- 1Department of Psychiatric Research, Akershus University Hospital, Nordbyhagen, Norway
- 2Inserm UMR1239, Laboratory of Neuronal and Neuroendocrine Differentiation and Communication, University of Rouen Normandy, Rouen, France
Adrenocorticotropic hormone together with arginine vasopressin and oxytocin, the neuropeptides regulating the stress response and the hypothalamic-pituitary-adrenal axis activity, are known to modulate aggressive behavior. The functional role of the adrenocorticotropic hormone immunoglobulin G autoantibodies in peptidergic signaling and motivated behavior, including aggression, has been shown in experimental and in vitro models. This review summarizes some experimental data implicating autoantibodies reactive with stress-related peptides in aggressive behavior.
Introduction
In humans, aggression has developed to become part of our defense and protection, but it may also be a symptom reflecting certain medical conditions. As a state of mind, it may be directed towards objects, animals, or human beings, without any obvious motive, and aggression could be a means of self-infliction or be a result from illegal use of drugs and anabolic steroids. It may be elicited by provocation (1, 2) and be detrimental to a person's health through stress as in e.g. cardiovascular disorders (3, 4). As stress-related, aggression involves cortisol and activity in the hypothalamic–pituitary axis (HPA axis) (5).
In his theory of the general adaptation syndrome, Hans Selye emphasized the role of the immune system following the response to stress (6). Since then, we have learned that stress is a broad category including some aversive events which can elicit an aggressive response (1), and that the immune system interferes with normal and pathological brain functioning and behavior (7). Pheromones and odors from the urine have been associated with aggressive behavior (8) and over the years, scientists have had several hypothesis such as the frustration-aggression hypothesis proposed as early as in 1941 (9). It is currently accepted that aggressive behavior can be viewed as a strategy by humans and animals to cope with stress, implying that neurobiological mechanisms involved in stress responses should underlie both physiological and pathological aggression (10–13). Studies of the HPA axis, has later linked the brain's control of cortisol secretion via pituitary release of the adrenocorticotropic hormone (ACTH) (14). Both deficient and increased activation of the HPA axis have been associated with aggressive behavior and Cortisol suppresses the activity of the HPA axis through a mechanism of negative feedback. Cortisol also modulates behavioral modalities including anxiety and distress (15), and diminishes the production of testosterone (16). Berkowitz (17) was convinced that high aggressive drive together with personality factors could explain aggression displacement whereas hypo-arousal-associated aggressiveness, a proposed characteristic of antisocial personality disorder, has been linked to glucocorticoid deficiency (18). In contrast, hyper-arousal-driven aggressiveness, which could be related to the acute exaggerated glucocorticoid response to stress, can be seen in conditions such as post-traumatic stress disorder (PTSD) and intermittent explosive disorder. In fact a study showed that more than twice the individuals with diagnosed intermittent explosive disorder (IED) met the PTSD criteria, compared to individuals without IED(19).
After the introduction of the neuropeptide concept (20, 21) further studies have revealed that peptide hormones are the key modulators of the homeostasis, stress response, and motivated behavior (22, 23). In this regard, not only the centrally produced, but also peripherally derived peptides can access the brain (24), including transport across the blood-brain barrier (25), and diffusion together with macromolecules via the perivascular spaces (26). The circumventricular organs in the brain, with their extensive and highly permeable capillaries, represent important sites of action of peripheral peptide hormones, e.g. the median eminence located in the vicinity of the ventromedial hypothalamic nucleus involved in the regulation of aggressive behavior (27). Thus, aggressive behavior may involve specific brain circuitries and activation of the HPA axis as a mechanism of altered response to stress, however, the biological background is so far not fully understood (28–31).
Immunoglobulins (Ig) or autoantibodies (autoAbs) reactive with neuropeptides and peptide hormones have been identified in humans and rodents showing associations of their plasma levels with aggressive or antisocial behavior, anxiety, and depression. For instance, in 2002 Fetissov et al. described IgG reactive with melanocortin peptides alpha-melanocyte-stimulating hormone (α-MSH) and ACTH in patients with eating disorders (ED) (32), results which later were followed by data showing increased plasma levels of ACTH-reactive autoAbs in subjects with increased aggressive and antisocial behavior (33). Most recently, a modulatory role of ACTH-reactive IgG in ACTH-induced cortisol secretion was demonstrated (34).
Understanding the modulatory role of autoAbs reactive with stress-related peptide hormones represents a new approach to aggressive behavior. Few studies are published on this immuno-modulated behavior, and the purpose of this review is to present the most recent knowledge integrating such autoAbs in neurobiological mechanisms of aggression.
Subtypes of Aggressive Behavior
There are long traditions of claiming that aggression falls into proactive or reactive types and that the basis for aggressive behavior is to inflict harm (12). Human aggression varies from purely reactive cases with unplanned fighting and strong emotions, to purely proactive, premeditated, and deliberate efforts to harm (35).
Reactive aggression is a response to a threat or a frustrating event, with the goal being only to remove the provoking stimulus. Reactive aggression is always associated with anger, as well as with a sudden increase in sympathetic activation and a failure of cortical regulation. In animals, reactive aggression is typically a response by the defender without any proactive elements (35), such as when a fight concerns food, whereas proactive aggression is seen rarer in most species.
Proactive aggression may refer to a planned attack with a purpose driven by an external or internal reward, and the proactivity is characterized by attention to a consistent target, and often by a lack of emotional arousal. Psychologists often distinguish between two different types of aggression, impulsive and instrumental. Impulsive or affective aggression with strong anger is not planned and it usually occurs during the heat of the moment, whereas in instrumental or predatory aggression, the aggressive behavior is goal oriented and thus normally well planned.
In this review, focus is set on the type of aggression seen in criminals sentenced to imprisonment due to their impulsive violent and extreme antisocial actions, but also where there are elements of both proactivity and premeditation, as well as of impulsivity and other personality issues. Consequently, some forms of aggressive behavior can be difficult to classify as being either one or the other, since an analysis of the kind of aggression observed in practice, often contains elements from various defined categories.
Hypothalamic-Pituitary-Adrenal Axis
The HPA axis refers to the interaction between the hypothalamus, the pituitary gland and the adrenal cortex, and the secretion of hormones involved in the stress response. This interaction is important for the early development and later consolidation of human behavior.
Neuronal co-localization of functionally related peptides is important for an immediate physiological response in which more than one transmitter participates. Neuropeptides, normally involved as a part of long-term response to stress, e.g. a trauma or an allergic- or inflammatory reaction, need more time to upregulate than classical neurotransmitters (36). Corticotropin-releasing hormone (CRH) links the HPA axis (14) to the brain's response with stress required behavior, and its activity may thus influence anxiety and stress reactions (15). For human beings, the impact of stress already experienced during a child's early rearing environment may influence the development of later psychopathology and possibly identify hormonal substrates related to behavioral changes as the child gets older (37). Recent data have revealed that the HPA axis and associated stress-related behavior can be influenced by immunoglobulins or natural autoAbs reactive with peptide hormones involved in regulation of the HPA axis activity (34). Furthermore, experimental studies have shown enhanced activation of the hypothalamic paraventricular nucleus and amygdala in glucocorticoid-deficient rats after exposure to the resident intruder (RI) stress protocol (33, 38).
Corticotrophin-Releasing Hormone
Specific neurons of the paraventricular nucleus (PVN) of the hypothalamus secrete CRH in response to stress. Under physiological conditions, its secretion varies during the 24 h cycle of the day; being highest in the morning and lowest during the night. The stimulation of ACTH secretion into the blood stream leads to the release of cortisol from the adrenal cortex (39). This is an automatic regulation based on negative feedback so that the blood levels of cortisol shuts down the relevant CRH release activity in the hypothalamus, thereby preventing CRH levels from becoming too high (40). It is related to aggressive behavior as part of the stress response (41).
Adrenocorticotrophic Hormone
ACTH consists of 39 amino acids and is a peptide originating from the precursor pro-opiomelanocortin (POMC) (42), synthetized mainly in the pituitary and in the brain. CRH stimulate the synthesis and secretion of ACTH and act in synergy with the central nervous modulatory effects of arginine vasopressin (AVP), releasing stored ACTH from corticotropic cells. ACTH binds to the melanocortin type 2 G protein-coupled receptor (MC2R) expressed in the fasciculate and reticular zones of the adrenal cortex (39, 43), and triggers intracellular signaling pathways regulating the adrenal cortisol production. Acute administrations of ACTH fragments increase fighting in mice, independently of corticosterone secretion (44), but ACTH injections in isolated mice may also decrease their aggressiveness (45). It was shown that there is a link between ACTH and aggressive behavior (46), and recently this link has been strengthened through studies on ACTH autoAbs (34).
Cortisol
Cortisol is a steroid and the body's main stress hormone, released from the adrenal cortex. One of the first studies described a model in which the HPA axis was linked to aggression (47) and later, cortisol and aggression were seen in wrestlers who after fighting showed an increased level in serum cortisol (48). Cortisol is known from general physiology to be released during stress (49), and it contributes positively to the hormonal balance throughout the body, and most of our cells have cortisol receptors. Examples of cortisol functions are control of blood sugar levels, regulation of metabolism, anti-inflammatory effects, help to forming our memory, and depression (50).
Melanocyte-Stimulating Hormone
The peptide hormone and neuropeptide melanocyte-stimulating hormone (MSH), is produced by the brain and pituitary and consists of α-MSH, β-MSH, and γ-MSH, which are in the family of melanocortin peptides. The sequence of α-MSH consists of the 13 first amino acids of the ACTH molecule with antibody cross-reactivity as a consequence since antibodies to ACTH and α-MSH are not specific and will detect POMC, but only to an unknown degree (51).
Experiments on male mice showed that when a dominant/subordinate pair was injected 15 min before the testing with α-MSH, the attacks on the α-MSH-treated animal were more frequent compared to when the MSH was administered 24 h before testing (52) indicating that α-MSH increases aggressive behavior. In the context of externalizing behavior, α-MSH involvement in stress (32) and aggression has been associated with melanocortin peptides since injection of α-MSH or ACTH fractions (amino acids 4–10) (45) induced aggression in mice. In addition, the melanocortin peptide pharmacophore also seems necessary for the pro-aggressive ACTH effects.
Oxytocin
Oxytocin (OT) and AVP are both nine amino acid peptide hormones (53) and their sequences differ by two amino acids (54). OT is acting as a neuromodulator in the brain regulating social and sexual behavior. It is involved in anxiety and stress response, and in aggression (22, 55, 56). OT has protective effects against stress and studies have shown that it modulates neural circuitry for social cognition and fear in humans (57), and may disrupt the common output from the amygdala to the rat brainstem effector sites of the autonomic nervous system (58). Intracerebral OT modulation is known to inhibit stress-induced activity of the HPA axis (59, 60), causing behavioral and neural effects such as reduced anxiety (61). Administration of OT with concomitant social support during stress exposure provides the lowest cortisol response and an anxiolytic effect (62).
Arginine Vasopressin
AVP has several peripheral and central functions, but relevant to this review regarding aggressive behavior, AVP functions as a neuromodulator. Its role in the central nervous system (CNS) seems to depend on the region in which AVP is released, including modulation of aggressive behavior (22, 56, 63, 64). AVP and CRH are found to co-exist in CRH nerve terminals (65, 66), and together with CRH, AVP strongly potentiates its ACTH-releasing activity (67, 68). As to the regulation, the function of the HPA axis has shown that in acute stress, CRH is a major player causing increased ACTH secretion, whilst in chronic stress; AVP modulation takes over as the main stimulator of ACTH release (69).
During an RI test, release of AVP, specifically in the hypothalamic mediolateral septum, was found to regulate intermale aggression in laboratory rats specifically bred for low (LAB)- or high (HAB)- anxiety‐related behavior (63). During the test exposure, LAB residents showed more aggression than the HAB residents, and the septal AVP release was found decreased in high-aggressive LAB rats compared to HAB males. Studying the patterns of AVP release within the hypothalamic mediolateral septum in the two respective groups of rats, revealed that changes in AVP release varied with intermale aggressive behavior. Thus, high levels of aggressive behavior, as seen in LAB residents, were associated with decreased release of AVP in the septum. On the other hand, the low levels of aggression found in HAB residents were associated with an increase in septal AVP release (63). Furthermore, during exposure to a non-social stressor, LAB rats responded with a stronger rise in plasma ACTH compared with HAB rats (70–72), reflecting a generally lower stressor susceptibility in the latter group, and at the same time, presenting a low trait anxiety. These findings are somewhat in line with the evidence that innate anxiety is inversely related to the level of intermale aggressive behavior (46).
Immunoglobulins
Ig are natural antibodies produced by B1 cells, including the processes in germ-free animals after activation by both T cell-dependent and independent mechanisms (73). Ig's are divided into five classes or isotypes of which IgG is the most common type of antibody.
An autoAb is an antibody directed against one or more of the individual's own proteins. The natural autoAbs are part of normal physiology of the innate and adaptive immune defense, and may in addition be involved in several homeostatic functions (74), e.g. in removal of old erythrocytes (75), and fighting any invaders or toxins in the body, including e.g. bacteria and viruses. The various IgG autoAbs are relatively stable throughout life as opposed to IgG-reactive to bacterial antigens, demonstrating individual differences which in turn increase as we get older (76). Natural autoAbs of immunoglobulin M (IgM), IgG and immunoglobulin A (IgA) classes are present in all human beings without health problems (74, 77), they are polyreactive, and bind with different affinities to a variety of unrelated antigens, including those from micro-organisms.
Although the functional role of peptide hormone-reactive autoabs still needs to be further clarified, it appears that autoabs play a role in the transportation of peptide hormones and cytokines (78–81), and they seem to protect these peptides from degradation by plasma enzymes, thereby preserving biological activity (82).
ACTH-Reactive Autoabs
The few studies on ACTH IgG autoAbs in human aggression shown in Table 1 are those we are left with after thorough research in available databases.
It has been shown that autoAbs reactive with peptide hormones of the HPA axis are naturally present in rodents and in healthy humans (84), and may contribute to a more general regulation of motivated behavior, emotion, and stress-response.
Results from RI tests have shown an ACTH increase only for the intruders, but not in the aggressive residents (85). Reduced and increased stimulation of the HPA axis are to be linked to aggressive behavior (85), possibly reflecting a coping strategy to stress exposure (11, 12). With this in mind, increased plasma levels of IgG ACTH autoAbs have been found in perpetrators with increased aggressive and antisocial behavior, thereby suggesting a possible association (33). Experiments on violent criminals and non–violent individuals describe elevated levels of ACTH autoAbs which may block ACTH secretion and an instant cortisol release during stress. The blocking and non-blocking IgG effects (34) have confirmed a role for ACTH autoAbs in the HPA axis response, but at the same time makes it less likely that aberrant IgG modulation of ACTH induced cortisol response can be causal for violent aggression.
A link between autoAbs and behavior (33) is supported by a study on the general population which found increased levels of ACTH-reactive IgG in adolescent males with antisocial behavior. Psychological stress-induced cortisol release in adolescents has been found to be negatively associated with anti-ACTH IgG levels (83) and hence, it is possible that some ACTH-reactive IgG may have the analogue blocking properties as described, thereby preventing ACTH-induced activation of cortisol.
In the latest study on aggression and ACTH autoAbs, ACTH-reactive IgG were found elevated in prisoners sentenced for common crimes, but not in the extremely violent aggressors (34). An ACTH epitope overview showed that IgG binding for the cortisol responders in the group of non-aggressive controls, occur at the ACTH sequence containing the MC2R pharmacophore KKRRP (amino acids 11–24). Violent aggressors showed no binding to the ACTH amino acids 11–24, but instead to the amino acids 1–13, a section containing the melanocortin pharmacophore HFRW (34) (see Figure 1).
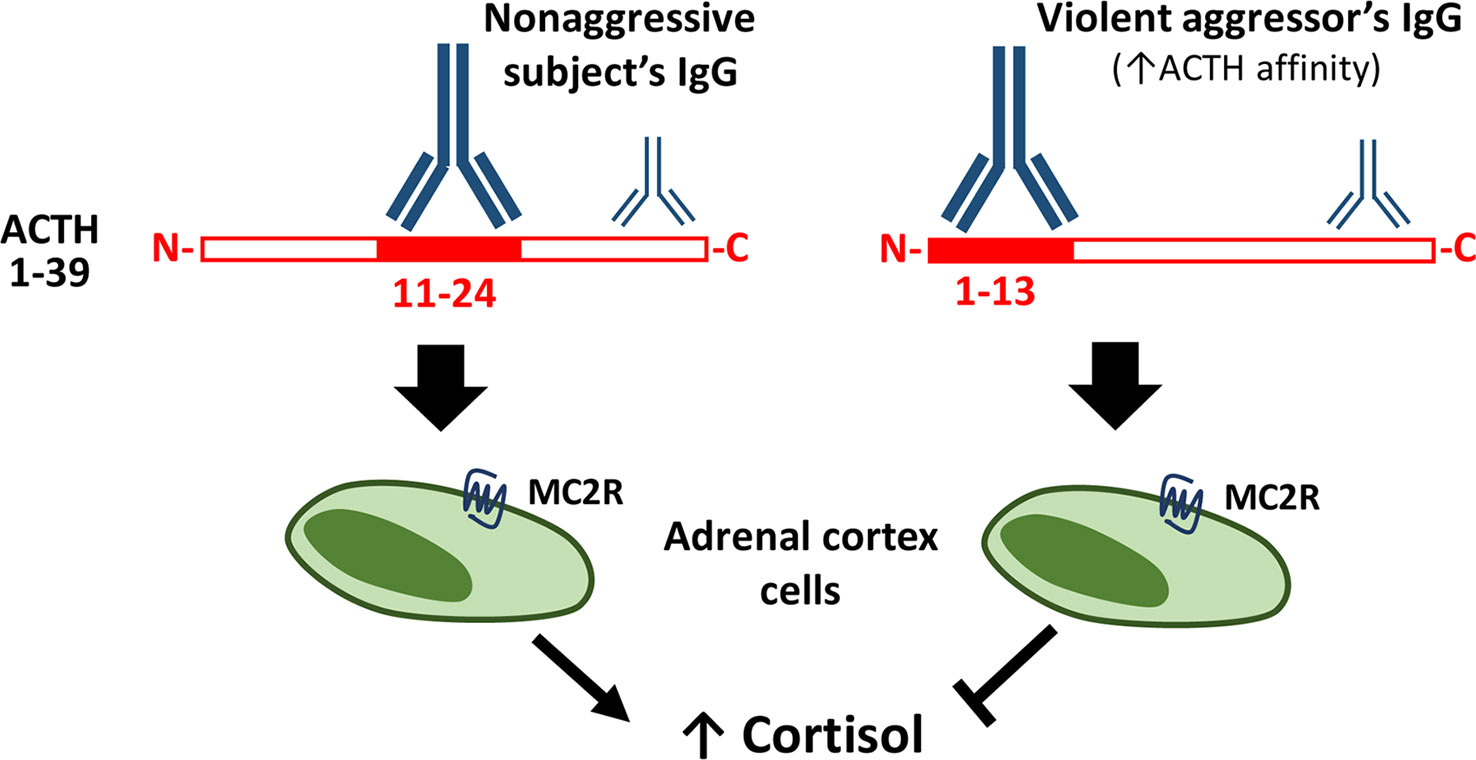
Figure 1 Plasma ACTH-reactive IgG modulate ACTH-induced cortisol secretion. Legend to Figure 1. ACTH-reactive IgG are naturally present in human plasma and modulate ACTH-induced cortisol secretion depending on the ACTH binding epitope. In the non-aggressive subjects IgG bind mainly the central part (11–24) of ACTH, containing the MC2R pharmacophore, while IgG in violent aggressors display increased affinity for ACTH and bind mainly its N-terminal part (1–13). Some IgG from both the non-aggressive and aggressive study persons have both been shown to prevent ACTH-induced cortisol secretion from the adrenal cortex cells, such inhibitory effect was associated with low IgG binding to the central ACTH part (11–24), i.e. similar to the binding pattern characterizing IgG of violent aggressors (34).
Some previously mentioned associations between ACTH autoAbs and α–MSH (32) and the blocking and non-blocking properties of the ACTH autoAbs in behavioral conditions (33) have been confirmed in the latest study, and a further step has been made, linking different epitope binding sites to non-aggressive subjects and extreme violent aggressors (34).
A lower association rate of IgG for ACTH, found in boys with antisocial behavior indicate that such autoAbs are different, not only by their plasma levels, but also possibly by their molecular structure (83). Consequently, since questions regarding potential differences in molecular structure still have to be clarified, final conclusions are premature
In a recent study applying the RI test, ACTH IgG injections from severely aggressive criminals shortened the latency for the first attack without affecting the total number of the resident's attacks (34). In addition, aggressive behavior was reduced in resident mice receiving ACTH together with IgG from non-aggressive controls (34). Other studies on rodents indicate that a rapid positive feedback exists, in which a social challenge or stressors unrelated to aggression can activate the HPA axis (86), (87).
It has been found that administration of ACTH alone, peripherally in resident mice, did not alter their aggressive behavioral expression significantly, but when co-injecting ACTH together with IgG from violent aggressors, the latency of the first attack was reduced without affecting the total number of attacks (34). Furthermore, the aggressive behavior was reduced in resident mice who received the combination of ACTH and IgG from non-aggressive controls. Such behavioral responses are supportive for a role where peripheral IgG regulates both impulsive and defensive aggressive behavior (34), and applying this model on healthy individuals, a result could be that plasma IgG may suppress natural aggressiveness (34).
OT-Reactive autoAbs
Relevance of autoAbs reactive with OT to human behavior has been shown by statistically significant correlation between levels of anti-OT IgG in plasma and conditions with symptoms of anxiety (88). Plasma anti-OT IgM has been found to correlate with interceptive awareness, maturity fears (89). Lower plasma levels of OT reactive IgG autoAbs were also found in patients suffering from moderate levels of major depressive disorder, levels which correlated negatively with the Montgomery and Aasberg Depression Rating Scale (MADRS) (90).
Elevated levels of IgM autoAbs against OT are found in male subjects with conduct disorder (CD) and in prison inmates as compared with non-aggressive healthy controls (33). Considering OT's modulatory role in the HPA axis, increased levels of OT-reactive autoAbs may possibly interfere with OT-mediated inhibition of HPA axis during stress response in the hypo-arousal type of aggressive behavior frequently characterizing CD. Various levels of OT and AVP reactive autoAbs in patients with CD suggest that the observed changes may result in an increased AVP to OT ratio and consequently to aggressive and antisocial behavior. Furthermore, significant changes in the respective levels of OT- and AVP-reactive autoAbs may represent a factor influencing the central mechanisms behind aggressive behavior (33).
AVP-Reactive autoAbs
Studies of autoAbs in mild and moderate depression have shown that mood changes can be associated with changes in antibody levels. However, such changes were not seen in binding the affinity of OT- and AVP-reactive autoAbs. Moreover, the levels of AVP-reactive autoAbs are associated with cortisol secretion (90). Low levels of total plasma AVP-reactive IgG autoAbs have been found in a greater proportion of depressed patients, and the free AVP IgG autoAbs showed positive correlation with plasma cortisol after physical activity (90). Similarly to anti-OT IgG, plasma levels of AVP-reactive IgG correlated with symptoms of anxiety and somatization (88) and that the levels of CRH-reactive IgG in plasma of healthy subjects correlate positively with obsessive or hypochondriac behavior, but negatively (as anti-AVP IgG) with somatization (88).
The central effects, including behavior, of AVP is as a neuromodulator. In male subjects with CD, both plasma levels of IgG and IgM classes of autoAbs reactive with AVP, have been found lower than in non-aggressive controls, but in some prison inmates, anti-AVP IgG levels were found elevated (33). If a role of the autoAbs is to protect and transport the peptide, such changes of levels of AVP-reactive autoAbs, would signify a diminished AVP-modulatory activation of the HPA axis and thus, being in agreement with the hypo-arousal theory of antisocial and aggressive behavior (91) in CD (33).
α-MSH-Reactive autoAbs
Recent data suggest that α-MSH autoAbs can interfere with normal signal transduction in the melanocortin type 4 receptors, involved in the regulation of feeding behavior (32, 92). AutoAbs reacting with α-MSH could play a pathogenic role in psychiatric-behavioral problems in subjects with ED (93), but the presence of α-MSH- and/or ACTH- reactive autoAbs does not imply the presence of dysfunctional feeding behavior, the molecular properties of such autoAbs underlie their physiological or pathogenic role (93). Stress and gut microbiota composition may represent common denominators for production of α-MSH- and ACTH- reactive antibodies with various binding properties (80, 93, 94).
Whether or not autoAbs can pass across the blood–brain barrier (BBB) or reach the brain possibly via circumventricular organs, is a matter of controversy. It has been claimed that certain regulatory peptides may cross the BBB in both directions (95) and that such passing can be blocked by corresponding antibodies (96). There is a study (97) showing that the tritiated synthetic ACTH (4–10) analogue, Met-Glu-His-Phe-Pro-Gly-Pro has been detected in brain extracts at two time points (97), which according to the hypothesis suggests penetration into the brain tissues (97). Thus, further evidence is needed to strengthen any claims that the BBB function allows crossing of autoAbs, including supportive evidence of their potential modulatory role in peptide transport to the brain.
Conclusions
Since the initial finding linking aggressive behavior to corticotrophin autoAbs by showing their high plasma levels in prisoners and in subjects with CD, there has been a development. Indeed, ACTH IgG were significantly associated with antisocial behavior and the HPA axis response in stressed adolescents. As such, higher plasma levels of ACTH IgG were present in boys with increased antisocial behavior. Girls however, showed a different picture, where the antisocial behavior was associated with low ACTH IgG levels. Moreover, recent studies were able, at least partly, to clarify the molecular mechanisms underlying an observed link between ACTH autoAbs, stress response, and aggressive behavior. It was found that in some subjects that ACTH IgG may prevent ACTH-induced cortisol secretion i.e. may disrupt a normal HPA activation. Such IgG blocking properties were associated with specific ACTH binding epitopes which were more prevalent in prisoners who had committed acts of violent aggression. Furthermore, experiments in mice showed ability of plasmatic IgG of aggressive subjects to facilitate ACTH-induced attacks in the resident-intruder test. Taken together, these data support a mechanistic role of ACTH-reactive autoAbs in the stress-related aggressive behavior. Moreover, since autoAbs reactive to others than ACTH stress-related peptides, such as OT and VP, were found at different levels in subjects with various neuropsychiatric disorders, it is likely that they may also modulate stress-induced aggressive behavior. Further studies should clarify the functional effects and origin of such autoAbs aiming at a better understanding of the neurobiological mechanism of aggressive behavior.
Limitations and Strengths
A limitation of this review is the low number of studies published on immunobiology of human aggression including peptide hormone-reactive autoAbs. Supportive for further research on ACTH IgG in aggression is that results from studies in other clinical conditions and disorders have underlined the functional significance for autoAbs also in a topic like aggressive behavior. In this regard, we believe that recent data from studies on the microbial antigens and peptide hormone cross-reactivity as well as the gut microbiota-brain axis will influence future design of studies.
Author Contributions
All authors listed have made substantial, direct, and intellectual contribution to the work and approved it for publication.
Conflict of Interest
The authors declare that the research was conducted in the absence of any commercial or financial relationships that could be construed as a potential conflict of interest.
Acknowledgments
HV received a grant from a private sponsor for the study of criminals and their behavior, the Alvhilde Eliassens Research Foundation. SF is supported by The Transversal Microbiota Program of Inserm, France.
References
1. Groves CL, Anderson CA. Aversive events and aggression. Curr Opin In Psychol (2018) 19:144–8. doi: 10.1016/j.copsyc.2017.03.027
2. Bettencourt B, Miller N. Gender differences in aggression as a function of provocation: a meta-analysis. Psychol Bull (1996) 119(3):422. doi: 10.1037/0033-2909.119.3.422
3. Bosma H, Marmot MG, Hemingway H, Nicholson AC, Brunner E, Stansfeld SA. Low job control and risk of coronary heart disease in Whitehall II (prospective cohort) study. BMJ (1997) 314(7080):558. doi: 10.1136/bmj.314.7080.558
4. Templin C, Ghadri JR, Diekmann J, Napp LC, Bataiosu DR, Jaguszewski M, et al. Clinical features and outcomes of Takotsubo (stress) cardiomyopathy. N Engl J Med (2015) 373(10):929–38. doi: 10.1056/NEJMoa1406761
5. Keynejad RC, Frodl T, Kanaan R, Pariante C, Reuber M, Nicholson TR. Stress and functional neurological disorders: mechanistic insights. J Neurol Neurosurg Psychiatry (2019) 90(7):813–21. doi: 10.1136/jnnp-2018-318297
6. Selye H. Stress and the general adaptation syndrome. Br Med J (1950) 1(4667):1383–92. doi: 10.1136/bmj.1.4667.1383
7. Khandaker GM, Cousins L, Deakin J, Lennox BR, Yolken R, Jones PB. Inflammation and immunity in schizophrenia: implications for pathophysiology and treatment. Lancet Psychiatry (2015) 2(3):258–70. doi: 10.1016/S2215-0366(14)00122-9
8. Mugford R, Nowell N. Pheromones and their effect on aggression in mice. Nature (1970) 226(5249):967–8. doi: 10.1038/226967a0
9. Miller NE. The frustration-aggression hypothesis. Psychol Rev (1941) 48(4):337. doi: 10.1037/h0055861
10. De Bellis MD, Van Dillen T. Childhood post-traumatic stress disorder: an overview. Child Adolesc Psychiatr Clin N Am (2005) 14(4):745–72. doi: 10.1016/j.chc.2005.05.006
11. Haller J, Kruk MR. Normal and abnormal aggression: human disorders and novel laboratory models. Neurosci Biobehav Rev (2006) 30(3):292–303. doi: 10.1016/j.neubiorev.2005.01.005
12. Nelson RJ, Trainor BC. Neural mechanisms of aggression. Nat Rev Neurosci (2007) 8(7):536–46. doi: 10.1038/nrn2174
13. de Boer SF, Olivier B, Veening J, Koolhaas JM. The neurobiology of offensive aggression: Revealing a modular view. Physiol Behav (2015) 146:111–27. doi: 10.1016/j.physbeh.2015.04.040
14. Chrousos GP, Gold PW. The concepts of stress and stress system disorders. Overview of physical and behavioral homeostasis. JAMA (1992) 267(9):1244–52. doi: 10.1001/jama.1992.03480090092034
15. Vedhara K, Miles J, Bennett P, Plummer S, Tallon D, Brooks E, et al. An investigation into the relationship between salivary cortisol, stress, anxiety and depression. Biol Psychol (2003) 62(2):89–96. doi: 10.1016/S0301-0511(02)00128-X
16. van Honk J, Peper JS, Schutter DJ. Testosterone reduces unconscious fear but not consciously experienced anxiety: implications for the disorders of fear and anxiety. Biol Psychiatry (2005) 58(3):218–25. doi: 10.1016/j.biopsych.2005.04.003
17. Berkowitz L. Anti-semitism and the displacement of aggression. J Abnormal Soc Psychol (1959) 59(2):182. doi: 10.1037/h0048227
18. Haller J, Mikics E, Halasz J, Toth M. Mechanisms differentiating normal from abnormal aggression: glucocorticoids and serotonin. Eur J Pharmacol (2005) 526(1-3):89–100. doi: 10.1016/j.ejphar.2005.09.064
19. Reardon AF, Hein CL, Wolf EJ, Prince LB, Ryabchenko K, Miller MW. Intermittent explosive disorder: associations with PTSD and other Axis I disorders in a US military veteran sample. J Anxiety Disord (2014) 28(5):488–94. doi: 10.1016/j.janxdis.2014.05.001
20. de Wied D, Gaffori O, Burbach JP, Kovács GL, Van Ree JM. Structure activity relationship studies with C-terminal fragments of vasopressin and oxytocin on avoidance behaviors of rats. J Pharmacol Exp Ther (1987) 241(1):268–74.
21. De Wied D. The neuropeptide concept. Prog Brain Res (1987) 72:93–108. doi: 10.1016/S0079-6123(08)60199-5
22. de Wied D, Diamant M, Fodor M. Central nervous system effects of the neurohypophyseal hormones and related peptides. Front Neuroendocrinol (1993) 14(4):251–302. doi: 10.1006/frne.1993.1009
23. Hökfelt T, Bartfai T, Bloom F. Neuropeptides: opportunities for drug discovery. Lancet Neurol (2003) 2(8):463–72. doi: 10.1016/S1474-4422(03)00482-4
24. Neumann ID, Maloumby R, Beiderbeck DI, Lukas M, Landgraf R. Increased brain and plasma oxytocin after nasal and peripheral administration in rats and mice. Psychoneuroendocrinology (2013) 38(10):1985–93. doi: 10.1016/j.psyneuen.2013.03.003
25. J Kastin A, Pan W. Concepts for biologically active peptides. Curr Pharm Des (2010) 16(30):3390–400. doi: 10.2174/138161210793563491
26. Iliff JJ, Wang M, Liao Y, Plogg BA, Peng W, Gundersen GA, et al. A paravascular pathway facilitates CSF flow through the brain parenchyma and the clearance of interstitial solutes, including amyloid β. Sci Transl Med (2012) 4(147):147ra111–147ra111. doi: 10.1126/scitranslmed.3003748
27. Lin D, Boyle MP, Dollar P, Lee H, Lein E, Perona P, et al. Functional identification of an aggression locus in the mouse hypothalamus. Nature (2011) 470(7333):221. doi: 10.1038/nature09736
28. Summers CH, Winberg S. Interactions between the neural regulation of stress and aggression. J Exp Biol (2006) 209(23):4581–9. doi: 10.1242/jeb.02565
29. de Almeida RMM, Cabral JCC, Narvaes R. Behavioural, hormonal and neurobiological mechanisms of aggressive behaviour in human and nonhuman primates. Physiol Behav (2015) 143:121–35. doi: 10.1016/j.physbeh.2015.02.053
30. Gronek P, Wieliński D, Gronek J. Genetic and non-genetic determinants of aggression in combat sports. Open Life Sci (2015) 10(1):7–17. doi: 10.1515/biol-2015-0002
31. Sohrabi S. BMC Proc: BioMed Central. In: The criminal gene: The link between MAOA and aggression. (2015). p. A49.
32. Fetissov SO, Hallman J, Oreland L, Klinteberg B, Grenbäck E, Hulting A-L, et al. Autoantibodies against α-MSH, ACTH, and LHRH in anorexia and bulimia nervosa patients. Proc Natl Acad Sci (2002) 99(26):17155–60. doi: 10.1073/pnas.222658699
33. Fetissov SO, Hallman J, Nilsson I, Lefvert AK, Oreland L, Hökfelt T. Aggressive behavior linked to corticotropin-reactive autoantibodies. Biol Psychiatry (2006) 60(8):799–802. doi: 10.1016/j.biopsych.2006.03.081
34. Vaeroy H, Adori C, Legrand R, Lucas N, Breton J, Cottard C, et al. Autoantibodies reactive to adrenocorticotropic hormone can alter cortisol secretion in both aggressive and nonaggressive humans. Proc Natl Acad Sci U. S. A. (2018) 115(28):E6576–e6584. doi: 10.1073/pnas.1720008115
35. Wrangham RW. Two types of aggression in human evolution. Proc Natl Acad Sci (2018) 115(2):245–53. doi: 10.1073/pnas.1713611115
36. Ekstrom PA, Kerekes N, Hokfelt T. Leukemia inhibitory factor null mice: unhampered in vitro outgrowth of sensory axons but reduced stimulatory potential by nerve segments. Neurosci Lett (2000) 281(2-3):107–10. doi: 10.1016/S0304-3940(00)00816-8
37. Toth M, Mikics E, Tulogdi A, Aliczki M, Haller J. Post-weaning social isolation induces abnormal forms of aggression in conjunction with increased glucocorticoid and autonomic stress responses. Horm Behav (2011) 60(1):28–36. doi: 10.1016/j.yhbeh.2011.02.003
38. Halász J, Liposits Z, Kruk MR, Haller J. Neural background of glucocorticoid dysfunction-induced abnormal aggression in rats: Involvement of fear-and stress-related structures. Eur J Neurosci (2002) 15(3):561–9. doi: 10.1046/j.0953-816x.2001.01883.x
39. Dutt M, Jialal I. Physiology, Adrenal Gland. StatPearls. Bethesda, USA: StatPearls Publishing (2019).
40. Keller-Wood ME, Dallman MF. Corticosteroid inhibition of ACTH secretion. Endocr Rev (1984) 5(1):1–24. doi: 10.1210/edrv-5-1-1
41. Kehne JH, Cain CK. Therapeutic utility of non-peptidic CRF1 receptor antagonists in anxiety, depression, and stress-related disorders: evidence from animal models. Pharmacol Ther (2010) 128(3):460–87. doi: 10.1016/j.pharmthera.2010.08.011
42. Li CH, Simpson ME, Evans HM. Isolation of Adrenocorticotropic Hormone from Sheep Pituitaries. Science (1942) 96 :450. doi: 10.1126/science.96.2498.450
43. Allen MJ, Sharma S. StatPearls. In: Physiology, Adrenocorticotropic Hormone (ACTH). Bethesda, USA: StatPearls Publishing (2019).
44. Brain PF, Evans AE. Acute influences of some ACTH-related peptides of fighting and adrenocortical activity in male laboratory mice. Pharmacol Biochem Behav (1977) 7(5):425–33. doi: 10.1016/0091-3057(77)90210-6
45. Brain PF, Nowell NW, Wouters A. Some relationships between adrenal function and the effectiveness of a period of isolation in inducing intermale aggression in albino mice. Physiol Behav (1971) 6(1):27–9. doi: 10.1016/0031-9384(71)90008-4
46. Veenema AH, Torner L, Blume A, Beiderbeck DI, Neumann ID. Low inborn anxiety correlates with high intermale aggression: link to ACTH response and neuronal activation of the hypothalamic paraventricular nucleus. Horm Behav (2007) 51(1):11–9. doi: 10.1016/j.yhbeh.2006.07.004
47. Brain PF. Possible role of the pituitary/adrenocortical axis in aggressive behaviour. Nature (1971) 233(5320):489–9. doi: 10.1038/233489a0
48. Elias M. Serum cortisol, testosterone, and testosterone-binding globulin responses to competitive fighting in human males. Aggressive Behav (1981) 7(3):215–24. doi: 10.1002/1098-2337(1981)7:3<215::AID-AB2480070305>3.0.CO;2-M
49. Boolani A, Channaveerappa D, Dupree EJ, Jayathirtha M, Aslebagh R, Grobe S, et al. Trends in analysis of cortisol and its derivatives. In: Advancements Mass Spectrometry In Biomed Research (2019) 1140:649–64. doi: 10.1007/978-3-030-15950-4_39
50. Herbert J. Cortisol and depression: three questions for psychiatry. Psychol Med (2013) 43(3):449–69. doi: 10.1017/S0033291712000955
51. Rousseau K, Kauser S, Pritchard LE, Warhurst A, Oliver RL, Slominski A, et al. Proopiomelanocortin (POMC), the ACTH/melanocortin precursor, is secreted by human epidermal keratinocytes and melanocytes and stimulates melanogenesis. FASEB J (2007) 21(8):1844–56. doi: 10.1096/fj.06-7398com
52. Nowell N, Thody A, Woodley R. α-Melanocyte stimulating hormone and aggressive behavior in the male mouse. Physiol Behav (1980) 24(1):5–9. doi: 10.1016/0031-9384(80)90006-2
53. Terashima Y, Kondo K, Oiso Y. Administration of oxytocin affects vasopressin V2 receptor and aquaporin-2 gene expression in the rat. Life Sci (1999) 64(16):1447–53. doi: 10.1016/S0024-3205(99)00078-8
54. Light A, Du Vigneaud V. On the nature of oxytocin and vasopressin from human pituitary. Proc Soc Exp Biol Med (1958) 98(4):692–6. doi: 10.3181/00379727-98-24154
55. Campbell A. Oxytocin and human social behavior. Pers Soc Psychol Rev (2010) 14(3):281–95. doi: 10.1177/1088868310363594
56. Stoop R. Neuromodulation by Oxytocin and Vasopressin. Neuron (2012) 76(1):142–59. doi: 10.1016/j.neuron.2012.09.025
57. Kirsch P, Esslinger C, Chen Q, Mier D, Lis S, Siddhanti S, et al. Oxytocin modulates neural circuitry for social cognition and fear in humans. J Neurosci (2005) 25(49):11489–93. doi: 10.1523/JNEUROSCI.3984-05.2005
58. Huber D, Veinante P, Stoop R. Vasopressin and oxytocin excite distinct neuronal populations in the central amygdala. Science (2005) 308(5719):245–8. doi: 10.1126/science.1105636
59. Neumann ID. Involvement of the brain oxytocin system in stress coping: interactions with the hypothalamo-pituitary-adrenal axis. Prog Brain Res (2002) 139:147–62. doi: 10.1016/S0079-6123(02)39014-9
60. Parker KJ, Buckmaster CL, Schatzberg AF, Lyons DM. Intranasal oxytocin administration attenuates the ACTH stress response in monkeys. Psychoneuroendocrinology (2005) 30(9):924–9. doi: 10.1016/j.psyneuen.2005.04.002
61. Heinrichs M, von Dawans B, Domes G. Oxytocin, vasopressin, and human social behavior. Front Neuroendocrinol (2009) 30(4):548–57. doi: 10.1016/j.yfrne.2009.05.005
62. Heinrichs M, Baumgartner T, Kirschbaum C, Ehlert U. Social support and oxytocin interact to suppress cortisol and subjective responses to psychosocial stress. Biol Psychiatry (2003) 54(12):1389–98. doi: 10.1016/S0006-3223(03)00465-7
63. Beiderbeck DI, Neumann ID, Veenema AH. Differences in intermale aggression are accompanied by opposite vasopressin release patterns within the septum in rats bred for low and high anxiety. Eur J Neurosci (2007) 26(12):3597–605. doi: 10.1111/j.1460-9568.2007.05974.x
64. Veenema AH, Beiderbeck DI, Lukas M, Neumann ID. Distinct correlations of vasopressin release within the lateral septum and the bed nucleus of the stria terminalis with the display of intermale aggression. Horm Behav (2010) 58(2):273–81. doi: 10.1016/j.yhbeh.2010.03.006
65. de Goeij DC, Kvetnansky R, Whitnall MH, Jezova D, Berkenbosch F, Tilders FJ. Repeated stress-induced activation of corticotropin-releasing factor neurons enhances vasopressin stores and colocalization with corticotropin-releasing factor in the median eminence of rats. Neuroendocrinology (1991) 53(2):150–9. doi: 10.1159/000125712
66. Bartanusz V, Jezova D, Bertini LT, Tilders FJ, Aubry JM, Kiss JZ. Stress-induced increase in vasopressin and corticotropin-releasing factor expression in hypophysiotrophic paraventricular neurons. Endocrinology (1993) 132(2):895–902. doi: 10.1210/endo.132.2.8425502
67. Gillies GE, Linton EA, Lowry PJ. Corticotropin releasing activity of the new CRF is potentiated several times by vasopressin. Nature (1982) 299(5881):355–7. doi: 10.1038/299355a0
68. Rivier C, Vale W. Modulation of stress-induced ACTH release by corticotropin-releasing factor, catecholamines and vasopressin. Nature (1983) 305(5932):325–7. doi: 10.1038/305325a0
69. Scott LV, Dinan TG. Vasopressin and the regulation of hypothalamic-pituitary-adrenal axis function: implications for the pathophysiology of depression. Life Sci (1998) 62(22):1985–98. doi: 10.1016/S0024-3205(98)00027-7
70. Landgraf R, Wigger A, Holsboer F, Neumann I. Hyper-reactive hypothalamo-pituitary-adrenocortical axis in rats bred for high anxiety-related behaviour. J Neuroendocrinol (1999) 11(6):405–7. doi: 10.1046/j.1365-2826.1999.00342.x
71. Salomé N, Salchner P, Viltart O, Sequeira H, Wigger A, Landgraf R, et al. Neurobiological correlates of high (HAB) versus low anxiety-related behavior (LAB): differential Fos expression in HAB and LAB rats. Biol Psychiatry (2004) 55(7):715–23. doi: 10.1016/j.biopsych.2003.10.021
72. Neumann I, Wigger A, Krömer S, Frank E, Landgraf R, Bosch O. Differential effects of periodic maternal separation on adult stress coping in a rat model of extremes in trait anxiety. Neuroscience (2005) 132(3):867–77. doi: 10.1016/j.neuroscience.2005.01.034
73. Casali P, Schettino EW. Structure and function of natural antibodies. Curr Top Microbiol Immunol (1996) 210:167–79. doi: 10.1007/978-3-642-85226-8_17
74. Lacroix-Desmazes S, Kaveri SV, Mouthon L, Ayouba A, Malanchère E, Coutinho A, et al. Self-reactive antibodies (natural autoantibodies) in healthy individuals. J Immunol Methods (1998) 216(1–2):117–37. doi: 10.1016/S0022-1759(98)00074-X
75. Kay MM. Role of physiologic autoantibody in the removal of senescent human red cells. J Supramol Struct (1978) 9(4):555–67. doi: 10.1002/jss.400090409
76. Lacroix-Desmazes S, Mouthon L, Coutinho A, Kazatchkine MD. Analysis of the natural human IgG antibody repertoire: life-long stability of reactivities towards self antigens contrasts with age-dependent diversification of reactivities against bacterial antigens. Eur J Immunol (1995) 25(9):2598–604. doi: 10.1002/eji.1830250929
77. Coutinho A, Kazatchkine MD, Avrameas S. Natural autoantibodies. Curr Opin Immunol (1995) 7(6):812–8. doi: 10.1016/0952-7915(95)80053-0
78. Dicou E, Nerriere V. Evidence that natural autoantibodies against the nerve growth factor (NGF) may be potential carriers of NGF. J Neuroimmunol (1997) 75(1-2):200–3. doi: 10.1016/S0165-5728(97)00008-8
79. Bendtzen K, Hansen MB, Ross C, Svenson M. High-avidity autoantibodies to cytokines. Immunol Today (1998) 19(5):209–11. doi: 10.1016/S0167-5699(98)01252-3
80. Hamze Sinno M, Do Rego JC, Coëffier M, Bole-Feysot C, Ducrotte P, Gilbert D, et al. Regulation of feeding and anxiety by α-MSH reactive autoantibodies. Psychoneuroendocrinology (2009) 34(1):140–9. doi: 10.1016/j.psyneuen.2008.08.021
81. Bouhajja H, Bougacha-Elleuch N, Lucas N, Legrand R, Marrakchi R, Kaveri SV, et al. Affinity kinetics of leptin-reactive immunoglobulins are associated with plasma leptin and markers of obesity and diabetes. Nutr Diabetes (2018) 8(1):32. doi: 10.1038/s41387-018-0044-y
82. Takagi K, Legrand R, Asakawa A, Amitani H, François M, Tennoune N, et al. Anti-ghrelin immunoglobulins modulate ghrelin stability and its orexigenic effect in obese mice and humans. Nat Commun (2013) 4(2685):1–11. doi: 10.1038/ncomms3685
83. Schaefer JM, Fetissov SO, Legrand R, Claeyssens S, Hoekstra PJ, Verhulst FC, et al. Corticotropin (ACTH)-reactive immunoglobulins in adolescents in relation to antisocial behavior and stress-induced cortisol response. TRAILS Study Psychoneuroendocrinol (2013) 38(12):3039–47. doi: 10.1016/j.psyneuen.2013.08.015
84. Fetissov SO, Hamze Sinno M, Coëffier M, Bole-Feysot C, Ducrotté P, Hökfelt T, et al. Autoantibodies against appetite-regulating peptide hormones and neuropeptides: putative modulation by gut microflora. Nutrition (2008) 24(4):348–59. doi: 10.1016/j.nut.2007.12.006
85. Ebner K, Wotjak CT, Landgraf R, Engelmann M. Neuroendocrine and behavioral response to social confrontation: residents versus intruders, active versus passive coping styles. Horm Behav (2005) 47(1):14–21. doi: 10.1016/j.yhbeh.2004.08.002
86. Kruk MR, Halasz J, Meelis W, Haller J. Fast positive feedback between the adrenocortical stress response and a brain mechanism involved in aggressive behavior. Behav Neurosci (2004) 118(5):1062–70. doi: 10.1037/0735-7044.118.5.1062
87. Craig IW. The importance of stress and genetic variation in human aggression. Bioessays (2007) 29(3):227–36. doi: 10.1002/bies.20538
88. Karaiskos D, Mavragani CP, Sinno MH, Déchelotte P, Zintzaras E, Skopouli FN, et al. Psychopathological and personality features in primary Sjogren's syndrome-associations with autoantibodies to neuropeptides. Rheumatology (2010) 49(9):1762–9. doi: 10.1093/rheumatology/keq158
89. Fetissov SO, Harro J, Jaanisk M, Järv A, Podar I, Allik J, et al. Autoantibodies against neuropeptides are associated with psychological traits in eating disorders. Proc Natl Acad Sci USA (2005) 102(41):14865–70. doi: 10.1073/pnas.0507204102
90. Garcia FD, Coquerel Q, Kiive E, Déchelotte P, Harro J, Fetissov SO. Autoantibodies reacting with vasopressin and oxytocin in relation to cortisol secretion in mild and moderate depression. Prog Neuropsychopharmacol Biol Psychiatry (2011) 35(1):118–25. doi: 10.1016/j.pnpbp.2010.09.021
92. Lucas N, Legrand R, Bôle-Feysot C, Breton J, Coëffier M, Akkermann K, et al. Immunoglobulin G modulation of the melanocortin 4 receptor signaling in obesity and eating disorders. Trans Psychiatry (2019) 9(1):87. doi: 10.1038/s41398-019-0422-9
93. Fetissov SO, Hökfelt T. On the origin of eating disorders: altered signaling between gut microbiota, adaptive immunity and the brain melanocortin system regulating feeding behavior. Curr Opin Pharmacol (2019) 48:82–91. doi: 10.1016/j.coph.2019.07.004
94. Tennoune N, Legrand R, Ouelaa W, Breton J, Lucas N, Bole-Feysot C, et al. Sex-related effects of nutritional supplementation of Escherichia coli: relevance to eating disorders. Nutrition (2015) 31(3):498–507. doi: 10.1016/j.nut.2014.11.003
95. Kastin AJ, Pan W. Peptide transport across the blood-brain barrier. Prog Drug Res (2003) 61:79–100. doi: 10.1007/978-3-0348-8049-7_3
96. Pan W, Solomon B, Maness LM, Kastin AJ. Antibodies to beta-amyloid decrease the blood-to-brain transfer of beta-amyloid peptide. Exp Biol Med (Maywood) (2002) 227(8):609–15. doi: 10.1177/153537020222700808
Keywords: human aggression, adrenocorticotropic hormone, oxytocin, vasopressin, autoantibodies, hypothalamic-pituitary-adrenal axis, cortisol, epitopes
Citation: Vaeroy H, Schneider F and Fetissov SO (2019) Neurobiology of Aggressive Behavior—Role of Autoantibodies Reactive With Stress-Related Peptide Hormones. Front. Psychiatry 10:872. doi: 10.3389/fpsyt.2019.00872
Received: 22 January 2019; Accepted: 05 November 2019;
Published: 04 December 2019.
Edited by:
Noèlia Fernàndez-Castillo, Center for Biomedical Research in the Network of Rare Diseases (CIBERER), SpainReviewed by:
Dora Zelena, Institute of Experimental Medicine (MTA), HungaryRoyce J. Lee, University of Chicago, United States
Copyright © 2019 Vaeroy, Schneider and Fetissov. This is an open-access article distributed under the terms of the Creative Commons Attribution License (CC BY). The use, distribution or reproduction in other forums is permitted, provided the original author(s) and the copyright owner(s) are credited and that the original publication in this journal is cited, in accordance with accepted academic practice. No use, distribution or reproduction is permitted which does not comply with these terms.
*Correspondence: Henning Vaeroy, SGVubmluZy5WYWVyb3lAYWh1cy5ubw==; Sergueï O. Fetissov, U2VyZ3VlaS5GZXRpc3NvdkB1bml2LXJvdWVuLmZy