- 1Centre for Research on Environmental Ecology and Fish Nutrition (CREEFN) of the Ministry of Agriculture, Shanghai Ocean University, Shanghai, China
- 2Key Laboratory of Freshwater Aquatic Genetic Resources, Ministry of Agriculture, Shanghai, China
- 3National Demonstration Center for Experimental Fisheries Science Education, Shanghai Ocean University, Shanghai, China
- 4Editorial Office, Journal of Shanghai Ocean University, Shanghai Ocean University, Shanghai, China
Tannin, an antinutritional component of plant proteins was fed to grass carp (Ctenopharyngodon idellus, 8. 18 ± 0.81 g) for 8 weeks to investigate their tolerance levels. Semi-purified diets (T0, T1, T2, and T3) with varying levels of hydrolysable tannin (0, 0.75, 1.25, and 1.75% respectively) were used. No significant difference was obtained in weight gain, while feed conversion ratio of T0 was significantly lower than T2. Muscle protein content of T0 and T3 were significantly higher than T2, while lipid content of T0 was significantly higher than other groups. Muscle and hepatic glycogen in T0 were significantly lower than other groups. Muscle saturated fatty acids in T3 were significantly higher than T0, and lowest in T1 and T2, while the poly-unsaturated fatty acids in T1 and T2 were higher than T0 and lowest in T3. Significant increases were obtained in trypsin and amylase activities as tannin levels increased, the lipase activity of T0 and T1 was significantly higher than T2 and T3. Activities of hepatic alanine aminotransferase and aspartate aminotransferase decreased with increasing tannin level. The total protein in serum of T2 was significantly higher than T0 and T1 and lowest in T3, whereas globulin of T2 was significantly higher than T0 and T3 and lowest in T1, while albumin of T1 was significantly higher than other groups. Urea nitrogen of T0 was significantly higher than other groups, triglyceride and total cholesterol significantly increased with tannin level and decreased in T3, significant decreases were obtained in low-density lipoprotein cholesterol and high-density lipoprotein cholesterol in T3. mRNA expression of intestinal TOR was significantly upregulated as dietary tannin increased. In hepatopancreas, the expression of glucokinase in T1 was significantly higher than T2, and lowest in T0 and T3, pyruvate kinase in T2 was significantly higher than T0 and T1 and lowest T3. The expression of lipoprotein lipase upregulated as tannin level and downregulated in T3, and fatty acid synthase downregulated as tannin level. In conclusion, grass carp could tolerate 1.75% dietary tannin without influencing growth. However, 1.25% tannin impaired digestion and metabolism of protein, decreased the deposition of lipid and promoted utilization of carbohydrate.
Introduction
Fishmeal is an excellent macro-ingredient in aquatic feeds. However, the high cost, increasing demands and supply fluctuations of fishmeal have seriously restricted the sustainable development of aquaculture (1). Therefore, a steady supply and relatively nutrition-balanced products have considerably been expanded to meet the demand for aquatic feeds (2, 3).
Plant proteins such as rapeseed meal (RM) have been widely used as fishmeal substitutes in aquatic feeds (4, 5). However, high level of dietary RM induces poor growth performance, low nutrition digestibility, and stress response of fish due to its anti-nutritional factors (ANFs), such as phytic acid, glucosinolate, and tannin (6, 7). Liu et al. (8) reported that, 4.7 g/kg phytic acid decreased the apparent digestibility and utilization of amino acids and minerals of grass carp (Ctenopharyngodon idellus). Burel et al. (9) suggested that, 26 μmol/g glucosinolate disturb the thyroid hormone and decrease growth performance in rainbow trout (Oncorhynchus mykiss). However, limited information is available about the influence and underlying mechanism of dietary tannin on fish.
Tannins are polyphenolic substances with various chemical structures, which are generally divided into hydrolysable and condensed tannins (10). Tannins exist in plant feed ingredients widely. There are 52 mg/kg (wet matter) total tannin in Pomegranate (Punica granatum L.), 0.13–1.69 mg/g (DM) total tannin in broad bean, 6–9 mg/g (DM) total tannin in alfalfa cultivars, 1.2–5.3 mg/g (DM) total tannin in soybean meal, 0.7% (DM) hydrolysable tannin in dehulled rapeseed meal, and 1.2–3.7% total tannin in rapeseed meal (11–18). Both condensed tannin and hydrolysable tannin exist in RM. Condensed tannins are known to induce some physiological effects such as antioxidant, anti-hypertensive, as well as antimicrobial activities. Also, hydrolysable tannic could influence the expression of some biological activities including antimutagenic, anticancer, and antioxidant properties (19). Both hydrolysable and condensed tannins could bind with feed components like proteins, vitamin, and minerals to influence digestion (20). Previous study on rats showed that, tannin decreased the digestibility of protein and lipid, and the fermentability of undigestible protein was affected (21). Another study on rats suggested tannin decreased α-amylase activity as well as in digestibility of available carbohydrates (22). Ge et al. (23) demonstrated that persimmon tannin could effectively reduce the cellular cholesterol levels in both HepG2 and Caco-2 cells via inhibiting the cholesterol accumulation-related genes.
Hydrolysable tannins are widely explored in mammal animals. In a study of pigs, a linear decrease in feed conversion ratio and daily body weight gain was observed with increasing dietary tannic level (24). However, in the studies of ruminant, 71.3 g/kg total tannin improved the body weight gain and feed conversion ratio in Holstein bulls (25). This may be because, tannin-protein complexes are less soluble and less accessible to proteolytic enzymes in the ruminal pH, and thereby reduce the degradation of proteins in the rumen and increased the degradation of proteins in the intestine (26). These results revealed dosage-dependent and species-special effect of tannin. There are many biological differences between mammals and aquatic organisms, and few studies have been carried out to characterize the effect of tannin on aquatic animals. Therefore, the underlying mechanism will be of great significance for aquatic animals.
Prusty et al. (27) reported that, Indian major carp (Labeo rohita) could tolerate 2% dietary hydrolysable tannin, and Buyukcapar et al. (20) showed Nile Tilapia (Oreochromis niloticus) could tolerate 0.5% dietary hydrolysable tannin. These studies focus on omnivorous fish. Herbivorous fish have been noted to tolerate higher quantity of plant proteins and ANFs, and as such makes it the best choice in further investigations.
Grass carp is a typical herbivorous euryhaline fish that has been largely cultured in East Asia. Previous studies suggested that grass carp could tolerate 35% dietary RM, perhaps it is an excellent model with strong tolerance to tannin (28, 29).
The present study characterized the biological indices of grass carp fed diets with graded levels of hydrolysable tannin supplementation, aimed to evaluate its impact on growth and nutrition metabolism of grass carp, so as to better understand the fundamental mechanism of antinutritional effects induced by hydrolysable tannin and provide theoretical evidence in rapeseed meal utilization.
Materials and Methods
Experimental Fish and Environmental Conditions
Grass carp with an average initial weight of 8.18 ± 0.81 g were obtained from Huzhou Nanxun Honghao Fisheries and transported to Shanghai Ocean University culture facility located at Binhai, Shanghai, China.
Fish were randomly distributed to 16 indoor net cages with volumes of 2.5 cubic meters each and 70–80 cm water depth fixed in indoor tanks; 20 fish per cage, randomly divided into four groups with four replicates. The fish were fed with commercial diets for a one-week acclimatization period.
Environmental and water quality indicators were monitored daily over the course of the experiment. The tanks were supplied with filtered pond water and uninterrupted aeration to maintain an appropriate level of dissolved oxygen (>5 mg/L), ammonium nitrogen (<0.6 mg/L), and the water temperature varied 24–32°C.
Diets and Feeding
The feeding trial lasted for 8 weeks and all fish were fed to apparent satiation three times daily (7:00 am, 12:00 pm, and 17:00 pm). Uneaten diet was collected 30 min after feeding, dried and weighed for correction of the feed intake. Four isonitrogenous (crude protein 31.01%) and isoenergetic (gross energy 17.34–17.63 MJ/kg) semi-purified diets were formulated. These diets contained 0% (T0), 0.75% (T1), 1.25% (T2), and 1.75% (T3) hydrolysable tannin supplementation, respectively, in which the major protein sources were casein and gelatin, and the major lipid source was soybean oil (Table 1).
All ingredients were grounded into fine powder through a 60-mesh sieve, weighed according to the formula and thoroughly mixed with soybean oil, then water was added and pelleted with an experimental feed mill, dried for about 12 h in a ventilated oven at 40°C. After drying, the diets were broken and sieved into proper pellet size (1.5 mm in diameter), and well-stored at a cool dry well-ventilated place.
Sample Collection
At the end of the 8-week feeding period, all fish in each cage were counted and weighed. Seven fish were randomly pooled from each cage (28 fish/group), anesthetized with eugenol solution (100 ppm) and killed via sharp blow to the cranium prior to dissection. After body weight and body length was measured, the exterior of the individuals was wiped clean and the abdomen was opened at the ventral mid line to separate and weigh hepatopancreas, intestine and viscera. Weight gain (WG), feed efficiency ratio (FER), feed intake (FI), relative intestine weight, relative hepatopancreas weight, and relative viscera weight were calculated by the following formulae:
Blood samples from seven fish each cage (28 fish/ group) were collected from the caudal vein, centrifugated at 4,000 × g for 10 min at 4°C, to obtain the serum and stored at −20°C for further analysis. Intestine, hepatopancreas and muscle were weighed and collected from the selected fish, frozen on ice prior to storage at −20°C.
Following the feeding period, other three fish were sampled from each group randomly, anesthetized and killed as above. The intestine and hepatopancreas were collected from the selected fish. For RNA extraction, the tissues were fixed in 1.5 ml of RNAse-free centrifuge tubes and then stored at −80°C. The expression of TOR mRNA (target of rapamycin), GK mRNA (glucokinase), PK mRNA (pyruvate kinase), CTP1 mRNA (Carnitine palmitoyl transferase), FAS mRNA (fatty acid synthase), and LPL mRNA (lipoprotein lipase) in different tissues were determined.
Muscle Composition Analysis
Muscle were dried at 70°C for 2 h and then to a constant weight at 105°C to determine the moisture content; protein was determined by measuring nitrogen (N × 6.25) using the Kjeldahl method, lipid by ether extraction using chloroform-methanol extraction (30) and crude ash by combustion at 550°C. Muscle glycogen were measured using specific analytical procedures and commercially obtained kits for fish (Jiancheng Bioengineering Institute, Nanjing, China). For amino acids (except for methionine), the muscle was freeze-dried, and then hydrolyzed with 6 N HCl at 110°C for 24 h, and analyzed by amino acid analyzer (Biochrom Ltd, Cambridge, Science Park, England). The lipid in muscle was esterified to fatty acid methyl esters (FAMEs) with 14% BF3 in methanol (31) and extracted with hexane, then fatty acids profile was detected in duplicated on an HP6890 gas chromatograph according to the method described by Huang et al. (32), the statistical analysis of fatty acid referring to the method of Li et al. (33).
Digestive Enzymes Activities and Biochemical Parameters
Hepatopancreas and intestine samples were homogenized by adding sterile 0.85% saline solution to prepare 10% (W:V) homogenates, and then centrifuged at 4,000 × g for 10 min at 4°C. Supernatants were used to analyze the activities of digestive enzyme and biochemical parameters in 12 h.
Serum parameters like total protein (TP), albumin (ALB), total cholesterol (TC), triglyceride, low density lipoprotein cholesterol (LDL-C), high density lipoprotein cholesterol (HDL-C), and urea nitrogen (UN) and activities of alanine aminotransferase (ALT), aspartate aminotransferase (AST) and hepatic glycogen of hepatopancreas were measured using specific analytical procedures and commercially obtained kits (Jiancheng Bioengineering Institute, Nanjing, China).
The intestinal activity of trypsin was analyzed following the method of Natalia et al. (34). The activity of alpha-amylase was measured according to Worthington (35). The activity of lipase was assayed based on measurement of fatty acids released due to enzymatic hydrolysis of triglycerides in a stabilized emulsion of olive oil (36) using specific analytical procedures and commercially available kits (Jiancheng Bioengineering Institute, Nanjing, China). All enzyme assays were performed in quadruplicate.
Isolation of Total RNA
Total RNA was extracted via the Trizol Reagent, based on the manufacturer's instructions (Invitrogen, USA). The RNA concentration was determined via absorbance at 260 nm with a UV spectrophotometer (Thermo Fisher Scientific, USA). We assessed the purity by measuring the RNA content and the OD260/OD280 ratio. The total RNA samples typically yielded 100 ng/μLRNA and an OD260/OD280 ratio between 1.8 and 2.0.
Real-Time Quantitative Reverse Transcription PCR
Based on conserved gene cDNA sequences of β-actin, TOR, LPL, FAS, CPT1, GK, and PK of grass carp, fluorescence quantitative primers (Table 2) were designed and synthesized with Primer5 software by the Jiangsu Jin Weizhi company (Jiangsu City, P.R. China). Real-time fluorescence quantitative PCR were measured via ABI7500 according to instructions using the SuperReal SYBR Green (TianGen, FP205) of the two-step RT-PCRkit. The volume of the reactions was 20 μL, in which 10 μL Goldstar PCR Master Mix (2 ×), 0.6 μL Primer, 0.4 μL Rox, 2 μL CDNA, and 7 μL dH2O were used. A fluorescent signal was collected every 0.3°C from 60 to 95°C with β-actin chosen as reference gene. The thermocycling conditions were as follows: initiated with transcript reaction at 95°C for 15 min, followed by forty cycles at 95°C for 15 s and 60°C for 30 s. Melting curve analysis was performed over a range of 50–95°C to verify that a single PCR product was generated. Both the target gene and the internal control β-actin gene were amplified from each cDNA sample under identical conditions on the same plate. Each sample was amplified in triplicate. The relative mRNA expression of genes was calculated via the 2−ΔΔCt method. We compared the relative mRNA expression levels of all experimental groups to those of the T0 group.
Data Analysis
Data were presented as means ± SD and analyzed via one-way analysis of variance (ANOVA) in SPSS version 17.0. Differences in mean values were analyzed via Duncan's multiple range test when ANOVA identified differences among groups. The level of significance was set to P < 0.05.
Result
Survival and Growth Performance
No significant (P > 0.05) difference was observed in survival rate, weight gain rate, relative hepatopancreas weight, and relative intestine weight (Table 3). The FCR and FI of T2 was higher than T1 and T3 (P > 0.05), and significantly higher than T0 (P < 0.05). The relative viscera weight of T0 was significantly (P < 0.05) higher than other groups.
Muscle Composition
No significant (P > 0.05) difference was observed in muscle moisture and ash. The protein content of T0 and T3 were significantly (P < 0.05) higher than T2 and lowest in T1(Table 4). The muscle lipid content decreased significantly (P < 0.05) with tannin level. The content of muscle glycogen in T0 was significantly (P < 0.05) lower than other groups. No significant (P > 0.05) difference was observed in the percentage of muscle essential amino acid (EAA), non-essential amino acid (NEAA), and total amino acid (TAA), in spite of marked difference on the percentage of methionine (Met) and proline (Pro) among groups (Table 5). As presented in Table 6, the percentage of muscle saturated fatty acid (SFA) in T3 was significantly (P < 0.05) higher than T1 and T2, and lowest in T3, whereas, poly-unsaturated fatty acid (PUFA) percentage in T1 and T2 were significantly (P < 0.05) higher than T0, and lowest in T3.
Digestive Enzyme Activities
Intestinal activities of trypsin and α-amylase significantly (P < 0.05) increased with dietary tannin level, while the lipase activity of T0 and T1 was significantly (P < 0.05) higher than T2 and T3 (Table 7).

Table 7. Digestive enzyme activities (Umg−1 protein) in intestine of grass carp (means ± SD, n = 4).
Hepatopancreas and Serum Biochemical Parameters
The hepatopancreas and serum biochemical parameters are presented in Tables 8, 9. In hepatopancreas, the activity of AST in T0 and T1 was significantly (P < 0.05) higher than T2 and T3, and the activity of ALT of T0 was significantly (P < 0.05) higher than T1, while lowest in T2 and T3 (P < 0.05). The hepatic glycogen content significantly (P < 0.05) increased with dietary tannin level and decreased at T3 (Table 8).
In serum, the TP content of T2 was significantly (P < 0.05) higher than T0 and T1, and lowest in T3 (Table 9). While ALB content of T1 was significantly (P < 0.05) higher than other groups, the globulin of T2 was significantly (P < 0.05) higher than T0 and T3, and lowest in T1. The UN content of T0 was significantly (P < 0.05) higher than T2 and T1, and lowest in T3. The triglyceride and TC significantly (P < 0.05) increased with dietary tannin level and then decreased in T3. The LDL-C content of T1 was significantly (P < 0.05) higher than T0, T0 is significantly (P < 0.05) higher than T2 and lowest in T3. The HDL-C content significantly (P < 0.05) decreased with dietary tannin level.
Reactions of mRNA Gene Expressions to Tannin Levels in Intestine and Hepatopancreas
The relative expression of TOR mRNA in intestine was significantly (P < 0.05) upregulated as dietary tannin increased (Figure 1).
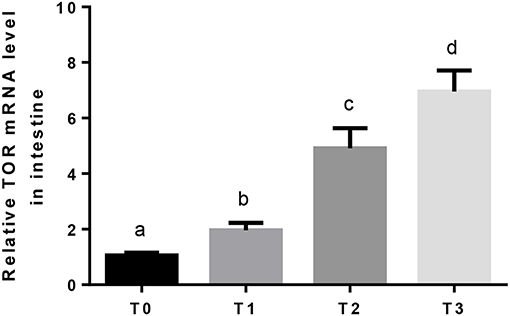
Figure 1. The relative expression of TOR mRNA in the intestine. The letters indicate the results of multiple range test, the different letters indicated significant differences (P < 0.05).
Hepatic GK mRNA in T1 was significantly (P < 0.05) higher than T2, and lowest in T0 and T3 (Figure 2). The relative expression of hepatic PK mRNA in T2 was significantly (P < 0.05) higher than T0 and T1, and lowest in T3 (Figure 3).
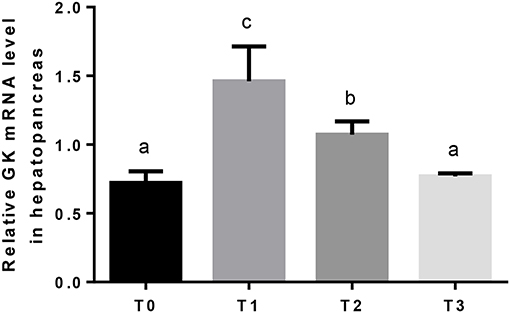
Figure 2. The relative expression of GK mRNA in the hepatopancreas. The letters indicate the results of multiple range test, the different letters indicated significant differences (P < 0.05).
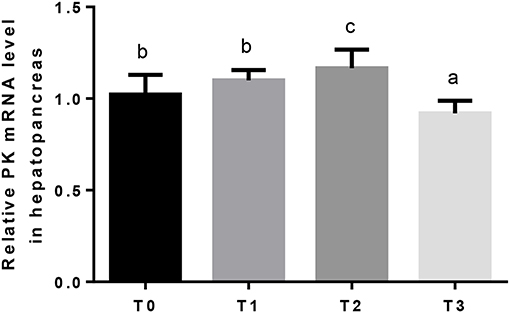
Figure 3. The relative expression of PK mRNA in the hepatopancreas. The letters indicate the results of multiple range test, the different letters indicated significant differences (P < 0.05).
The mRNA expression of hepatic LPL was significantly (P < 0.05) upregulated as tannin level and downregulated in T3 (Figure 4). The relative expression of FAS mRNA downregulated significantly (P < 0.05) as tannin level (Figure 5). No significant (P > 0.05) difference was observed in the relative expression of hepatic CPT1 mRNA (Figure 6).
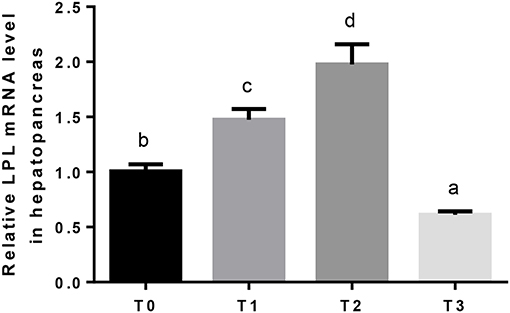
Figure 4. The relative expression of LPL mRNA in the hepatopancreas. The letters indicate the results of multiple range test, the different letters indicated significant differences (P < 0.05).
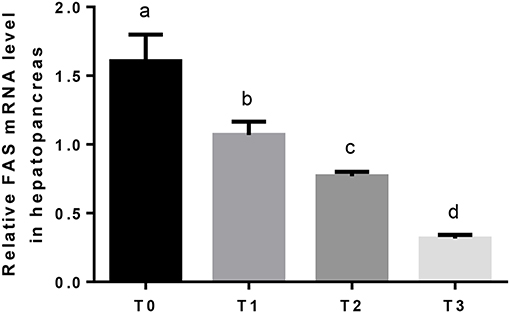
Figure 5. The relative expression of FAS mRNA in the hepatopancreas. The letters indicate the results of multiple range test, the different letters indicated significant differences (P < 0.05).

Figure 6. The relative expression of CPT1 mRNA in the hepatopancreas. The letters indicate the results of multiple range test, no letter indicated no significant differences (P > 0.05).
Discussion
The Effect of Tannin on Growth Performance
In order to protect global fish stocks and reduce costs, numerous studies on replacing fishmeal by other protein sources have been demonstrated. Tannin, as one of the ANFs, is widely present in plant products, such as rapeseed meal, seaweed, hazelnut seed, soybean, wheat, sunflower, field pea, chickpea, sorghum, and microalgal biomass (37–41). Therefore, the tolerable threshold and effect of tannin on fish should be investigated.
In the present study, no apparent negative effect was observed on growth performance, but the FI and FCR increased. Similar results were reported in the study of Hamilton (Labeo rohita) fingerlings when 2% dietary hydrolysable tannin was added in their diet (27). However, when 2.5% dietary hydrolysable tannin was added in the diet of Nile Tilapia (Oreochromis niloticus), the weight gain decreased and the FCR increased (20). Similar results were obtained when 3% dietary tannin was added in the diet of European seabass (Dicentrarchus labrax L) (42). It has been indicated that mammalian herbivores have an innate defensive mechanism against dietary polyphenolics; the existence of proline rich proteins in their saliva would bind with tannin and protect them against polyphenolics (43). It is reasonable to assume that, the tolerable threshold of dietary tannin was connected with feeding habits, and herbivorous fish could tolerate higher level of tannin without influencing the growth compare to omnivorous and carnivorous fish. Furthermore, different energy sources may be importance as well, where studies have evaluated that, carnivorous fish rely heavily on protein and lipid as energy sources, while omnivorous and herbivorous fish have a greater proclivity for utilizing plant feedstuffs and carbohydrate for energy (44). Consequently, grass carp may have strong adaptability to tannin and establish a special regulatory mechanism of growth.
The Effect of Tannin on Protein Metabolism
Protein is a major energy source for aquatic organisms, and the growth of animal is connected with nutrient deposition especially protein deposition for fish (45).
The deposition of protein is related to the balance of absorption and biosynthesis. McCurdy and March (46) reported that, tannins bind with dietary proteins and digestive enzyme, and reduce the digestibility of proteins as well as diet palatability. This is in agreement with the results from Nile tilapia (20) and European seabass (42) that, the digestibility of protein showed an inverse relationship with dietary tannin levels. However, in the present study, the activity of trypsin increased with dietary tannin levels. Tannins are known to interfere with digestive enzymes, and thereby, in order to increase digestibility, more trypsin needs to be secreted. Therefore, we put forward the hypothesis that, with the increased activity of trypsin, the digestibility of protein does not always increase. This speculation is supported by the increasing expression of TOR mRNA in intestine. TOR pathway is closely linked with protein synthesis, which could modulate protein translation, stimulate protein synthesis and regulate cellular growth, proliferation and metabolism (47, 48). In the present study, the increasing expression of TOR mRNA may promote the synthesis of digestive enzymes.
Serum TP, ALB and UN are important indicators in protein metabolism, of which UN is the main end products of protein metabolism. Low TP and ALB content indicate poor nutrition and protein metabolic status in aquatic organisms. Globulin is a crucial indictor in fish immunity, which would increase when fish is injured (49). In the present study, the TP content in serum decreased in T3, and ALB decreased in T2, which indicated that, excess dietary tannin impacted the metabolism of protein. In addition, the content of serum UN decreased and globulin increased in T2, which indicated that, the catabolism of amino acids decreased and hepatopancreas may be damaged to a certain extent (50). Pervious study demonstrated that, tannin partially inhibit the antioxidant enzymes and induct the damage of liver (51). In a study of carp (Cyprinus carpio L), when 10 mg/kg body tannin were injected, the toxicity for fish due to oxidative stress induction and antioxidant enzyme inhibition were observed (52).
AST and ALT are the main aminotransferase in hepatopancreas. The aminotransferases catabolize amino acids which are absorbed in intestine and transfer amino groups to alpha-keto acids (53). In the present study, the activities of hepatic AST and ALT reduced with dietary tannin levels, which indicated that, the biosynthesis of protein was injured. Deficient digestible amino acids derived by tannin may be an important reason and the hepatopancreas may present a tendency to be injured (53), as hydrolysable tannins are readily hydrolyzed in the intestine to small phenolic compounds that are absorbed and may cause toxicity to hepatopancreas (52, 54). In mice, a single subcutaneous injection of tannin at 700 mg/kg body weight caused a significant break down of polyribosomes in liver and inhibited the incorporation of amino acids into hepatic proteins (10). When rat and chick were fed with diet containing 1.6% tannin extract from faba beans, hydropic degeneration of hepatocytes were observed (55) and a similar result was also presented in goat (56).
The above results manifested that, when 1.25% dietary tannin was fed to grass carp, the digestion, biosynthesis, and catabolism of protein were impaired, the antinutritional function and toxicity of tannin may be responsible for these.
The Effect of Tannin on Lipid Metabolism
Lipid and digestible carbohydrate are the major non-protein energy sources in fish diets, but as a typical herbivorous fish like grass carp, excessive lipid accumulation occurs in practical culture (57, 58). Excessive lipid accumulation in fish body and tissue affects the quality in nutritional value and injure the health of fish by lipid oxidation and peroxidation (59, 60). In the present study, dietary tannin decreased muscle lipid content. Similar result was reported in European seabass and Nile Tilapia where dietary tannin was supplemented at 2 and 1.5%, respectively (20, 42). The reduced muscle lipid was probably due to lower digestibility induced by low activity of lipase (61). In the present study, the lipase activity in intestine decreased in T2 and T3. Similar result was reported in rat fed diet contained 2.5% tannin (21). Previous study reported that, because of the structure of lipase, the binding strength between the tannin and lipases was large and hard to modify in vivo, and this partly account for the low muscle lipid content and lipase activity (21, 62). The increases in muscle PUFAs percentages in T1 and T2, may be suggested to be influenced by the anti-oxidative properties of tannin (63, 64).
Hepatopancreas is the main crossing organ for exogenous and endogenous transport of lipids. FAS is the key regulatory enzymes in fatty acid synthesis (65, 66). In the present study, the relative expression of hepatic FAS downregulated with dietary tannin levels, indicating that the biosynthesis of lipid was influenced.
LDL-C transports cholesterol from liver to peripheral tissues, while HDL-C mediates the reverse transport of cholesterol from peripheral tissues to the liver, and HDL-C/LDL-C ratio is a marker of cholesterol transport (67, 68). It has been shown that tannin could reduce serum cholesterol levels (69). In a study on rats injected 30 mg/week hydrolysable tannin, serum cholesterol and triglycerides reduced, and lipogenesis was suppressed by insulin (70, 71). This may because that tannin could bind with, increase fecal excretion of bile acids and acceleration of cholesterol catabolism to a level that is greater than that of cholesterol synthesis in the liver (72–74). In the present study, however, the total cholesterol (TC) content in serum increased with the dietary tannin levels and decreased in T3, whereas HDL-C decreased, and LDL-C increased in T1 and then decreased, and the HDL-C/LDL-C ratio decreased in T1 and then increased. These indicate that, 1.25% dietary hydrolysable tannin would improve cholesterol transport in grass carp.
In general, fat accumulation results from the balance between absorbed dietary lipid, lipogenesis and lipolysis. In fish, the metabolic pathways of lipid biosynthesis and oxidation are essentially the same as those observed in mammals (75). Through oxidation, the fatty acids can be used as energy source, or stored and deposited in adipose tissues (76). LPL is considered to be a key enzyme in the lipid deposition and metabolism, which hydrolyzes triacylglycerols present in plasma lipoproteins and supplies free fatty acids for storage in adipose tissue, or for oxidation in other tissues (77–79). In the present study, the relative expression of hepatic LPL mRNA upregulated and subsequently downregulated in T3, and the serum triglyceride showed similar tendency with LPL. Carnitine palmitoyltransferase I (CPT1) is considered to be the main regulatory enzyme in long-chain fatty acid oxidation which catalyzes the conversion of fatty acid-CoAs into fatty acid-carnitines for entry into the mitochondrial matrix (80). The relative expression of CPT1mRNA did not show significant differences. These results suggest that, fish reduced lipid deposition when fed diet contained hydrolysable tannin, and the oxidation of fatty acid was not injured. 1.25% dietary tannin seems the best level to decrease the deposition of lipid and increased cholesterol transport.
The Effect of Tannin on Carbohydrate Metabolism
Compared to dietary lipid, carbohydrate is relatively cheaper and a readily available source of energy to herbivorous fish (81). Higher amylase activity in intestine and metabolic enzyme activity are important reasons (82). After carbohydrate is digested, the glycogen is mainly deposited in muscle and liver. Liver glycogen content indicates accumulation in the liver as both lipid and glycogen after conversion (83), and muscle glycogen serve as an energy reserve for that particular organ (84). In the present study, the activity of amylase increased with dietary tannin levels, and the glycogen content in hepatopancreas and muscle increased and then decreased in T3. These indicate that, dietary tannin improved the digestion and accumulation of carbohydrate.
Glycolysis is the major route of glucose metabolism in fish as in other animals (85, 86). Hepatic GK and PK are important enzymes that play crucial roles in glycolysis regulation. GK removes glucose from the blood after feeding and partakes in phosphorylation of glucose as the first step of glucose utilization, and PK catalyzes the last step of glycolysis, the conversion of phosphoenolpyruvate to pyruvate (87, 88). In the present study, when dietary tannin was supplemented, the relative expression of GK in hepatopancreas upregulated, and the relative expression of PK upregulated in T2 and then downregulated. The elevated GK and PK transcript induce an increase in glucose uptake from circulation into hepatopancreas, and increase glucose storage in hepatopancreas for subsequent energy supply. Based on above results, it was considered that, 0.75 and 1.25% dietary tannin could improve the digestion and glycolysis of carbohydrate, and these may spare the consumption of protein and lipid for energy (89, 90).
In conclusion, grass carp could tolerate 1.75% dietary hydrolysable tannin without influencing growth. However, 1.25% dietary tannin impaired the digestion and metabolism of protein. But the utilization of carbohydrate was promoted, the digestion and biosynthesis of lipid decreased, and lipolysis increased to decrease the deposition of lipid and increase cholesterol transport. Furthermore, the present study provides a theoretical reference to the use of tannin-rich plant sources in aquatic feed. From this perspective of tannin, dietary rapeseed meal in grass carp should not exceed 50%, and dietary broad bean should not exceed 75%.
Data Availability Statement
All datasets generated for this study are included in the article/supplementary material.
Ethics Statement
All procedures were carried out according to national and institutional regulations on the care and use of experimental animals. The experimental handling and treatment of experimental fish were conducted in accordance with the regulations made by the Institutional Animal Care and Use Committee (IACUC), Shanghai Ocean University (SHOU), and this study was approved by the IACUC of SHOU, Shanghai, China.
Author Contributions
Six authors are justifiably credited with authorship. XH, JY, and PC conceived the study and designed the experiments. JY, GZ, and ZH contributed to performing the experiment. JY and PC did the data analysis and wrote the paper. XH and AA revised the manuscript.
Funding
This study was supported by the Key Laboratory of Freshwater Aquatic Genetic Resources, the Ministry of Agriculture, the Shanghai Ocean University, Shanghai, China.
Conflict of Interest
The authors declare that the research was conducted in the absence of any commercial or financial relationships that could be construed as a potential conflict of interest.
References
1. Olsen RL, Hasan MR. A limited supply of fishmeal: impact on future increases in global aquaculture production. Trends Food Sci Technol. (2012) 27:120–8. doi: 10.1016/j.tifs.2012.06.003
2. Hardy RW. Utilization of plant proteins in fish diets: effects of global demand and supplies of fishmeal. Aquac Res. (2010) 41:770–6. doi: 10.1111/j.1365-2109.2009.02349.x
3. Tacon AGJ, Metian M. Feed matters: satisfying the feed demand of aquaculture. Rev Fish Sci Aquac. (2015) 23:1–10. doi: 10.1080/23308249.2014.987209
4. Ngo DT, Wade NM, Pirozzi I, Glencross BD. Effects of canola meal on growth, feed utilisation, plasma biochemistry, histology of digestive organs and hepatic gene expression of barramundi (Asian seabass; Lates calcarifer). Aquaculture. (2016) 464:95–105. doi: 10.1016/j.aquaculture.2016.06.020
5. Luo Z, Li XD, Wang WM, Tan XY, Liu X. Partial replacement of fish meal by a mixture of soybean meal and rapeseed meal in practical diets for juvenile Chinese mitten crab Eriocheir sinensis: effects on growth performance and in vivo digestibility. Aquac Res. (2011) 42:1615–22. doi: 10.1111/j.1365-2109.2010.02751.x
6. Luo Y, Ai Q, Mai K, Zhang W, Xu W, Zhang Y. Effects of dietary rapeseed meal on growth performance, digestion and protein metabolism in relation to gene expression of juvenile cobia (Rachycentron canadum). Aquaculture. (2012) 368–369:109–16. doi: 10.1016/j.aquaculture.2012.09.013
7. Bu XY, Wang YY, Chen FY, Tang BB, Luo CZ, Wang Y, et al. An evaluation of replacing fishmeal with rapeseed meal in the diet of Pseudobagrus ussuriensis: growth, feed utilization, nonspecific immunity, and growth-related gene expression. J World Aquac Soc. (2018) 6:1068–80. doi: 10.1111/jwas.12470
8. Liu LW, Liang XF, Li J, Yuan XC, Fang JG. Effects of supplemental phytic acid on the apparent digestibility and utilization of dietary amino acids and minerals in juvenile grass carp (Ctenopharyngodon idellus). Aquac Nutr. (2017) 24:850–7. doi: 10.1111/anu.12614
9. Burel C, Boujard T, Kaushik SJ, Boeuf G, Mol KA, Van der Geyten S, et al. Effects of rapeseed meal-glucosinolates on thyroid metabolism and feed utilization in rainbow trout. Gen Comp Endocrinol. (2001) 124:343–58. doi: 10.1006/gcen.2001.7723
10. Chung KT, Wei CI, Johnson MG. Are tannins a double-edged sword in biology and health? Trends Food Sci Technol. (1998) 9:168–75. doi: 10.1016/S0924-2244(98)00028-4
11. Muhacir-Güzel N, Türkyilmaz M, Yemiş O, Tagi S, Özkan M. Changes in hydrolysable and condensed tannins of pomegranate (Punica granatum L. cv. Hicaznar) juices from sacs and whole fruits during production and their relation with antioxidant activity. LWT Food Sci Technol. (2014) 59:933–40. doi: 10.1016/j.lwt.2014.07.025
13. Wu DG, Wang SS, Liu CZ, Hu GX, Du JL, He CG. Effects of herbivore stress by Acyrthosiphon pisum on the contents of tannin and physiological activity in different alfalfa cultivars. Acta Agrectir Sinica. (2011) 19:351–5. doi: 10.3724/SP.J.1011.2011.00353
14. Xiao Z, Wu M, Shen D, Xiao R. Analysis on the effect of extrusion on tannin content in rapeseed meal. Trans Chin Soc Agric Mach. (2008) 39:85–9.
15. Yapar Z, Clandinin DR. Effect of tannins in rapeseed meal on its nutritional value for chicks. Poult Sci. (1972) 51:222–8. doi: 10.3382/ps.0510222
16. Fenwick GR, Pearson AW, Greenwood NM, Butler EJ. Rapeseed meal tannins and egg taint. Anim Feed Sci Technol. (1981) 6:421–31. doi: 10.1016/0377-8401(81)90020-1
17. Li D, Qiu J, Gu X, Li JG, Yang SM. Analysis of the difference of tannin content in soybean seed. Feed Ind. (2016) 1:38–41. doi: 10.13302/j.cnki.fi.2016.01.008
18. Li H, Kang Y. Research progress on metabolic pathway of tannin in faba bean during germination and its inactivation technologies. Food Nutr China. (2013) 19:61–5. doi: 10.3969/j.issn.1006-9577.2013.09.015
19. Romani A, Ieri F, Turchetti B, Mulinacci N, Vincieri FF, Buzzini P. Analysis of condensed and hydrolysable tannins from commercial plant extracts. J Pharm Biomed Anal. (2006) 41:415–20. doi: 10.1016/j.jpba.2005.11.031
20. Buyukcapar HM, Atalay A, Kamalak A. Growth performance of Nile Tilapia (Oreochromis niloticus) fed with diets containing different levels of hydrolysable and condensed tannin. J Agric Sci Technol. (2011) 13:1045–51. doi: 10.1007/s10460-011-9341-y
21. Nyman ME, BjORck IM. In vivo effects of phytic acid and polyphenols on the bioavailability of polysaccharides and other nutrients. J Food Sci. (1989) 54:1332–5. doi: 10.1111/j.1365-2621.1989.tb05985.x
22. Moseley G. The effect of diets containing field beans of high or low polyphenolic content on the activity of digestive enzymes in the intestines of rats. J Sci Food Agric. (2010) 31:255–9. doi: 10.1002/jsfa.2740310307
23. Ge Z, Zhu W, Peng J, Deng X, Li C. Persimmon tannin regulates the expression of genes critical for cholesterol absorption and cholesterol efflux by LXRα independent pathway. J Funct Foods. (2016) 23:283–93. doi: 10.1016/j.jff.2016.02.033
24. Lee SH, Shinde PL, Choi JY, Kwon IK, Lee JK, Pak SI, et al. Effects of tannic acid supplementation on growth performance, blood hematology, iron status and faecal microflora in weanling pigs. Livest Sci. (2010) 131:281–6. doi: 10.1016/j.livsci.2010.04.013
25. Jolazadeh AR, Dehghan-Banadaky M, Rezayazdi K. Effects of soybean meal treated with t annins extracted from pistachio hulls on performance, ruminal fermentation, blood metabolites and nutrient digestion of Holstein bulls. Anim Feed Sci Technol. (2015) 203:33–40. doi: 10.1016/j.anifeedsci.2015.02.005
26. Rivera-Méndez C, Plascencia A, Torrentera N, Zinn RA. Effect of level and source of supplemental tannin on growth performance of steers during the late finishing phase. J Appl Anim Res. (2016) 45:199–203. doi: 10.1080/09712119.2016.1141776
27. Prusty AK, Sahu NP, Pal AK, Reddy AK, Kuma S. Effect of dietary tannin on growth and haemato- immunological parameters of Labeo rohita (Hamilton) fingerlings. Anim Feed Sci Technol. (2007) 136:96–108. doi: 10.1016/j.anifeedsci.2006.08.023
28. Tan Q, Liu Q, Chen X, Wang M, Wu Z. Growth performance, biochemical indices and hepatopancreatic function of grass carp, Ctenopharyngodon idellus, would be impaired by dietary rapeseed meal. Aquaculture. (2013) 414–415:119–26. doi: 10.1016/j.aquaculture.2013.07.036
29. Suya J. Effects of feeding four kinds of rapeseed meal on growth performance of grass carp (Ctenopharyngodon idella). Chin J Anim Nutr. (2011) 23:349–56. doi: 10.1016/S1671-2927(11)60313-1
30. Wang SM, Chen SL, Cui YB. On the procedures of chloroform-methanol extraction for the determination of lipid content of fish samples. Acta Hydrobiol Sin. (1993) 17:193–6.
31. Morrison WR. Preparation of fatty acid methyl esters and dimethyl acetals from lipid with boron florides-methanol. J Lipid Res. (1964) 5:600–8.
32. Huang X, Yin Y, Shi Z, Li W, Zhou H, Lv W. Lipid content and fatty acid composition in wild-caught silver pomfret (Pampus argenteus) broodstocks: effects on gonad development. Aquaculture. (2010) 310:192–9. doi: 10.1016/j.aquaculture.2010.10.015
33. Li S, Wen W, Gong X, Huang X, Chen N. Variation of lipids and fatty acids composition in the tissues of wild devil stinger (Inimicus japonicas) during sexual maturation. Aquac Fish. (2018) 3:115–21. doi: 10.1016/j.aaf.2018.05.004
34. Natalia Y, Hashim R, Ali A, Chong A. Characterization of digestive enzymes in a carnivorous ornamental fish, the Asian bony tongue Scleropages formosus (Osteoglossidae). Aquaculture. (2004) 233:305–20. doi: 10.1016/j.aquaculture.2003.08.012
35. Worthington. Worthington Enzyme Manual: Enzymes and Related Biochemicals. Lakewood, NJ: Worthington Biochemical Corporation (1993).
36. Borlongan IG. Studies on the digestive lipases of milkfish, Chanos chanos. Aquaculture. (1990) 89:315–25. doi: 10.1016/0044-8486(90)90135-A
37. Valente LMP, Gouveia A, Rema P, Matos J, Gomes EF, Pinto IS. Evaluation of three seaweeds Gracilaria bursa-pastoris, Ulva rigida and Gracilaria cornea as dietary ingredients in European sea bass (Dicentrarchus labrax.) juveniles. Aquaculture. (2006) 252:85–91. doi: 10.1016/j.aquaculture.2005.11.052
38. Emre Y, Sevgili H, Sanli M. A preliminary study on the utilization of hazelnut meal as a substitute for fish meal in diets of European sea bass (Dicentrarchus labrax L.). Aquac Res. (2010) 39:324–8. doi: 10.1111/j.1365-2109.2007.01881.x
39. Abdalla I. Effect of radiation process followed by fermentation and/or cooking on nutritional quality of high tannin sorghum cultivar. Am J Exp Agricult. 2014 4:651–64. doi: 10.9734/AJEA/2014/7978
40. Adamidou S, Nengas I, Henry M, Grigorakis K, Rigos G, Nikolopoulou D, et al. Growth, feed utilization, health and organoleptic characteristics of European seabass (Dicentrarchus labrax) fed extruded diets including low and high levels of three different legumes. Aquaculture. (2009) 293:260–71. doi: 10.1016/j.aquaculture.2009.04.045
41. Messina M, Piccolo G, Tulli F, Messina CM, Cardinaletti G, Tibaldi E. Lipid composition and metabolism of European sea bass (Dicentrarchus labrax L.) fed diets containing wheat gluten and legume meals as substitutes for fish meal. Aquaculture. (2013) 376–379:6–14. doi: 10.1016/j.aquaculture.2012.11.005
42. Omnes MH, Le Goasduff J, Le Delliou H, Le Bayon N, Quazuguel P, Robin JH. Effects of dietary tannin on growth, feed utilization and digestibility, and carcass composition in juvenile European seabass (Dicentrarchus labrax, L.). Aquac Rep. (2017) 6:21–7. doi: 10.1016/j.aqrep.2017.01.004
43. Mole S, Butler LG, Iason G. Defense against dietary tannin in herbivores: a survey for proline rich salivary proteins in mammals. Biochem Syst Ecol. (1990) 18:287–93. doi: 10.1016/0305-1978(90)90073-O
44. Medaka A. Expanding the utilization of sustainable plant products in aquafeeds. Aquac Res. (2010) 38:551–79. doi: 10.1111/j.1365-2109.2007.01704.x
45. Kim N, Son SH, Maeng JS. Effects of dietary non-protein energy source levels on growth performance, body composition and lipid metabolism in herbivorous grass carp (Ctenopharyngodon idellaVal.). Aquac Res. (2015) 46:1197–208. doi: 10.1111/are.12275
46. McCurdy SM, March BE. Processing of canola meal for incorporation in trout and salmon diets. J AOCS. (1992) 69:213–20. doi: 10.1007/BF02635889
47. Holz MK, Ballif BA, Gygi SP, Blenis J. mTOR and S6K1 mediate assembly of the translation preinitiation complex through dynamic protein interchange and ordered phosphorylation events. Cell. (2005) 123:569–80. doi: 10.1016/j.cell.2005.10.024
48. Seiliez I, Gabillard JC, Skiba-Cassy S, Garcia-Serrana D, Gutiérrez J, Kaushik S, et al. An in vivo and in vitro assessment of TOR signaling cascade in rainbow trout (Oncorhynchus mykiss). AJP Regulat Integr Comp Physiol. (2008) 295:329–35. doi: 10.1152/ajpregu.00146.2008
49. Qi Q, Liu X, Liu H, Zhang Y, Zhang Y, Zhang H, et al. Effect of Chinese medicinal herb compound on serum biochemical indicator of Siberian sturgeon (Acipenser baeri). J Northeast Agric Univ. (2012) 12:134–8. doi: 10.1007/s11783-011-0280-z
50. Urschel KL, Shoveller AK, Uwiera RR, Pencharz PB, Ball RO. citrulline is an effective arginine precursor in enterally fed neonatal piglets. J Nutr. (2006) 136:1806–13. doi: 10.1093/jn/136.7.1806
51. Holliman A. Acorn poisoning in ruminants. Vet Rec. (1985) 116:546–52. doi: 10.1136/vr.116.20.546
52. Varanka Z, Rojik I, Varanka I, Nemcsók J, Abrahám M. Biochemical and morphological changes in carp (Cyprinus carpio L.) liver following exposure to copper sulfate and tannic acid. Comp Biochem Physiol Part C Toxicol Pharmacol. (2001) 128:467–77. doi: 10.1016/S1532-0456(01)00166-1
53. Cheng Z, Ai Q, Mai K, Xu W, Ma H, Li Y, et al. Effects of dietary canola meal on growth performance, digestion and metabolism of Japanese seabass, Lateolabrax japonicus. Aquaculture. (2010) 305:102–8. doi: 10.1016/j.aquaculture.2010.03.031
54. Singleton VL, Kratzer FH. Toxicity and related physiological activity of phenolic substances of plant origin. J Agric Food Chem. (1969) 17:497–512 doi: 10.1021/jf60163a004
55. Ortiz LT, Alzueta C, Treviño J, Castaño M. Effects of faba bean tannins on the growth and histological structure of the intestinal tract and liver of chicks and rats. Br Poult Sci. (1994) 35:743–54. doi: 10.1080/00071669408417739
56. Murdiati TB, McSweeney CS, Campbell RS, Stoltz DS. Prevention of hydrolysable tannin toxicity in goats fed Clidemia hirta by calcium hydroxide supplementation. J Appl Toxicol. (2010) 10:325–31. doi: 10.1002/jat.2550100504
57. Dias J, Alvarez MJ, Diez A, Arzel J, Corraze G, Bautista JM, et al. Regulation of hepatic lipogenesis by dietary protein/energy in juvenile European seabass (Dicentrarchus Labrax). Aquaculture. (1998) 161:169–86. doi: 10.1016/S0044-8486(97)00268-8
58. Tian LX, Liu YJ, Yang HJ, Liang GY, Niu J. Effects of different dietary wheat starch levels on growth, feed efficiency and digestibility in grass carp (Ctenopharyngodon idella). Aquac Int. (2012) 20:283–93. doi: 10.1007/s10499-011-9456-6
59. Craig SR, Washburn BS, Gatlin DM. Effects of dietary lipids on body composition and liver function in juvenile red drum, Sciaenops ocellatus. Fish Physiol Biochem. (1999) 21:249–55. doi: 10.1023/A:1007843420128
60. Chaiyapechara S, Casten MT, Hardy RW, Dong FM. Fish performance, fillet characteristics, and health assessment index of rainbow trout (Oncorhynchus mykiss) fed diets containing adequate and high concentrations of lipid and vitamin E. Aquaculture. (2003) 219:715–38. doi: 10.1016/S0044-8486(03)00025-5
61. Shan X, Xiao Z, Huang W, Dou S. Effects of photoperiod on growth, mortality and digestive enzymes in miiuy croaker larvae and juveniles. Aquaculture. (2008) 281:70–6. doi: 10.1016/j.aquaculture.2008.05.034
62. Bell J M. Factors affecting the nutritional value of canola meal: A review. Can J Anim Sci. (1993) 73:689–97. doi: 10.4141/cjas93-075
63. Liu HW, Gai F, Gasco L, Brugiapaglia A, Lussiana C, Guo KJ, et al. Effects of chestnut tannins on carcass characteristics, meat quality, lipid oxidation and fatty acid composition of rabbits. Meat Sci. (2009) 83:678–83. doi: 10.1016/j.meatsci.2009.08.003
64. Lau DW, King AJ. Pre- and post-mortem use of grape seed extract in dark poultry meat to inhibit development of thiobarbituric acid reactive substances. J Agric Food Chem. (2003) 51:1602–7. doi: 10.1021/jf020740m
65. Carvalho CDS, Fernandes MN. Effect of copper on liver key enzymes of anaerobic glucose metabolism from freshwater tropical fish Prochilodus lineatus. Comp Biochem Physiol Part A Mol Integr Physiol. (2008) 151:437–42. doi: 10.1016/j.cbpa.2007.04.016
66. Chen QL, Luo Z, Liu X, Song YF, Liu CX, Zheng JL, et al. Effects of waterborne chronic copper exposure on hepatic lipid metabolism and metal-element composition in Synechogobius hasta. Arch Environ Contam Toxicol. (2013) 64:301–15. doi: 10.1007/s00244-012-9835-7
67. Brown WV. High-density lipoprotein and transport of cholesterol and triglyceride in blood. J Clin Lipidol. (2007) 1:7–19. doi: 10.1016/j.jacl.2007.02.001
68. Yun B, Mai K, Zhang W, Xu W. Effects of dietary cholesterol on growth performance, feed intake and cholesterol metabolism in juvenile turbot (Scophthalmus maximus L.) fed high plant protein diets. Aquaculture. (2011) 319:105–10. doi: 10.1016/j.aquaculture.2011.06.028
69. Stensvold I. Tea consumption. Relationship to cholesterol, blood pressure, and coronary and total mortality. Prev Med. (1992) 21:546–53. doi: 10.1016/0091-7435(92)90062-M
70. Yugarani T, Tan B, Das N. The effects of tannic acid on serum lipid parameters and tissue lipid peroxides in the spontaneously hypertensive and wistar kyoto rats. Planta Med. (1993) 59:28–31. doi: 10.1055/s-2006-959598
71. Yugarani T, Tan BKH, Das NP. The effects of tannic acid on serum and liver lipids of RAIF and RICO rats fed on high fat diet. Comp Biochem Physiol A Physiol. (1993) 104:339–43. doi: 10.1016/0300-9629(93)90326-Y
72. Matsumoto K, Kadowaki A, Ozaki N, Takenaka M, Ono H, Yokoyama S, et al. Bile acid-binding ability of kaki-tannin from young fruits of persimmon (Diospyros kaki) in vitro and in vivo. Phytother Res. (2011) 25:624–8. doi: 10.1002/ptr.3306
73. Zou B, Ge ZZ, Zhang Y, Du J, Xu Z, Li CM. Persimmon tannin accounts for hypolipidemic effects of persimmon through activating of AMPK and suppressing NF-κB activation and inflammatory responses in high-fat diet rats. Food Funct. (2014) 5:1536–46. doi: 10.1039/C3FO60635J
74. Zou B, Li CM, Chen JY, Dong XQ, Zhang Y, Du J. High molecular weight persimmon tannin is a potent hypolipidemic in high-cholesterol diet fed rats. Food Res Int. (2012) 48:970–7. doi: 10.1016/j.foodres.2012.05.024
75. Henderson RJ, Tocher DR. The lipid composition and biochemistry of freshwater fish. Prog Lipid Res. (1987) 26:281–347. doi: 10.1016/0163-7827(87)90002-6
76. Tocher DR. Metabolism and functions of lipids and fatty acids in teleost fish. Rev Fish Sci. (2003) 11:107–84. doi: 10.1080/713610925
77. Albalat A, Saera-Vila A, Capilla E, Gutiérrez J, Pérez-Sánchez J, Navarro I. Insulin regulation of lipoprotein lipase (LPL) activity and expression in gilthead sea bream (Sparus aurata). Comp Biochem Physiol Part B Biochem Mol Biol. (2007) 148:151–9. doi: 10.1016/j.cbpb.2007.05.004
78. Albalat A, Sánchez-Gurmaches J, Gutiérrez J, Navarro I. Regulation of lipoprotein lipase activity in rainbow trout (Oncorhynchus mykiss) tissues. Gen Comp Endocrinol. (2006) 146:226–35. doi: 10.1016/j.ygcen.2005.11.011
79. Leaver MJ, Bautista JM, Björnsson BT, Jönsson E, Krey G, Tocher DR, et al. Towards fish lipid nutrigenomics: current state and prospects for fin-fish aquaculture. Rev Fish Sci. (2008) 16:73–94. doi: 10.1080/10641260802325278
80. Kerner J, Hoppel C. Fatty acid import into mitochondria. Biochim Biophys Acta. (2000) 1486:0–17. doi: 10.1016/S1388-1981(00)00044-5
81. Stone DAJ, Allan GL, Anderson AJ. Carbohydrate utilization by juvenile silver perch, Bidyanus bidyanus (Mitchell). II Digestibility and utilization of starch and its breakdown products. Aquac Res. (2015) 34:109–21. doi: 10.1046/j.1365-2109.2003.00776.x
82. Hidalgo MC, Urea E, Sanz A. Comparative study of digestive enzymes in fish with different nutritional habits. Proteolytic and amylase activities. Aquaculture. (1999) 170:267–83. doi: 10.1016/S0044-8486(98)00413-X
83. Lanari D, Poli BM, Ballestrazzi R, Lupi P, D'Agaro E, Mecatti M. The effects of dietary fat and NFE levels on growing European sea bass (Dicentrarchus labrax L.). Growth rate, body and fillet composition, carcass traits and nutrient retention efficiency. Aquaculture. (1999) 179:351–64. doi: 10.1016/S0044-8486(99)00170-2
84. Hemre GI, Mommsen TP, Krogdahl Å. Carbohydrates in fish nutrition: effects on growth, glucose metabolism and hepatic enzymes. Aquac Nutr. (2002) 8:175–94. doi: 10.1046/j.1365-2095.2002.00200.x
85. Leung LY, Woo NYS. Effects of growth hormone, insulin-like growth factor I, triiodothyronine, thyroxine, and cortisol on gene expression of carbohydrate metabolic enzymes in sea bream hepatocytes. Comp Biochem Physiol Part A Mol Integr Physiol. (2010) 157:272–82. doi: 10.1016/j.cbpa.2010.07.010
86. Leung LY, Woo NYS. Influence of dietary carbohydrate level on endocrine status and hepatic carbohydrate metabolism in the marine fish Sparus sarba. Fish Physiol Biochem. (2012) 38:543–54. doi: 10.1007/s10695-011-9534-8
87. van de Werve G, Lange A, Newgard C, Méchin MC, Li Y, Berteloot A. New lessons in the regulation of glucose metabolism taught by the glucose 6-phosphatase system. Febs J. (2010) 267:1533–49. doi: 10.1046/j.1432-1327.2000.01160.x
88. Resources N. Nutrient Requirements of Fish and Shrimp (2011). Washington, DC: National Academies Press.
89. Enes P, Panserat S, Kaushik S, Oliva-Teles A. Effect of normal and waxy maize starch on growth, food utilization and hepatic glucose metabolism in European sea bass (Dicentrarchus labrax) juveniles. Comp Biochem Physiol Part A Mol Integr Physiol. (2006) 143:89–96. doi: 10.1016/j.cbpa.2005.10.027
90. Enes P, Panserat S, Kaushik S, Oliva-Teles A. Hepatic glucokinase and glucose-6-phosphatase responses to dietary glucose and starch in gilthead sea bream (Sparus aurata) juveniles reared at two temperatures. Comp Biochem Physiol Part A Mol Integr Physiol. (2008) 149:80–6. doi: 10.1016/j.cbpa.2007.10.012
Keywords: Ctenopharyngodon idellus, hydrolysable tannin, metabolism, protein, lipid, carbohydrate
Citation: Yao J, Chen P, Apraku A, Zhang G, Huang Z and Hua X (2019) Hydrolysable Tannin Supplementation Alters Digestibility and Utilization of Dietary Protein, Lipid, and Carbohydrate in Grass Carp (Ctenopharyngodon idellus). Front. Nutr. 6:183. doi: 10.3389/fnut.2019.00183
Received: 17 June 2019; Accepted: 21 November 2019;
Published: 17 December 2019.
Edited by:
Kathleen L. Hefferon, Cornell University, United StatesReviewed by:
Zhen-Yu Du, East China Normal University, ChinaSomnath Mandal, Uttar Banga Krishi Viswavidyalaya, India
Copyright © 2019 Yao, Chen, Apraku, Zhang, Huang and Hua. This is an open-access article distributed under the terms of the Creative Commons Attribution License (CC BY). The use, distribution or reproduction in other forums is permitted, provided the original author(s) and the copyright owner(s) are credited and that the original publication in this journal is cited, in accordance with accepted academic practice. No use, distribution or reproduction is permitted which does not comply with these terms.
*Correspondence: Xueming Hua, eG1odWFAc2hvdS5lZHUuY24=
†These authors have contributed equally to this work