- 1Department of Physiology, St. John's Medical College, Bengaluru, India
- 2Centre for Human and Applied Physiological Sciences, King's College London, London, United Kingdom
- 3Division of Nutrition, St. John's Research Institute, Bengaluru, India
- 4Division of Epidemiology and Biostatistics, St. John's Research Institute, Bengaluru, India
- 5Apollo Hospitals, Bengaluru, India
Background: Type 2 Diabetes (T2D) is a major concern among Asian Indians, not least because many develop T2D at despite having a normal BMI (body mass index), and with relatively low body fat. Asian Indians are also generally considered to have relatively low skeletal muscle mass and strength, this has not been explored in the context of T2D.
Aim: The present study aimed to compare skeletal muscle mass, function and contractile quality (strength/mass) between healthy controls, those with prediabetes (PD) as well as T2D middle-aged non-obese Asian Indians.
Methods: Adult males between the age of 20–50 years, consisting of healthy controls (n = 44), PD (n = 125) and T2D (n = 55) were studied. Skeletal muscle mass was measured using Dual X-ray Absorptiometry (DXA). Isometric and dynamic muscle function was measured using an isokinetic dynamometer (at 0, 60, 120, 180 degree/s). Muscle contractile quality was derived by dividing the peak muscle torque with the respective LMM (lower limb muscle mass). Fasting blood glucose (FBG) and insulin were used to derive insulin resistance (HOMA-IR).
Results: The control group was on average 10 years younger than the other two groups (p < 0.01). The LMM was similar across the three study groups. However, the age-adjusted mean muscle torque was significantly lower in both absolute and normalized isometric and isokinetic strength in PD and T2D groups compared to controls (p ≤ 0.01), with the difference persisting even after adjusting for age and other covariates. However, there was no difference in muscle strength and contractile quality between the PD and T2D study groups.
Conclusions: Muscle strength and contractile quality would appear to be sensitive and early indices of the trajectory toward diabetes in Asian Indians and more so than skeletal muscle mass. It is thus important to recognize the importance of functional measurements among this population when considering the role of muscle in diabetes. The data also would suggest that specific muscle conditioning (e.g., resistance training) might have efficacy in improving function as well as muscle mass, and thus aiding in the prevention of the trajectory toward the development of T2D.
Introduction
Both contractile and metabolic signals play integral roles in regulating muscle homeostasis (1). The interrelationships between metabolic and mechanical signals help maintain contractile function (strength), metabolic health, physical well-being, and quality of life (2). Ideally, muscle bulk (mass) along with function should reflect an optimum balance between metabolic and contractile function (3). Therefore, skeletal muscle mass alone has been used as a reflection of nutritional and physical activity status in both health and disease (4). However, muscle mass is a static measure, depending on its composition of contractile and cytoskeletal proteins, as well as intra and intermyocellular fat (5). Recent evidence suggests that there is excessive intramyocellular fat in Indians with PD (6), and therefore it is likely that muscle mass may not be altered in such subjects. In such circumstances, it is likely that skeletal muscle contractile function may provide more sensitive diagnostic information, particularly in chronic disease. An example of this is the finding of a relation between all-cause mortality or morbidity and reduced forearm muscle strength (7).
A further dimension of muscle function is contractile quality. Whilst this reflects force per unit area or mass, it can also be considered relevant to an array of muscle characteristics, including glucose metabolism, intramuscular adipose tissue, capillary density, structural composition, and fatigability (8, 9). Currently, there is a lack of literature exploring the role of skeletal muscle mass, function, and contractile quality in chronic disease. This is important, since, for example, muscle function, or greater muscle contractility, has been linked to upregulation of glucose transporters (10). Therefore, it is critical to evaluate muscle function, skeletal muscle mass as well as contractile quality in populations who are considered to have low muscle mass (10), and who have a predisposition for T2D (11).
T2D is now a major concern among Asian Indians, particularly in the subcontinent, where the number has increased markedly from 26 million in 1990 to 65 million in 2016, with an increase in crude prevalence from 5.5 to 7.7% in the same time period (12). Increasing modernization and adoption of a more sedentary lifestyle that not only results in a positive energy balance and fat deposition, but also less skeletal muscle use, could contribute to the marked increase in T2D among Asian Indians (13). This occurs at a normal BMI and has led to the terms “metabolic obesity” and “the thin-fat Indian” (13). While age-related loss in muscle mass and function among T2D has been studied extensively in western populations (14, 15), there are no studies in young and middle-aged Asian Indians who constitute a major proportion of the population in India. There are also no studies that compare skeletal muscle mass and function in healthy Asian Indians with those with PD and T2D. The aim of the present study was thus to compare skeletal muscle mass, function and contractile quality of the major limb muscle (quadriceps) between healthy males, PD and T2D middle-aged non-obese Asian Indians.
Materials and Methods
Participants
The study population consisted of healthy controls (n = 44), prediabetes (n = 125) and T2D (n = 55). Adult males between the age of 20–50 years were recruited in and around St. John's Medical College and Hospital and through advertisements. The participants were reviewed for inclusion criteria at the first point of contact including their preexisting medical records. All participants were having either fasting blood sugar or HbA1C data already available at the first point of contact. This information was used to shortlist the eligible candidates. They were then invited to undergo a 75-g oral glucose tolerance test (OGTT) and HbA1C. The eligible participants were investigated for plasma glucose obtained by the GOD POD method (Beckman Coulter AU480, Japan), and glycosylated hemoglobin (HbA1c, HPLC, BioRad, Variant Turbo II, India). The previous day of the OGTT clear instructions were given to the participant to fast for 8–10 h before the testing. The American Diabetes Association Expert Committee criteria (16) were used for the diagnosis of PD. For the control group, the following criteria were used FBG, ≤ 100 mg/dL, postprandial plasma glucose, ≤ 140 mg/dL, or HbA1c ≤ 5.6%. If dysglycemia was detected by either fasting pl glucose (101–125 mg/dL), postprandial plasma glucose (141–199 mg/dL) or HbA1c (5.7–6.4%) the subject was characterized as PD. For T2D, those diagnosed earlier, with duration of <5 years, and on treatment with oral hypoglycaemic agents were recruited. The purpose of the study and the potential risks involved were explained to each subject and written informed consent was obtained. All were in good health as determined by medical history and physical examination. Those with conditions such as anemia, joint injuries, hypertension, cardiovascular disease, tuberculosis, cancer, and thyroid disorders were excluded. All participants underwent serum lipid profiling, including serum cholesterol, high-density lipoprotein (HDL), low-density lipoprotein (LDL) and triglyceride estimations using a Chemiluminescence Immunoassay (Siemens, Model EXL with LM 1 & 2, Germany). Plasma insulin was measured by electrochemiluminescence (ADVIA Centaur CP, Siemen's Healthineers, India). Insulin resistance and beta-cell function (HOMA-IR and HOMA-%B) were assessed by the homeostatic method using standard formulae for calculation (17). Physical activity levels (PAL) were calculated based on a physical activity questionnaire (18). All protocols were approved by the Institutional Ethics Committee, St. John's National Academy of Health Sciences, Bengaluru, India (IEC reference number 66-2014/112-2014 /116-2015).
Body Composition
All participants underwent anthropometric assessment. This included weight recorded in minimal clothing to the nearest 0.1 kg, using a digital scale (Salter digital scale, 9069 PK3R, Tonbridge, UK) and height to the nearest 0.1 cm, using a Stadiometer (Holtain, Crymych, UK). Their waist and hip circumferences were measured using a standard non-stretchable tape at the narrowest point between the iliac crest and ribcage (waist) and at the level of the greater trochanter (hip). Body composition was assessed using DXA (Model DPXMD 7254, Lunar Corporation, Madison, WI). The mass of lean soft tissue, fat, and bone mineral for both the whole body and specific regions were measured. The defined central adiposity included fat (kg) in the trunk, including the android region. The trunk region included the neck, chest, abdominal, and pelvic areas. Its upper perimeter was the inferior edge of the chin and the lower borders intersected the middle of the femoral necks without touching the brim of the pelvis. The android region was the area between the ribs and the pelvis and was totally enclosed by the trunk region. Appendicular muscle index (AMI) an indicator of sarcopenia status was derived using appendicular muscle mass (AMM) which is equivalent to the sum of lean soft tissue in both the right and left arms and legs (19). Equation used for AMI was, AMI = AMM/Ht2 (20).
Muscle Strength and Contractile Quality
Skeletal muscle strength of the knee extensors (quadriceps) of the dominant leg was assessed using an isokinetic dynamometer (Kin Com AP1, Chattanooga Group, Tennessee, USA). All participants underwent familiarization session before the actual protocol. For majority of the participants, familiarization was performed on the previous day of the experiment. If participants could not come on 2 days, we performed the familiarization session on the experimental day. However, adequate rest period (1–2 h) was given before the actual skeletal muscle strength assessment. Participants were instructed to perform a standardized 5-min warm-up indoor brisk walk, following which they were seated in the dynamometer in an upright position. Stabilization straps were placed across the chest and the hip to prevent the involvement of upper limb and trunk muscles during the test. The thigh was stabilized by placing support over the distal third of the thigh. The axis of dynamometer was aligned with the axis of rotation of knee (lateral condyle) and the distal support pad was placed proximal to the malleoli. The lever arm between the center of rotation of the dynamometer and the point of application of force-length was recorded. Maximal isometric torque (0 degree/s) was measured with the lever arm locked at the position of 30 degrees of knee joint extension from the knee flexed (90 degree) position. Peak isokinetic strength for knee extensor was assessed at 3 angular velocities 60, 120, 180 degree/s. A slow angular velocity of 60 degrees/s was used as the main dynamic measure for relation to the metabolic data as this angular velocity allows sufficient time for force development and complete muscle activation to have occurred at the point of the measurement (21). The best of three maximal voluntary contractions for knee extension was used for the analysis. For majority of the participant's right leg was used for the assessment, however if there was any history of pain or injury to the right leg, left leg was used for the assessment. The corresponding lower limb muscle mass (LMM) was considered for the contractile quality calculation. Subjects were provided with a rest interval of 2–3 min between each contraction and 5 min rest between each velocity. Muscle contractile quality was derived by dividing the peak torque (Nm) at each angular velocity by the appropriate lower limb muscle mass (Nm/kg) (22).
Statistical Analyses
Descriptive statistics are reported as mean and SD/SE for normally distributed continuous variables, else median with 25 and 75th percentiles, and number and percentage for the categorical variables. The comparison of specific characteristics between the three groups was performed using analysis of variance for normally distributed data. Post-hoc multiple comparisons were performed using the Bonferroni correction. For the non-normally distributed data, the Kruskal–Wallis test was used to compare between the three groups and the Mann–Whitney U-test for two group comparisons. Age-adjusted analyses for between-group differences in muscle strength and contractile quality at various angular velocities were performed using ANCOVA. Multivariate linear regression analysis was performed to find the association between muscle strength and contractile quality for isokinetic strength (60 degree/s) in T2D, PD compared to controls, adjusted for age, percent fat and PAL. The relationships between LMM, strength and contractile quality with FBG, HbA1c, HOMA-IR were assessed using correlation coefficient analyses. Partial correlations were adjusted for age. In all analyses, P < 5% was considered statistically significant. All analyses were performed using SPSS version 24.0.
Results
Among 224 participants enrolled in the study, 24.5, 55.8, and 19.6% belonged to the T2D, PD and healthy control groups, respectively. The average self-reported duration of T2D was 3.0 ± 1.5 years. Overall, the mean age of the study subjects was 39.2 ± 10.8 years. Descriptive statistics for the characteristics of study subjects by study groups are presented in Table 1. The mean age of the study subjects was significantly different across the study groups with controls being the youngest.
There was no significant difference in the mean BMI, percent fat and total lean mass between the groups (Table 1). The mean android fat and muscle to fat ratio were significantly different between PD and control groups with no significant difference between T2D and prediabetes groups. The AMI was similar across the study groups. The reported levels of physical activity were significantly different between the PD and T2D groups. The AMI for T2D and PD study groups were not ≤ 2 SD of the young control mean values.
Basal plasma insulin levels were not significantly different between the groups. Mean FBG, HbA1c, and HOMA -IR were significantly higher in T2D compared to PD and control groups, with no significant difference between PD and control groups. Mean HOMA β was significantly lower in T2D compared to PD and control groups.
The comparison of LMM between the study groups is represented in Figure 1A. Mean LMM was not significantly different between the groups (p = 0.07). The comparison of muscle strength and contractile quality between the study groups at the different angular velocities is shown in Figures 1B,C, respectively. There was no significant association between age with muscle strength, and contractile quality (ρ = −0.11, P = 0.11; ρ = −0.12, P = 0.08). However, age was significantly different between the study groups. When an age-adjusted analysis was performed the data still showed significant differences in absolute muscle strength (torque) and strength normalized for muscle mass (contractie quality) between the study groups (p ≤ 0.01) across all angular velocities. Age-adjusted results revealed that both maximal muscle strength and contractile quality were significantly lower in T2D and PD groups compared to the control group (p ≤ 0.01). However, there were no significant differences between the T2D and PD groups in any of the functional measurements. Muscle strength and contractile quality derived from isometric (0 degree) and isokinetic (60 degree) were compared between the three groups using multiple linear regression adjusting for different covariates (Table 2). After adjusting for age, percent fat and PAL as co-variates, muscle strength (β = −24.6, 95% CI −35.9 to −13.2 for isometric; β = −17.2, 95% CI −26.5 to −8.0 for isokinetic) and contractile quality (β = −2.75; 95% CI −4.02 to −1.47 for isometric; β = −2.04; 95% CI −3.12 to −0.95; for isokinetic) were significantly lower in PD compared to controls with no significant difference between PD and T2D or Control and T2D. There was a significant correlation between skeletal muscle strength and contractile quality with the duration of type 2 diabetes (ρ = −0.33, P = 0.04; ρ = −0.34, P = 0.03). After adjusting the same with age, body fat and PAL in regression analysis the significance disappears (β = −4.18, p = 0.06; β = −0.10, p = 0.17).
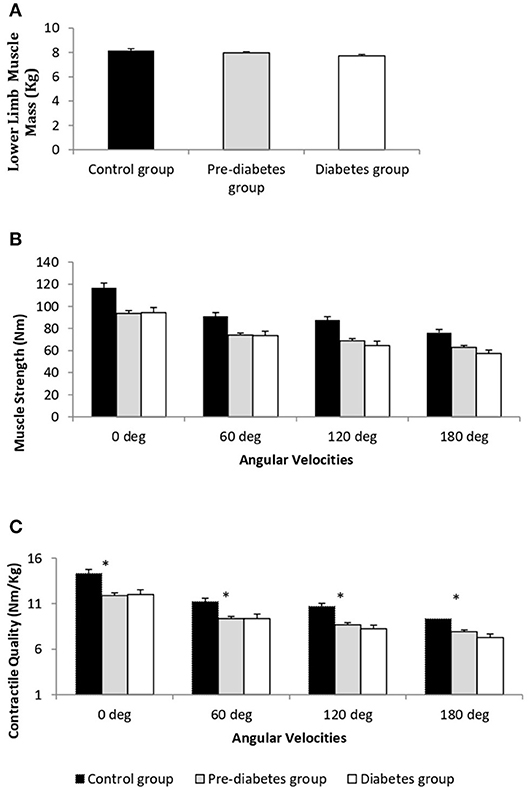
Figure 1. Comparison of lower limb muscle mass (kg) (A), absolute muscle strength (Torque, Nm) (B), and muscle contractile quality (Nm/kg limb muscle mass) (C) in the diabetes (n = 55), pre-diabetes (n = 125), and control (n = 44) groups. *T2DM and PD groups are significantly different from control group p < 0.01; age adjusted data represented as mean ± SE.
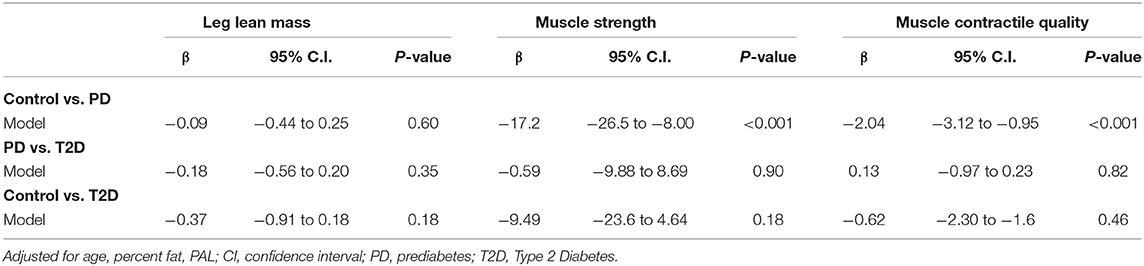
Table 2. Multivariate regression models for muscle mass, strength, and contractile quality across the study groups.
A pooled analysis was performed to explore the relationship between LMM, muscle strength and contractile quality with HOMA-IR (Figure 2). After adjusting for age, there was a significant positive association between LMM and HOMA-IR (r = 0.22, p < 0.01). Skeletal muscle strength was not associated with HOMA-IR, however, there was a significant, inverse relationship between muscle contractile quality (Isokinetic 60 degree) and HOMA-IR (r = −0.15, p = 0.03). It was noted that for every unit increase in HOMA-IR, muscle contractile quality decreased by 0.54 Nm/kg (β = −0.54; 95% C.I. −0.97 to −0.09). Age-adjusted associations between FBG, HbA1c with LMM, muscle strength and contractile quality were not significant.
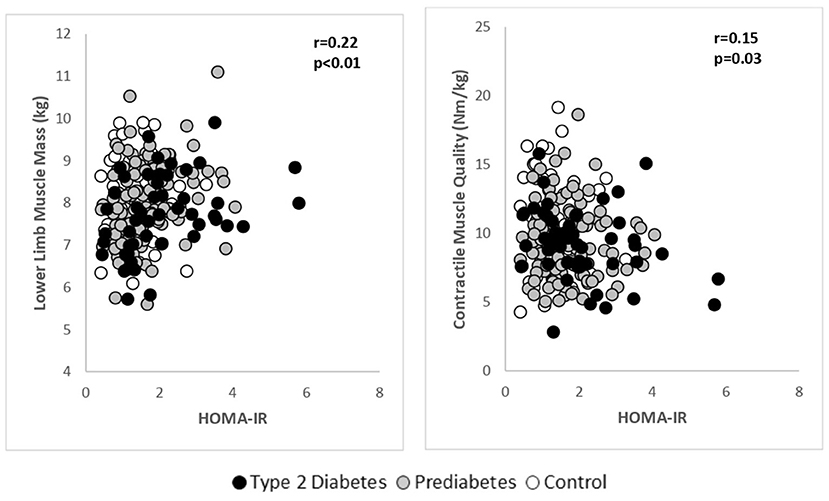
Figure 2. Age adjusted relationships between lower limb muscle mass and contractile muscle quality with HOMA-IR in the pooled study groups.
Discussion
This data is the first to examine skeletal muscle mass and function in major limb muscles in regard to metabolic disease in an Indian population. The data from the present study shows that quadriceps function when measured both in absolute (Nm) and relative (Nm/kg muscle mass) terms, and for both isometric and dynamic contractions, was similar between individuals with PD and T2D. However, the contractile function was lower in both of these groups compared with healthy controls, even when allowing for the younger age of the controls. Interestingly however, muscle mass (LMM) did not differ between the three study groups when corrected for age. The study also revealed a significant positive association between insulin resistance (HOMA-IR) and muscle mass for pooled data, whilst muscle contractile quality was inversely and significantly associated with insulin resistance.
A loss of muscle mass and function has been reported among T2D individuals particularly in the lower extremities compared to healthy individuals (23). The majority of these reports come from European (24) or American (25) or East Asian elderly population (26) with T2D, in whom age-related changes cannot be separated from the changes due to their diabetes status (27, 28). One possible explanation for the lower force per unit mass in the PD and T2D individuals in the present study concerns the level of neural drive to the muscle during the voluntary contractions. The assumption was that this would be similar in the three groups, but it cannot be excluded that PD and T2D individuals were less able to activate their knee extensor muscles during contractions. For activations to be determined, the twitch interpolation technique (29) would need to be utilized, which was not possible in the present study.
The proposed mechanisms by which T2D/PD could by themselves induce changes in skeletal muscle, beyond aging, could be the independent effect of insulin resistance on mitochondrial dysfunction, protein degradation, and autophagy pathways in the skeletal muscle (30–32). One of the potential mechanisms by which insulin resistance could induce mitochondrial dysfunction and negatively impacting muscle mass and function could be lipid accumulation or the lipotoxicity hypothesis. Excessive lipid accumulation due to defective muscle lipid oxidation could cause impaired mitochondrial number or function (reduced oxidative enzyme capacity) (33). However, Nair et al. demonstrated that Indians irrespective of their diabetes status had higher OXPHOS capacity than Northern European Americans indicating a dissociation between mitochondrial dysfunction and insulin resistance (34). However, this study was performed on a small sample of migrant Indians in the USA. With environmental factors and lifestyle habits differing across native compared to migrant Indians, extrapolation of the data needs further exploration.
There was a significant positive association between lower limb muscle mass and insulin resistance in the present study. This finding has not been reported earlier in Asian Indians. The majority of studies have demonstrated an inverse association between insulin resistance and muscle mass. For instance, the third National Health and Nutrition Examination Survey demonstrated that relative muscle mass, as estimated by bioelectrical impedance, was inversely associated with insulin resistance and PD (35). Similarly, a 10-year longitudinal study demonstrated that there was an independent inverse association between insulin resistance and muscle mass among middle-aged and older healthy Americans of Japanese origin (36). However, the Baltimore Longitudinal Study of Aging (2003–2011) reported hyperglycemia was not related to decreased skeletal muscle mass over time among multiracial Americans (37). The fact that muscle mass was related to insulin resistance positively in the current study even after adjusting for age needs further exploration, but maybe explained, in part, by inter- and intramyocellular lipid accumulation, contributing to the muscle bulk. A recently published analysis of muscle biopsy samples from our group showed evidence of excessive intramyocellular fat among Asian Indians with PD compared to healthy controls (6).
Contractile quality was shown to be inversely associated with HOMA-IR in the present study. This finding was similar to The Health ABC elderly cohort (70–79 years), with muscle mass and contractile quality demonstrating similar association, but not with strength (38). The current study population being younger with an average age of 39 years and included PD and T2D compared to the elderly population from the Health ABC elderly cohort. With every unit increase in HOMA-IR, there was a 0.54 Nm/kg decrement in contractile quality in the present study. In a similar study performed on Japanese population with Type 2 Diabetes, it was noted that knee extensor contractile quality decreased by 0.40 Nm/kg (body weight) with every unit increase in HOMA IR (39). The Korea National and Health Examination Survey (KNHANES) VI on Type 2 Diabetes population demonstrated that the hand grip strength normalized for body weight demonstrated a decrease of 3.70 kg/kg with every unit increase in HOMA IR (40).The fact that the current study group might have changes in skeletal muscle earlier than other population emphasizes the need for early evaluation of skeletal muscle mass and function including planning of preventive strategies.
Based on a recent systematic review and meta-analysis, the role of insulin on the human skeletal muscle anabolism through increased muscle protein synthesis (MPS) has been shown to be influenced by amino acid delivery (41). The branched chain amino acid leucine is known to play vital role in the MPS (42, 43). However, the leucine requirement in normal, well-nourished Western and Indian men has been determined to be 2.5 times higher than the 1985 FAO/WHO/UNU recommendation. On the contrary, lysine has been shown to be the most limiting in cereal protein and at a much lower concentration with relatively poor digestibility and utilization (44, 45). With majority of Asian Indians consuming a cereal based diet they pose a risk of a deficiency of quality protein, in terms of indispensable amino acid (particularly lysine). This is due to the fact that 60% of their protein are from cereals with relatively low quality and digestibility (46). Whether high-quality protein intake could harness the positive effects of insulin on muscle metabolism needs to be explored particularly among Asian Indians. However, the role of insulin in reducing muscle protein breakdown (MPB) is independent of amino acid availability (37). It is suggested that impaired insulin signaling leading to insulin resistance could have an effect on muscle metabolism (MPS and MPB) and endothelial dysfunction beyond glucose intolerance (47). Further, Mesinovic et al. suggested that there could be a bidirectional link between T2D and skeletal muscle changes, and the existence of one condition may increase the risk of developing the other. Physical inactivity, insulin resistance, inflammation, increased advanced glycation end-products accumulation, increased oxidative stress, and vascular complications can all affect various components of muscle health (muscle mass, strength, and contractile quality) and impaired muscle health could further contribute toward the development and progression of T2D (48). Therefore, it is important to explore, through a multipronged approach, the different mechanisms that could regulate skeletal muscle mass, strength, and contractile quality in Asian Indians.
While muscle function among PD and T2D was reduced in comparison with healthy controls, the PD and T2D groups were comparable in both muscle mass, absolute strength, and contractile quality. We had expected a graded response between the control, PD and T2D study groups as the metabolic disease progressed, even though this was a cross-sectional study. The reasons for this lack of difference between PD and T2D remain to be elucidated, but these findings suggest that in a population where reported prevalence rates of T2D are high, a simple functional evaluation of muscle strength may not only act as an early prognostic tool but also may act as a positive feedback mechanism for participants trying to increase their levels of activity and physical fitness. After adjusting for age, percent fat and PAL there was no significant difference in muscle strength and contractile quality between T2D and control groups. This was surprising and could suggest that there are factors beyond those which been explored as part of the study. The fact that the T2D individuals were on oral hypoglycemic agents (OHA) and which might have had an independent effect on muscle strength and contractile quality cannot be ruled out. With a body of literature suggesting that OHA could cause muscle atrophy and rest demonstrating no effect (49). It is nearly impossible to differentiate the effect of OHA from that of a disease process in diabetes mellitus. With the small sample size and lack of detailed pharmacological profiling available as part of the study, we could only speculate at this stage and could form the basis of future studies.
Conclusion
Skeletal muscle mass, strength, and contractile quality were similar between PD and T2D Asian Indians, but lower compared to healthy controls, with the findings persisting even after adjusting for the age differences between the groups. This would suggest that the measurement of skeletal muscle function, as well as mass, among Asian Indians is important in order to detect early deterioration in metabolic health. The data also suggest the need to evaluate the role of resistance exercise in improving muscle strength and quality through well-designed clinical studies in Asian Indians.
Data Availability Statement
The datasets for this manuscript are not publicly available because they are a part of an on-going trial. Requests to access the datasets should be directed to SSa, c3VjaGFyaXRhLmRyQGdtYWlsLmNvbQ==.
Ethics Statement
The studies involving human participants were reviewed and approved by Institutional Review Board, St. John's National Academy of Health Sciences, Bangalore, India. The patients/participants provided their written informed consent to participate in this study.
Author Contributions
SSa was part of the conception and design, the performance of the measurements and analyses, interpretation of the data, and drafted the manuscript. AK and SH were part of conception and design, interpretation of data, writing of manuscript, and final approval of manuscript. NS was involved in the performance of measurements and analyses. PR involved in screening and recruitment of subjects. SSe was involved in statistical analyses.
Funding
The major support for this study came from the Wellcome Trust/DBT India Alliance Fellowship [grant number IA/I/13/1/500907] awarded to SSa. The study was also supported partially by funding received by the Rajiv Gandhi University of Health Sciences, Bangalore, India and The Physiological Society's International Senior Research Grant, UK.
Conflict of Interest
The authors declare that the research was conducted in the absence of any commercial or financial relationships that could be construed as a potential conflict of interest.
Acknowledgments
Authors would like to acknowledge Ms. Ammu Anna Kurien, Mr. Sreejith B, Mrs. Princiya Rufus, and Dr. Pranathi Racha for their support during the data collection. We would also like to thank Dr. Tinku Thomas and Dr. Ronald J. Bosch for constant input during the designing of the study. Dr. Ganapathi Bantwal and Dr. Vageesh Ayyar for helping with the recruitment of the participants.
References
1. Lai N, Gladden LB, Carlier PG, Cabrera ME. Models of muscle contraction and energetics. Drug Discov Today Dis Models. (2008) 5:273–88. doi: 10.1016/j.ddmod.2009.07.001
2. Correa-de-Araujo R, Harris-Love MO, Miljkovic I, Fragala MS, Anthony BW, Manini TM. The need for standardized assessment of muscle quality in skeletal muscle function deficit and other aging-related muscle dysfunctions: a symposium report. Front Physiol. (2017) 8:87. doi: 10.3389/fphys.2017.00087
3. McLeod M, Breen L, Hamilton DL, Philp A. Live strong and prosper: the importance of skeletal muscle strength for healthy ageing. Biogerontology. (2016) 17:497–510. doi: 10.1007/s10522-015-9631-7
4. Wolfe RR. The underappreciated role of muscle in health and disease. Am J Clin Nutr. (2006) 84:475–82. doi: 10.1093/ajcn/84.3.475
5. Schrauwen-Hinderling VB, Hesselink MK, Schrauwen P, Kooi ME. Intramyocellular lipid content in human skeletal muscle. Obesity. (2006) 14:357–67. doi: 10.1038/oby.2006.47
6. Sucharita S, Pranathi R, Correa M, Keerthana P, Ramesh LJ, Bantwal G, et al. Evidence of higher intramyocellular fat among normal and overweight Indians with prediabetes. Eur J Clin Nutr. (2019) 73:1373–81. doi: 10.1038/s41430-019-0402-4
7. Li R, Xia J, Zhang XI, Gathirua-Mwangi WG, Guo J, Li Y, et al. Associations of muscle mass and strength with all-cause mortality among US older adults. Med Sci Sports Exerc. (2018) 50:458–67. doi: 10.1249/MSS.0000000000001448
8. Hazzard WR, Halter JB. Hazzard's Geriatric Medicine and Gerontology. 6th ed. New York, NY: McGraw-Hill Medical Pub. Division (2009).
9. Cawthon PM, Fox KM, Gandra SR, Delmonico MJ, Chiou CF, Anthony MS, et al. Do muscle mass, muscle density, strength, and physical function similarly influence risk of hospitalization in older adults? J Am Geriatr Soc. (2009) 57:1411–9. doi: 10.1111/j.1532-5415.2009.02366.x
10. Bryant NJ, Govers R, James DE. Regulated transport of the glucose transporter GLUT4. Nat Rev Mol Cell Biol. (2002) 3:267–77. doi: 10.1038/nrm782
11. Mohan V. Why are Indians more prone to diabetes? J Assoc Physicians India. (2004) 52:468–74. Available online at: http://www.japi.org/june2004/R-468.pdf
12. India State-Level Disease Burden Initiative Diabetes Collaborators. The increasing burden of diabetes and variations among the states of India: the Global Burden of Disease Study 1990-2016. Lancet Glob Health. (2018) 6:e1352–62. doi: 10.1016/S2214-109X(18)30387-5
13. Kurpad AV, Varadharajan KS, Aeberli I. The thin-fat phenotype and global metabolic disease risk. Curr Opin Clin Nutr Metab Care. (2011) 14:542–7. doi: 10.1097/MCO.0b013e32834b6e5e
14. Umegaki H. Sarcopenia and diabetes: hyperglycemia is a risk factor for age-associated muscle mass and functional reduction. J Diabetes Investig. (2015) 6:623–4. doi: 10.1111/jdi.12365
15. Kim TN, Park MS, Yang SJ, Yoo HJ, Kang HJ, Song W, et al. Prevalence and determinant factors of sarcopenia in patients with type 2 diabetes: the Korean Sarcopenic Obesity Study (KSOS). Diabetes Care. (2010) 33:1497–9. doi: 10.2337/dc09-2310
16. Chamberlain JJ, Rhinehart AS, Shaefer CF Jr, Neuman A. Diagnosis and management of diabetes: synopsis of the 2016 American Diabetes Association Standards of Medical Care in Diabetes. Ann Intern Med. (2016) 164:542–52. doi: 10.7326/M15-3016
17. Matthews DR, Hosker JP, Rudenski AS, Naylor BA, Treacher DF, Turner RC. Homeostasis model assessment: insulin resistance and β-cell function from fasting plasma glucose and insulin concentrations in man. Diabetologia. (1985) 28:412–9. doi: 10.1007/BF00280883
18. Vaz M, Bharathi AV, Thomas T, Yusuf S, Kurpad AV. The repeatability of self-reported physical activity patterns in rural South India. Asia Pac J Clin Nutr. (2009) 18:71–5. Available online at: http://apjcn.nhri.org.tw/server/APJCN/18/1/71.pdf
19. Kuriyan R, Thomas T, Ashok S, Jayakumar J, Kurpad AV. A 4-compartment model based validation of air displacement plethysmography, dual energy X-ray absorptiometry, skinfold technique & bio-electrical impedance for measuring body fat in Indian adults. Indian J Med Res. (2014) 139:700–7. Available online at: http://www.ijmr.org.in/article.asp?issn=0971-5916;year=2014;volume=139;issue=5;spage=700;epage=707;aulast=Kuriyan
20. Kwon HJ, Ha YC, Park HM. The reference value of skeletal muscle mass index for defining the sarcopenia of women in Korea. J Bone Miner Metab. (2015) 22:71–5. doi: 10.11005/jbm.2015.22.2.71
21. Jones DA, Rutherford OM, Parker DF. Physiological changes in skeletal muscle as a result of strength training. Q J Exp Physiol. (1989) 74:233–56. doi: 10.1113/expphysiol.1989.sp003268
22. Metter EJ, Lynch N, Conwit R, Lindle R, Tobin J, Hurley B. Muscle quality and age: cross-sectional and longitudinal comparisons. J Gerontol A Biol Sci Med Sci. (1999) 54:B207–18. doi: 10.1093/gerona/54.5.B207
23. Guerrero N, Bunout D, Hirsch S, Barrera G, Leiva L, Henriquez S, et al. Premature loss of muscle mass and function in type 2 diabetes. Diabetes Res Clin Pract. (2016) 117:32–8. doi: 10.1016/j.diabres.2016.04.011
24. Cruz-Jentoft AJ, Baeyens JP, Bauer JM, Boirie Y, Cederholm T, Landi F, et al. Sarcopenia: European consensus on definition and diagnosis: report of the European working group on sarcopenia in older people. Age Ageing. (2010) 39:412–23. doi: 10.1093/ageing/afq034
25. Kalyani RR, Tra Y, Yeh HC, Egan JM, Ferrucci L, Brancati FL. Quadriceps strength, quadriceps power, and gait speed in older U.S. adults with diabetes mellitus: results from the National Health and Nutrition Examination Survey, 1999-2002. J Am Geriatr Soc. (2013) 61:769–75. doi: 10.1111/jgs.12204
26. Chen LK, Liu LK, Woo J, Assantachai P, Auyeung TW, Bahyah KS, et al. Sarcopenia in Asia: consensus report of the Asian Working Group for Sarcopenia. J Am Med Dir Assoc. (2014) 15:95–101. doi: 10.1016/j.jamda.2013.11.025
27. Kirkman MS, Briscoe VJ, Clark N, Florez H, Haas LB, Halter JB, et al. Consensus development conference on diabetes and older adults. Diabetes in older adults: a consensus report. J Am Geriatr Soc. (2012) 60:2342–56. doi: 10.1111/jgs.12035
28. Abdelhafiz AH, Sinclair AJ. Management of type 2 diabetes in older people. Diabetes Ther. (2013) 4:13–26. doi: 10.1007/s13300-013-0020-4
29. Herbert RD, Gandevia SC. Twitch interpolation in human muscles: mechanisms and implications for measurement of voluntary activation. J Neurophysiol. (1999) 82:2271–83. doi: 10.1152/jn.1999.82.5.2271
30. Wang X, Hu Z, Hu J, Du J, Mitch WE. Insulin resistance accelerates muscle protein degradation: activation of the ubiquitin-proteasome pathway by defects in muscle cell signaling. Endocrinology. (2006) 147:4160–8. doi: 10.1210/en.2006-0251
31. Wada J, Nakatsuka A. Mitochondrial dynamics and mitochondrial dysfunction in diabetes. Acta Med Okayama. (2016) 70:151–8. Available online at: http://www.lib.okayama-u.ac.jp/www/acta/pdf/70_3_151.pdf
32. Sarparanta J, García-Macia M, Singh R. Autophagy and mitochondria in obesity and type 2 diabetes. Curr Diabetes Rev. (2017) 13:352–69. doi: 10.2174/1573399812666160217122530
33. He J, Watkins S, Kelley DE. Skeletal muscle lipid content and oxidative enzyme activity in relation to muscle fiber type in type 2 diabetes and obesity. Diabetes. (2001) 50:817–23. doi: 10.2337/diabetes.50.4.817
34. Nair KS, Bigelow ML, Asmann YW, Chow LS, Coenen-Schimke JM, Klaus KA, et al. Asian Indians have enhanced skeletal muscle mitochondrial capacity to produce ATP in association with severe insulin resistance. Diabetes. (2008) 57:1166–75. doi: 10.2337/db07-1556
35. Srikanthan P, Karlamangla AS. Relative muscle mass is inversely associated with insulin resistance and prediabetes. findings from the third national health and nutrition examination survey. J Clin Endocrinol Metab. (2011) 96:2898–903. doi: 10.1210/jc.2011-0435
36. Han SJ, Boyko EJ, Kim SK, Fujimoto WY, Kahn SE, Leonetti DL. Association of thigh muscle mass with insulin resistance and incident type 2 diabetes mellitus in Japanese Americans. Diabetes Metab J. (2018) 42:488–95. doi: 10.4093/dmj.2018.0022
37. Kalyani RR, Metter EJ, Egan J, Golden SH, Ferrucci L. Hyperglycemia predicts persistently lower muscle strength with aging. Diabetes Care. (2015) 38:82–90. doi: 10.2337/dc14-1166
38. Barzilay JI, Cotsonis GA, Walston J, Schwartz AV, Satterfield S, Miljkovic I, et al. Insulin resistance is associated with decreased quadriceps muscle strength in nondiabetic adults aged > = 70 years. Diabetes Care. (2009) 32:736–8. doi: 10.2337/dc08-1781
39. Nomura T, Ikeda Y, Nakao S, Ito K, Ishida K, Suehiro T, et al. Muscle strength is a marker of insulin resistance in patients with type 2 diabetes: a pilot study. Endocr J. (2007) 54:791–6. doi: 10.1507/endocrj.K07-055
40. Lee MR, Jung SM, Bang H, Kim HS, Kim YB. Association between muscle strength and type 2 diabetes mellitus in adults in Korea: data from the Korea national health and nutrition examination survey (KNHANES) VI. Medicine. (2018) 97:e10984. doi: 10.1097/MD.0000000000010984
41. Abdulla H, Smith K, Atherton PJ, Idris I. Role of insulin in the regulation of human skeletal muscle protein synthesis and breakdown: a systematic review and meta-analysis. Diabetologia. (2016) 59:44–55. doi: 10.1007/s00125-015-3751-0
42. Breen L, Churchward-Venne TA. Leucine: a nutrient 'trigger' for muscle anabolism, but what more? J Physiol. (2012) 590:2065–6. doi: 10.1113/jphysiol.2012.230631
43. Jackman SR, Witard OC, Philp A, Wallis GA, Baar K, Tipton KD. Branched-chain amino acid ingestion stimulates muscle myofibrillar protein synthesis following resistance exercise in humans. Front Physiol. (2017) 8:390. doi: 10.3389/fphys.2017.00390
44. Kurpad AV, Regan MM, Raj T, Vasudevan J, Kuriyan R, Gnanou J, et al. Lysine requirements of chronically undernourished adult Indian men, measured by a 24-h indicator amino acid oxidation and balance technique. Am J Clin Nutr. (2003) 77:101–8. doi: 10.1093/ajcn/77.1.101
45. Kurpad AV, Regan MM, Raj T, Varalakshmi S, Gnanou J, Thankachan P, et al. Leucine requirement and splanchnic uptake of leucine in chronically undernourished adult Indian subjects. Am J Clin Nutr. (2003) 77:861–7. doi: 10.1093/ajcn/77.4.861
46. Minocha S, Thomas T, Kurpad AV. Dietary protein and the health–nutrition–agriculture connection in India. J Nutr. (2017) 147:1243–50. doi: 10.3945/jn.116.243980
47. Muniyappa R, Sowers JR. Role of insulin resistance in endothelial dysfunction. Rev Endocr Metab Disord. (2013) 14:5–12. doi: 10.1007/s11154-012-9229-1
48. Mesinovic J, Zengin A, De Courten B, Ebeling PR, Scott D. Sarcopenia and type 2 diabetes mellitus: a bidirectional relationship. Diabetes Metab Syndr Obes. (2019) 12:1057–72. doi: 10.2147/DMSO.S186600
Keywords: skeletal muscle, muscle mass, muscle function, body fat, diabetes mellitus, prediabetes
Citation: Sambashivaiah S, Harridge SDR, Sharma N, Selvam S, Rohatgi P and Kurpad AV (2019) Asian Indians With Prediabetes Have Similar Skeletal Muscle Mass and Function to Those With Type 2 Diabetes. Front. Nutr. 6:179. doi: 10.3389/fnut.2019.00179
Received: 15 July 2019; Accepted: 12 November 2019;
Published: 27 November 2019.
Edited by:
Andrew Philp, Garvan Institute of Medical Research, AustraliaReviewed by:
Neil Schwarz, University of South Alabama, United StatesKevin A. Zwetsloot, Appalachian State University, United States
Copyright © 2019 Sambashivaiah, Harridge, Sharma, Selvam, Rohatgi and Kurpad. This is an open-access article distributed under the terms of the Creative Commons Attribution License (CC BY). The use, distribution or reproduction in other forums is permitted, provided the original author(s) and the copyright owner(s) are credited and that the original publication in this journal is cited, in accordance with accepted academic practice. No use, distribution or reproduction is permitted which does not comply with these terms.
*Correspondence: Sucharita Sambashivaiah, c3VjaGFyaXRhLmRyQGdtYWlsLmNvbQ==
†ORCID: Stephen D. R. Harridge orcid.org/0000-0002-8203-0769