- 1Centre des Sciences du Goût et de l'Alimentation, AgroSup Dijon, CNRS, INRA, Université de Bourgogne, Dijon, France
- 2Institut NuMeCan, INRA, INSERM, Université Rennes, Domaine de la Prise, Saint-Gilles, France
- 3Institut Micalis, INRA, AgroParisTech, Université Paris-Saclay, Domaine de Vilvert, Jouy-en-Josas, France
High variability exists in individual susceptibility to develop overweight in an obesogenic environment and the biological underpinnings of this heterogeneity are poorly understood. In this brief report, we show in mice that the vulnerability to diet-induced obesity is associated with low level of polysialic acid-neural cell adhesion molecule (PSA-NCAM), a factor of neural plasticity, in the hypothalamus. As we previously shown that reduction of hypothalamic PSA-NCAM is sufficient to alter energy homeostasis and promote fat storage under hypercaloric pressure, inter-individual variability in hypothalamic PSA-NCAM might account for the vulnerability to diet-induced obesity. These data support the concept that reduced plasticity in brain circuits that control appetite, metabolism and body weight confers risk for eating disorders and obesity.
Introduction
Changes in lifestyle and in the availability and quality of food largely explain the worldwide epidemic of obesity (1). However, high variability exists in individual susceptibility to develop overweight in an obesogenic environment (2). This implies that genetic risk factors among individuals substantially influence the variability in body mass index (3). A better understanding of the molecular basis of this heterogeneity might help to fight against obesity and its related disorders. In the past, numerous animal models have been used to investigate pre-existing differences that are established before the onset of corpulence and that confer a risk for common obesity (4–7). In this way, prospective studies in rats have shown that some early metabolic responses to high fat diet (HFD) at normal weight are predictive of propensity to develop obesity and liver diseases on the long term (8–11). In the present study, we found in mice that aberrant feeding behavior in response to dietary fat constitutes a latent vulnerability trait to obesity. This predictive model can be relevant to identify biological risk factors for maladpative eating behaviors and obesity before the onset of the disease. Recent genetic studies in human indicated that most of the genes associated to the body mass index are enriched in the brain and related to neuronal biology and synaptic plasticity (12, 13). Thus, we further examined the expression of polysialic acid-neural cell adhesion molecule (PSA-NCAM), a marker of neural plasticity, in mice prone to obesity, and we found that the vulnerability to obesity was correlated with decreased hypothalamus level of PSA-NCAM.
Methods
Animals
Protocols including manipulation of animals (registration number 853.01) were reviewed and approved by our local Institutional Animal Care and Use Committee (“Comité d'éthique en expérimentation animale n°105”), and were in strict accordance with the European Community guidelines (directive 86/906). Experiments were carried out with 2 months-old male C57Bl/6JOla mice from Harlan Laboratories. Mice were housed individually, fed either a standard diet (catalog number A04; Safe Laboratories; Augy, France; 3.3 kcal/kg; energy from carbohydrate/fat/protein: 72.4/8.4/19.3) or a customized highly palatable high-fat diet (catalog number U8954P V.7; Safe Laboratories; 4.4 kcal/kg; energy from carbohydrate/fat/protein: 40.8/42.9/16.3). Free access was given for food and water. Recordings of food intake and body weight were done manually at 9:00 h. For tissue collection, mice were killed between 14:00 and 16:00 h after a 6-h fast.
Medio-Basal Hypothalamus Dissection
After sacrifice, brains were quickly removed and immersed in cold PBS solution. A 2 mm coronal slice was cut with a brain matrix. The slice was laid on a 6% agarose block and the MBH was dissected under stereomicroscope and cold-light illumination using a scalpel. Tissues were immediately frozen in liquid nitrogen and stored at −80°C until experiments.
PSA-NCAM Assay
Tissues were lysed and homogenized in RIPA lysis buffer using the TissueLyser system (Qiagen; Courtaboeuf, France) and 5 mm stainless steel beads (Qiagen). The homogenates were centrifuged 5 min at 5,000 g and supernatants were collected for PSA-NCAM assay using an enzyme-linked immunosorbent assay kit (PSA NCAM ELISA kit; Eurobio; Courtaboeuf, France) and for protein assay (Bio-Rad Protein Assay Kit ll; Biorad; Marnes-la-Coquette, France).
Statistical Analysis
All data are expressed as means. Error bars indicate standard errors of the mean (SEM). Multiple comparisons between groups were carried out by one- or two-way ANOVA using Prism 5.0 software (GraphPad Software; San Diego, CA, United States). Post-hoc analyses were done when main effects reached significance. Before comparison, Bartlett's and Shapiro–Wilk's tests were applied to check equality of variances and to evaluate the normality of the distribution, respectively.
Results
The Homeostatic Feeding Response to a Dietary Fat Challenge Reveals Obesity Susceptibility
For a 2-month old mice fed with standard diet, the typical daily energy intake is 0.5 kcal per gram of body weight (The Jackson Laboratory, Mouse Phenome Database, http://phenome.jax.org/). It is well-known that high-energy foods cause transient overeating in most of the mice, which corresponds to an acute increase in energy intake during a few days (14–16), as illustrated in Figure S1A. In this study, the period of overconsumption was variable between mice and normalization of energy intake occurred after 2 days or more (Figure S1B; in kcal/gram of body weight). Consequently, the individual cumulative energy intake during 1-week HFD, hereinafter referred to as the “feeding response,” ranged from 3.96 to 6.92 kcal/g (Figure S1C). According to this response, we identified by a median split HFD-intolerant mice with high feeding response due to slow normalization, and HFD-tolerant mice with low feeding response due to fast normalization (Figures 1A,B). The two groups of mice were initially undistinguishable with respect to their energy intake on standard diet or to their initial body weight (Figures 1C,D). On HFD, tolerant mice normalized their energy intake in only 2 days after HFD introduction, whereas intolerant mice normalized it after 10 days (Figure 1E; in kcal/gram of body weight). After 2 weeks on HFD, energy intake was normalized for all mice. Nevertheless, intolerant mice kept on HFD for a long term had further episodic increases in raw energy intake which appeared after 5 weeks on HFD (Figure 1E; in kcal). Therefore, the cumulative energy intake over 3 months with HFD was significantly higher in intolerant mice than in tolerant mice (Figure 1F). Moreover, the longitudinal follow-up showed that body weight of intolerant mice maintained on HFD increased strongly after 8 weeks in comparison to tolerant mice (Figure 1G). Hence, the body weight gain between the two groups of mice was significantly different after 3 months on HFD (Figure 1H). Importantly, the terminal body weight gain was positively correlated with the feeding response to HFD (Figure 1I). By contrast, it was not correlated with the initial energy intake on standard diet or with the terminal energy intake on HFD (Figures 1J,K). These results indicated that the homeostatic feeding response to an acute HFD challenge in mice is a latent trait predictive of the propensity to gain weight under persistent caloric pressure.
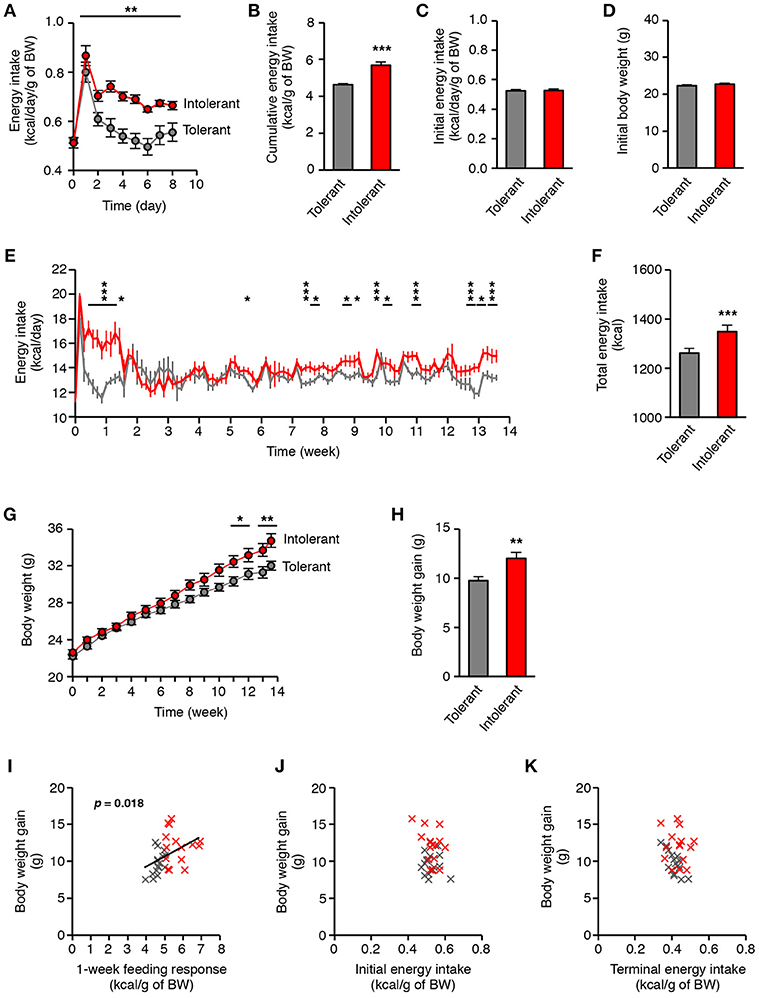
Figure 1. Individual differences in the homeostatic feeding response to HFD reveal susceptibility to diet-induced obesity. (A) Energy intake per gram of body weight of HFD-tolerant (n = 16) and HFD-intolerant (n = 15) mice during 1-week HFD (**p < 0.01, HFD-tolerant vs. HFD-intolerant mice; two-way ANOVA and Bonferroni post-hoc test). (B) Cumulative energy intake per gram of body weight of HFD-tolerant and HFD-intolerant mice after 1 week on HFD (***p < 0.001; Mann Whitney test). (C,D) Initial energy intake per gram of body weight and body weight of HFD-tolerant and HFD-intolerant mice were similar on standard chow, i.e., before HFD introduction. (E) Energy intake of HFD-tolerant and HFD-intolerant mice during 13 weeks-HFD (*p < 0.05, ***p < 0.001, HFD-tolerant vs. HFD-intolerant mice; two-way ANOVA and Bonferroni post-hoc test). (F) Total energy intake of HFD-tolerant and HFD-intolerant mice after 3-month HFD (***p < 0.001; unpaired t-test). (G) Body weight of HFD-tolerant and HFD-intolerant mice during 13-week HFD (*p < 0.05, **p < 0.01, HFD-tolerant vs. HFD-intolerant mice; two-way ANOVA and Bonferroni post-hoc test). (H) Body weight gain of HFD-tolerant and HFD-intolerant mice after 13-week HFD (**p < 0.01; unpaired t-test). (I) Correlation analysis between the acute feeding response to HFD and the body weight gain after 3-month HFD. HFD-tolerant mice are in gray, HFD-intolerant mice are in red (p < 0.018; linear regression). (J) Correlation analysis between the initial energy intake on standard diet and the body weight gain after 3-month HFD. (K) Correlation analysis between the terminal energy intake on HFD and the body weight gain after 3-month HFD. All results are mean ± SEM.
Relation Between Fat Tolerance and Hypothalamus Polysialylation
Identification of individuals prone to obesity before the onset of the disease allows investigation of the biological underpinnings of susceptibility to obesity and makes possible the discovery of biological risk factors. Pre-existing differences in brain circuits controlling appetite and energy homeostasis might account for the vulnerability to common obesity (17, 18). Recent genetic studies formally implicated the brain in obesity pathology and pinpointed neuronal genes regulating synaptic plasticity (12, 19, 20). Moreover, rodent data consistently evidenced alterations of neural function and plasticity in brain feeding circuits in animals genetically predisposed to obesity (21, 22). Thus, we hypothesized that innate alteration in molecules involved in brain neuroplasticity would confer risk for obesity.
The polysialic acid-neural cell adhesion molecule (PSA-NCAM) is a cell-surface molecule that promotes various plasticity-related changes in brain circuits (23). We recently identified PSA-NCAM in the hypothalamus as a permissive factor for the brain control of energy balance (16, 24, 25). We therefore postulated that low level of hypothalamic PSA-NCAM would incite vulnerability to obesity. To test this hypothesis, we identified in a second cohort of mice (n = 14), HFD-tolerant and HFD-intolerant individuals according to their feeding response to 1-week HFD and then we examined the concentration of PSA-NCAM in the medio-basal hypothalamus (MBH) collected after this oral fat tolerance test. Intolerant mice showed significant lower levels of hypothalamic PSA-NCAM in comparison to tolerant mice (Figure 2; tolerant: 684.7 ± 59.4 ng/mg; intolerant: 505.9 ± 27.1 ng/mg; p = 0.038). As a reference, the constitutive level of PSA-NCAM in the MBH of control mice fed a standard diet was 508.5 ± 31 ng/mg protein (n = 16). The level of PSA-NCAM in the MBH of intolerant mice did not differ from the constitutive value. These data suggest that tolerant mice might have higher ability to mobilize PSA-NCAM to trigger synaptic plasticity in the hypothalamus than intolerant mice.
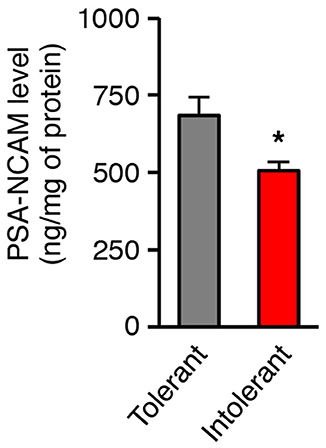
Figure 2. Individual differences in the tolerance to HFD are linked to the level of hypothalamic PSA-NCAM. PSA-NCAM levels were measured in mediobasal hypothalamus after a 1-week HFD challenge allowing identification of HFD-tolerant and HFD-intolerant mice (n = 7 HFD-tolerant, n = 7 HFD-intolerant; *p < 0.05; Mann Whitney test). Results are mean ± SEM.
Discussion
In this study, we measured the feeding response to 1-week HFD in mice. We found variability in the behavioral response with high responders who failed to rapidly normalize their energy intake during the metabolic challenge. High responders also gained more weight when they consumed the hypercaloric diet for a long time. Thus, the 1-week HFD challenge may be considered as a Dietary Fat Tolerance Test (DFTT) for identifying mice prone to nutritional obesity. Similar predictive tests have been used in the past showing that short-term response to HFD constitutes a phenotypic trait of metabolic flexibility which provides information on vulnerability to metabolic diseases (8–11). The present study further shows that the DFTT could be based on the behavioral response only, and does not necessarily imply collecting biopsies for metabolic investigation.
The DFTT might be relevant for investigating intrinsic factors that produce maladaptive eating disorders and vulnerability to obesity before the clinical onset of the disease. Some of these factors have been already evidenced, including efficiency of peripheral endocrinological and metabolic processes (8, 9, 26, 27), and responsivness of the dopaminergic system to food cues (28). Here we show that interindividual variability in the feeding response to short-term HFD is also linked to the intrahypothalamic PSA-NCAM signaling. This is in line with previous works from our group showing that experimental removal of hypothalamic PSA-NCAM increases the feeding response to HFD and the body weight gain (16, 24).
After the DFTT, intrahypothalamic PSA-NCAM content was elevated in HFD-tolerant mice, while it remained low and similar to the constitutive level in HFD-intolerant mice. This result suggests that inter-individual variability exists in the ability to upregulate PSA-NCAM production in the hypothalamus in response to the metabolic challenge, and that PSA-NCAM production was stimulated in HFD-tolerant mice. The source of variation of PSA-NCAM expression between HFD-tolerant and -intolerant animals is not known. Similar intrinsic differences in healthy animals have been already reported, linking the level of prefrontal PSA-NCAM to the susceptibility for addiction-related behaviors (29). Interindividual differences in learning-induced PSA-NCAM levels in the hippocampus is also linked to learning ability in rats (30). Interestingly, acute stress that predisposes young rats to mood and anxiety disorders during adulthood, as well as chronic stress in adult, cause dramatic alteration in PSA-NCAM expression in the limbic system (31, 32). These results show that brain PSA-NCAM synthesis is affected not only by genetic heritage but also by experience. Since litter size and maternal care are early determinants of vulnerability to obesity (33–36), PSA-NCAM variation in our model might by linked to perinatal experiences. At the molecular level, biosynthesis of PSA-NCAM in adult brain requires successive biochemical reactions, which are catalyzed by enzymes including UDP-GlcNAc 2-epimerase/ManNAc kinase and polysialyltransferase 1, encoded by Gne and St8sia4 genes, respectively. One can propose that these genes might be differentially upregulated on HFD depending on individuals. Gene polymorphism association studies and epigenetic research on these specific genes in murine models and in humans are thus promising research to increase our knowledge on obesity susceptibility.
In several models of neuropsychological disorders, the loss of neural PSA-NCAM alters physiological defenses, exacerbates symptoms or worsens the disease (29, 37–40), pointing out the potential protective role of PSA-NCAM against brain dysfunctions involving a plasticity-related component. In previous works, we found that removal of hypothalamic PSA is sufficient to alter short-term homeostatic responses to dietary fat and increase body weight gain on a long term (16, 24, 25), confirming that hypothalamic PSA-NCAM is critical to maintain energy homeostasis upon metabolic challenge. Together, these data suggest a causal link between hypothalamic PSA-NCAM and the propensity for obesity. Given the role of PSA-NCAM in brain plasticity (23, 41), our findings further strengthen the concept that factors controlling neural plasticity are critical in the individual susceptibility to develop overweight with obesogenic foods (22, 42, 43). Consistently, a growing body of evidence indicates that weight gain in human is associated to genes encoding plasticity-related molecules (12, 19, 20, 44–47).
Future research is now needed to understand molecular basis of inter-individual heterogeneity in hypothalamic PSA-NCAM level and to identify life stressors that influence the expression of this factor in the hypothalamus.
Author Contributions
All authors listed have made a substantial, direct and intellectual contribution to the work, and approved it for publication.
Funding
This work was funded by CNRS, INRA (Action prioritaire BiotaBrain), the Région Bourgogne-Franche Comté (PARI and FEDER-FSE Bourgogne 2014–2020 funding's), and the ANR (ANR-13-JSV1-0003-01).
Conflict of Interest Statement
The authors declare that the research was conducted in the absence of any commercial or financial relationships that could be construed as a potential conflict of interest.
Acknowledgments
We thank the staff of the CSGA animal facility and in particular Anne Lefranc for the care to animals.
Supplementary Material
The Supplementary Material for this article can be found online at: https://www.frontiersin.org/articles/10.3389/fnut.2018.00125/full#supplementary-material
Figure S1. Acute feeding response of HFD-fed mice. (A) Average homeostatic feeding response to HFD in 2-month old male mice (C57Bl/6JOla) (n = 31; *p < 0.05, **p < 0.01, and ***p < 0.001; one-way ANOVA for repeated measures followed by multiple comparison Dunnett post-hoc test vs. day 0). (B) Graphical representation of the individual feeding responses to 1-week HFD in 2-month old male mice (C57Bl/6JOla) (n = 31). (C) Plotting of the cumulative energy intake of mice on HFD for 1 week. Median split separated HFD-tolerant mice with low feeding response (gray; n = 16) and HFD-intolerant mice with high feeding response (red; n = 15).
References
1. Hill JO, Peters JC. Environmental contributions to the obesity epidemic. Science (1998) 280:1371–4. doi: 10.1126/science.280.5368.1371
2. El-Sayed Moustafa JS, Froguel P. From obesity genetics to the future of personalized obesity therapy. Nat Rev Endocrinol. (2013) 9:402–13. doi: 10.1038/nrendo.2013.57
3. Friedman JM. A war on obesity, not the obese. Science (2003) 299:856–8. doi: 10.1126/science.1079856
4. Levin BE, Sullivan AC. Glucose-induced norepinephrine levels and obesity resistance. Am J Physiol. (1987) 253:R475–81. doi: 10.1152/ajpregu.1987.253.3.R475
5. Chang S, Graham B, Yakubu F, Lin D, Peters JC, Hill JO. Metabolic differences between obesity-prone and obesity-resistant rats. Am J Physiol. (1990) 259:R1103–10. doi: 10.1152/ajpregu.1990.259.6.R1103
6. Buchanan TA, Fisler JS, Underberger S, Sipos GF, Bray GA. Whole body insulin sensitivity in Osborne-Mendel and S 5B/Pl rats eating a low- or high-fat diet. Am J Physiol. (1992) 263:R785–9. doi: 10.1152/ajpregu.1992.263.4.R785
7. Ji H, Friedman MI. Reduced hepatocyte fatty acid oxidation in outbred rats prescreened for susceptibility to diet-induced obesity. Int J Obes. (2008) 32:1331–4. doi: 10.1038/ijo.2008.71
8. Pagliassotti MJ, Knobel SM, Shahrokhi KA, Manzo AM, Hill JO. Time course of adaptation to a high-fat diet in obesity-resistant and obesity-prone rats. Am J Physiol. (1994) 267:R659–64. doi: 10.1152/ajpregu.1994.267.3.R659
9. Dourmashkin JT, Chang GQ, Hill JO, Gayles EC, Fried SK, Leibowitz SF. Model for predicting and phenotyping at normal weight the long-term propensity for obesity in Sprague-Dawley rats. Physiol Behav. (2006) 87:666–78. doi: 10.1016/j.physbeh.2006.01.008
10. Darling JN, Ross AP, Bartness TJ, Parent MB. Predicting the effects of a high-energy diet on fatty liver and hippocampal-dependent memory in male rats. Obesity (2013) 21:910–7. doi: 10.1002/oby.20167
11. Vaanholt LM, Sinclair RE, Mitchell SE, Speakman JR. Factors influencing individual variability in high fat diet-induced weight gain in out-bred MF1 mice. Physiol Behav. (2015) 144:146–55. doi: 10.1016/j.physbeh.2015.03.029
12. Locke AE, Kahali B, Berndt SI, Justice AE, Pers TH, Day FR, et al. Genetic studies of body mass index yield new insights for obesity biology. Nature (2015) 518:197–206. doi: 10.1038/nature14177
13. Hoffmann TJ, Choquet H, Yin J, Banda Y, Kvale MN, Glymour M, et al. A large multi-ethnic genome-wide association study of adult body mass index identifies novel loci. Genetics (2018) 210:499–515. doi: 10.1534/genetics.118.301479
14. Butler AA, Marks DL, Fan W, Kuhn CM, Bartolome M, Cone RD. Melanocortin-4 receptor is required for acute homeostatic responses to increased dietary fat. Nat Neurosci. (2001) 4:605–11. doi: 10.1038/88423
15. Ellacott KL, Morton GJ, Woods SC, Tso P, Schwartz MW. Assessment of feeding behavior in laboratory mice. Cell Metab. (2010) 12:10–7. doi: 10.1016/j.cmet.2010.06.001
16. Benani A, Hryhorczuk C, Gouaze A, Fioramonti X, Brenachot X, Guissard C, et al. Food intake adaptation to dietary fat involves PSA-dependent rewiring of the arcuate melanocortin system in mice. J Neurosci. (2012) 32:11970–9. doi: 10.1523/JNEUROSCI.0624-12.2012
17. Schwartz MW, Porte DJr. Diabetes, obesity, and the brain. Science (2005) 307:375–9. doi: 10.1126/science.1104344
18. Eckel RH, Kahn SE, Ferrannini E, Goldfine AB, Nathan DM, Schwartz MW, et al. Obesity and type 2 diabetes: what can be unified and what needs to be individualized? J Clin Endocrinol Metab. (2011) 96:1654–63. doi: 10.1210/jc.2011-0585
19. Thorleifsson G, Walters GB, Gudbjartsson DF, Steinthorsdottir V, Sulem P, Helgadottir A, et al. Genome-wide association yields new sequence variants at seven loci that associate with measures of obesity. Nat Genet. (2009) 41:18–24. doi: 10.1038/ng.274
20. Willer CJ, Speliotes EK, Loos RJ, Li S, Lindgren CM, Heid IM, et al. Six new loci associated with body mass index highlight a neuronal influence on body weight regulation. Nat Genet. (2009) 41:25–34. doi: 10.1038/ng.287
21. Bouret SG, Gorski JN, Patterson CM, Chen S, Levin BE, Simerly RB. Hypothalamic neural projections are permanently disrupted in diet-induced obese rats. Cell Metab. (2008) 7:179–85. doi: 10.1016/j.cmet.2007.12.001
22. Horvath TL, Sarman B, Garcia-Caceres C, Enriori PJ, Sotonyi P, Shanabrough M, et al. Synaptic input organization of the melanocortin system predicts diet-induced hypothalamic reactive gliosis and obesity. Proc Natl Acad Sci USA. (2010) 107:14875–80. doi: 10.1073/pnas.1004282107
23. Rutishauser U. Polysialic acid in the plasticity of the developing and adult vertebrate nervous system. Nature Rev Neurosci. (2008) 9:26–35. doi: 10.1038/nrn2285
24. Brenachot X, Rigault C, Nedelec E, Laderriere A, Khanam T, Gouaze A, et al. The histone acetyltransferase MOF activates hypothalamic polysialylation to prevent diet-induced obesity in mice. Mol Metab. (2014) 3:619–29. doi: 10.1016/j.molmet.2014.05.006
25. Brenachot X, Gautier T, Nedelec E, Deckert V, Laderriere A, Nuzzaci D, et al. Brain control of plasma cholesterol involves polysialic acid molecules in the hypothalamus. Front Neurosci (2017) 11:245. doi: 10.3389/fnins.2017.00245
26. Levin BE, Dunn-Meynell AA, Balkan B, Keesey RE. Selective breeding for diet-induced obesity and resistance in Sprague-Dawley rats. Am J Physiol. (1997) 273:R725–30. doi: 10.1152/ajpregu.1997.273.2.R725
27. Perez-Echarri N, Perez-Matute P, Martinez JA, Marti A, Moreno-Aliaga MJ. Serum and gene expression levels of leptin and adiponectin in rats susceptible or resistant to diet-induced obesity. J Physiol Biochem. (2005) 61:333–42. doi: 10.1007/BF03167050
28. Rada P, Bocarsly ME, Barson JR, Hoebel BG, Leibowitz SF. Reduced accumbens dopamine in Sprague-Dawley rats prone to overeating a fat-rich diet. Physiol Behav. (2010) 101:394–400. doi: 10.1016/j.physbeh.2010.07.005
29. Barker JM, Torregrossa MM, Taylor JR. Low prefrontal PSA-NCAM confers risk for alcoholism-related behavior. Nat Neurosci. (2012) 15:1356–8. doi: 10.1038/nn.3194
30. Sandi C, Cordero MI, Merino JJ, Kruyt ND, Regan CM, Murphy KJ. Neurobiological and endocrine correlates of individual differences in spatial learning ability. Learn Mem. (2004) 11:244–52. doi: 10.1101/lm.73904
31. Sandi C, Merino JJ, Cordero MI, Touyarot K, Venero C. Effects of chronic stress on contextual fear conditioning and the hippocampal expression of the neural cell adhesion molecule, its polysialylation, and L1. Neuroscience (2001) 102:329–39. doi: 10.1016/S0306-4522(00)00484-X
32. Tsoory M, Guterman A, Richter-Levin G. Exposure to stressors during juvenility disrupts development-related alterations in the PSA-NCAM to NCAM expression ratio: potential relevance for mood and anxiety disorders. Neuropsychopharmacology (2008) 33:378–93. doi: 10.1038/sj.npp.1301397
33. Roberts JL, Whittington FM, Enser M. Effects of litter size and subsequent gold-thioglucose-induced obesity on adipose tissue weight, distribution and cellularity in male and female mice: an age study. Br J Nutr. (1988) 59:519–33. doi: 10.1079/BJN19880061
34. Voits M, Forster S, Rodel S, Voigt JP, Plagemann A, Fink H. Obesity induced by unspecific early postnatal overfeeding in male and female rats: hypophagic effect of CCK-8S. Naunyn Schmiedebergs Arch Pharmacol. (1996) 354:374–8. doi: 10.1007/BF00171071
35. Mcewen BS. Understanding the potency of stressful early life experiences on brain and body function. Metabolism (2008) 57 (Suppl. 2):S11–5. doi: 10.1016/j.metabol.2008.07.006
36. Clarke MA, Stefanidis A, Spencer SJ. Postnatal overfeeding leads to obesity and exacerbated febrile responses to lipopolysaccharide throughout life. J Neuroendocrinol. (2012) 24:511–24. doi: 10.1111/j.1365-2826.2011.02269.x
37. El Maarouf A, Kolesnikov Y, Pasternak G, Rutishauser U. Polysialic acid-induced plasticity reduces neuropathic insult to the central nervous system. Proc Natl Acad Sci USA. (2005) 102:11516–20. doi: 10.1073/pnas.0504718102
38. Lopez-Fernandez MA, Montaron MF, Varea E, Rougon G, Venero C, Abrous DN, et al. Upregulation of polysialylated neural cell adhesion molecule in the dorsal hippocampus after contextual fear conditioning is involved in long-term memory formation. J Neurosci. (2007) 27:4552–61. doi: 10.1523/JNEUROSCI.0396-07.2007
39. Markram K, Lopez Fernandez MA, Abrous DN, Sandi C. Amygdala upregulation of NCAM polysialylation induced by auditory fear conditioning is not required for memory formation, but plays a role in fear extinction. Neurobiol Learn Mem. (2007) 87:573–82. doi: 10.1016/j.nlm.2006.11.007
40. Mccall T, Weil ZM, Nacher J, Bloss EB, El Maarouf A, Rutishauser U, et al. Depletion of polysialic acid from neural cell adhesion molecule (PSA-NCAM) increases CA3 dendritic arborization and increases vulnerability to excitotoxicity. Exp Neurol. (2013) 241:5–12. doi: 10.1016/j.expneurol.2012.11.028
41. Durbec P, Cremer H. Revisiting the function of PSA-NCAM in the nervous system. Mol Neurobiol. (2001) 24:53–64. doi: 10.1385/MN:24:1-3:053
42. Dietrich MO, Horvath TL. Hypothalamic control of energy balance: insights into the role of synaptic plasticity. Trends Neurosci. (2013) 36:65–73. doi: 10.1016/j.tins.2012.12.005
43. Nuzzaci D, Laderriere A, Lemoine A, Nedelec E, Penicaud L, Rigault C, et al. Plasticity of the melanocortin system: determinants and possible consequences on food intake. Front Endocrinol (Lausanne). (2015) 6:143. doi: 10.3389/fendo.2015.00143
44. Speliotes EK, Willer CJ, Berndt SI, Monda KL, Thorleifsson G, Jackson AU, et al. Association analyses of 249,796 individuals reveal 18 new loci associated with body mass index. Nat Genet. (2010) 42:937–48. doi: 10.1038/ng.686
45. Rathjen T, Yan X, Kononenko NL, Ku MC, Song K, Ferrarese L, et al. Regulation of body weight and energy homeostasis by neuronal cell adhesion molecule 1. Nat Neurosci. (2017) 20:1096–103. doi: 10.1038/nn.4590
46. Bochukova EG, Lawler K, Croizier S, Keogh JM, Patel N, Strohbehn G, et al. A transcriptomic signature of the hypothalamic response to fasting and bdnf deficiency in prader-willi syndrome. Cell Rep. (2018) 22:3401–8. doi: 10.1016/j.celrep.2018.03.018
Keywords: food intake, obesity, maladaptive eating behavior, synaptic plasticity, PSA-NCAM, polysialylation, brain, hypothalamus
Citation: Brenachot X, Nédélec E, Ben Fradj S, Boudry G, Douard V, Laderrière A, Lemoine A, Liénard F, Nuzzaci D, Pénicaud L, Rigault C and Benani A (2018) Lack of Hypothalamus Polysialylation Inducibility Correlates With Maladaptive Eating Behaviors and Predisposition to Obesity. Front. Nutr. 5:125. doi: 10.3389/fnut.2018.00125
Received: 12 September 2018; Accepted: 26 November 2018;
Published: 10 December 2018.
Edited by:
Julie A. Chowen, Hospital Infantil Universitario Niño Jesús, SpainReviewed by:
Nicolas Chartrel, Institut National de la Santé et de la Recherche Médicale (INSERM), FranceMariano Ruiz-Gayo, CEU San Pablo University, Spain
Copyright © 2018 Brenachot, Nédélec, Ben Fradj, Boudry, Douard, Laderrière, Lemoine, Liénard, Nuzzaci, Pénicaud, Rigault and Benani. This is an open-access article distributed under the terms of the Creative Commons Attribution License (CC BY). The use, distribution or reproduction in other forums is permitted, provided the original author(s) and the copyright owner(s) are credited and that the original publication in this journal is cited, in accordance with accepted academic practice. No use, distribution or reproduction is permitted which does not comply with these terms.
*Correspondence: Alexandre Benani, alexandre.benani@u-bourgogne.fr
†These authors have contributed equally to this work
‡Present Address: Luc Pénicaud, Stromalab, CNRS, EFS, INSERM, Université de Toulouse, Toulouse, France