- 1Pharmaceutical Sciences, School of Pharmacy, Center for Basic and Translational Stroke Research, West Virginia University, Morgantown, WV, United States
- 2Division of Intramural Research, NIEHS/NIH, Durham, NC, United States
Previously, we showed vascular endothelial overexpression of human-CYP2J2 enhances coronary reactive hyperemia in Tie2-CYP2J2 Tr mice, and eNOS−/− mice had overexpression of CYP2J-epoxygenase with adenosine A2A receptor-induced enhance relaxation, but we did not see the response in CYP2J-epoxygenase knockout mice. Therefore, we hypothesized that Cyp2j5-gene deletion affects acetylcholine- and 5'-N-ethylcarboxamidoadenosine (NECA) (adenosine)-induced relaxation and their response is partially inhibited by angiotensin-II (Ang-II) in mice. Acetylcholine (Ach)-induced response was tested with N-(methylsulfonyl)-2-(2-propynyloxy)-benzenehexanamide (MS-PPOH, CYP-epoxygenase inhibitor; 10−5M) and Ang-II (10−6M). In Cyp2j5−/− mice, ACh-induced relaxation was different from C57Bl/6 mice, at 10−5 M (76.1 ± 3.3 vs. 58.3 ± 5.2, P < 0.05). However, ACh-induced relaxation was not blocked by MS-PPOH in Cyp2j5−/−: 58.5 ± 5.0%, P > 0.05, but blocked in C57Bl/6: 52.3 ± 7.5%, P < 0.05, and Ang-II reduces ACh-induced relaxation in both Cyp2j5−/− and C57Bl/6 mice (38.8 ± 3.9% and 45.9 ± 7.8, P <0.05). In addition, NECA-induced response was tested with Ang-II. In Cyp2j5−/− mice, NECA-induced response was not different from C57Bl/6 mice at 10−5M (23.1 ± 2.1 vs. 21.1 ± 3.8, P > 0.05). However, NECA-induced response was reduced by Ang-II in both Cyp2j5−/− and C57Bl/6 mice (−10.8 ± 2.3% and 3.2 ± 2.7, P < 0.05). Data suggest that ACh-induced relaxation in Cyp2j5−/− mice depends on nitric oxide (NO) but not CYP-epoxygenases, and the NECA-induced different response in male vs. female Cyp2j5−/− mice when Ang-II treated.
Introduction
Arachidonic acid (AA) can be metabolized into epoxyeicosatrienoic acids (EETs) through cytochrome P450 (CYP)-epoxygenases, like CYP2Cs and CYP2Js, and the cytochrome P450 (CYP) ω-hydroxylases (CYP4A, CYP4B, CYP4F, CYP4V, CYP4X, CYP4Z, etc.) hydroxylate AA to HETEs (hydroxyeicosatetraenoic acids), including 20-HETE which is a potent vasoconstrictor (Hoopes et al., 2015). CYP2C and CYP2J-epoxygenases generate four distinct EET regioisomers: 5,6-, 8,9-, 11,12-, and 14,15-EET, and these EETs are involved in numerous biological functions, such as hyperpolarization and relaxation in vascular smooth muscle cells (Campbell et al., 1996; Ellinsworth et al., 2014). Further, several members of CYP2C (Cyp2c29) and CYP2J (CYP2J2, Cyp2j5) subfamilies have been detected on the vascular endothelium (Fisslthaler et al., 1999; Node et al., 1999; Zeldin and Liao, 2000; Zeldin et al., 2001; Nayeem et al., 2008; Nayeem et al., 2009; Nayeem et al., 2011; Ponnoth et al., 2012a; Pradhan et al., 2014; Hanif et al., 2017b). In addition, Yang et al., and Hanif et al., reported that an overexpression of CYP2J2 protects vascular endothelium against hypoxia-reoxygenation injury/ischemia/reperfusion injury with enhanced coronary reactive hyperemic (CRH) response in isolated mouse heart model (Yang et al., 2001; Hanif et al., 2017b), and the vascular endothelial CYP2Cs and CYP2Js are the main source of EETs (5,6-, 8,9-, 11,12-, and 14,15-EET regioisomers) generation (Rosolowsky and Campbell, 1996; Fisslthaler et al., 1999; Node et al., 1999). EETs have shown to be more effective on small-resistance vessels, as well as in coronary arteries and aorta (Fang et al., 2001; Nayeem et al., 2008; Nayeem et al., 2013; Hanif et al., 2016a; Hanif et al., 2016b; Hanif et al., 2017b). EETs have been observed to produce relaxation in isolated coronary arteries at concentrations as low as 10 pM and are involved in increased CRH response (Fang et al., 2001; Hanif et al., 2016a; Hanif et al., 2016b; Hanif et al., 2017b). In addition, the EET-induced relaxation in bovine coronary arteries and mouse aorta is inhibited by the EET antagonist, 14,15-epoxyeicosa-5(Z)-enoic acid (Gauthier et al., 2002; Nayeem et al., 2009; Nayeem et al., 2013; Pradhan et al., 2014).
EETs dilate blood vessels and also have natriuretic effects (Oltman et al., 1998; Imig et al., 2001; McGiff and Quilley, 2001), and the renal CYP-epoxygenases (CYP2C and CYP2J subfamilies) are believed to be under the regulatory control of dietary salt (Makita et al., 1994). Therefore, spontaneously hypertensive rats (SHR) have shown altered protein expression levels and activities for both renal CYP-epoxygenase and ω-hydroxylase (Schwartzman et al., 1996; Yu et al., 2000). Athirakul et al. reported that Cyp2j5 gene disruption resulted in increased blood pressure in female mice compared to their wild-type counterparts (Athirakul et al., 2008), and the elevated blood pressure was associated with increased left ventricular mass and enhanced renal (afferent arterioles) vasoconstriction due to angiotensin II (Athirakul et al., 2008). Further, an increase in renal vascular reactivity to angiotensin II has been reported in the early stage of hypertension in both rodents and humans (Simon et al., 1995; Imig, 2000). Cyp2j5 enzyme is abundant in kidney as well as in the mouse aorta where it is very active in arachidonic acid metabolism to generate EETs (Ma et al., 2004; Nayeem et al., 2008; Nayeem et al., 2013). Mouse kidneys demonstrated higher Cyp2j5 expression in male compared to female mice after puberty, and Northern analysis also revealed that Cyp2j5 transcripts were more abundant in adult male versus adult female kidneys (Ma et al., 2004). Burgess et al. showed that Cyp2j5 is responsible for production of primarily 14,15- and 11,12-EETs in visceral adipose tissue (Burgess et al., 2012), and overexpression of Cyp2j5 and Cyp4a proteins were observed in Ephx2−/− vs. C57Bl/6 mice with enhanced adenosine (NECA) and CGS 21680 (A2A AR)-induced aortic relaxation (Nayeem et al., 2013). An overexpression of Cyp2j5 and Cyp4a proteins in Ephx2−/− vs. C57Bl/6 mice may be involved as a compensatory mechanism to maintain vascular tone (Nayeem et al., 2013).
The conversion of arachidonic acid into epoxides takes place in the presence of CYP-epoxygenases, which have beneficial cardiovascular properties. Inhibition or deletion of CYP-epoxygenases including Cyp2j5 may cause less EET-generation from arachidonic acid, leading to decreased vascular relaxation. Likewise, knocking out the Cyp4a10 gene caused salt-sensitive hypertension with reduced EET excretion in the urine (Nakagawa et al., 2006). Some studies revealed that single nucleotide polymorphisms (SNPs) in the CYP4A11 and CYP2J2 genes were associated with human hypertension (Gainer et al., 2005; King et al., 2005). In addition, adenosine A2A receptor (A2A AR) knockout mice had lower expression of CYP-epoxygenases and higher expression of ω-hydroxylases with enhanced NECA (adenosine agonist) and CGS 21680 (A2A AR-agonist)-induced vasoconstriction (aortic) compared to their respective wild-type mice (Nayeem et al., 2008; Pradhan et al., 2014; Pradhan et al., 2015). Further, Hanif et al. recently reported that A2A AR−/− mice had less plasma EETs compared to wild-type counterparts with reduced coronary reactive hyperemic response (Hanif et al., 2017a). An increase in blood pressure has been reported with enhanced renal vasoconstriction with angiotensin II in female Cyp2j5−/− compared to female wild-type mice (Athirakul et al., 2008). To date, there are no studies addressing the vascular (aortic) response using acetylcholine and adenosine in male and female Cyp2j5−/− vs. C57Bl/6 mice, though Cyp2j5 enzyme is abundant in the kidneys as well as in mouse aorta where EETs get generated (Ma et al., 2004; Nayeem et al., 2008; Nayeem et al., 2013). Also, the effects of angiotensin-II in their vascular response in both Cyp2j5−/− and wild type mice has not been investigated. Therefore, we hypothesized that Cyp2j5-gene deletion affects acetylcholine- and NECA-induced relaxation and their response is partially inhibited by Ang-II in mice.
Materials and Methods
Animals
Athirakul et al. (2008) described the generation of Cyp2j5−/− mice. Cyp2j5−/− and their respective C57Bl/6 mice were provided by Dr. Zeldin, National Institute of Environmental Health Sciences/National Institutes of Health (NIH). We used both male and female mice (14–18 week old) equally in our study.
Materials
Phenylephrine, acetylcholine (ACh) (Sigma Chemicals, St. Louis, MO), and angiotensin II (Human), BACHEM (Bubendorf, Switzerland) dissolved in distilled water. N-(Methylsulfonyl)-2-(2-propynyloxy)-benzenehexanamide (MS-PPOH), dibromo-dodecenyl-methylsulfimide (DDMS) (Cayman Chemicals), 5'-N-ethylcarboxamidoadenosine (NECA), and CGS 21680 (Sigma Chemicals, St. Louis, MO) were dissolved in dimethyl sulfoxide (DMSO) (Nayeem et al., 2013).
Muscle Bath Experiments
Cyp2j5−/− and C57Bl/6 mice were euthanized with pentobarbital sodium (100 mg/kg intraperitoneally). The aortas from Cyp2j5−/− and C57Bl/6 mice were removed after thoracotomy, the removed and cleaned aortas cut into four rings of 3–4 mm in length. The rings were hung between two wire hooks. The rings were suspended in organ baths of the tissue bath containing 10 ml of modified Krebs-Henseleit buffer in each bath. After the equilibration period (~60 min), aortic rings were contracted with KCl (50 mM) to assess the viability of the ring. Rings were then contracted with phenylephrine (PE; 10−7 M), and the ring tension was monitored continuously with a fixed range precision force transducer (125C; BIOPAC Systems) connected to amplifiers (Data Acquisition System 100B; BIOPAC Systems). Data were recorded using MP100 WSW, BIOPAC digital acquisition system and analyzed using Acknowledge 3.5.7 software (BIOPAC Systems). ACh (10−12–10−5 M) and NECA (adenosine, 10−9–10−5 M)-concentration dependent response experiments were conducted, as previously described with NECA, adenosine A1 receptor agonist (CCPA), and adenosine A2A receptor agonist (CGS 21680) (Nayeem et al., 2008; Nayeem et al., 2009; Nayeem et al., 2010; Nayeem et al., 2011; Ponnoth et al., 2012a; Ponnoth et al., 2012b; Nayeem et al., 2013; Pradhan et al., 2014; Pradhan et al., 2015). The aortic rings were washed several times with Krebs-Henseleit solution and allowed to equilibrate for 30 min before the experimental protocol began. Results expressed as percentages (%) downward or upward on PE-induced precontracted rings.
Effects of N-(methylsulfonyl)-2-(2-propynyloxy)-benzenehexanamide (MS-PPOH) (CYP-epoxygenase inhibitor on ACh-induced colorectal carcinoma (CRC) in Cyp2j5−/− and C57Bl/6 mice. ACh-concentration dependent response curves (DDRCs) were obtained by cumulative addition of drugs in 1-log increments as described by us (Nayeem et al., 2008; Nayeem et al.,2009; Nayeem et al., 2010; Nayeem et al., 2011; Ponnoth et al., 2012a; Ponnoth et al., 2012b; Nayeem et al., 2013; Pradhan et al., 2014; Pradhan et al., 2015). A single DDRC was constructed for each ring in parallel in pairs of rings from either Cyp2j5−/− and C57Bl/6 mice in the same organ bath. ACh-DDRC was obtained with and without MS-PPOH (10−5 M), and MS-PPOH was added 30 min before the phenylephrine (PE) contraction and was present throughout the ACh DDRC. Similar as described earlier by us (Hanif et al., 2016b; Hanif et al., 2017b).
Effects of angiotensin-II (Ang-II, 1 µM) on ACh-induced DDRC in Cyp2j5−/− and C57Bl/6 mice. ACh-DDRC was obtained with and without Ang-II (1 µM), and Ang-II was added 30 min before the phenylephrine (PE) contraction and was present throughout the ACh-DDRC as described earlier by us (Ponnoth et al., 2012a; Yadav et al., 2015).
Effects of 20-HETE synthesis inhibitor, dibromo-dodecenyl-methylsulfimide (DDMS, 10−5 M) on adenosine (NECA) or CGS 21680-induced DDRC in Cyp2j5−/− and C57Bl/6 mice. NECA or CGS 21680-DDRC was obtained by cumulative addition of drugs in 1-log increments as described by us (Nayeem et al., 2008; Nayeem et al., 2009; Nayeem et al., 2010; Nayeem et al., 2011; Ponnoth et al., 2012a; Ponnoth et al., 2012b; Nayeem et al., 2013; Pradhan et al., 2014; Pradhan et al., 2015). A single DDRC was constructed for each ring in parallel in pairs of rings from either Cyp2j5−/− and C57Bl/6 mice in the same organ bath. NECA or CGS 21680-DDRC was obtained with and without DDMS (10−5 M), and DDMS was added 30 min before the PE contraction and was present throughout the NECA or CGS 21680-DDRC.
Effects of angiotensin-II (Ang-II, 10−6 M) on top of DDMS in NECA or CGS 21680-induced DDRC in Cyp2j5−/− and C57Bl/6 mice. NECA or CGS 21680-induced DDRC was obtained with and without Ang-II (10−6 M) and DDMS (10−5 M), and Ang-II ± DDMS was added 30 min before the PE contraction and was present throughout the NECA or CGS 21680-induced DDRC. Similar as described earlier by us (Nayeem et al., 2009; Nayeem et al., 2010; Nayeem et al., 2011; Ponnoth et al., 2012a; Yadav et al., 2015).
Statistical Analysis
The data reported as means ± SE. One-way ANOVA was used to compare difference among groups, and two-way ANOVA was used for repeated measures, followed by Tukey post hoc test to compare the ACh/NECA/CGS 21680-induced vascular responses of non-treated vs. inhibitor/antagonist (MS-PPOH, DDMS, and Ang-II)-treated Cyp2j5−/− and C57Bl/6 mice. Differences (Cyp2j5−/− vs. C57Bl/6), non-treated vs. (MS-PPOH, DDMS, and Ang-II)-treated Cyp2j5−/− and C57Bl/6 mice were considered significant when P < 0.05. All the statistical analyses performed using GraphPad Prism statistical package.
Results
Concentration dependent response curve (DDRC) for ACh-induced relaxation in Cyp2j5−/− and C57Bl/6 mice: ACh caused a concentration (10−7–10−5 M)-dependent relaxation in both C57Bl/6 and Cyp2j5−/− mice. The response was significantly different (ACh-10−7–10−6 M, P < 0.05) between C57Bl/6 vs. Cyp2j5−/− mice (Figure 1).
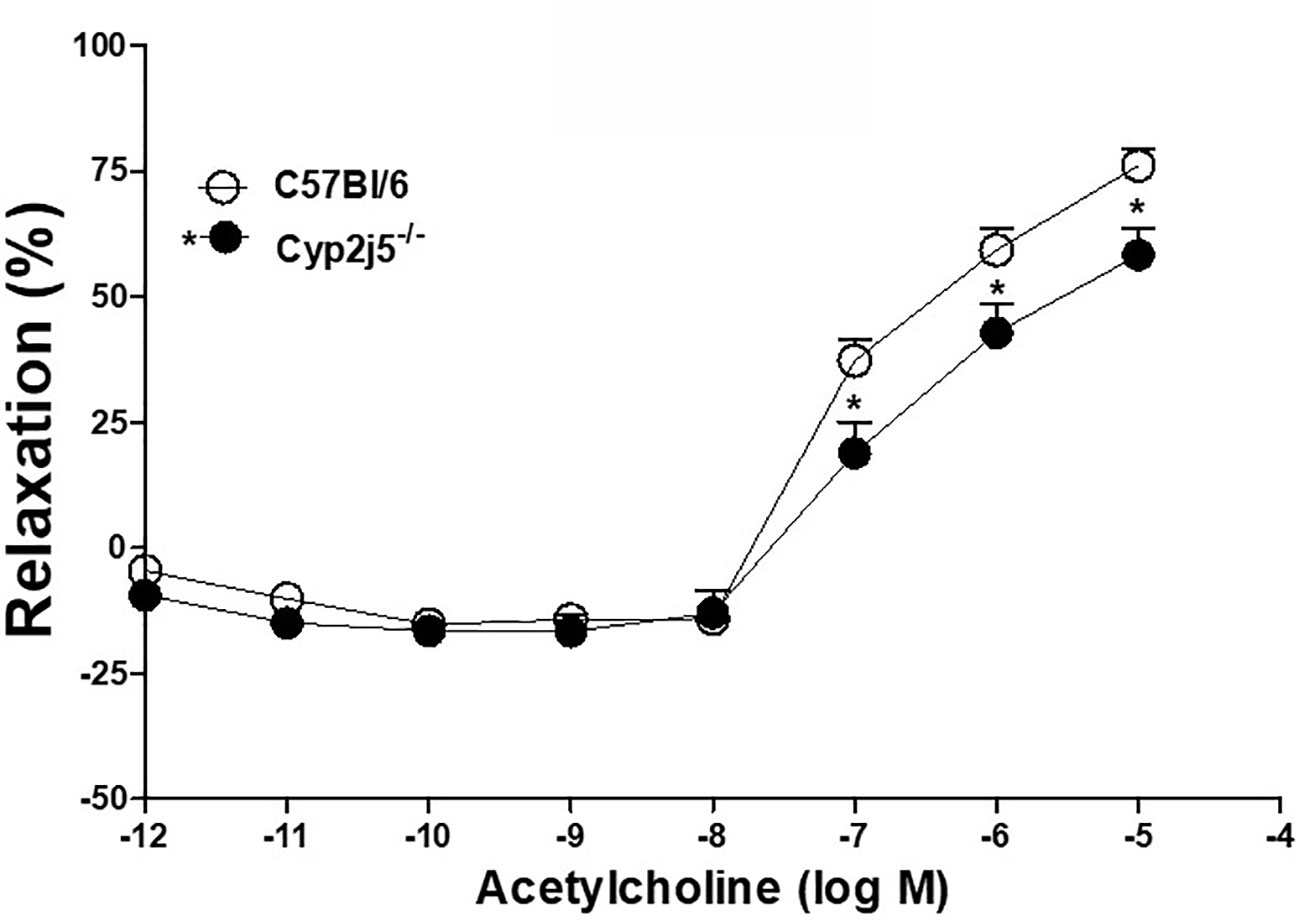
Figure 1 Acetylcholine (ACh)-induced concentration dependent vascular response in aortic rings of C57Bl/6 and Cyp2j5−/− mice. *P < 0.05 between C57Bl/6 vs. Cyp2j5−/− mice, there is a significant difference found in (ACh)-induced concentration (10−7M–10−5M) dependent vascular response between C57Bl/6 and Cyp2j5−/− mice (*P < 0.05). Values expressed as means ± SE. n = eight per group.
DDRC for ACh with or without MS-PPOH (CYP-epoxygenases inhibitor, 10−5 M) treated C57Bl/6 and Cyp2j5−/− mice: at 10−5 M ACh, MS-PPOH reduced the ACh-induced concentration-dependent relaxation in C57Bl/6 (Figure 2). There was no change in treated Cyp2j5−/− mice vs. non-treated (P > 0.05, Figure 2). However, there was a significant change between C57Bl/6 vs. Cyp2j5−/− mice (at 10−5 M ACh, 76.1 ± 3.3 vs. 58.3 ± 5.2, P < 0.05, Figure 2).
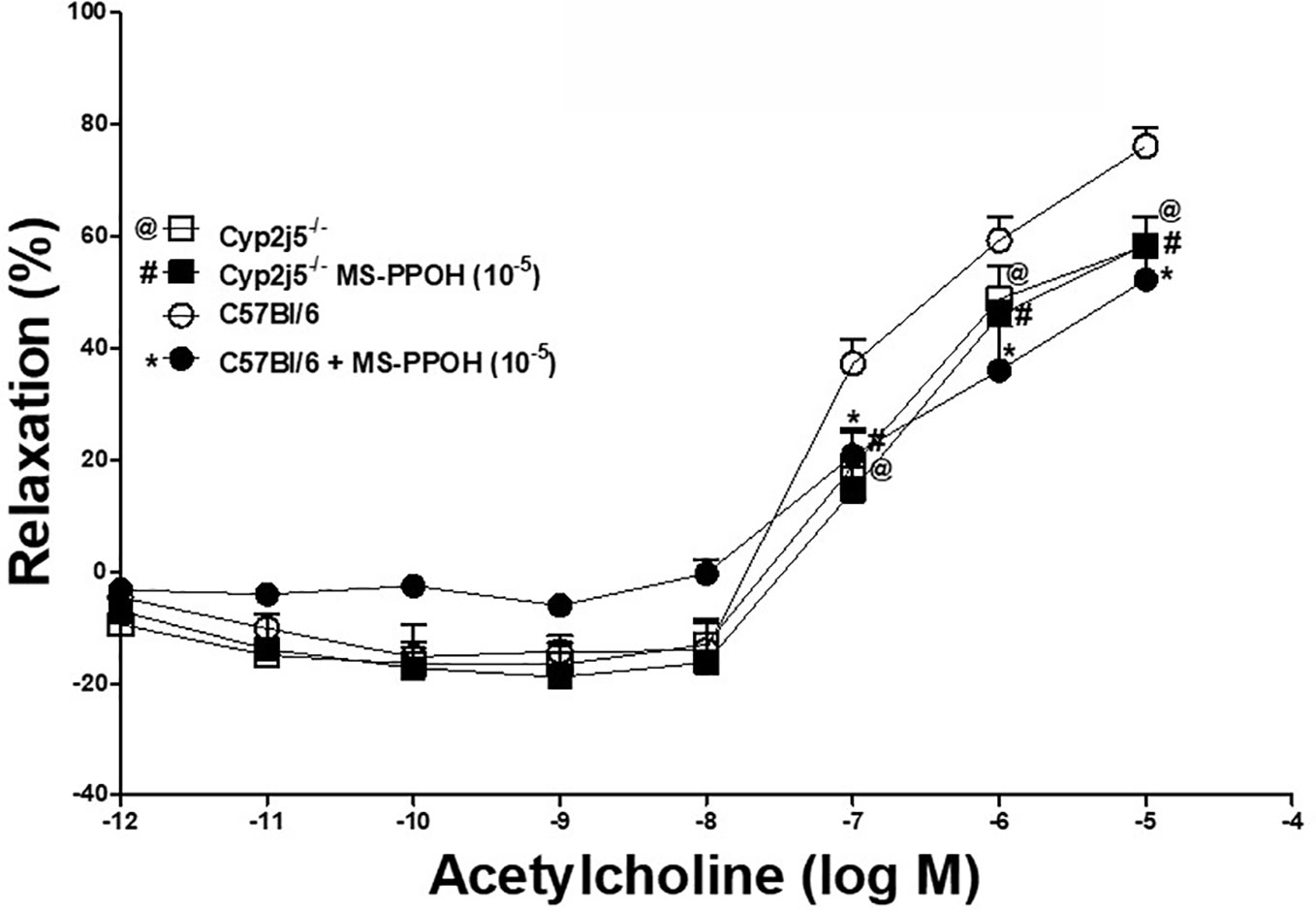
Figure 2 Effect of MS-PPOH (10−5 M) on ACh-induced concentration dependent vascular response in aortic rings of C57Bl/6 and Cyp2j5−/− mice. *P < 0.05 between non-treated C57Bl/6 vs. MS-PPOH treated C57Bl/6 mice, @P < 0.05 between non-treated C57Bl/6 vs. non-treated Cyp2j5−/− mice, #P < 0.05 between non-treated C57Bl/6 vs. MS-PPOH-treated Cyp2j5−/− mice. #@P > 0.05 between non-treated Cyp2j5−/− and MS-PPOH treated Cyp2j5−/− mice and between MS-PPOH treated Cyp2j5−/− vs. MS-PPOH treated C57Bl/6 mice (#*P > 0.05). Values expressed as means ± SE. n = eight per group.
DDRC for ACh and the effects of Ang-II (10−6 M) in Cyp2j5−/− and C57Bl/6 mice: ACh produced a concentration-dependent relaxation in both Cyp2j5−/− and C57Bl/6 mice. Ang-II (10−6 M) attenuated ACh-induced concentration-dependent relaxation in both C57Bl/6 and Cyp2j5-/-treated vs. non-treated (P < 0.05, Figure 3) mice. Interestingly, Ang-II treatment attenuated more ACh-induced concentration-dependent relaxation in Cyp2j5−/− vs. Ang-II treated C57Bl/6 (P < 0.05, Figure 3). In addition, the effect of Ang-II was significantly different at the low concentrations of ACh 10−9–10−8 M in Cyp2j5−/− vs. non-treated Cyp2j5−/− mice (P < 0.05, Figure 3).
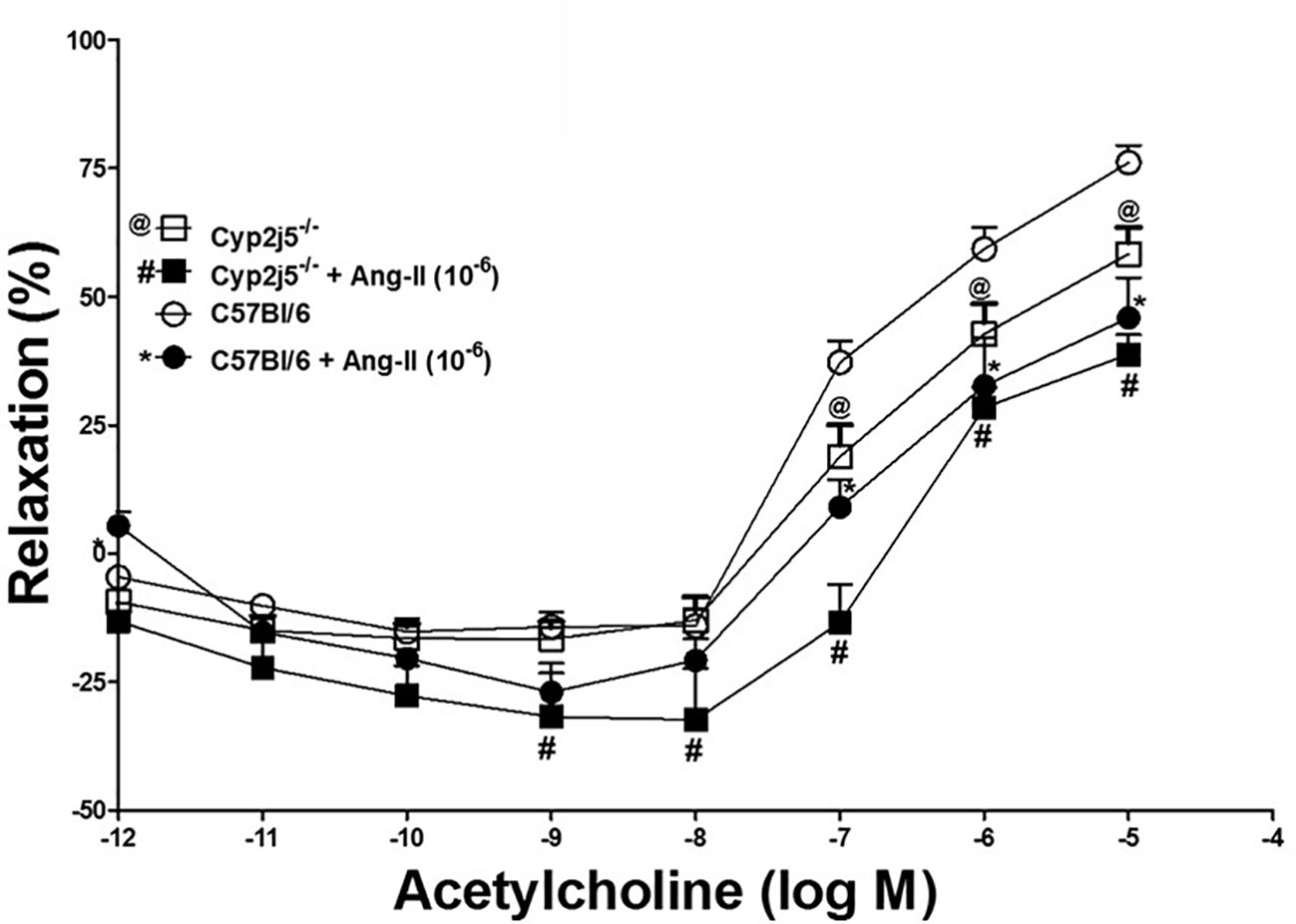
Figure 3 Effect of angiotensin II (Ang-II) (10−6 M) on acetylcholine (Ach)-induced concentration dependent vascular response in aortic rings of C57Bl/6 and Cyp2j5−/− mice. *P < 0.05 between non-treated C57Bl/6 vs. Ang-II treated C57Bl/6 mice, @P < 0.05 between non-treated C57Bl/6 vs. non-treated Cyp2j5−/− mice, #P < 0.05 between non-treated C57Bl/6 vs. Ang-II-treated Cyp2j5−/− mice. #@P < 0.05 between non-treated Cyp2j5−/− and Ang-II treated Cyp2j5−/− mice and between Ang-II treated Cyp2j5−/− vs. Ang-II treated C57Bl/6 mice (#*P > 0.05). Values expressed as means ± SE. n = eight per group.
DDRC for adenosine (NECA)-induced relaxation in Cyp2j5−/− and C57Bl/6 mice: NECA caused a concentration (10−7–10−5 M)-dependent relaxation, but the response was not different between male and female C57Bl/6 mice (NECA-10−7–10−6 M, Figure 4A, P > 0.05) or Cyp2j5−/− mice (NECA-10−7–10−6 M, Figures 4B–D, P > 0.05).
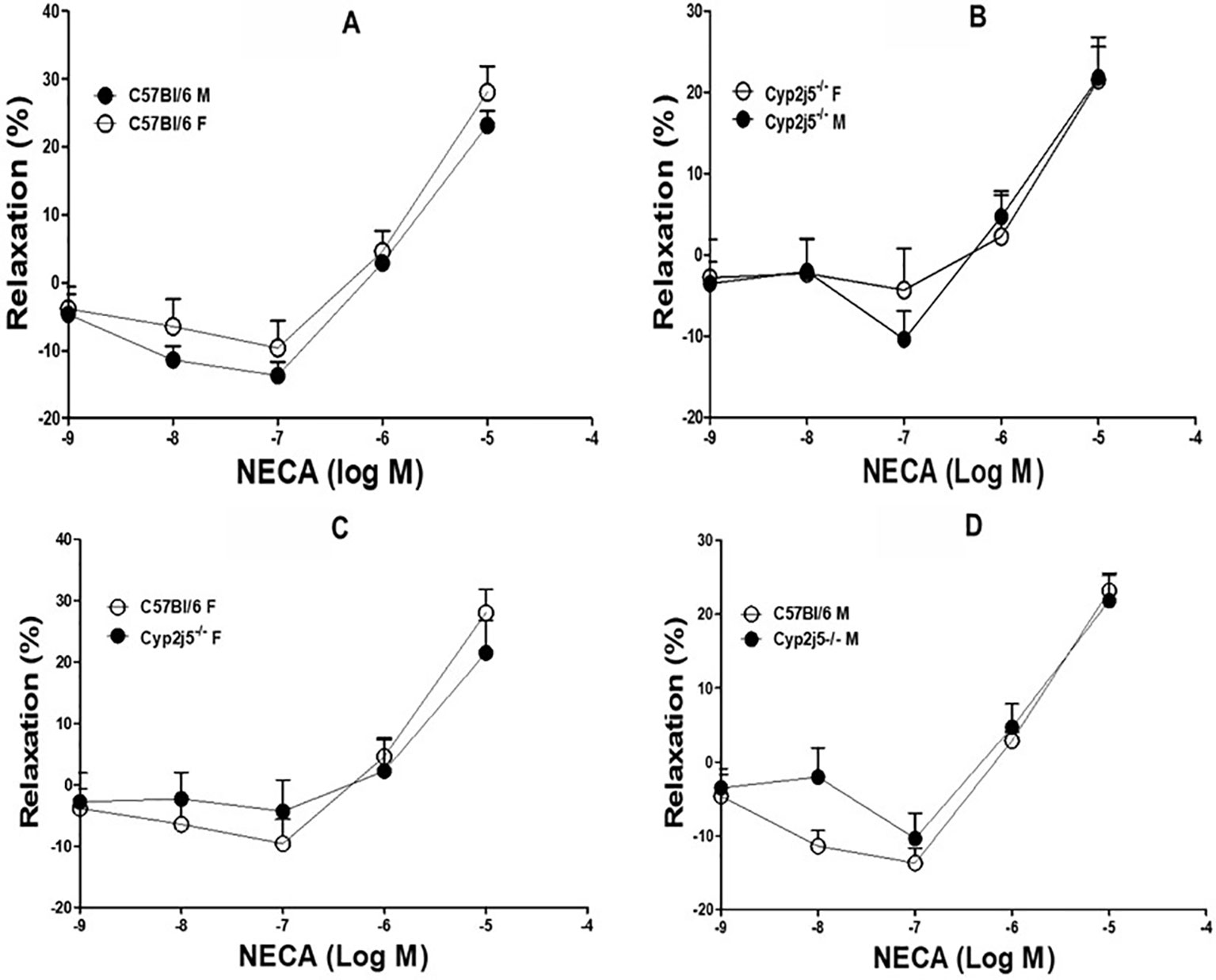
Figure 4 5'-N-Ethylcarboxamidoadenosine (NECA)-induced concentration dependent vascular response in aortic rings of C57Bl/6 and Cyp2j5−/− mice. P > 0.05 between C57Bl/6 (M) vs. C57Bl/6 (F) mice (A), P > 0.05 between Cyp2j5−/− (M) vs. Cyp2j5−/− (F) mice (B), P > 0.05 between Cyp2j5−/− (F) vs. C57Bl/6 (F) mice (C) and P > 0.05 between Cyp2j5−/− (M) vs. C57Bl/6 (M) mice (D). Values expressed as means ± SE. n = eight per group.
DDRC for NECA and the effect of Ang-II (10−6 M) on male Cyp2j5−/− vs. male C57Bl/6 mice: NECA produced a concentration-dependent relaxation in both male Cyp2j5−/− and male C57Bl/6 mice. Ang-II (10−6 M) attenuates NECA-induced concentration-dependent relaxation in both male C57Bl/6 (at NECA 10−7–10−5 M; −17.8 ± 1.4, −*9.5 ± 1.1, *3.2 ± 2.7 vs. non-treated −13.7 ± 2.0, 2.9 ± 1.1, 23.1 ± 2.1, *P < 0.05, Figure 5A) and male Cyp2j5−/− (at NECA 10−7–10−5 M; −#20.85 ± 3.2, −#17.1± 2.3, −#10.8 ± 2.3 vs. non-treated −10.4 ± 3.5, 4.7 ± 3.1, 21.8 ± 3.8, #P < 0.05, Figure 5A) mice. Interestingly, Ang-II treatment attenuates NECA-induced concentration-dependent relaxation in Cyp2j5−/− vs. Ang-II treated C57Bl/6 mice (P <0.05, Figure 5A). However, no significant difference was observed between male Cyp2j5−/− vs. male C57Bl/6 mice (NECA-10−7–10−6 M, Figure 5A, P >0.05).
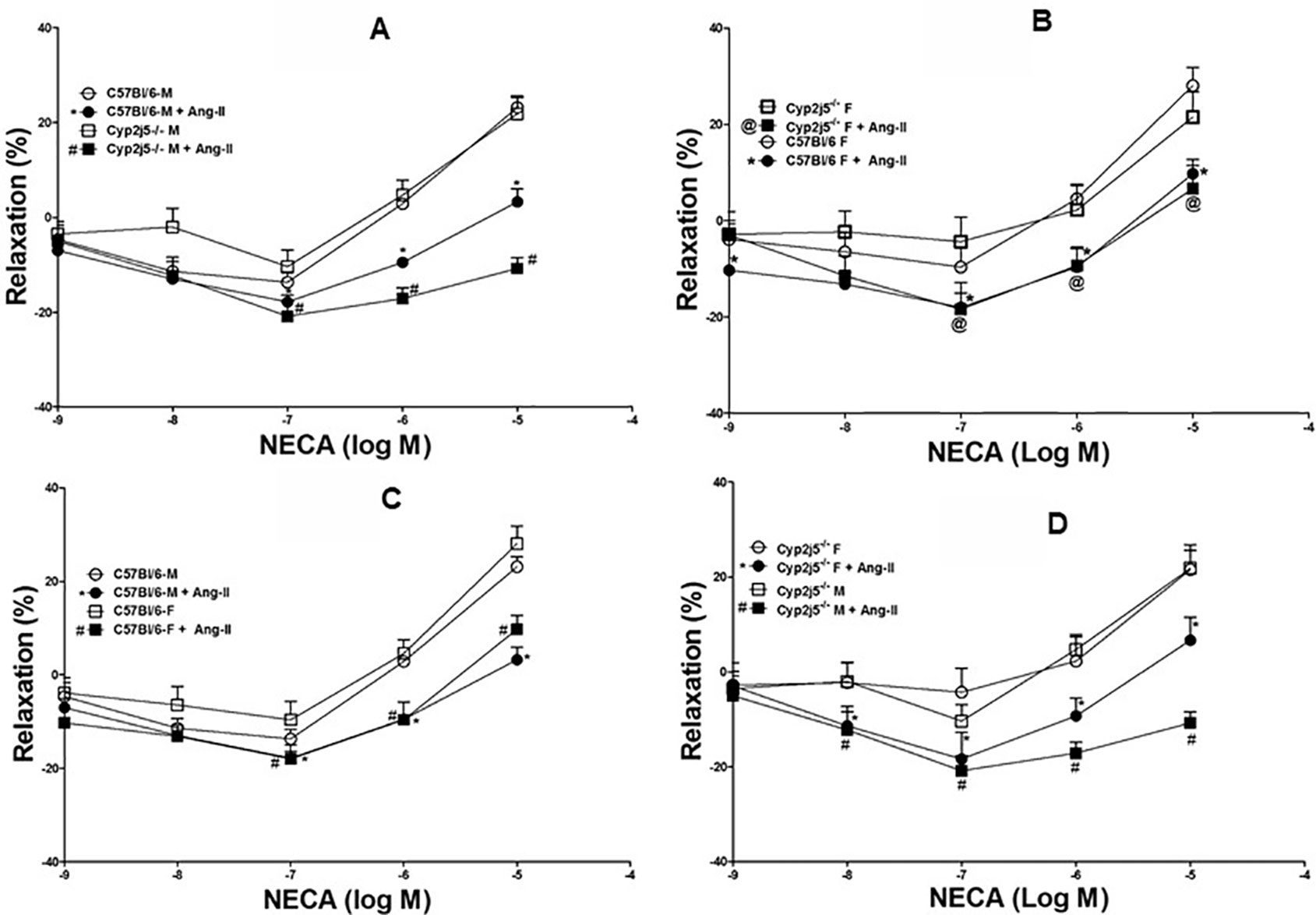
Figure 5 (A) Effect of angiotensin II (Ang-II) (10−6 M) on 5'-N-ethylcarboxamidoadenosine (NECA)-induced concentration dependent vascular response in aortic rings of C57Bl/6 (M) and Cyp2j5−/− (M) mice. *P < 0.05 between non-treated C57Bl/6 (M) vs. Ang-II treated C57Bl/6 (M) mice, P > 0.05 between non-treated C57Bl/6 (M) vs. non-treated Cyp2j5−/− (M) mice, #P < 0.05 between non-treated C57Bl/6 (M) vs. Ang-II-treated Cyp2j5−/− (M) mice. #P < 0.05 between non-treated Cyp2j5−/− (M) and Ang-II treated Cyp2j5−/− (M) mice and between Ang-II treated Cyp2j5−/− (M) vs. Ang-II treated C57Bl/6 (M) mice (#*P < 0.05). Values expressed as means ± SE. n = eight per group (5A). (B) Ang-II effect was tested on NECA-induced response in C57Bl/6 (F) and Cyp2j5−/− (F) mouse aortas. *P < 0.05 between non-treated C57Bl/6 (F) vs. Ang-II treated C57Bl/6 (F) mice, P > 0.05 between non-treated C57Bl/6 (F) vs. non-treated Cyp2j5−/− (F) mice, @P < 0.05 between non-treated C57Bl/6 (F) vs. Ang-II-treated Cyp2j5−/− (F) mice. @P < 0.05 between non-treated Cyp2j5−/− (F) and Ang-II treated Cyp2j5−/− (F) mice and between Ang-II treated Cyp2j5−/− (F) vs. Ang-II treated C57Bl/6 (F) mice (@*P > 0.05). Values expressed as means ± SE. n = eight per group (5B). (C) NECA-induced concentration dependent vascular response was tested with Ang-II (10−6 M) in aortic rings of C57Bl/6 (M) and C57Bl/6 (F) mice. *P < 0.05 between non-treated C57Bl/6 (M) vs. Ang-II treated C57Bl/6 (M) mice, P > 0.05 between non-treated C57Bl/6 (M) vs. non-treated C57Bl/6 (F) mice, #P < 0.05 between non-treated C57Bl/6 (F) vs. Ang-II-treated C57Bl/6 (F) mice. @P < 0.05 between non-treated C57Bl/6 (F) and Ang-II treated C57Bl/6 (F) mice and between Ang-II treated C57Bl/6 (F) vs. Ang-II treated C57Bl/6 (M) mice (#*P > 0.05). Values expressed as means ± SE. n = eight per group (C). (D) Effects of Ang-II on NECA-induced concentration dependent vascular response in aortic rings of Cy2j5−/− (M) and Cy2j5−/− (F) mice. #P < 0.05 between non-treated Cy2j5−/− (M) vs. Ang-II treated Cy2j5−/− (M) mice, P > 0.05 between non-treated Cy2j5−/− (M) vs. non-treated Cy2j5−/− (F) mice, *P < 0.05 between non-treated Cy2j5−/− (F) vs. Ang-II-treated Cy2j5−/− (F) mice. #P < 0.05 between non-treated Cy2j5−/− (M) and Ang-II treated Cy2j5−/− (F) mice, and between Ang-II treated Cy2j5−/− (F) vs. Ang-II treated Cy2j5−/− (M) mice (#*P < 0.05). Values expressed as means ± SE. n = eight per group (5D).
DDRC for NECA and the effects of Ang-II (10−6 M) in female Cyp2j5−/− vs. female C57Bl/6 mice: NECA produced a concentration-dependent relaxation in both female Cyp2j5−/− and female C57Bl/6 mice. Ang-II (10−6 M) attenuates NECA-induced concentration-dependent relaxation in both female C57Bl/6 (at NECA 10−7–10−5 M; −*18.00 ± 3.02, −*9.6 ± 3.8, *9.7 ± 3.0 vs. non-treated −9.6 ± 4.0, 4.6 ± 3.0, 28.0 ± 3.8, *P < 0.05, Figure 5B) and female Cyp2j5−/− (at NECA 10−7–10−5 M; −@18.4 ± 5.6, −@9.3± 3.8, @6.6 ± 4.8 vs. non-treated −4.3 ± 5.1, 2.2 ± 5.1, 21.5 ± 5.3, @P < 0.05, Figure 5B) mice. Ang-II treatment attenuates NECA-induced concentration-dependent relaxation equally in both in female Cyp2j5−/− and female C57Bl/6 mice vs. non-treated female Cyp2j5−/− and female C57Bl/6 mice (P < 0.05, Figure 5B). However, no significant difference was observed between female Cyp2j5−/− vs. female C57Bl/6 mice (NECA-10−7–10−6 M, Figure 5B, P > 0.05).
DDRC for NECA and the effects of Ang-II (10−6 M) in male C57Bl/6 vs. female C57Bl/6 mice: NECA produced a concentration-dependent relaxation in both male C57Bl/6 and female C57Bl/6 mice. Ang-II (10−6 M) attenuates NECA-induced concentration-dependent relaxation in both female C57Bl/6 (at NECA 10−7–10−5 M; −*18.0 ± 3.0, −*9.6 ± 3.8, *9.7 ± 3.0 vs. non-treated −9.6 ± 4.0, 4.6 ± 3.0, 28.0 ± 3.8, *P < 0.05, Figure 5C) and male C57Bl/6 (at NECA 10−7–10−5 M; −#17.8 ± 1.4, −#9.5± 1.1, #3.2 ± 2.7 vs. non-treated −13.7 ± 2.0, 2.9 ± 1.1, 23.1 ± 2.1, #P < 0.05, Figure 5C) mice. Ang-II treatment attenuates NECA-induced concentration-dependent relaxation equally in both in female C57Bl/6 and male C57Bl/6 mice vs. non-treated female and male C57Bl/6 mice (P < 0.05, Figure 5C). However, no significant difference was observed between female C57Bl/6 vs. male C57Bl/6 mice (NECA-10−7–10−5 M, Figure 5C, P > 0.05).
DDRC for NECA and the effects of Ang-II (10−6 M) in male Cyp2j5−/− vs. female Cyp2j5−/− mice: NECA produced a concentration-dependent relaxation in both male Cyp2j5−/− and female Cyp2j5−/− mice. Ang-II (10−6 M) attenuates NECA-induced concentration-dependent relaxation in both female Cyp2j5−/− (at NECA 10−7–10−5 M; −*18.4 ± 5.6, −*9.3 ± 3.8, *6.6 ± 4.8 vs. non-treated −4.3 ± 5.1, 2.2 ± 5.1, 21.5 ± 5.3, *P < 0.05, Figure 5D) and male Cyp2j5−/− (at NECA 10−7–10−5 M; −#20.8 ± 3.2, −#17.1± 2.3, −#10.8 ± 2.3 vs. non-treated −10.4 ± 3.5, 4.7 ± 3.1, 21.8 ± 3.8, #P < 0.05, Figure 5D) mice. Interestingly, Ang-II treatment attenuates more NECA-induced concentration-dependent relaxation in male Cyp2j5−/− mice vs. female Cyp2j5−/− mice (P <0.05, Figure 5D). However, no significant difference was observed between female Cyp2j5−/− vs. male Cyp2j5−/− mice (NECA-10−7–10−6 M, Figure 5D, P > 0.05).
DDRC for CGS 21680 (A2A AR-agonist) in DDMS (10−5 M, 20-HETE synthesis inhibitor) + Ang-II (10−6 M) in male Cyp2j5−/− vs. non-treated male Cyp2j5−/− mice: CGS 21680 produced an enhanced concentration-dependent relaxation in male DDMS-treated Cyp2j5−/− mice (at CGS 21680 10−7–10−5 M; 9.7 ± 2.5, 21.5 ± 2.8, 30.5 ± 2.7) vs. non-treated male Cyp2j5−/− mice (at CGS 21680 10−7–10−5M; −@1.6 ± 1.1, @12.7 ± 1.1, @20.5 ± 1.8, @P < 0.05). In addition, no significant change was observed between DDMS + Ang-II treated male Cyp2j5−/− mice (at CGS 21680 10−7–10−5M; −6.4 ± 1.7, 9.4 ± 1.7, 15.8 ± 1.9) vs. non-treated male Cyp2j5−/− mice (at CGS 21680 10−7–10−5M; −1.6 ± 1.1, 12.7 ± 1.1, 20.5 ± 1.8, P > 0.05). However, a significant difference was observed between DDMS-treated male Cyp2j5−/− mice (at CGS 21680 10−7–10−5M; 9.7 ± 2.5, 21.5 ± 2.8, 30.5 ± 2.7) vs. DDMS + Ang-II treated male Cyp2j5−/− mice (at CGS 21680 10−7–10−5M; −*6.4 ± 1.7, *9.4 ± 1.7, *15.8 ± 1.9, *P < 0.05). Similar trends were observed earlier in DDMS treated vs. non-treated C57Bl/6 mice (Nayeem et al., 2009; Nayeem et al., 2010; Nayeem et al., 2011; Ponnoth et al., 2012a; Yadav et al., 2015).
DDRC for CGS 21680 in DDMS (10−5 M) + Ang-II (10−6 M) in female Cyp2j5−/− vs. non-treated female Cyp2j5−/− mice: CGS 21680 produced an enhanced concentration-dependent relaxation in female DDMS-treated Cyp2j5−/− mice (at CGS 21680 10−7–10−5M; 7.9 ± 2.1, 17.2 ± 2.3, 25.2 ± 2.4) vs. non-treated female Cyp2j5−/− mice (at CGS 21680 10−7–10−5M; −4.6 ± 1.1, 5.7 ± 1.1, 15.5 ± 1.8, @P < 0.05). In addition, no significant change was observed between DDMS + Ang-II treated female Cyp2j5−/− mice (at CGS 21680 10−7–10−5M; −15.8 ± 2.6, 2.3 ± 1.2, 9.2 ± 1.5) vs. non-treated female Cyp2j5−/− mice except at 10−7 M (at CGS 21680 10−7–10−5 M; −*4.6 ± 1.1, 5.7 ± 1.1, 15.5 ± 1.8, *P < 0.05). Whereas, a significant difference was observed between female DDMS-treated Cyp2j5−/− mice (at CGS 21680 10−7–10−5 M; 7.9 ± 2.1, 17.2 ± 2.3, 25.2 ± 2.4) vs. DDMS + Ang-II treated female Cyp2j5−/− mice (at CGS 21680 10−7–10−5 M; −*15.8 ± 2.6, *2.3 ± 1.2, *9.2 ± 1.5, *P < 0.05). Similar trends were observed earlier in DDMS treated vs. non-treated C57Bl/6 mice (Nayeem et al., 2009; Nayeem et al., 2010; Nayeem et al., 2011; Ponnoth et al., 2012a; Yadav et al., 2015).
Discussion
This is the first study to investigate the ACh-, adenosine (NECA)-, and A2A AR agonist (CGS 21680)-induced concentration-dependent vascular (aortic) response and their interactions among CYP-epoxygenases, ω-hydroxlases and Ang-II in male vs. female Cyp2j5−/− mice and Cyp2j5−/− vs. C57Bl/6 mice. We report novel findings regarding the vascular (aortic) response between Cyp2j5−/− vs. C57Bl/6 mice and their interactions among CYP-epoxygenase, ω-hydroxlase and Ang-II. (1) Disruption of Cyp2j5 gene in mice (Cyp2j5−/−) reduced ACh-induced concentration-dependent relaxation compared to C57Bl/6 mice. Thus, it is clear that Cyp2j5 has its own role in ACh-induced vascular response. Loss of Cyp2j5 showed reduced ACh-induced relaxation, and Cyp2j5 protein is also detected in kidneys/mouse aorta/visceral adipose tissues, and is involved in the conversion of AAs into EETs (Ma et al., 2004; Burgess et al., 2012; Nayeem et al., 2013). (2) CYP-epoxygenase inhibitor (MS-PPOH) partially attenuated ACh-induced concentration dependent relaxation in C57Bl/6, but not in Cyp2j5−/− mice. Thus, Cyp2j5 enzyme activity is involved in ACh-induced concentration dependent relaxation in C57Bl/6 mice. (3) Ang-II attenuates ACh-induced concentration dependent relaxation in Cyp2j5−/− compared to C57Bl/6 mice. Therefore, we conclude that Cyp2j5 enzyme is involved in ACh-induced concentration dependent vascular response in Ang-II–treated mice. (4) There was no difference found between the presence and absence of Cyp2j5 in mice related to adenosine (NECA)-induced vascular response. (5) Ang-II treatment significantly attenuated NECA-induced concentration dependent relaxation in Cyp2j5−/− (M) and C57Bl/6 (M) mice, and the attenuation of NECA-induced concentration dependent relaxation due to Ang-II infused Cyp2j5−/− (M) is much higher than C57Bl/6 (M) mice. Therefore, presence of Cyp2j5 in C57Bl/6 (M) mice plays an important role in resisting the reduction of NECA-induced concentration dependent relaxation due to Ang-II in male mice. (6) Ang-II treatment was able to significantly attenuate NECA-induced concentration dependent relaxation in both Cyp2j5−/− (F) and C57Bl/6 (F) mice, and a similar effect was observed in Cyp2j5−/− (M) vs. C57Bl/6 (M) mice after Ang-II treatment vs. non-treated. (7) However, the attenuation of NECA-induced concentration dependent relaxation due to Ang-II treated Cyp2j5−/− (M) is much higher than Cyp2j5−/− (F) mice. Therefore, the Cyp2j5−/− (M) may be more sensitive to Ang-II in NECA-induced response compared to Cyp2j5−/− (F) mice. (8) ω-hydroyxlase inhibitor (DDMS) enhances A2AAR (CGS 21680)-induced concentration dependent relaxation in both male and female Cyp2j5−/− mice compared non-treated male and female Cyp2j5−/− mice, and DDMS + Ang-II treated male/female Cyp2j5−/− mice are not different from the non-treated male/female Cyp2j5−/− mice in regards to their CGS 21680-induced concentration dependent vascular response. Thus, ω-hydroyxlases are involved in the attenuation of CGS 21680-induced concentration dependent vascular relaxation while Ang-II infused in both male and female Cyp2j5−/− mice. According to our data, Cyp2j5 plays an important role in the in ACh-, NECA-, and CGS 21680-induced concentration dependent regulation of vascular response.
CYP450-epoxygenase (CYP2J2) polymorphisms have been reported in different populations related to its role in cardiovascular function, including hypertension (Lee et al., 2007; Feng et al., 2008; Fava et al., 2010; Jie et al., 2010; Wang et al., 2010; Zordoky and El-Kadi, 2010; Xu et al., 2011). The Cyp2j5-epoxygenase in mouse (chromosome-4) appears to be as important as CYP2J2-epoxygenase in human (chromosome-1) and CYP2J4 in rat (chromosome-5) (Olona et al., 2018). Variations in soluble epoxide hydrolase and ω-hydroxylase genes in human population also alter the risk of coronary heart disease, ischemic stroke, restenosis, diabetes heart, heart failure, ischemic stroke in Caucasians, Chinese, and in the African Americans with hypertension (Lee et al., 2006; Burdon et al., 2008; Monti et al., 2008; Kullmann et al., 2009; Fava et al., 2010; Zordoky and El-Kadi, 2010).
Role of Cyp2j5 in ACh-induced vascular response: ACh-induced concentration dependent aortic relaxation in Cyp2j5−/− was significantly different compared to C57Bl/6 mice (Figure 1). ACh data between Cyp2j5−/− and C57Bl/6 mice suggest that lack of Cyp2j5-epoxygenase (less EETs generation) contributes less in ACh-induced concentration dependent aortic relaxation in Cyp2j5−/− compared to C57Bl/6 mice, and ACh-induced relaxation is almost completely dependent on NO. As Hercule, et al. indicated, CYP450-eicosanoids activates endothelial nitric oxide (NO) synthase and NO release in Ephx2−/− (more EETs) and wild-type (WT) mouse mesenteric arteries (Hercule et al., 2009). In addition, EETs have been demonstrated to increase NO release; in bovine aortic endothelial cells (cultured) EETs can induce NO release, which may modulate vascular tone (Wang et al., 2003; Hercule et al., 2009). Therefore, according to the current study, we believe Cyp2j5−/− mice, which lack Cyp2j5, may have reduced EET formation and subsequent ACh-induced and NO-dependent aortic relaxation compared to C57Bl/6 mice. Because, we found overexpression of Cyp2j5 protein and up-regulation of EET oxylipins (isolated heart perfusate) in Ephx2−/− compared to C57Bl/6 mice with enhanced aortic and coronary reactive hyperemic response (Nayeem et al., 2013; Hanif et al., 2016a), and endothelial vascular overexpression of human CYP2J2 had up-regulation of EET oxylipins (isolated heart perfusate) in CYP2J2 (Tie2-CYP2J2 Tr) compared to C57Bl/6 mice with enhanced coronary reactive hyperemic response (Hanif et al., 2017b).
Role of CYP-epoxygenases in ACh-induced relaxation: MS-PPOH (CYP-epoxygenases inhibitor) was able to partially block ACh-induced concentration dependent aortic relaxation in C57Bl/6 mice, but not in Cyp2j5−/− compared to non-treated mice (Figure 2). There is no difference was found between MS-PPOH treated Cyp2j5−/− vs. non-treated Cyp2j5−/− mice. This suggests that Cyp2j5 is a main contributor in the formation of epoxides from AA in mouse aorta, as demonstrated previously that mouse kidneys have higher Cyp2j5 expression in male compared to female mice after puberty (Ma et al., 2004), and Northern analysis also revealed that Cyp2j5 transcripts were more abundant in adult male versus adult female kidneys (Ma et al., 2004). Burgess et al., showed that CYP2J5 is responsible for production of primarily 14,15 and 11,12 EETs in visceral adipose tissue (Burgess et al., 2012), and the overexpression of Cyp2j5 and Cyp4a proteins were observed in Ephx2−/− vs. C57Bl/6 mice with enhanced adenosine (NECA) and CGS 21680 (A2A AR)-induced aortic relaxation (Nayeem et al., 2013). Relative loss of ACh-induced concentration dependent aortic relaxation in Cyp2j5−/− vs. C57Bl/6 mice and no change between MS-PPOH treated Cyp2j5−/− vs. non-treated Cyp2j5−/− mice indicates a central role of CYP-epoxygenases like Cyp2j5 in ACh-induced concentration dependent aortic relaxation. This is similar to that reported in mouse mesenteric arteries (Hercule et al., 2009).
Effect of Ang-II in ACh-induced relaxation: Ang-II was able to partially block ACh-induced concentration dependent aortic relaxation in both Cyp2j5−/− and C57Bl/6 mice compared to non-treated mice (Figure 3), and a significant difference was found between the Ang-II-treated C57Bl/6 vs. non-treated C57Bl/6 mice and Ang-II-treated Cyp2j5−/− vs. non-treated Cyp2j5−/− mice (Figure 3). Attenuation of ACh-induced concentration dependent aortic relaxation was greater in Ang-II-treated Cyp2j5−/− vs. non-treated C57Bl/6 mice compared to Ang-II-treated C57Bl/6 vs. non-treated C57Bl/6 mice. This shows that the lack of Cyp2j5 gene in Cyp2j5−/− mice has less advantage over C57Bl/6 to block the action of Ang-II in ACh-induced concentration dependent aortic relaxation.
Deletion of Cyp2j5 (Cyp2j5−/−) or inhibition (through MS-PPOH) of CYP-epoxygenases activity causes less EET generation from arachidonic acid (AA) metabolism and leads to a decrease in ACh-concentration dependent relaxation. Ang-II blocked ACh-induced concentration dependent relaxation in both Cyp2j5−/− and C57Bl/6 mice. However, in the absence of Cyp2j5, Ang-II blocking action is significantly more than the blocking action in C57Bl/6 mice. Therefore, we conclude that inhibition or deletion of CYP-epoxygenases in mice may enhance the toxic action of Ang-II in ACh-induced concentration dependent relaxation in Cyp2j5−/− compared to C57Bl/6 mice. Also, deletion or inhibition of CYP-epoxygenases activity causes less EETs to form and involved in vascular contraction, reduced coronary reactive hyperemic response with the association of vascular inflammation (Nayeem et al., 2008; Nayeem et al., 2009; Nayeem et al., 2010; Nayeem et al., 2011; Ponnoth et al., 2012b; Hanif et al., 2016b; Hanif et al., 2017b).
Role of Cyp2j5 in NECA-induced vascular response and effect of Ang-II in NECA-induced vascular response: there was no difference between the presence or absence of Cyp2j5 in both male vs. female or male vs. female C57Bl/6 mice regarding NECA-induced concentration dependent relaxation (Figures 4A–D), which is not similar to ACh-induced concentration dependent vascular response where Cyp2j5−/− had lesser relaxation compared C57Bl/6 mice (Figure 1). But, Ang-II treatment was able to block NECA-induced concentration dependent aortic relaxation in both male Cyp2j5−/− and male C57Bl/6 mice compared to non-treated male mice (Figure 5A), and a large difference was found between the Ang-II-treated male C57Bl/6 vs. non-treated male C57Bl/6 mice and a significant difference was observed between Ang-II-treated male Cyp2j5−/− vs. non-treated male Cyp2j5−/− mice (Figure 5A). Attenuation of NECA-induced concentration dependent aortic relaxation was greater in Ang-II-treated male Cyp2j5−/− vs. Ang-II-treated male C57Bl/6 mice (Figure 5A). In addition, there was significant difference observed between Ang-II-treated male Cyp2j5−/− vs. Ang-II-treated male C57Bl/6 mice (Figure 5A). This shows that the lack of Cyp2j5 gene in Cyp2j5−/− mice has less advantage over C57Bl/6 to block the action of Ang-II in NECA-induced concentration dependent aortic relaxation. However, Ang-II blocking action on the NECA-induced concentration dependent relaxation is stronger in male Cyp2j5−/− than the blocking action in male C57Bl/6 mice. Therefore, we conclude that inhibition or deletion of CYP-epoxygenases in mice may have stronger toxic action of Ang-II in NECA-induced concentration dependent relaxation in male Cyp2j5−/− compared to male C57Bl/6 mice, because deletion or inhibition of CYP-epoxygenases activity causes less EETs to form and involved in vasoconstriction, reduced coronary reactive hyperemic response with the association of vascular inflammation (Nayeem et al., 2008; Nayeem et al., 2009; Nayeem et al., 2010; Nayeem et al., 2011; Ponnoth et al., 2012b; Hanif et al., 2016b; Hanif et al., 2017b).
There were no significant differences observed between female Cyp2j5−/− vs. female C57Bl/6 mice, similar to male Cyp2j5−/− vs. male C57Bl/6 mice. Lower NECA-induced relaxation was observed in Ang-II infused female C57Bl/6/Ang-II infused female Cyp2j5−/− mice compared to non-treated female Cyp2j5−/−/female C57Bl/6 mice (Figure 5B). However, no significant difference in NECA-induced concentration dependent vascular response was observed between Ang-II infused female C57Bl/6 vs. Ang-II infused female Cyp2j5−/− mice (Figure 5B), and there was no significant difference in NECA-induced concentration dependent vascular response observed between Ang-II infused male C57Bl/6 vs. Ang-II infused female C57Bl/6 mice (Figure 5C). In contrast, Ang-II blocking action on the NECA-induced concentration dependent relaxation is stronger in male Cyp2j5−/− vs. Ang-II + male C57Bl/6 mice compared to Ang-II + female Cyp2j5−/− vs. Ang-II + C57Bl/6 female mice (Figures 5A, B). Also, Ang-II blocking action on the NECA-induced concentration dependent relaxation is more dominant in male Cyp2j5−/− vs. Ang-II + female Cyp2j5−/− mice compared to Ang-II + female Cyp2j5−/− vs. female Cyp2j5−/− mice (Figure 5D). This disparity between Ang-II treated male Cyp2j5−/− vs. Ang-II treated female Cyp2j5−/− mice were prominent in NECA-induced vascular response. However, this type of difference in NECA-induced vascular response was not observed between non-treated male vs. female Cyp2j5−/− mice. This observation is in agreement with Ma et al. where mouse kidneys demonstrated higher Cyp2j5 expression in male compared to female mice after puberty, and Northern analysis also revealed that Cyp2j5 transcripts were more abundant in adult male versus adult female kidneys (Ma et al., 2004). However, an increased in blood pressure reported with enhanced renal (afferent arterioles) vasoconstriction with angiotensin II in female Cyp2j5−/− compared to its respective female wild-type mice (Athirakul et al., 2008). In contrast, we demonstrated in the current study that an enhanced adenosine (NECA)-induced aortic vasoconstriction in male vs. female Cyp2j5−/− mice when Ang-II infused. This difference may be due to different blood vessels (renal afferent arterioles vs. aorta) and different media (Ang-II alone vs. NECA + Ang-II). Before Ang-II treatment, there was no significant difference noticed between male vs. female Cyp2j5−/− and between C57Bl/6 vs. Cyp2j5−/− mice in NECA-induced aortic response. However, there was no significant difference observed in acetylcholine-concentration dependent aortic response between male vs. female Cyp2j5−/− mice when Ang-II infused, but the significant difference was observed between C57Bl/6 vs. Cyp2j5−/− mice in acetylcholine-concentration dependent aortic response without Ang-II treatment. Also, Burgess et al., showed that Cyp2j5 is responsible for production of primarily 14,15- and 11,12-EETs in visceral adipose tissue (Burgess et al., 2012), and the overexpression of Cyp2j5 and Cyp4a proteins were observed in Ephx2−/− vs. C57Bl/6 mice with enhanced adenosine (NECA) and CGS 21680 (A2A AR)-induced aortic relaxation (Nayeem et al., 2013). Any deletion or inhibition of CYP-epoxygenases activity causes less EETs to form and participate in vasoconstriction and reduced coronary reactive hyperemic response with the association of vascular inflammation (Nayeem et al., 2008; Nayeem et al., 2009; Nayeem et al., 2010; Nayeem et al., 2011; Ponnoth et al., 2012b; Hanif et al., 2016b; Hanif et al., 2017b).
NECA is an analogue of adenosine, and adenosine activates four adenosine receptors (A1AR, A2A AR, A2B AR, and A3 AR). Out of these four receptors, A1AR and A3 AR are involved in vascular contraction, whereas A2A AR and A2B AR are vasodilators. Previously, we have NECA concentration dependent vascular response with Ang-II treatment in both Cyp2j5−/− and C57Bl/6 mice. Now, we used CGS 21680 (A2A AR agonist), DDMS (20-HETE synthesis inhibitor, dibromo-dodecenyl-methylsulfimide), and Ang-II. There was a significant difference noted between DDMS-treated male Cyp2j5−/− vs. non-treated male Cyp2j5−/− mice, DDMS enhanced CGS 21680-concentration dependent relaxation male Cyp2j5−/− vs. non-treated male Cyp2j5−/− mice, whereas, no significant difference in CGS 21680-concentration dependent vascular response was observed in between DDMS + Ang-II treated male Cyp2j5−/− vs. non-treated male Cyp2j5−/− mice. In contrast, a huge difference in CGS 21680-concentration dependent vascular response was observed between DDMS + Ang-II treated male Cyp2j5−/− vs. DDMS-treated male Cyp2j5−/− mice. Similarly, there was a significant difference observed between DDMS-treated female Cyp2j5−/− vs. non-treated female Cyp2j5−/− mice, DDMS treatment increased CGS 21680-concentration dependent relaxation in female Cyp2j5−/− vs. non-treated female Cyp2j5−/− mice, whereas, no significant difference in CGS 21680-concentration dependent vascular response was observed in between DDMS + Ang-II treated female Cyp2j5−/− vs. non-treated female Cyp2j5−/− mice, except at 10−7 M CGS 21680. However, a highly significant difference in CGS 21680-concentration dependent vascular response was observed between DDMS + Ang-II treated female Cyp2j5−/− vs. DDMS-treated female Cyp2j5−/− mice. These observations are in agreement with our earlier work, where A2A AR (CGS 21680)-induced concentration dependent enhanced vascular (aortic) relaxation in C57Bl/6 vs. A2A AR−/− mice, enhanced relaxation was abolished with MS-PPOH (CYP-epoxygenases inhibitor) and enhanced relaxation in Ephx2−/− (more EETs) vs. C57Bl/6 mice (Nayeem et al., 2008; Ponnoth et al., 2012b; Nayeem et al., 2013; Pradhan et al., 2014; Pradhan et al., 2015). Also, our current observation is in agreement with our lab reports, where, NECA- or CGS 21680-induced vascular relaxation were enhanced with the treatment of DDMS (ω-hydroxylase or 20-HETE inhibitor) or HET0016 (ω-hydroxylase or 20-HETE inhibitor) in mouse aorta or mouse mesenteric arteries with or without Ang-II treatment (Nayeem et al., 2009; Nayeem et al., 2010; Nayeem et al., 2011; Ponnoth et al., 2012a; Kunduri et al., 2013; Yadav et al., 2015; Yadav et al., 2016).
In summary, the result reported here provide new insights that inhibition or absence of Cyp2j5 enzyme through deletion of Cyp2j5 gene appears to be critical for acetylcholine- or NECA- or CGS 21680-induced concentration dependent vascular response while challenged by angiotensin-II or MS-PPOH (Figure 6). When the Cyp2j5 gene was absent (Cyp2j5−/− mice), ACh-induced concentration dependent relaxation was significantly lower than C57Bl/6 mice. However, when the ACh-induced concentration dependent relaxation was challenged by MS-PPOH, absence of Cyp2j5 made no significant difference in the ACh-induced concentration dependent relaxation compared to the non-treated Cyp2j5−/− mice. In contrast MS-PPOH-treated C57Bl/6 mice made a significant difference compared to non-treated C57Bl/6 mice. When the ACh-induced concentration dependent relaxation was challenged by Ang-II in Cyp2j5−/− mice, a significant difference was observed in the blocking action on ACh-induced concentration dependent relaxation compared to C57Bl/6 mice. Similarly, when the NECA-induced concentration dependent relaxation was challenged by Ang-II in male Cyp2j5−/− vs. female Cyp2j5−/− mice and male Cyp2j5−/− vs. male C57Bl/6 mice, a significantly huge difference in the blocking of NECA-induced concentration dependent relaxation was observed. However, NECA-induced concentration dependent vascular response itself did not make any significant difference between the Cyp2j5−/− vs. C57Bl/6 mice. In addition, DDMS enhanced CGS 21680-induced concentration dependent relaxation in DDMS treated Cyp2j5−/− vs. non-treated Cyp2j5−/− mice, and there was no difference was observed between DDMS + Ang-II + Cyp2j5−/− vs. non-treated Cyp2j5−/− mice. Therefore, we conclude that inhibition or deletion of Cyp2j5 in mice may have an increase in toxic action of both Ang-II and MS-PPOH in ACh/NECA-induced concentration dependent relaxation in Cyp2j5−/− compared to C57Bl/6 mice, and DDMS may have rescued from the toxic effect of Ang-II treatment in Cyp2j5−/− mice.
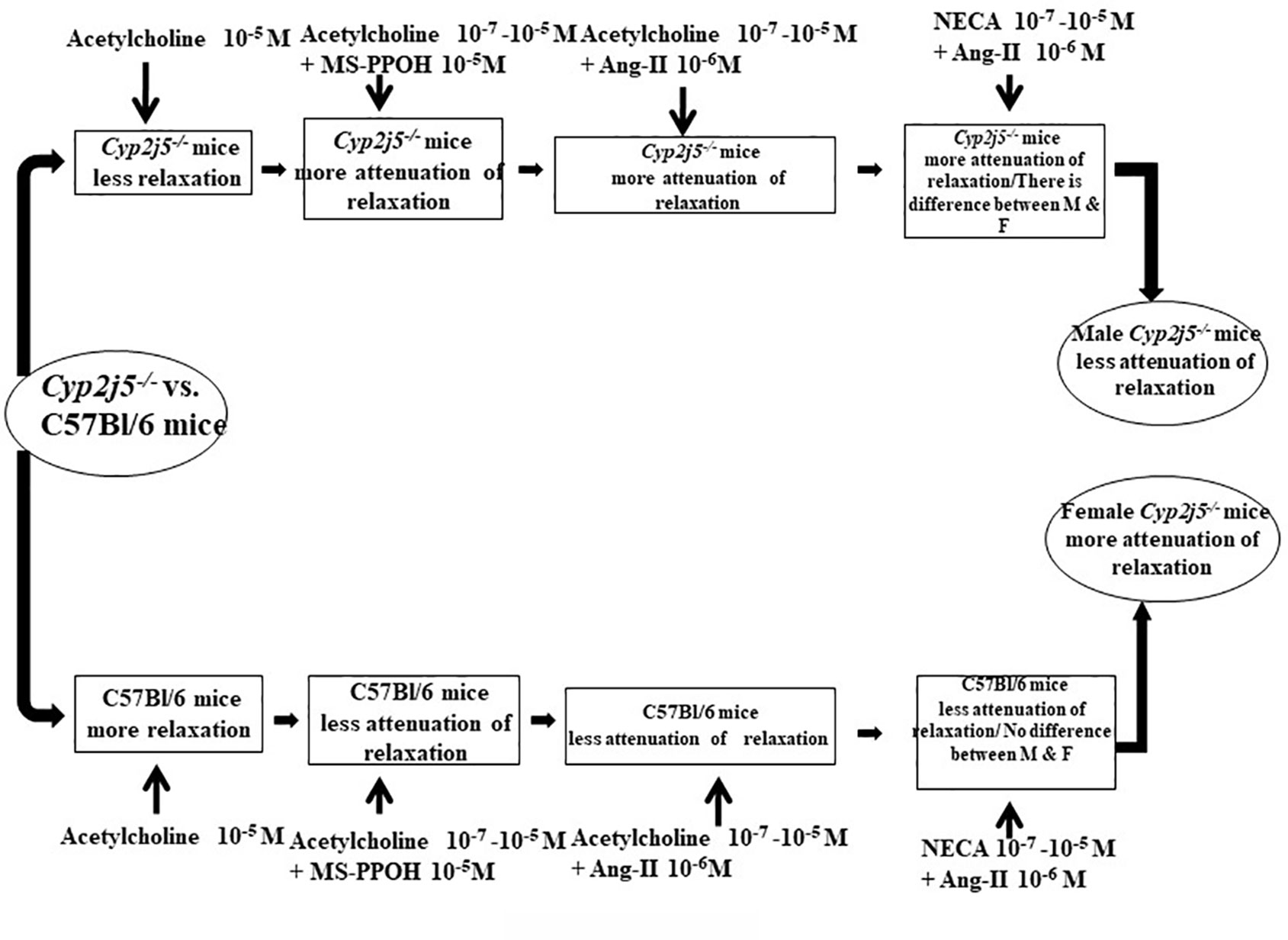
Figure 6 Flow diagram: comparison between Cyp2j5−/− vs. C57Bl/6 mice treated with acetylcholine, acetylcholine + MS-PPOH, acetylcholine + angiotensin II (Ang-II), 5'-N-ethylcarboxamidoadenosine (NECA), NECA + Ang-II, and CGS 21680 + dibromo-dodecenyl-methylsulfimide (DDMS) + Ang-II.
Note: Unfortunately, we lost Cyp2j5−/− mouse colony, and this colony is not available anywhere except frozen embryos, therefore, some areas of this manuscript has some limitations. In future, we will revive this colony as the grant gets funded.
Data Availability Statement
All datasets generated for this study are included in the article/supplementary material.
Ethics Statement
West Virginia University Institutional Animal Care and Use Committee approved all animal care and experimentation protocols, which were in accordance with the principles and guidelines of the NIH's Guide for the Care and Use of Laboratory Animals.
Author Contributions
MN: conception, design of research, performing experiments, analysis drafting and editing. SA and AH cooperated in the experimentations, reading, correction, editing and input. ME helped in correction, editing and input and DZ advised, read, corrected, edited, provided transgenic mice and input.
Funding
This work was supported by a National Institutes of Health Grant HL-114559 to MN and a National Institute of Environmental Health Sciences Grant Z01 ES025034 to DZ.
Conflict of Interest
The authors declare that the research was conducted in the absence of any commercial or financial relationships that could be construed as a potential conflict of interest.
Acknowledgments
We are very much thankful to Ms. Brandy J. Wilmoth, B.S., RVT (Biology Technician) for performing tissue bath experiments and thankful to Amanda G. Ammer, PhD. (Research Assistant Professor), Department of Microbiology, Immunology and Cell Biology for editing and correcting this manuscript.
References
Athirakul, K., Bradbury, J. A., Graves, J. P., DeGraff, L. M., Ma, J., Zhao, Y., et al. (2008). Increased blood pressure in mice lacking cytochrome P450 2J5. FASEB J. 22 (12), 4096–4108. doi: 10.1096/fj.08-114413
Burdon, K. P., Lehtinen, A. B., Langefeld, C. D., Carr, J. J., Rich, S. S., Freedman, B. I., et al. (2008). Genetic analysis of the soluble epoxide hydrolase gene, EPHX2, in subclinical cardiovascular disease in the diabetes heart study. Diabetes Vasc. Dis. Res. 5 (2), 128–134. doi: 10.3132/dvdr.2008.021
Burgess, A. P., Vanella, L., Bellner, L., Gotlinger, K., Falck, J. R., Abraham, N. G., et al. (2012). Heme oxygenase (HO-1) rescue of adipocyte dysfunction in HO-2 deficient mice via recruitment of epoxyeicosatrienoic acids (EETs) and adiponectin. Cell Physiol. Biochem. 29 (1-2), 99–110. doi: 10.1159/000337591
Campbell, W. B., Gebremedhin, D., Pratt, P. F., Harder, D. R. (1996). Identification of epoxyeicosatrienoic acids as endothelium-derived hyperpolarizing factors. Circ. Res. 78 (3), 415–423. doi: 10.1161/01.RES.78.3.415
Ellinsworth, D. C., Shukla, N., Fleming, I., Jeremy, J. Y. (2014). Interactions between thromboxane A(2), thromboxane/prostaglandin (TP) receptors, and endothelium-derived hyperpolarization. Cardiovasc. Res. 102 (1), 9–16. doi: 10.1093/cvr/cvu015
Fang, X., Kaduce, T. L., Weintraub, N. L., Harmon, S., Teesch, L. M., Morisseau, C., et al. (2001). Pathways of epoxyeicosatrienoic acid metabolism in endothelial cells. Implications for the vascular effects of soluble epoxide hydrolase inhibition. J. Biol. Chem. 276 (18), 14867–14874. doi: 10.1074/jbc.M011761200
Fava, C., Montagnana, M., Almgren, P., Hedblad, B., Engström, G., Berglund, G., et al. (2010). The common functional polymorphism -50G > T of the CYP2J2 gene is not associated with ischemic coronary and cerebrovascular events in an urban-based sample of Swedes. J. Hypertens. 28 (2), 294–299. doi: 10.1097/HJH.0b013e328333097e
Feng, M., Whitesall, S., Zhang, Y., Beibel, M., D'Alecy, L., DiPetrillo, K. (2008). Validation of volume-pressure recording tail-cuff blood pressure measurements. Am. J. Hypertens. 21 (12), 1288–1291. doi: 10.1038/ajh.2008.301
Fisslthaler, B., Popp, R., Kiss, L., Potente, M., Harder, D. R., Fleming, I., et al. (1999). Cytochrome P450 2C is an EDHF synthase in coronary arteries. Nature 401 (6752), 493–497. doi: 10.1038/46816
Gainer, J. V., Bellamine., A., Dawson, E. P., Womble, K. E., Grant, S. W., Wang, Y., et al. (2005). Functional variant of CYP4A11 20-hydroxyeicosatetraenoic acid synthase is associated with essential hypertension. Circulation 111 (1), 63–69. doi: 10.1161/01.CIR.0000151309.82473.59
Gauthier, K. M., Deeter, C., Krishna, U. M., Reddy, Y. K., Bondlela, M., Falck, J. R., et al. (2002). 14,15-Epoxyeicosa-5(Z)-enoic acid: a selective epoxyeicosatrienoic acid antagonist that inhibits endothelium-dependent hyperpolarization and relaxation in coronary arteries. Circ. Res. 90 (9), 1028–1036. doi: 10.1161/01.RES.0000018162.87285.F8
Hanif, A., Edin, M. L., Zeldin, D. C., Morisseau, C., Nayeem, M. A. (2016a). Deletion of soluble epoxide hydrolase enhances coronary reactive hyperemia in isolated mouse heart: role of oxylipins and PPARgamma. Am. J. Physiol. Regul. Integr. Comp. Physiol. 311 (4), R676–R688. doi: 10.1152/ajpregu.00237.2016
Hanif, A., Edin, M. L., Zeldin, D. C., Morisseau, C., Nayeem, M. A. (2016b). Effect of soluble epoxide hydrolase on the modulation of coronary reactive hyperemia: role of Oxylipins and PPARgamma. PloS One 11 (9), e0162147. doi: 10.1371/journal.pone.0162147
Hanif, A., Edin, M. L., Zeldin, D. C., Morisseau, C., Falck, J. R., Ledent, C., et al. (2017a). Reduced coronary reactive hyperemia in mice was reversed by the soluble epoxide hydrolase inhibitor (t-AUCB): role of adenosine A2A receptor and plasma oxylipins. Prostaglandins Other Lipid Mediat 131, 83–95. doi: 10.1016/j.prostaglandins.2017.09.001
Hanif, A., Edin, M. L., Zeldin, D. C., Morisseau, C., Falck, J. R., Nayeem, M. A. (2017b). Vascular endothelial overexpression of human CYP2J2 (Tie2-CYP2J2 Tr) modulates cardiac oxylipin profiles and enhances coronary reactive hyperemia in mice. PloS One 12 (3), e0174137. doi: 10.1371/journal.pone.0174137
Hercule, H. C., Schunck, W. H., Gross, V., Seringer, J., Leung, F. P., Weldon, S. M., et al. (2009). Interaction between P450 eicosanoids and nitric oxide in the control of arterial tone in mice. Arterioscler. Thromb. Vasc. Biol. 29 (1), 54–60. doi: 10.1161/ATVBAHA.108.171298
Hoopes, S. L., Garcia, V., Edin, M. L., Schwartzman, M. L., Zeldin, D. C. (2015). Vascular actions of 20-HETE. Prostaglandins Other Lipid Mediat 120, 9–16. doi: 10.1016/j.prostaglandins.2015.03.002
Imig, J. D., Falck, J. R., Wei, S., Capdevila, J. H. (2001). Epoxygenase metabolites contribute to nitric oxide-independent afferent arteriolar vasodilation in response to bradykinin. J. Vasc. Res. 38 (3), 247–255. doi: 10.1159/000051053
Imig, J. D. (2000). Afferent arteriolar reactivity to angiotensin II is enhanced during the early phase of angiotensin II hypertension. Am. J. Hypertens. 13 (7), 810–818. doi: 10.1016/S0895-7061(00)00264-8
Jie, Z., Hong, K., Jianhong, T., Biao, C., Yongmei, Z., Jingchuan, L. (2010). Haplotype analysis of the CYP2J2 gene associated with myocardial infarction in a Chinese Han population. Cell Biochem. Funct. 28 (6), 435–439. doi: 10.1002/cbf.1661
King, L. M., Gainer, J. V., David, G. L., Dai, D., Goldstein, J. A., Brown, N. J., et al. (2005). Single nucleotide polymorphisms in the CYP2J2 and CYP2C8 genes and the risk of hypertension. Pharmacogenet. Genomics 15 (1), 7–13. doi: 10.1097/01213011-200501000-00002
Kullmann, S., Binner, P., Rackebrandt, K., Huge, A., Haltern, G., Lankisch, M., et al. (2009). Variation in the human soluble epoxide hydrolase gene and risk of restenosis after percutaneous coronary intervention. BMC Cardiovasc. Disord. 9 (48), 1–7. doi: 10.1186/1471-2261-9-48
Kunduri, S. S., Mustafa, S. J., Ponnoth, D. S., Dick, G. M., Nayeem, M. A. (2013). Adenosine A1 receptors link to smooth muscle contraction via CYP4a, protein kinase C-alpha, and ERK1/2. J. Cardiovasc. Pharmacol. 62 (1), 78–83. doi: 10.1097/FJC.0b013e3182919591
Lee, C. R., North, K. E., Bray, M. S., Fornage, M., Seubert, J. M., Newman, J. W., et al. (2006). Genetic variation in soluble epoxide hydrolase (EPHX2) and risk of coronary heart disease: the Atherosclerosis Risk in Communities (ARIC) study. Hum. Mol. Genet. 15 (10), 1640–1649. doi: 10.1093/hmg/ddl085
Lee, C. R., North, K. E., Bray, M. S., Couper, D. J., Heiss, G., Zeldin, D. C. (2007). CYP2J2 and CYP2C8 polymorphisms and coronary heart disease risk: the Atherosclerosis Risk in Communities (ARIC) study. Pharmacogenet. Genomics 17 (5), 349–358. doi: 10.1097/FPC.0b013e32809913ea
Ma, J., Graves, J., Bradbury, J. A., Zhao, Y., Swope, D. L., King, L., et al. (2004). Regulation of mouse renal CYP2J5 expression by sex hormones. Mol. Pharmacol. 65 (3), 730–743. doi: 10.1124/mol.65.3.730
Makita, K., Takahashi, K., Karara, A., Jacobson, H. R., Falck, J. R., Capdevila, J. H. (1994). Experimental and/or genetically controlled alterations of the renal microsomal cytochrome P450 epoxygenase induce hypertension in rats fed a high salt diet. J. Clin. Invest. 94 (6), 2414–2420. doi: 10.1172/JCI117608
McGiff, J. C., Quilley, J. (2001). 20-hydroxyeicosatetraenoic acid and epoxyeicosatrienoic acids and blood pressure. Curr. Opin. Nephrol. Hypertens. 10 (2), 231–237. doi: 10.1097/00041552-200103000-00012
Monti, J., Fischer, J., Paskas, S., Heinig, M., Schulz, H., Gosele, C., et al. (2008). Soluble epoxide hydrolase is a susceptibility factor for heart failure in a rat model of human disease. Nat. Genet. 40 (5), 529–537. doi: 10.1038/ng.129
Nakagawa, K., Holla, V. R., Wei, Y., Wang, W. H., Gatica, A., Wei, S., et al. (2006). Salt-sensitive hypertension is associated with dysfunctional Cyp4a10 gene and kidney epithelial sodium channel. J. Clin. Invest. 116 (6), 1696–1702. doi: 10.1172/JCI27546
Nayeem, M. A., Poloyac, S. M., Falck, J. R., Zeldin, D. C., Ledent, C., Ponnoth, D. S., et al. (2008). Role of CYP epoxygenases in A2A AR-mediated relaxation using A2A AR-null and wild-type mice. Am. J. Physiol. Heart Circ. Physiol. 295 (5), H2068–H2078. doi: 10.1152/ajpheart.01333.2007
Nayeem, M. A., Ponnoth, D. S., Boegehold, M. A., Zeldin, D. C., Falck, J. R., Mustafa, S. J. (2009). High-salt diet enhances mouse aortic relaxation through adenosine A2A receptor via CYP epoxygenases. Am. J. Physiol. Regul. Integr. Comp. Physiol. 296 (3), R567–R574. doi: 10.1152/ajpregu.90798.2008
Nayeem, M. A., Zeldin, D. C., Boegehold, M. A., Morisseau, C., Marowsky, A., Ponnoth, D. S., et al. (2010). Modulation by salt intake of the vascular response mediated through adenosine A(2A) receptor: role of CYP epoxygenase and soluble epoxide hydrolase. Am. J. Physiol. Regul. Integr. Comp. Physiol. 299 (1), R325–R333. doi: 10.1152/ajpregu.00823.2009
Nayeem, M. A., Zeldin, D. C., Boegehold, M. A., Falck, J. R. (2011). Salt modulates vascular response through adenosine A(2A) receptor in eNOS-null mice: role of CYP450 epoxygenase and soluble epoxide hydrolase. Mol. Cell Biochem. 350 (1-2), 101–111. doi: 10.1007/s11010-010-0686-0
Nayeem, M. A., Pradhan, I., Mustafa, S. J., Morisseau, C., Falck, J. R., Zeldin, D. C. (2013). Adenosine A2A receptor modulates vascular response in soluble epoxide hydrolase-null mice through CYP-epoxygenases and PPARgamma. Am. J. Physiol. Regul. Integr. Comp. Physiol. 304 (1), R23–R32. doi: 10.1152/ajpregu.00213.2012
Node, K., Huo, Y., Ruan, X., Yang, B., Spiecker, M., Ley, K., et al. (1999). Anti-inflammatory properties of cytochrome P450 epoxygenase-derived eicosanoids. Science 285 (5431), 1276–1279. doi: 10.1126/science.285.5431.1276
Olona, A., Terra, X., Ko, J. H., Grau-Bove, C., Pinent, M., Ardevol, A., et al. (2018). Epoxygenase inactivation exacerbates diet and aging-associated metabolic dysfunction resulting from impaired adipogenesis. Mol. Metab. 11, 18–32. doi: 10.1016/j.molmet.2018.03.003
Oltman, C. L., Weintraub, N. L., VanRollins, M., Dellsperger, K. C. (1998). Epoxyeicosatrienoic acids and dihydroxyeicosatrienoic acids are potent vasodilators in the canine coronary microcirculation. Circ. Res. 83 (9), 932–939. doi: 10.1161/01.RES.83.9.932
Ponnoth, D. S., Nayeem, M. A., Kunduri, S. S., Tilley, S. L., Zeldin, D. C., Ledent, C., et al. (2012a). Role of omega-hydroxylase in adenosine-mediated aortic response through MAP kinase using A2A-receptor knockout mice. Am. J. Physiol. Regul. Integr. Comp. Physiol. 302 (4), R400–R408. doi: 10.1152/ajpregu.00481.2011
Ponnoth, D. S., Nayeem, M. A., Tilley, S. L., Ledent, C., Mustafa, S. J. (2012b). CYP-epoxygenases contribute to A2A receptor-mediated aortic relaxation via sarcolemmal KATP channels. Am. J. Physiol. Regul. Integr. Comp. Physiol. 303 (10), R1003–R1010. doi: 10.1152/ajpregu.00335.2012
Pradhan, I., Zeldin, D. C., Ledent, C., Mustafa, J. S., Falck, J. R., Nayeem, M. A. (2014). High salt diet exacerbates vascular contraction in the absence of adenosine A(2)A receptor. J. Cardiovasc. Pharmacol. 63 (5), 385–394. doi: 10.1097/FJC.0000000000000058
Pradhan, I., Ledent, C., Mustafa, S. J., Morisseau, C., Nayeem, M. A. (2015). High salt diet modulates vascular response in A2AAR (+/+) and A 2AAR (-/-) mice: role of sEH, PPARgamma, and K ATP channels. Mol. Cell Biochem. 404 (1-2), 87–96. doi: 10.1007/s11010-015-2368-4
Rosolowsky, M., Campbell, W. B. (1996). Synthesis of hydroxyeicosatetraenoic (HETEs) and epoxyeicosatrienoic acids (EETs) by cultured bovine coronary artery endothelial cells. Biochim. Biophys. Acta 1299 (2), 267–277. doi: 10.1016/0005-2760(95)00216-2
Schwartzman, M. L., da Silva, J. L., Lin, F., Nishimura, M., Abraham, N. G. (1996). Cytochrome P450 4A expression and arachidonic acid omega-hydroxylation in the kidney of the spontaneously hypertensive rat. Nephron 73 (4), 652–663. doi: 10.1159/000189154
Simon, G., Abraham, G., Cserep., G. (1995). Pressor and subpressor angiotensin II administration. Two experimental models of hypertension. Am. J. Hypertens. 8 (6), 645–650. doi: 10.1016/0895-7061(95)00047-S
Wang, H., Lin, L., Jiang, J., Wang, Y., Lu, Z. Y., Bradbury, J. A., et al. (2003). Up-regulation of endothelial nitric-oxide synthase by endothelium-derived hyperpolarizing factor involves mitogen-activated protein kinase and protein kinase C signaling pathways. J. Pharmacol. Exp. Ther. 307 (2), 753–764. doi: 10.1124/jpet.103.052787
Wang, C. P., Hung, W. C., Yu, T. H., Chiu, C. A., Lu, L. F., Chung, F. M., et al. (2010). Genetic variation in the G-50T polymorphism of the cytochrome P450 epoxygenase CYP2J2 gene and the risk of younger onset type 2 diabetes among Chinese population: potential interaction with body mass index and family history. Exp. Clin. Endocrinol. Diabetes 118 (6), 346–352. doi: 10.1055/s-0029-1243604
Xu, Y., Ding, H., Peng, J., Cui, G., Liu, L., Cianflone, K., et al. (2011). Association between polymorphisms of CYP2J2 and EPHX2 genes and risk of coronary artery disease. Pharmacogenet. Genomics 21 (8), 489–494. doi: 10.1097/FPC.0b013e3283485eb2
Yadav, V. R., Nayeem, M. A., Tilley, S. L., Mustafa, S. J. (2015). Angiotensin II stimulation alters vasomotor response to adenosine in mouse mesenteric artery: role for A1 and A2B adenosine receptors. Br. J. Pharmacol. 172 (20), 4959–4969. doi: 10.1111/bph.13265
Yadav, V. R., Hong, K. L., Zeldin, D. C., Nayeem, M. A. (2016). Vascular endothelial over-expression of soluble epoxide hydrolase (Tie2-sEH) enhances adenosine A1 receptor-dependent contraction in mouse mesenteric arteries: role of ATP-sensitive K+ channels. Mol. Cell Biochem. 422 (1-2), 197–206. doi: 10.1007/s11010-016-2821-z
Yang, B., Graham, L., Dikalov, S., Mason, R. P., Falck, J. R., Liao, J. K., et al. (2001). Overexpression of cytochrome P450 CYP2J2 protects against hypoxia-reoxygenation injury in cultured bovine aortic endothelial cells. Mol. Pharmacol. 60 (2), 310–320. doi: 10.1124/mol.60.2.310
Yu, Z., Huse, L. M., Adler, P., Graham, L., Ma, J., Zeldin, D. C., et al. (2000). Increased CYP2J expression and epoxyeicosatrienoic acid formation in spontaneously hypertensive rat kidney. Mol. Pharmacol. 57 (5), 1011–1020. doi: 12933/821210
Zeldin, D. C., Liao, J. K. (2000). Reply: cytochrome P450-derived eicosanoids and the vascular wall. Trends Pharmacol. Sci. 21 (4), 127–128. doi: 10.1016/S0165-6147(00)01454-1
Zeldin, D. C., Wohlford-Lenane, C., Chulada, P., Bradbury, J. A., Scarborough, P. E., Roggli, V., et al. (2001). Airway inflammation and responsiveness in prostaglandin H synthase-deficient mice exposed to bacterial lipopolysaccharide. Am. J. Respir. Cell Mol. Biol. 25 (4), 457–465. doi: 10.1165/ajrcmb.25.4.4505
Keywords: CYP-epoxygenases, acetylcholine, adenosine, adenosine A2A receptor, angiotensin-II, relaxation
Citation: Agba S, Hanif A, Edin ML, Zeldin DC and Nayeem MA (2020) Cyp2j5-Gene Deletion Affects on Acetylcholine and Adenosine-Induced Relaxation in Mice: Role of Angiotensin-II and CYP-Epoxygenase Inhibitor. Front. Pharmacol. 11:27. doi: 10.3389/fphar.2020.00027
Received: 02 December 2019; Accepted: 10 January 2020;
Published: 05 February 2020.
Edited by:
Apostolos Zarros, University of Glasgow, United KingdomReviewed by:
Ahmed Nadeem, King Saud University, Saudi ArabiaAyman Ouda El-Kadi, University of Alberta, Canada
Zhichao Zhou, Karolinska Institutet (KI), Sweden
Copyright © 2020 Agba, Hanif, Edin, Zeldin and Nayeem. This is an open-access article distributed under the terms of the Creative Commons Attribution License (CC BY). The use, distribution or reproduction in other forums is permitted, provided the original author(s) and the copyright owner(s) are credited and that the original publication in this journal is cited, in accordance with accepted academic practice. No use, distribution or reproduction is permitted which does not comply with these terms.
*Correspondence: Mohammed A. Nayeem, mnayeem@hsc.wvu.edu