- 1Department of Neurorehabilitation Sciences, Casa Cura Policlinico, Milan, Italy
- 2Department of Psychology, University of Roehampton, London, United Kingdom
This opinion article highlights the importance of targeting critical brain regions during reconsolidation to gain insight into the brain mechanisms of memory dynamics and modulating existing memories.
Accumulating evidence has shown that reactivated existing memories become sensitive to modification during reconsolidation (Sandrini et al., 2015; Lee et al., 2017; see Figure 1). This post-reactivation state of plasticity is a topic of intense scientific investigation not only for the basic understanding of memory processes but also for the development of novel clinical interventions to modulate existing memories.
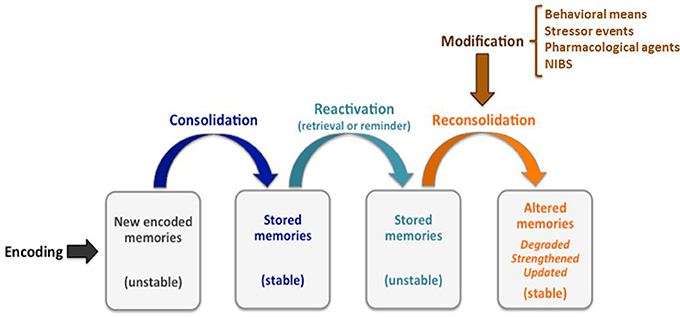
Figure 1. For a limited time after encoding, memories undergo an initial fragile/unstable phase, before being stabilized through the consolidation process. However, consolidated memories may return to a fragile phase when they are retrieved or reactivated by a reminder, thus requiring a restabilization process that is known as reconsolidation. During this time-limited reconsolidation window, existing memories can be degraded, strengthened, or updated by the inclusion of new information. Memories can be modified through behavioral means (e.g., interference, extinction), stressor events, pharmacological agents (e.g., propranolol, a beta blocker) or noninvasive brain stimulation (NIBS) techniques. Modified from Sandrini et al. (2015) with permission from Elsevier.
Noninvasive brain stimulation (NIBS) (Dayan et al., 2013) is a safe approach for studying brain mechanisms of memory reconsolidation. A seminal repetitive transcranial magnetic stimulation (rTMS) study showed that the primary motor cortex (M1) is essential for successful modification of motor memory strength (Censor et al., 2010). Subsequent rTMS work demonstrated that lateral prefrontal cortex (PFC) plays a causal role in strengthening episodic memory through reconsolidation (Sandrini et al., 2013). Similar effects on episodic memory have been documented with transcranial direct current stimulation (tDCS) applied to the PFC in young and older adults (Javadi and Cheng, 2013; Sandrini et al., 2014; Manenti et al., 2017). In addition, tDCS to the PFC after a reminder induced longer-lasting positive effects (up to 1 month) relative to tDCS during encoding (Manenti et al., 2016). Future NIBS work should investigate whether beneficial effects can be observed also in individuals with Mild Cognitive Impairment (MCI)—a population at risk of developing dementia such as Alzheimer's Disease (AD).
Regarding the possibility to disrupt “intrusive” maladaptive memories, a pilot study showed that the combination of brief exposure to a traumatic event with deep rTMS to the medial PFC induced beneficial effects in patients with post-traumatic stress disorder (Isserles et al., 2013). Another study showed that tDCS applied to the PFC after a reminder enhanced fear memories (Mungee et al., 2014). However, there is a lack of evidence that NIBS can disrupt maladaptive memories through reconsolidation. So far, only electroconvulsive therapy administered after memory reactivation in patients with unipolar depression has been shown to disrupt reactivated, but not non-reactivated, emotional episodic memories (Kroes et al., 2014).
Overall, it is important to keep in mind that the observed behavioral effects, up to now, are very transient and modest, despite encouraging. Replication studies from independent research groups are also needed.
Future research should also use rhythmic rTMS or transcranial alternating current stimulation to study the causal role of neural oscillations (e.g., in the beta frequency, Hanslmayr et al., 2014) for memory reconsolidation. In addition, the combination of NIBS with imaging offers the possibility to identify the causal systems-level mechanisms underlying memory reconsolidation (Censor et al., 2014a,b). For example, M1-rTMS interference with reactivated motor memory modulated M1-striatum intrinsic functional connectivity, which predicted offline memory modification (Censor et al., 2014b).
From a clinical perspective, an important issue that requires investigations is the interaction between NIBS during reconsolidation and pharmacological interventions. Several neurotransmitter systems (e.g., dopaminergic, serotonergic, cholinergic, and noradrenergic) have a role in modulating plasticity (Nitsche et al., 2012), and there is evidence that medications from very different pharmacological classes can enhance or hinder both NIBS-induced excitatory and inhibitory plasticity [i.e., long-term potentiation (LTP) and long-term depression (LTD)-like plasticity, respectively]. Effects of central nervous system (CNS) agents on NIBS-induced plasticity are complex and depend on different factors among which drug type and dosage, disease stage, NIBS type, and protocol. Lorazepam, a widely used benzodiazepine, delays, enhances, and prolongs LTP-like plasticity elicited by tDCS, but has no effect on tDCS-induced LTD-like plasticity (Nitsche et al., 2004a,b). The widely prescribed citalopram, a serotonin reuptake inhibitor antidepressant, enhances, and prolongs tDCS induced LTP-like plasticity, while reverting tDCS induced LTD-like plasticity to LTP-like plasticity (Nitsche et al., 2009). Among psychoactive substances, nicotine (Thirugnanasambandam et al., 2011) and alcohol (Conte et al., 2008) both cause complex modulation of NIBS-induced plasticity. It has been pointed out that studies on the clinical effectiveness of NIBS-induced plasticity in patients should take into account the concomitant use of multiple medications and the importance of reporting medication use in NIBS clinical trials (Huang et al., 2017; McLaren et al., 2018). In this regard, it is noteworthy that several non-CNS agents can actually modulate NIBS-induced plasticity. For example, antihypertensive drugs (e.g., beta blockers) reduce both tDCS LTP and LTD-like plasticity (Nitsche et al., 2004a). Alpha blockers, often used for treating urinary symptoms, abolish LTP-like plasticity induced by paired associative stimulation (PAS) (Korchounov and Ziemann, 2011). In real world context, patients that would benefit from NIBS-induced plasticity are often elderly people with comorbid conditions who are chronically taking several medications. As an example, sedatives, antidepressants, and antihypertensives are common medications for stroke patients—a condition in which NIBS-induced plasticity is often studied as a therapeutic tool. In view of the above, unchecked medications could cause unwanted, detrimental interactions with NIBS-induced plasticity. However, we want to stress that there is a strong rationale according to which NIBS and medications can actually work together for boosting therapeutic plasticity, eventually leading to a better clinical outcome (Perez et al., 2014). For example, the combination of tDCS and antidepressant drugs increases the efficacy of each single treatment in major depression (Brunoni et al., 2013) and tDCS associated with citalopram enhances declarative memory formation in young and older adults (Prehn et al., 2017).
Since NIBS techniques are promising interventions for treating declarative memory impairments that are often the first symptoms of dementia, the combination of NIBS with pharmacological interventions could prove to be an important symptomatic therapy for subjects with MCI due to AD (Albert et al., 2011), which today represents the population of interest for the use of the so-called Disease-Modifying Therapies (DMTs).
Finally, future research should address the issue of inter-individual variability in the effects of NIBS during reconsolidation. In particular, the possibility that variations in the presence of specific genetic polymorphisms (e.g., brain-derived neurotrophic factor gene; Chaieb et al., 2014) or brain structure (i.e., gray and white matter integrity; Censor et al., 2016) may influence the individual responsiveness are still open questions.
In conclusion, a better understanding of these basic and clinical issues will be instrumental to the development of NIBS clinical interventions for improving memory and symptoms through reconsolidation.
Author Contributions
All authors listed have made a substantial, direct and intellectual contribution to the work, and approved it for publication.
Funding
MS has received funding by the European Union's Horizon 2020 research and innovation programme under the Marie Skłodowska-Curie grant agreement No. 658496.
Conflict of Interest Statement
The authors declare that the research was conducted in the absence of any commercial or financial relationships that could be construed as a potential conflict of interest.
References
Albert, M. S., DeKosky, S. T., Dickson, D., Dubois, B., Feldman, H. H., Fox, N. C., et al. (2011). The diagnosis of mild cognitive impairment due to Alzheimer's disease: recommendations from the National Institute on Aging-Alzheimer's Association workgroups on diagnostic guidelines for Alzheimer's disease. Alzheimers Dement. 7, 270–279. doi: 10.1016/j.jalz.2011.03.008
Brunoni, A. R., Valiengo, L., Baccaro, A., Zanão, T. A., de Oliveira, J. F., Goulart, A., et al. (2013). The sertraline vs. electrical current therapy for treating depression clinical study: results from a factorial, randomized, controlled trial. JAMA Psychiatry 70, 383–391. doi: 10.1001/2013.jamapsychiatry.32
Censor, N., Buch, E. R., Nader, K., and Cohen, L. G. (2016). Altered human memory modification in the presence of normal consolidation. Cereb. Cortex 26, 3828–3837. doi: 10.1093/cercor/bhv180
Censor, N., Dayan, E., and Cohen, L. G. (2014a). Cortico-subcortical neuronal circuitry associated with reconsolidation of human procedural memories. Cortex 58, 281–288. doi: 10.1016/j.cortex.2013.05.013
Censor, N., Dimyan, M. A., and Cohen, L. G. (2010). Modification of existing human motor memories is enabled by primary cortical processing during memory reactivation. Curr. Biol. 20, 1545–1549. doi: 10.1016/j.cub.2010.07.047
Censor, N., Horovitz, S. G., and Cohen, L. G. (2014b). Interference with existing memories alters offline intrinsic functional brain connectivity. Neuron 81, 69–76. doi: 10.1016/j.neuron.2013.10.042
Chaieb, L., Antal, A., Ambrus, G. G., and Paulus, W. (2014). Brain-derived neurotrophic factor: its impact upon neuroplasticity and neuroplasticity inducing transcranial brain stimulation protocols. Neurogenetics 15, 1–11. doi: 10.1007/s10048-014-0393-1
Conte, A., Attilia, M. L., Gilio, F., Iacovelli, E., Frasca, V., Bettolo, C. M., et al. (2008). Acute andchronic effects of ethanol on cortical excitability. Clin. Neurophysiol. 119, 667–674. doi: 10.1016/j.clinph.2007.10.021
Dayan, E., Censor, N., Buch, E. R., Sandrini, M., and Cohen, L. G. (2013). Noninvasive brain stimulation: from physiology to network dynamics and back. Nat. Neurosci. 16, 838–844. doi: 10.1038/nn.3422
Hanslmayr, S., Matuschek, J., and Fellner, M. C. (2014). Entrainment of prefrontal beta oscillations induces an endogenous echo and impairs memory formation. Curr. Biol. 24, 904–909. doi: 10.1016/j.cub.2014.03.007
Huang, Y. Z., Lu, M. K., Antal, A., Classen, J., Nitsche, M., Ziemann, U., et al. (2017). Plasticity induced by non-invasive transcranial brain stimulation: a position paper. Clin. Neurophysiol. 128, 2318–2329. doi: 10.1016/j.clinph.2017.09.007
Isserles, M., Shalev, A. Y., Roth, Y., Peri, T., Kutz, I., Zlotnick, E., et al. (2013). Effectiveness of deep transcranial magnetic stimulation combined with a brief exposure procedure in post-traumatic stress disorder–a pilot study. Brain Stimul. 6, 377–383. doi: 10.1016/j.brs.2012.07.008
Javadi, A. H., and Cheng, P. (2013). Transcranial direct current stimulation (tDCS) enhances reconsolidation of long-term memory. Brain Stimul. 6, 668–674. doi: 10.1016/j.brs.2012.10.007
Korchounov, A., and Ziemann, U. (2011). Neuromodulatory neurotransmitters influence LTP-like plasticity in human cortex: a pharmaco-TMS study. Neuropsychopharmacology 36, 1894–1902. doi: 10.1038/npp.2011.75
Kroes, M. C., Tendolkar, I., van Wingen, G. A., van Waarde, J. A., Strange, B. A., and Fernández, G. (2014). An electroconvulsive therapy procedure impairs reconsolidation of episodic memories in humans. Nat. Neurosci. 17, 204–206. doi: 10.1038/nn.3609
Lee, J. L. C., Nader, K., and Schiller, D. (2017). An update on memory reconsolidation updating. Trends Cogn. Sci. 21, 531–545. doi: 10.1016/j.tics.2017.04.006
Manenti, R., Sandrini, M., Brambilla, M., and Cotelli, M. (2016). The optimal timing of stimulation to induce long-lasting positive effects on episodic memory in physiological aging. Behav. Brain Res. 311, 81–86. doi: 10.1016/j.bbr.2016.05.028
Manenti, R., Sandrini, M., Gobbi, E., Cobelli, C., Brambilla, M., Binetti, G., et al. (2017). Strengthening of existing episodic memories through non-invasive stimulation of prefrontal cortex in older adults with subjective memory complaints. Front. Aging Neurosci. 9:401. doi: 10.3389/fnagi.2017.00401
McLaren, M. E., Nissim, N. R., and Woods, A. J. (2018). The effects of medication use in transcranial direct current stimulation: a brief review. Brain Stimul. 11, 52–58. doi: 10.1016/j.brs.2017.10.006
Mungee, A., Kazzer, P., Feeser, M., Nitsche, M. A., Schiller, D., and Bajbouj, M. (2014). Transcranial direct current stimulation of the prefrontal cortex: a means to modulate fear memories. Neuroreport 25, 480–484. doi: 10.1097/WNR.0000000000000119
Nitsche, M. A., Grundey, J., Liebetanz, D., Lang, N., Tergau, F., and Paulus, W. (2004a). Catecholaminergic consolidation of motor cortical neuroplasticity in humans. Cereb. Cortex 14, 1240–1245. doi: 10.1093/cercor/bhh085
Nitsche, M. A., Kuo, M. F., Karrasch, R., Wächter, B., Liebetanz, D., and Paulus, W. (2009). Serotonin affects transcranial direct current-induced neuroplasticity in humans. Biol. Psychiatry 66, 503–508. doi: 10.1016/j.biopsych.2009.03.022
Nitsche, M. A., Liebetanz, D., Schlitterlau, A., Henschke, U., Fricke, K., Frommann, K., et al. (2004b). GABAergic modulation of DC stimulation-induced motor cortex excitability shifts in humans. Eur. J. Neurosci. 19, 2720–2726. doi: 10.1111/j.0953-816X.2004.03398.x
Nitsche, M. A., Müller-Dahlhaus, F., Paulus, W., and Ziemann, U. (2012). The pharmacology of neuroplasticity induced by non-invasive brain stimulation: building models for the clinical use of CNS active drugs. J. Physiol. 590, 4641–4662. doi: 10.1113/jphysiol.2012.232975
Perez, C., Morales-Quezada, L., and Fregni, F. (2014). A combined therapeutic approach in stroke rehabilitation: a review on non-invasive brain stimulation plus pharmacotherapy. Int. J. Neurorehabil. 1, 123. doi: 10.4172/2376-0281.1000123
Prehn, K., Stengl, H., Grittner, U., Kosiolek, R., Ölschläger, A., Weidemann, A., et al. (2017). Effects of anodal transcranial direct current stimulation and serotonergic enhancement on memory performance in young and older adults. Neuropsychopharmacology 42, 551–561. doi: 10.1038/npp.2016.170
Sandrini, M., Brambilla, M., Manenti, R., Rosini, S., Cohen, L. G., and Cotelli, M. (2014). Noninvasive stimulation of prefrontal cortex strengthens existing episodic memories and reduces forgetting in the elderly. Front. Aging Neurosci. 6:289. doi: 10.3389/fnagi.2014.00289
Sandrini, M., Censor, N., Mishoe, J., and Cohen, L. G. (2013). Causal role of prefrontal cortex in strengthening of episodic memories through reconsolidation. Curr. Biol. 23, 2181–2184. doi: 10.1016/j.cub.2013.08.045
Sandrini, M., Cohen, L. G., and Censor, N. (2015). Modulating reconsolidation: a link to causal systems-level dynamics of human memories. Trends Cogn. Sci. 19, 475–482. doi: 10.1016/j.tics.2015.06.002
Keywords: reconsolidation, reactivation, retrieval, memory, tDCS, TMS, brain stimulation, patients
Citation: Sandrini M, Caronni A and Corbo M (2018) Modulating Reconsolidation With Non-invasive Brain Stimulation—Where We Stand and Future Directions. Front. Psychol. 9:1430. doi: 10.3389/fpsyg.2018.01430
Received: 12 April 2018; Accepted: 23 July 2018;
Published: 13 August 2018.
Edited by:
Alexander T. Sack, Maastricht University, NetherlandsReviewed by:
Simone Rossi, University of Siena, ItalyCopyright © 2018 Sandrini, Caronni and Corbo. This is an open-access article distributed under the terms of the Creative Commons Attribution License (CC BY). The use, distribution or reproduction in other forums is permitted, provided the original author(s) and the copyright owner(s) are credited and that the original publication in this journal is cited, in accordance with accepted academic practice. No use, distribution or reproduction is permitted which does not comply with these terms.
*Correspondence: Marco Sandrini, bWFyY28uc2FuZHJpbmlAcm9laGFtcHRvbi5hYy51aw==