigs. Immunology. (1979) 37:609–13.
91. Lee TD, and McGibbon A. Potentiation of IgE responses to third-party antigens mediated by Ascaris suum soluble products. Int Arch Allergy Immunol. (1993) 102:185–90. doi: 10.1159/000236570
92. Ahumada V, García E, Dennis R, Rojas MX, Rondón MA, Pérez A, et al. IgE responses to ascaris and mite tropomyosins are risk factors for asthma. Clin Exp Allergy. (2015) 45:1189–200. doi: 10.1111/cea.12513
93. Layland LE, Straubinger K, Ritter M, Loffredo-Verde E, Garn H, Sparwasser T, et al. Schistosoma mansoni-mediated suppression of allergic airway inflammation requires patency and Foxp3+ Treg cells. PLoS Negl Trop Dis. (2013) 7:e2379. doi: 10.1371/journal.pntd.0002379
Keywords: allergen, house dust mite, Ascaris, cockroach allergen, glutathione S-transferase, IgE, tropics
Citation: Zakzuk J, Lozano A and Caraballo L (2021) Allergological Importance of Invertebrate Glutathione Transferases in Tropical Environments. Front. Allergy 2:695262. doi: 10.3389/falgy.2021.695262
Received: 14 April 2021; Accepted: 19 May 2021;
Published: 14 June 2021.
Edited by:
Paolo Colombo, Institute for Biomedical Research and Innovation, National Research Council (CNR), ItalyReviewed by:
Helton Da Costa Santiago, Federal University of Minas Gerais, BrazilCopyright © 2021 Zakzuk, Lozano and Caraballo. This is an open-access article distributed under the terms of the Creative Commons Attribution License (CC BY). The use, distribution or reproduction in other forums is permitted, provided the original author(s) and the copyright owner(s) are credited and that the original publication in this journal is cited, in accordance with accepted academic practice. No use, distribution or reproduction is permitted which does not comply with these terms.
*Correspondence: Josefina Zakzuk, anpha3p1a3NAdW5pY2FydGFnZW5hLmVkdS5jbw==
Disclaimer: All claims expressed in this article are solely those of the authors and do not necessarily represent those of their affiliated organizations, or those of the publisher, the editors and the reviewers. Any product that may be evaluated in this article or claim that may be made by its manufacturer is not guaranteed or endorsed by the publisher.
Research integrity at Frontiers
Learn more about the work of our research integrity team to safeguard the quality of each article we publish.