- 1Centre for Marine Science and Technology, Curtin University, Perth, WA, Australia
- 2Institute of Marine Sciences, University of Portsmouth, Portsmouth, United Kingdom
- 3School of Environmental Sciences, Nelson Mandela University, Port Elizabeth, South Africa
- 4Harry Butler Institute, Murdoch University, Perth, WA, Australia
- 5School of Biological and Marine Sciences, University of Plymouth, Plymouth, United Kingdom
The number of marine watercraft is on the rise—from private boats in coastal areas to commercial ships crossing oceans. A concomitant increase in underwater noise has been reported in several regions around the globe. Given the important role sound plays in the life functions of marine mammals, research on the potential effects of vessel noise has grown—in particular since the year 2000. We provide an overview of this literature, showing that studies have been patchy in terms of their coverage of species, habitats, vessel types, and types of impact investigated. The documented effects include behavioral and acoustic responses, auditory masking, and stress. We identify knowledge gaps: There appears a bias to more easily accessible species (i.e., bottlenose dolphins and humpback whales), whereas there is a paucity of literature addressing vessel noise impacts on river dolphins, even though some of these species experience chronic noise from boats. Similarly, little is known about the potential effects of ship noise on pelagic and deep-diving marine mammals, even though ship noise is focused in a downward direction, reaching great depth at little acoustic loss and potentially coupling into sound propagation channels in which sound may transmit over long ranges. We explain the fundamental concepts involved in the generation and propagation of vessel noise and point out common problems with both physics and biology: Recordings of ship noise might be affected by unidentified artifacts, and noise exposure can be both under- and over-estimated by tens of decibel if the local sound propagation conditions are not considered. The lack of anthropogenic (e.g., different vessel types), environmental (e.g., different sea states or presence/absence of prey), and biological (e.g., different demographics) controls is a common problem, as is a lack of understanding what constitutes the ‘normal’ range of behaviors. Last but not least, the biological significance of observed responses is mostly unknown. Moving forward, standards on study design, data analysis, and reporting are badly needed so that results are comparable (across space and time) and so that data can be synthesized to address the grand unknowns: the role of context and the consequences of chronic exposures.
Introduction
Marine traffic in the world’s oceans is increasing. This includes watercraft ranging from small boats to large ships. Commercial ships are increasing in number as well as size, linked to overall economic growth (United Nations Conference on Trade and Development [UNCTAD], 2018). Between World War II and 2008, the global number of ships rose by a factor 3.5 and the total gross tonnage by a factor 10 (Frisk, 2012). Based on satellite altimetry, global ship density increased by a factor 4 between 1992 and 2012, with the greatest increase in the Indian Ocean (Tournadre, 2014). Ship noise is rising concomitantly. In fact, ships have become the most ubiquitous and pervasive source of anthropogenic noise in the oceans. Ship traffic is responsible for the steady rise in ambient noise at low frequencies (10–100 Hz) in many ocean regions—a rate that has been reported to be as high as 3 dB/decade (Andrew et al., 2002, 2011; Chapman and Price, 2011; Miksis-Olds et al., 2013; Miksis-Olds and Nichols, 2016).
Concern about the potential effects of ship noise on marine mammals is not recent, but instead has been raised for decades (e.g., Payne and Webb, 1971; Myrberg, 1978; Geraci and St Aubin, 1980). As ship noise peaks in the low frequencies, early studies primarily focused on low-frequency specialist species such as mysticetes (i.e., baleen whales) (e.g., Eberhardt and Evans, 1962; Cummings and Thompson, 1971). Mysticetes produce and use sound at the frequencies emitted by large ships, and they are considered to be more sensitive at these low frequencies than are other marine mammals (e.g., Parks et al., 2007b; Cranford and Krysl, 2015). However, ships also emit significant energy at higher frequencies (tens of kHz) (e.g., Arveson and Vendittis, 2000; Hermannsen et al., 2014; Veirs et al., 2016), and so odontocetes (i.e., toothed whales, dolphins, and porpoises), which specialize in high-frequency sound usage, can also be affected (e.g., Marley et al., 2017b). Not only commercial ship traffic but also numbers of small boats have been increasing around the world. For example, the number of registered recreational vessels in the United States increased by 1% per annum between 1980 and 2017 (U.S. Department of Homeland Security, 2018). In the state of Florida, there is approximately one registered recreational boat per 17 people (Sidman and Fik, 2005). Similarly, parts of Australia saw increases of 3% per annum between 1999 and 2009 (Nsw Government Maritime, 2010). In Sydney Harbour, 70% of overall vessel traffic is comprised of recreational boats (Widmer and Underwood, 2004). Noise from small boats peaks at higher frequencies (e.g., Erbe, 2013; Erbe et al., 2016b) at which coastal odontocetes are more sensitive (e.g., Houser and Finneran, 2006).
The noise field around a boat or ship is not isotropic (i.e., it is not the same in all directions; e.g., Arveson and Vendittis, 2000). It depends on source frequency and the environment in which the vessel travels, and it changes with vessel speed, load, size, and other factors (e.g., Ross, 1976; Urick, 1983). Consequently, it is not straightforward to translate acoustic recordings made in one environment to others. Obtaining quality recordings of watercraft noise is a science of its own, with numerous flaws that are commonly unrecognized in the literature.
Similarly, determining the responses of marine mammals to watercraft noise has numerous challenges, including constraints in experimental design; variability in species-, population-, and individual-specific characteristics and responses; and context-specific factors that may need to be considered. For example, many studies suffer bias from observer presence in that the majority of marine mammal studies are, by necessity, vessel-based. This introduces a potential source of bias from the presence of the research vessel, as well as the noise it creates. Furthermore, many studies struggle to differentiate between the effects of vessel presence and vessel noise, resulting in confounding explanatory variables. Even if researchers can be confident in noise as the source of disturbance, measurements are often inconsistent between studies, thus complicating comparisons. Animal behavioral responses can also take many forms. Due to the challenges associated with studying these fast-moving, far-ranging, often-submerged animals, the majority of marine mammal behavioral response studies in the wild concentrate on visible changes to physical behavior at the sea surface, such as changes in occurrence or cessation of certain activities. Far fewer consider a combination of behavioral changes, including acoustical behaviors. The resulting knowledge gaps, biases, and uncertainties may be minimized by standardization and interdisciplinary cooperation.
In fact, the effects of watercraft noise on marine mammals is an interdisciplinary field: Sound generation, propagation, measurement, and modeling are physics problems, yet monitoring animals, determining impacts, and understanding biological significance are biological problems. Misinformation and miscommunication have led to numerous issues with underwater acoustic quantities, units, recording and reporting, as well as experimental design, statistical analysis, and interpretation. This review provides an overview of the field of watercraft noise impacts on marine mammals, explains the fundamental physical and biological concepts, highlights common issues and problems, identifies data gaps, and discusses research needs.
Generation and Propagation of Watercraft Noise
There is a large variety of motorized boats and ships, such as recreational boats, passenger and car ferries, high-speed hovercraft, cruise ships, tug boats, dredges, dry and liquid cargo vessels, fishing vessels, oil and gas production platforms, research vessels, naval ships, submarines, etc. All of these produce noise. Source levels1 of 130–160 dB re 1 μPa m have been reported for small watercraft such as jetskis and rigid-hulled inflatable boats (Erbe, 2013; Erbe et al., 2016b). Large and powerful watercraft such as ferries, container ships, and icebreakers have source levels of 200 dB re 1 μPa m and more (e.g., Erbe and Farmer, 2000; Simard et al., 2016; Gassmann et al., 2017). Source levels may vary by 20–40 dB within a ship class due to variability in design, maintenance, and operational parameters such as speed (Simard et al., 2016; Joy et al., 2019).
The strongest noise source is typically the propeller when it cavitates (Ross, 1976). Propeller cavitation involves the formation of bubble clouds behind the propeller. Bubbles of all sizes are created, then grow, vibrate and collapse, producing an overall broadband noise spectrum that ranges from a few Hz to over 100 kHz (Ross, 1976). Traveling at low speed and/or great depth (hence pressure; e.g., submarines) can reduce and avoid propeller cavitation noise. Cavitation noise increases with vessel speed, size, and load (e.g., Ross, 1976; Urick, 1983; Scrimger and Heitmeyer, 1991; Hamson, 1997; Trevorrow et al., 2008; Simard et al., 2016). Cavitation noise is typically amplitude-modulated by the propeller blade rate (i.e., the number of propeller blades times the number of rotations per second; Ross, 1976). ‘Propeller singing’ refers to narrow-band noise that is a result of vibrating propeller blades. The engine and any machinery onboard a ship also produce noise, and this may couple well into the water through the ship’s hull (Urick, 1983). The engine generates narrow-band noise consisting of the engine firing-rate plus overtones (Arveson and Vendittis, 2000). Furthermore, hydrodynamic flow past the hull can lead to vibration of appendages or cavities generating additional narrow-band noise (Urick, 1983). Overall, the noise spectrum emitted by a ship may have multiple sources that contribute noise from different locations about the ship, at different frequencies and into different directions—leading to a complicated and dynamic noise field.
The noise field varies with frequency and angle about a vessel (Arveson and Vendittis, 2000; Trevorrow et al., 2008; Gassmann et al., 2017). Given that boats and ships operate at the water surface and the propeller sits, at maximum, a few meters below the surface, emitted noise reflects at the water surface leading to a strongly downward-directed noise emission pattern (e.g., Gassmann et al., 2017). In physical terms, the source of the watercraft noise and its image source (in air) create a dipole radiation pattern. This means that watercraft noise radiates very well to great depth in the ocean. Radiation in the horizontal plane, near the sea surface, is greatly reduced because of destructive interference of the image source with the real source (i.e., the Lloyd’s mirror effect; note that the interference pattern is frequency-dependent). In addition, the hull may shield sound propagation from the propeller in the forward direction. These acoustic radiation phenomena might explain why marine mammals that spend a lot of time at the water surface are prone to vessel strike (e.g., right whales and sirenians) and why bow-riding marine mammals (Würsig, 2018) are not disturbed by the vessel’s noise (Gerstein et al., 2005).
As a vessel travels through different environments, from coastal to offshore waters, its noise field changes. In shallow water, the propagating noise repeatedly interacts with the water surface and seafloor, where it is reflected, scattered, and partly absorbed (e.g., Cole and Podeszwa, 1967). The directionality of the noise field is highly variable. In deep water, the directionality is dipolar (i.e., strongly downward) and interactions with, and hence acoustic energy losses at, the seafloor and sea surface are reduced. The noise from watercraft traveling in deep water easily couples into the deep sound channel [i.e., the so-called Sound Fixing And Ranging (SOFAR) channel; e.g., Williams and Horne, 1967; Shockley et al., 1982], where it can traverse entire oceans with very little acoustic energy loss. The noise from watercraft traveling over sloping bathymetry (such as the continental slope) can enter the SOFAR channel with just one seafloor reflection (Figure 1). Animals in coastal versus offshore waters or at low versus great depth may experience quite different noise fields—even at the same range from the same vessel.
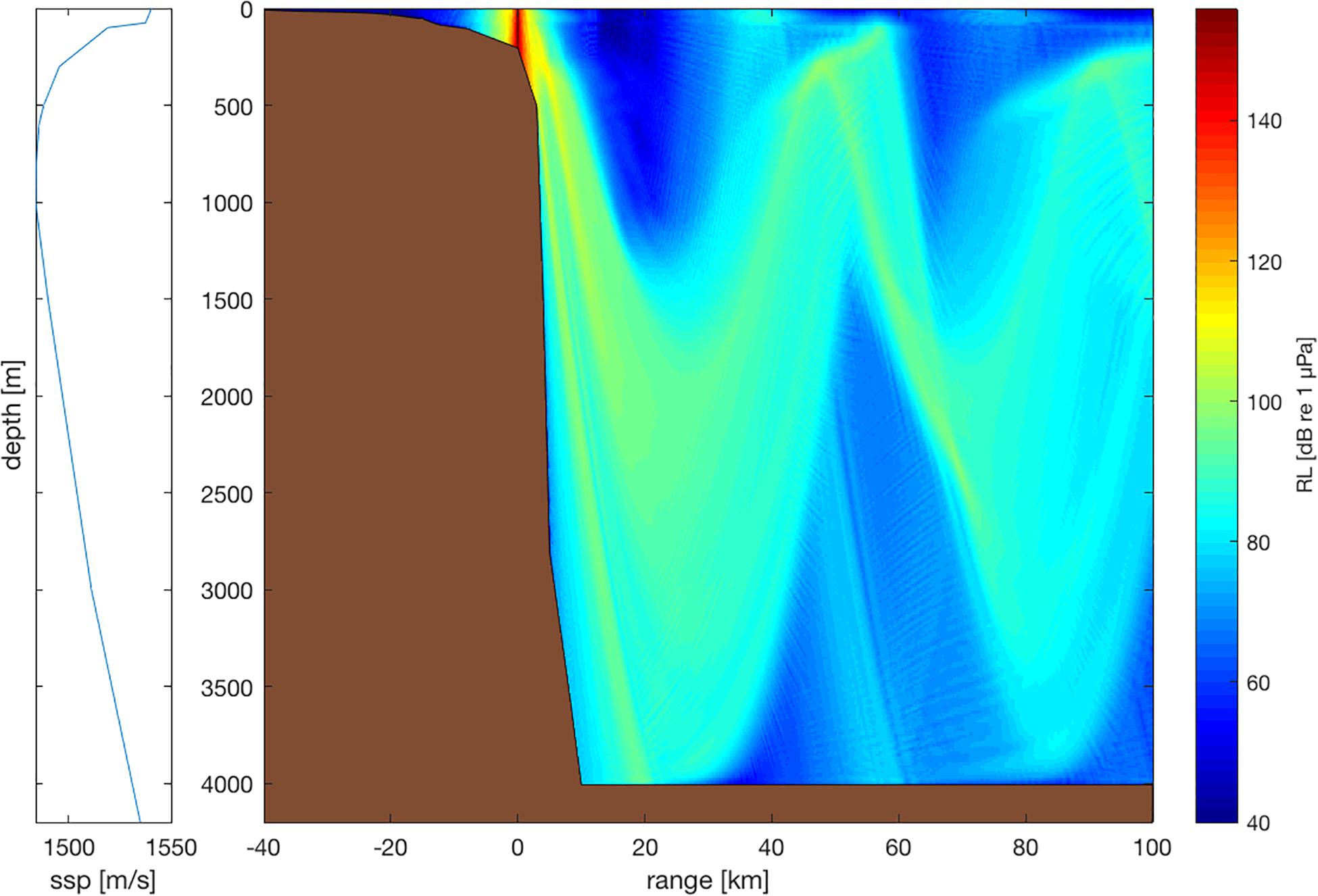
Figure 1. Sketch of the noise field of a cruise ship at the continental slope [at location (0| 0)]. Source spectrum representative of vessel class ‘L5’ (length: 156 m, speed: 15 knot, source level: 191 dB re 1 μPa m; Erbe et al., 2012). Propagation loss model: RAMGeo in AcTUP V2.8 (https://cmst.curtin.edu.au/products/underwater/). Equatorial sound speed profile (ssp; left panel; taken from Shockley et al., 1982). Seafloor modeled as hard, dense limestone (Hamilton, 1980). No ambient noise is included in this plot. Broadband received levels (RL) are color-coded using the scale in the right panel. The dipole radiation pattern (i.e., most energy radiating downward) is clearly visible. While sound energy propagates poorly into shallow water (with received levels rapidly decreasing with increasing range), it propagates well (i.e., with little loss) into deep water.
Impacts of Watercraft Noise on Marine Mammals
The effects of underwater noise from anthropogenic activities on marine mammals have been summarized in several works and include the following: behavioral responses, acoustic interference (i.e., masking), temporary or permanent shifts in hearing threshold (TTS, PTS), and stress (e.g., Richardson et al., 1995; Nowacek et al., 2007; Erbe et al., 2018). Acute effects on individual animals are more easily observed, more frequently published, and hence better understood than long-term effects on populations from chronic exposures. Watercrafts are the primary source of chronic noise exposures on marine mammals.
We set out to review the effects of watercraft noise on marine mammals by compiling the literature from a Web of Science search2, augmented by our personal libraries. The following criteria had to be met for articles to be included in the review. Studies:
• must have dealt with marine vessels;
• must have dealt with marine mammals in water (hence excluding hauled-out pinnipeds);
• may have focused on one or the other;
• must have measured, observed, modeled, or estimated responses (i.e., articles that addressed the potential effects of vessel noise only in the Discussion were excluded); and
• did not need to have measured or modeled source levels or received levels of noise.
A total of 154 articles were included in this review. A rapid growth in the number of publications has occurred since the year 2000 (Figure 2). Forty-seven marine mammal species have been studied. The most studied species are the bottlenose dolphin (Tursiops truncatus), humpback whale (Megaptera novaeangliae), and then beluga whale (Delphinapterus leucas) (Figure 3). Figure 4 maps the different study sites by species.
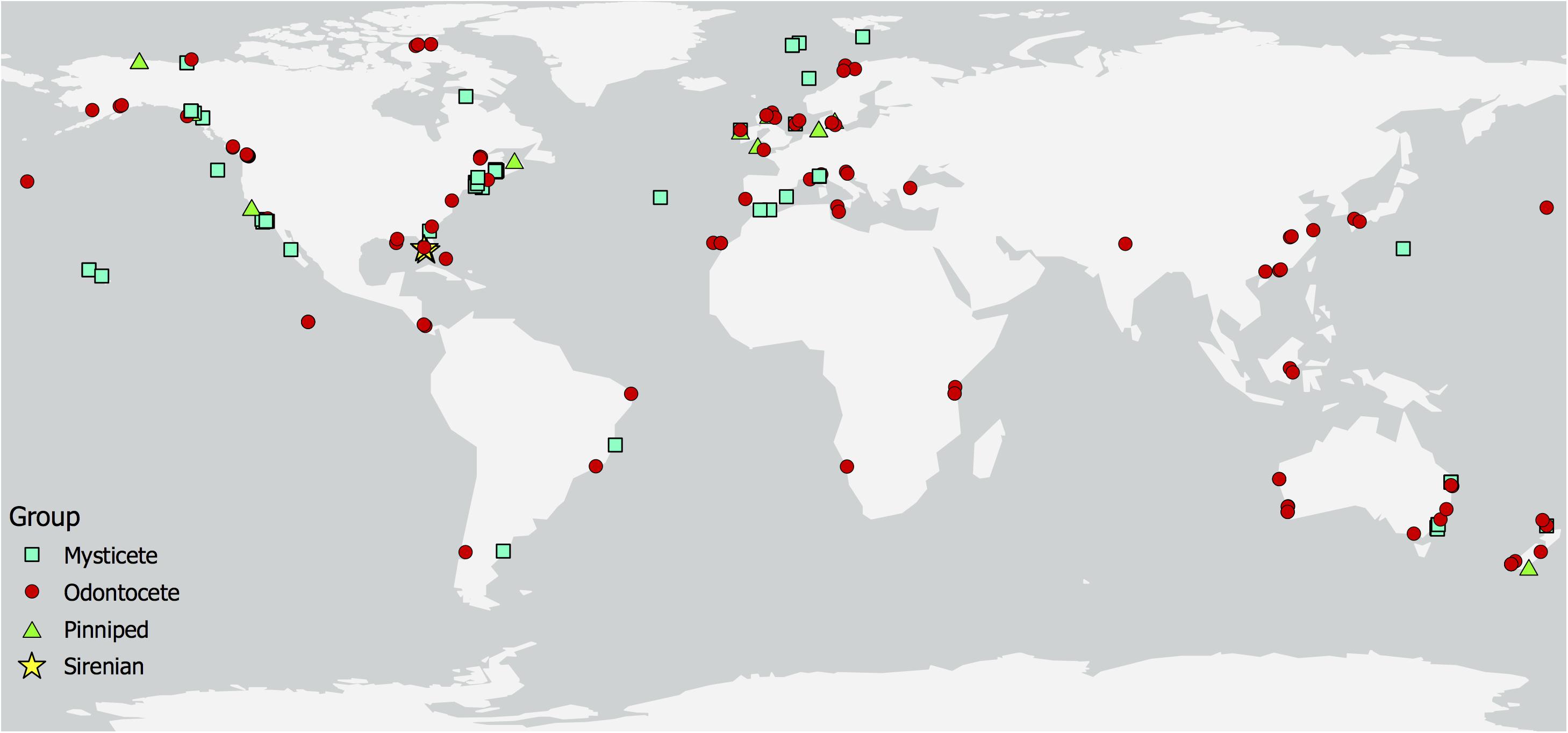
Figure 4. World-map showing locations where the effects of watercraft noise on marine mammals have been studied.
The reported effects of boat or ship noise on marine mammals include changes in both physical and acoustic behavior, masking of communication and echolocation sounds, and stress.
Supplementary Table S1 lists the articles we reviewed and provides information on the following: the types of vessels and marine mammal species studied; the study location, objectives, design, and methodology; and the animal responses observed or modeled. Several interesting patterns are revealed, which are presented in the following sections, along with discussions of the key findings for particular species groups. Additionally, a number of common issues and problems are identified, which highlight research needs.
Mysticetes
In the early 1980s, concern about the effects of shipping and hydrocarbon development in the Arctic led to several multi-year studies on underwater noise effects on bowhead whales (Balaena mysticetus; e.g., Richardson et al., 1982; Greene, 1985; Richardson et al., 1985; Johnson et al., 1986). In these studies, experimental approaches of bowhead whales by small vessels at high speed showed that whales generally moved away, thereby interrupting foraging, socializing, and playing behavior, while spending less time at the surface. The early 1980s also saw the first and only playback experiment on the response to vessel noise by gray whales (Eschrichtius robustus) in their breeding and nursery habitat off Mexico (Dahlheim, 1987; Dahlheim and Castellote, 2016). Gray whales have a limited repertoire of low-frequency (40–4000 Hz) vocalizations, overlapping with watercraft noise (Dahlheim et al., 1984; Moore and Ljungblad, 1984; Dahlheim and Castellote, 2016; Burnham et al., 2018). In the presence of ships and boats, gray whales increased their vocalization rate, and at times of increased outboard engine noise, received levels from gray whales were higher (interpreted as an increase in source levels; Dahlheim, 1987; Dahlheim and Castellote, 2016).
An increase in studies on the potential effects of vessel noise on a wider range of mysticete species has occurred in recent years. The most extensively studied species is the humpback whale. Humpback whales in Glacier Bay National Park, AK, United States of America, are prone to high noise exposures from tourism vessels and have been shown to increase the amplitude of their vocalizations by 0.8 dB for every 1.0 dB increase in ambient noise, while vocalizing less frequently (Frankel and Gabriele, 2017; Fournet et al., 2018). Similarly, singing individuals near Chichi-jima Island ceased their song after a passenger-cargo vessel passed within 1400 m (Tsujii et al., 2018). Humpback whales off the Australian east coast exhibited great variation in behavioral responses to seismic survey vessels with the airguns turned off. While no behavioral change was seen in some trials, others revealed a decrease in dive duration, travel speed, and the number of breaches (Dunlop et al., 2015, 2016, 2017a,b, 2018). Most humpback whales did not respond to sonar vessels with the sonar turned off (Sivle et al., 2016; Wensveen et al., 2017). Tsujii et al. (2018) found that humpback whales moved away from large vessels, while others noted changes in respiratory behavior (Baker and Herman, 1989; Frankel and Clark, 2002) and a cessation of foraging activities (Blair et al., 2016). The large number of studies on humpback whales and the resulting variety of documented responses demonstrate that context affects behavior.
Conversely, North Atlantic right whales (Eubalaena glacialis) show no behavioral response to ship noise at all, or at least not to received levels of 132–142 dB re 1 μPa rms from large ships passing within 1 nm distance, nor to received levels of 129–139 dB re 1 μPa rms (main energy between 50 and 500 Hz) from ship noise playback (Nowacek D.P. et al., 2004). A lack of behavioral response of right whales to ship noise is particularly concerning due to the high levels of ship strike in this species (Laist et al., 2001), affecting their conservation status (Kraus et al., 2005). Nevertheless, analyses of North Atlantic right whale fecal samples suggested that noise from large commercial vessels might increase stress levels (Rolland et al., 2012). In addition, studies suggest that right whales have vocally adapted to environments with increased low-frequency noise through a shift in vocalization frequency and duration (Parks et al., 2007a, 2009, 2011), which may have been a response to compensate for a loss in communication range (Clark et al., 2009). Tennessen and Parks (2016) modeled the communication space of mother-calf pair up-calls in the vicinity of container vessels and found that an up-call would only be detected when the receiving whale was 25 km from the moving vessel and within 320 m of the transmitting whale. Another important social call for right whales, the gunshot, was also found susceptible to masking by vessel noise (Cunningham and Mountain, 2014).
A decrease in communication range as a result of increased levels of ship noise has also been modeled for Bryde’s (Balaenoptera edeni), fin (Balaenoptera physalus), humpback, and minke whales (Balaenoptera acutorostrata) (Clark et al., 2009; Cholewiak et al., 2018; Gabriele et al., 2018; Putland et al., 2018). The Lombard effect comprises changes in the spectral features of vocalizations (i.e., in frequency and level) and in vocalization rates, in order to compensate for masking (Lombard, 1911). In addition to the examples from gray, humpback, and right whales above, fin whales lowered the bandwidth, peak frequency, and center frequency of their vocalizations under increased levels of background noise from large vessels (Castellote et al., 2012).
Less attention has been paid to the effects of noise generated by smaller vessels. Dunlop (2016b) predicted an increase in humpback whale social call source levels and the proportion of surface-generated sounds under increased vessel noise, as observed in response to increased wind noise. However, no behavioral changes were observed at received levels of 91–124 dB re 1 μPa rms from a recreational fishing vessel. Au and Green (2000) studied the response of humpback whales to four different whale-watching vessels, each with their own acoustic signature, approaching to 91 m distance. Individual whales responded strongest (i.e., abrupt changes in direction and longer dive durations) to the vessel with the highest received level (127 dB re 1 μPa, 1/3 octave band level at 315 Hz). Several other studies report on the behavioral responses of mysticete whales to smaller vessels in the absence of noise measurements. These studies indicate avoidance of vessels at close range (Palka and Hammond, 2001; Stamation et al., 2010). Changes in behavioral state and respiratory behavior were also observed (Jahoda et al., 2003; Morete et al., 2007), with mother-calf pairs eliciting stronger responses than adults (Morete et al., 2007).
Odontocetes
Much of the significant early work on the potential effects of watercraft noise on odontocetes was—similar to studies on mysticetes—a result of concern about Arctic industrial development (hydrocarbons, mining, and shipping) in the early 1980s (e.g., LGL Ltd., 1986; Finley et al., 1990; Richardson et al., 1990). The focal species were beluga whales and narwhals (Monodon monoceros). In response to icebreakers, beluga whales lost pod integrity, commenced rapid movement, asynchronous and shallow dives, and changed their vocal behavior (i.e., vocalization types) at received levels of 94–105 dB re 1 μPa rms (20–1000 Hz), while narwhals changed their locomotion (i.e., exhibited more directed and slower movement, became motionless, and sank) and fell silent at received levels of about 124 dB re 1 μPa rms (20–1000 Hz) (LGL Ltd., 1986; Cosens and Dueck, 1988; Finley et al., 1990). Since the 1990s, beluga whale responses to boats and ships have been studied more extensively in the St. Lawrence Estuary, Canada. Here, beluga whales have shown increasing avoidance (i.e., increased dive duration and swim speed) with the number of boats, as well as other changes in both physical and acoustic behavior (Blane and Jaakson, 1994; Lesage et al., 1999). The Lombard effect has been demonstrated as an increase in source level, vocalization rate, and frequency (i.e., shift to higher frequencies; Lesage et al., 1999; Scheifele et al., 2005).
In the case of beaked whales, much effort has been spent on understanding the potential effects of ship-based sonar transmissions given coincident strandings and naval exercises (e.g., DeRuiter et al., 2013; Goldbogen et al., 2013; Sivle et al., 2015; Kvadsheim et al., 2017). The effects of ship noise without sonars have been investigated less. Using passive acoustic monitoring and acoustic tags, ship noise at received levels of approximately 135 dB re 1 μPa rms (0.1–45 kHz) affected beaked whale foraging by reducing both the horizontal area in which animals foraged and the number of successful prey captures (as indicated by the number of feeding buzzes recorded), with foraging efficiency reduced by >50% (Aguilar Soto et al., 2006; Pirotta et al., 2012). Similarly, fewer clicks were recorded of sperm whales (Physeter macrocephalus) during vessel passes (Azzara et al., 2013), and decreases in surface time, respiration interval, and the number of ventilations were reported in the presence of whale-watching boats (Gordon et al., 1992). A different study found no decrease of sperm whale acoustic detections in ship noise (André et al., 2017). Rather, an increase in sperm whale acoustic and visual detections was found near longline fishing vessels, and propeller cavitation noise (to be exact, changes in that noise corresponding to typical operational changes in longline fishing vessel speeds) was identified as the ‘dinner bell’ attracting sperm whales to depredate (Thode et al., 2007). Such diverse responses (avoidance, no response, and attraction) highlight the importance of context in assessments of underwater noise.
Killer whales (Orcinus orca) in British Columbia and Washington State have recently received much attention with regards to impacts from ships, given the steady decline in their population size. Changes in behavior (i.e., less foraging and increased surface-active behavior), respiration, and swim speed and direction occurred at received levels above 130 dB re 1 μPa rms (0.01–50 kHz), and the Lombard effect (i.e., increased source level and vocalization duration) has been reported in ship noise levels above 98 dB re 1 μPa rms (1–40 kHz) (Foote et al., 2004; Holt et al., 2009, 2011; Lusseau et al., 2009; Noren et al., 2009; Williams et al., 2002, 2014). This geographic area has seen a lot of ship noise recording, quantification, and impact modeling studies (e.g., Erbe, 2002; Erbe et al., 2012, 2014; Williams et al., 2015; Cominelli et al., 2018; Joy et al., 2019).
A great deal of research has also focused upon smaller delphinids. Occupying habitats from freshwater rivers to coastal estuaries and the open ocean, dolphins often experience high habitat overlap with human activities. In particular, the potential impacts from dolphin-watching tourism vessels have been investigated (e.g., Scarpaci et al., 2000; Lusseau, 2003a, 2005, 2006; Constantine et al., 2004; Lusseau and Higham, 2004; Bejder et al., 2006; Stensland and Berggren, 2007; Arcangeli and Crosti, 2009; Christiansen et al., 2010; Steckenreuter et al., 2012; Guerra et al., 2014; May-Collado and Quinones-Lebron, 2014; Symons et al., 2014; Heiler et al., 2016; Pérez-Jorge et al., 2016). Dolphins were displaced or changed their site occupancy in response to vessel traffic (Lusseau, 2005; Bejder et al., 2006; Rako et al., 2013; Pirotta et al., 2015b; Pérez-Jorge et al., 2016). They altered their movement patterns within an area in response to vessel traffic, with animals changing their direction of travel, beginning to travel erratically, or significantly increasing traveling speeds when approached by vessels (Au and Perryman, 1982; Nowacek et al., 2001; Mattson et al., 2005; Lemon et al., 2006; Lusseau, 2006; Christiansen et al., 2010; Marley et al., 2017b). Watercrafts can cause a shift in dolphin behavioral budgets, generally increasing time spent traveling whilst decreasing time spent resting and socializing (Lusseau, 2003a; Constantine et al., 2004; Stensland and Berggren, 2007; Arcangeli and Crosti, 2009; Steckenreuter et al., 2012; Marley et al., 2017b). Other changes in behavior can include alterations to dive patterns, displays of breathing synchrony, and changes in inter-animal distances (Janik and Thompson, 1996; Nowacek et al., 2001; Hastie et al., 2003; Kreb and Rahadi, 2004; Stensland and Berggren, 2007). Furthermore, dolphins have been observed to alter their whistle characteristics, such as their frequency range, in elevated noise conditions or in the presence of vessels (Morisaka et al., 2005; May-Collado and Wartzok, 2008; Guerra et al., 2014; May-Collado and Quinones-Lebron, 2014; Papale et al., 2015; Heiler et al., 2016; Rako Gospić and Picciulin, 2016; Marley et al., 2017b). Changes to whistle duration have also been reported (May-Collado and Wartzok, 2008; Guerra et al., 2014; May-Collado and Quinones-Lebron, 2014), as have increases in whistle production rates (Scarpaci et al., 2000; Van Parijs and Corkeron, 2001; Buckstaff, 2004; Guerra et al., 2014; Martins et al., 2018).
However, delphinid studies are heavily biased toward particular species, with some receiving considerably more research attention than others. The bottlenose dolphin (Tursiops spp.) has been the focus of the most research effort of all the odontocetes. Bottlenose dolphins have a cosmopolitan distribution, ranging from northern Scotland to southern New Zealand and occupying both coastal and pelagic habitats. As a result, they are available to marine mammalogists around the world, and so dominate the literature. Bottlenose dolphins are also the most common cetacean kept in captivity, which has facilitated a range of physiological studies regarding the impacts of noise that have not been possible for other species; e.g., studies on how behavioral and acoustical changes affect energetics. Dolphin metabolic rates increase during periods of vocal effort and sound production, with energy requirements varying according to the type of sound produced (Noren et al., 2013; Holt et al., 2015, 2016). This combined with increased energy expenditure due to more time spent traveling, moving at speed, avoiding vessels, or leaving impacted areas, results in disturbance having potential cumulative energetic consequences.
Conversely, little is known about the responses of dolphin species that inhabit relatively constrained systems that are also some of the world’s busiest waterways. The river systems utilized by these species are known to have high levels of vessel traffic and, in some cases, there is evidence of river dolphins being the target of tourism activities (e.g., boto, Inia geoffrensis, in Brazil; de Sá Alves et al., 2012). Ganges river dolphins (Platanista gangetica gangetica) showed mixed responses to approaching vessels, including changing direction to orient away from the boat, prolonging dive times, and displaying attraction toward the boat, as well as no obvious effect (Bashir et al., 2013). Such variability, again, shows the importance of context in behavioral responses. Finally, there is a clear paucity of publications addressing the responses of river dolphins (Families Iniidae, Platanistidae, Pontoporiidae, and Lipotidae) to vessel traffic or noise.
Similarly, of the porpoise species, only harbor porpoises (Phocoena phocoena) and finless porpoises (Indo-Pacific, Neophocaena phocaenoides; Yangtze, N. asiaeorientalis asiaeorientalis) have been studied with regards to the impact of watercraft. Harbor porpoises moved away from vessels (Palka and Hammond, 2001), showed higher levels of porpoising in the presence of boats (Dyndo et al., 2015), changed behavioral states (Akkaya Bas et al., 2017), reduced foraging behavior (Wisniewska et al., 2018), and experienced decreased communication ranges (Hermannsen et al., 2014). Acoustic tags (DTAGs) placed on harbor porpoises in Danish waters showed that animals encountered vessel noise 17–89% of the time, and exhibited vigorous fluking, bottom diving, interrupted foraging, and cessation of echolocation during some high vessel noise events (received level > 96 dB re 1 μPa at 16 kHz 1/3 octave band; Wisniewska et al., 2018). Meanwhile the Yangtze finless porpoise has been shown to forage in busy (port) areas exhibiting high vessel traffic, with no detected impact on echolocation behavior (Dong et al., 2012; Wang et al., 2014). Wang et al. (2015) proposed that the high prey densities in the ports in comparison to surrounding areas mean porpoises need to forage there regardless of boat traffic. The closely related Indo-Pacific finless porpoise appears not to exhibit the same pattern, with echolocation behavior showing a negative correlation with ship traffic (Akamatsu et al., 2008). Porpoises may be more vulnerable to this type of disturbance due to their small size and low fat reserves, such that any disturbance that reduces foraging opportunities may result in negative fitness consequences (Nabe-Nielsen et al., 2014; Wisniewska et al., 2016).
Sirenians
Knowledge about the potential effects of watercraft noise on sirenians grew from curiosity of why these animals did not avoid approaching boats and whether they perhaps could not hear them. Fatal collision with watercraft is a serious problem that has been recognized since the 1970s (Ackerman et al., 1992; O’Shea, 1995; Marsh et al., 2001; Rycyk et al., 2018). The majority of these fatalities are a result of blunt force trauma rather than propeller cuts (Lightsey et al., 2006). Vessel strike is the main source of mortality for some populations (e.g., 25% of all Florida manatee, Trichechus manatus latirostris, deaths; Calleson and Kipp Frohlich, 2007). Consequently, an understanding of the hearing capabilities of sirenians has been of interest to determine the capabilities of sirenians to detect watercraft noise. There are no data for dugong (Dugong dugon); however, manatee hearing underwater is sensitive at 1–30 kHz (Klishin et al., 1990; Popov and Supin, 1990; Gerstein et al., 1999; Gaspard et al., 2012). This overlaps with the spectrum of noise from boats, raising the question of why manatees do not manage to avoid a vessel strike. The current hypothesis is that, as they spend a great deal of time very close to the sea surface, received noise levels from watercraft are low due to the Lloyd’s mirror effect and less sound radiation toward the bow. This, combined with manatees’ relatively low movement speed, leaves manatees vulnerable to vessel strikes (e.g., Gerstein et al., 1999).
Conversely, some behavioral studies have concluded that manatees (Trichechus spp.) are able to detect and respond to approaching boats, often changing their orientation (heading or roll), depth, diving behavior, behavioral state, and swimming speed (Nowacek S.M. et al., 2004; Miksis-Olds et al., 2007b; Rycyk et al., 2018). Such responses to vessels were more pronounced for vessels in close proximity and traveling at speed (Nowacek S.M. et al., 2004). Dugongs were also affected by close boat approaches and less likely to continue feeding when vessels traveled within 50 m (Hodgson and Marsh, 2007). Manatees foraged in habitat with lower ambient noise (that included vessel noise below 1 kHz), particularly at times with less boat density (Miksis-Olds et al., 2007a). Playback experiments simulating different boats at different speeds approaching to within 10 m supported earlier behavioral response studies that manatees swam to deeper waters in the presence of boat noise (Miksis-Olds et al., 2007b).
Pinnipeds
Pinnipeds are amphibious and haul out on land or ice to breed, pup, molt, and rest. Consequently, much of the research examining vessel traffic has focused on the easily observable reactions of hauled-out pinnipeds to approaching boats and ships. This includes the haul-out behavior of harbor seals (Phoca vitulina) (Andersen et al., 2012; Blundell and Pendleton, 2015), Australian fur seals (Arctocephalus pusillus doriferus) (Stafford-Bell et al., 2012), Saimaa ringed seals (Phoca hispida saimensis) (Niemi et al., 2013), Australian sea lions (Neophoca cinerea) (Osterrieder et al., 2017), and walrus (Odobenus rosmarus) (Øren et al., 2018). A small number of studies also extend observations to the water surrounding haul-out sites (Osterrieder et al., 2017). Common reactions of pinnipeds to approaching vessels include flushing off haul-out sites into the sea (Jansen et al., 2010; Andersen et al., 2012; Blundell and Pendleton, 2015), increased alertness (Henry and Hammill, 2001), and head raising (Niemi et al., 2013). However, these studies focused on the reactions of pinnipeds to the presence of a vessel rather than perceived levels of vessel noise. Studies that incorporate in-air noise generation, transmission, and reception are very rare (Tripovich et al., 2012). In-air watercraft noise and the perception of sound in air are notably different from their underwater equivalents (Kastak and Schusterman, 1998). Therefore, the remainder of this section and Supplementary Table S1 focus on studies investigating the impacts of underwater watercraft noise on pinnipeds.
Underwater noise from watercraft has the potential to mask or alter the communication of pinnipeds. Bagočius (2014) showed that gray seal (Halichoerus grypus) vocalizations recorded underwater in captivity overlapped with the noise spectrum of a vehicle/passenger ship. Terhune et al. (1979) reported a decrease in the loudness of underwater harp seal (Pagophilus groenlandicus) vocalizations after the presence of a vessel was recorded acoustically near whelping sites in the Gulf of St. Lawrence. This may have reflected a change in seal vocalizations or the movement of seals away from the recording area (Terhune et al., 1979).
Studies on the behavioral responses of pinnipeds to shipping noise have been undertaken at a range of spatial scales. A national-scale assessment of seals and shipping in the United Kingdom showed high rates of co-occurrence between gray seals or harbor seals and shipping traffic within 50 km of the coastline near haul-out sites (Jones et al., 2017). At regional and local scales, it was estimated, using sound propagation models, that harbor seals in the Moray Firth were exposed to 24-h cumulative SEL3 between 170 dB re 1 μPa2s (95% CI 168–172) and 189 dB re 1 μPa2s (95% CI 173–206) from shipping (Jones et al., 2017). When considering the upper limits of the 95% confidence intervals, these predicted values exceeded the estimated thresholds for the onset of TTS (Southall et al., 2007, 2019). Locally in Broadhaven Bay, Ireland, gray seals potentially varied habitat use in response to vessels as indicated by a negative correlation between the numbers of gray seals and construction vessels (Anderwald et al., 2013). A recent study using acoustic tags (DTAGs) that record sound and behavior concurrently showed that harbor and gray seals were exposed to vessel noise 2.2–20.5% of their time at sea (Mikkelsen et al., 2019). In response to vessel noise, a tagged seal changed its diving behavior, switching quickly from a dive ascent to descent (Mikkelsen et al., 2019). This observation agrees with descriptions of changes in diving reported during the development of early acoustic recording tags on juvenile northern elephant seals (Mirounga angustirostris) (Fletcher et al., 1996; Burgess et al., 1998). Studies using acoustic recording tags on pinnipeds demonstrate the potential opportunities, and the need, to further explore the impact of shipping noise on the at-sea behavior of pinnipeds.
Approaches to Study Design
In order to compare studies, identify focus areas and research gaps, and point out common issues and problems, we defined a ‘study’ as a unique combination of publication reference and species. For example, if a publication dealt with two species, then this was counted as two studies. However, if a publication investigated the same species at two different sites, then this was counted as one study.
With this definition, an approximately equal number of studies dealt with large ships as with small boats (ratio: 1.05:1). Animal responses to these vessels were observed in the wild in 82% of studies, while 4% of studies were done in captivity and 14% of studies used models instead of live animals. The majority of studies on live animals dealt with real vessels in situ, while 5% were playback studies of pre-recorded sound.
In terms of measuring animal responses, 34% of studies undertook vessel-based observations, 19% land-based observations, and 8% aerial observations. Passive Acoustic Monitoring (PAM) was employed in 33% of studies, and tags were used in 13% of studies. Some studies used more than one method of observation. Studies were designed as controlled exposure experiments (14%) or before-during-after observations (29%), while 21% were opportunistic in nature.
Out of all studies, 28% determined the received noise level at the study animals, 13% measured the received level, 12% used a sound propagation model to determine the received level, and 3% applied a geometric propagation loss. In addition to determining the received level, 15% of studies also considered frequency-dependent hearing sensitivity of the animals (e.g., audiograms or critical bands). A total of 41% of studies neither estimated the received level nor the range of the vessel to the animals.
In terms of context, 58% of studies considered vessel-related factors such as vessel numbers, types, speeds, distances, directions of approach, etc. Environmental factors such as location, habitat type, bathymetry, tide, sea state, temperature, prey presence, and ambient noise (in addition to vessel noise) were considered by 42% of studies. Biological factors such as group demographics, behavioral state, speed of movement etc., were considered by 46% of studies. Only 17% of studies did not consider any contextual variables. However, the majority used only very few and basic contextual variables such as range to the vessel, ambient noise, and current behavioral state.
Common Issues and Problems
With Physics: Estimation of Exposure, Recording, and Playback of Vessel Noise
Studies on the effects of watercraft noise on marine mammals would ideally be able to determine the sound levels received by the animals and the total sound exposure (i.e., the integral of the squared sound pressure over time; International Organization for Standardization, 2017). Few studies employed acoustic recording tags on the animals, which store a record of received levels over time right at the animal. The majority of studies that determined received levels did so by modeling and estimation. In this case, watercrafts are recorded at some site, source levels are estimated, and these estimates are then applied to mostly different situations (i.e., locations, environments, and times of year) for the computation of received levels. There are common problems with all of these steps.
Measuring ship noise is not as simple as lowering a hydrophone over the side of a boat. Over-the-side deployments as well as hydrophones suspended straight from surface buoys may record noise from wave action against the boat or buoy, and show artifacts from the hydrophone moving through the water with the waves, affecting acoustic recordings at frequencies from a few Hz to a few kHz (e.g., Strasberg, 1979; Cato, 2008; Erbe et al., 2016c). Common in moored deployments, flow noise is an artifact of recording resulting from hydrodynamic flow past the hydrophone, which causes non-acoustic pressure fluctuations at approximately 0.005–1 kHz range (e.g., Buck and Greene, 1980; Erbe et al., 2015). Strong currents might set mooring ropes and legs into vibration and resonance, causing mooring noise at a few hundred Hz to a few kHz (e.g., Koper et al., 2016). Metal chains and shackles in moorings cause clonking noise in the same frequency range (∼100 Hz to a few kHz; e.g., Marley et al., 2017a). Many such artifacts can be minimized with hydrophones deployed on the seafloor (e.g., McCauley et al., 2017), though soft seafloor material such as sand moving over the hydrophone may contaminate acoustic recordings up to a few kHz (e.g., Erbe, 2009). Alternatives are arrangements that drift freely with the currents. The recorder is suspended from a buoy via a suspension system, which may comprise a drogue and a bungee that decouple the hydrophone from surface wave action. Similarly, a catenary (or distributed buoyancy) arrangement will decouple the hydrophone and spatially remove it from potential noise generated at the surface buoy (Figure 5). Building noise-free moorings is an art, and different designs may be required for different situations.
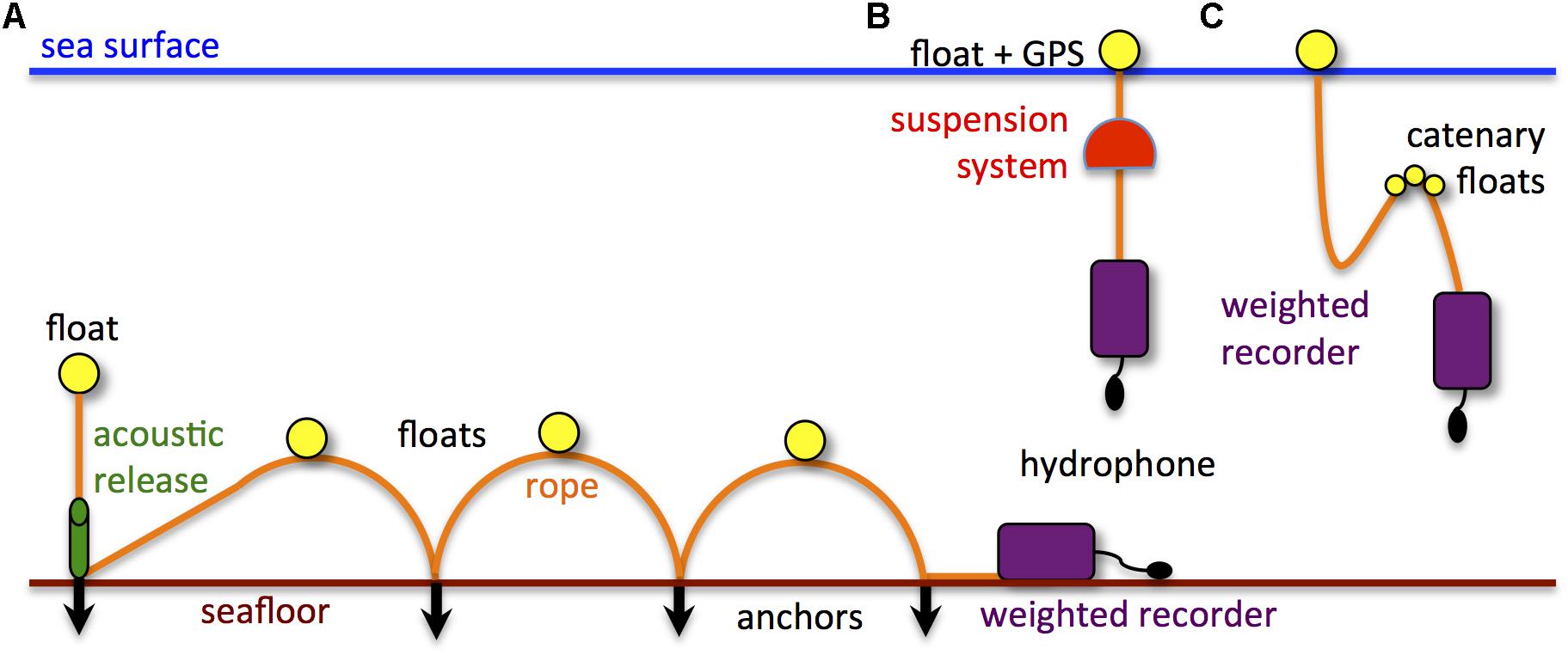
Figure 5. Sketch of hydrophone deployments that minimize mooring artifacts: (A) recorder on the seafloor and spatially decoupled from the release mechanism; (B) recorder suspended from a surface float via a decoupling suspension system and freely drifting; and (C) recorder suspended via a catenary system. The latter could be freely drifting from a surface float of attached to a seafloor anchor.
An international standard has recently been developed for the measurement of ship noise in deep water (i.e., water depth more than 150 m or 1.5 × ship length, whichever is greater) (International Organization for Standardization, 2016). The ship travels along a pre-defined course, and recordings are taken from both port and starboard aspects. While the standard does not specify a certain speed, it would be good to obtain measurements at multiple speeds representing typical operational speeds. Recording is done in the geometric far field (i.e., closest point of approach 100 m or 1 × ship length, whichever is greater) with a vertical array, having three hydrophones at specified inclination angles from the ship. The ‘radiated noise level’ (RNL, referenced to 1 m) is computed by applying a geometric (spherical) spreading loss term [20 log10(range)] over the slant range for each hydrophone and then averaging over all hydrophones. This averaging smooths over the Lloyd’s mirror interference pattern. The RNL is useful for noise emission studies, but may lead to large errors when used to estimate received levels at animals in other environments. This is because the environment in which the ship was recorded affects RNL. The recent release of Part 2 of this standard (International Organization for Standardization, 2019) provides formulae to estimate equivalent monopole source levels that correct for surface effects.
In order to compute environment-corrected monopole source levels, sound propagation models need to be applied that translate levels recorded at long range to levels normalized to 1 m range. There are a number of sound propagation models to choose from—depending on the environment (e.g., Etter, 2003; Jensen et al., 2011). The resulting source levels can then be inputted into sound propagation models for other environments in order to estimate received levels at the animals (e.g., Erbe et al., 2013; Williams et al., 2014). If a spherical spreading loss term is applied rather than a sound propagation model, then source levels are commonly under-estimated if the recording hydrophone was at shallow inclinations from the ship. Conversely, if monopole source levels are taken from the literature and a spherical loss is applied, then received levels may be over-estimated, when the receiving animal is at shallow inclinations from the ship. These are likely common problems in the literature. For example, the RNLs (re 1 m) of cargo vessels reported by McKenna et al. (2012) and Veirs et al. (2016) were up to 15 and 25 dB less than the source levels (re 1 m) of Simard et al. (2016), respectively, likely due to an underestimation of propagation loss. This is because of the dipole radiation pattern of a ship and its image source, yielding a propagation loss well above the wrongly, yet commonly applied 20 log10(range) at shallow inclination angles (e.g., Ainslie et al., 2014). Using sound propagation models, Chen et al. (2017) showed that gray seals experienced step changes of up to 20 dB in the received ship noise levels as they dove throughout the water column in the Celtic Sea. This was because of environmental features such as thermoclines, which a geometric propagation loss model cannot account for.
Finally, once recordings of watercraft have been obtained, they are sometimes played back to animals in different environments for response studies. The recorded sound was affected (in frequency and level) by the environment in which the recordings were made and by the recording system. It will likely be broadcast in yet another, different environment, resulting in further affected received spectrum levels. In addition, the speaker used for playback will have a frequency response, which can distort the signal. Ideally, the speaker’s frequency response is measured, and the playback signal is digitally filtered with the inverse of the frequency response before the playback study. Furthermore, the underwater speaker used will have a rather different sound radiation (i.e., directivity) pattern from the recorded vessel. Finally, it is impossible to simulate an approaching vessel with a single, moored speaker, because not only the received level changes as a vessel approaches, but also its spectrum and directionality.
With Biology: Experimental Design, Disturbance Differentiation, and Biological Significance
One of the most fundamental aspects of experimental design is ensuring that fair comparisons are made. In many response studies, this requires having some idea of ‘normal’ animal behavior in the form of a control group, with which treatment groups can then be compared for deviations that could imply disturbance (Johnson and Besselsen, 2002). However, here field-based marine mammal studies typically hit a problem: Despite the advancements of acoustic and visual monitoring techniques over recent decades, many fundamental questions regarding marine mammal behavior remain unanswered. As a result, the scientific community are still trying to determine the realms of normal behavior, hindered by continual new discoveries describing range expansions, diving abilities, hearing capabilities, and so on (e.g., Schorr et al., 2014; Cranford and Krysl, 2015; Accardo et al., 2018). Furthermore, all animals are individuals and the response of any given individual may change based on its current requirements and motivational states (e.g., health, reproductive status, age, energetic requirements; Pirotta et al., 2015a). Overall, this means that within the same species, individuals may respond differently in different environments and at different times, depending upon their previous experience with man-made noise and the importance of the habitat they are occupying for their current life-function requirements. Additionally, as previously discussed, animal behavioral responses can take many forms. This can make it difficult to conclusively identify when disturbance has occurred.
Similarly, a lack of control contexts can further confound results. There are few environments globally which have not experienced anthropogenic stressors (Halpern et al., 2015). Thus, there are few ‘naive’ populations of marine mammals to serve as baselines in behavioral response studies. This raises the question of habituation (e.g., Cox et al., 2001). Do we see no behavioral response to noise because the population is already used to the presence of such sounds? If so, did behavioral responses ever occur or have animals developed strategies to deal with these noisy environments? And, if such strategies exist, do they evoke an energetic or reproductive cost to the animals involved?
It is possible to account for anthropogenic, biological, and environmental contexts by including a suite of additional variables. In fact, the majority of studies we reviewed tried to account for at least one form of context. Some contextual factors, however, have not been addressed in impact assessments of underwater noise, such as the role of nearby conspecifics (Dunlop, 2016a) or nearby animals of other species (e.g., Koper and Plön, 2016). Contextual data of any type may not always be available or obtainable at a sufficient spatial or temporal resolution to coincide with quick behavioral events (Mannocci et al., 2017). And so, this leads to the issue of sample size. Statistical models with too many variables and insufficient sample size will fail to converge. Consequently, there are minimum sample sizes required for different statistical tests and levels of precision (e.g., Hampton et al., 2019). Unfortunately, the optimum sample size generally cannot be calculated until after the study has been completed. Methods for estimating it beforehand require some knowledge about variation within the study population (Dell et al., 2002); but, such variation remains poorly understood for the majority of marine mammal species. While increasing the sample size is statistically preferable, the majority of marine mammal studies suffer sample-size restrictions due to logistics and economics.
Once the best possible experimental design has been implemented, there is the problem of disturbance differentiation. Firstly, impact assessment studies are often confounded by the fact that the majority of marine mammal studies are boat-based. This introduces a potential source of observer bias from the presence of the research vessel and the noise it creates. Such bias is unavoidable in many situations, although increasingly researchers are attempting to include this in their analyses (e.g., Lusseau, 2003b). In coastal settings, land-based observations are more readily implementable and may help reduce (or totally exclude) any influence from observer presence. However, this does not assist in resolving the question of whether animals respond to the physical presence of a vessel or if responses are due to the noise that vessel creates, or to any other factor in the environment.
And so, despite the best intentions, many response studies may be restricted to relatively simple analyses, such as the use of basic comparative statistics (such as t-tests, ANOVAs, and non-parametric equivalents) to look at one particular behavioral response with and without the presence of ships. This is not to say that such studies are of no value—every result adds another piece to the overall puzzle. But they by no means capture the full context of the situation. Now that long-term datasets are in existence, researchers are increasingly able to apply more complex analytical techniques, consider individual motivations in the study species, and even make predictions using agent- or context-based modeling (e.g., Ellison et al., 2012; Nabe-Nielsen et al., 2014; Pirotta et al., 2014).
Once analytical techniques have been applied, the final question is whether any observed response actually matters in terms of biological significance. Behavioral changes associated with anthropogenic activities are often assumed to equate to a biologically significant effect (New et al., 2013; Curé et al., 2016). Individuals exposed to novel forms or chronic levels of disturbance may be displaced from critical habitat, disrupted from key activities, and thus suffer lower individual fitness, reproductive success, or overall survival (New et al., 2013). However, this may not be the case for infrequent disturbance resulting in instantaneous or short-term responses. For example, although animals may initially leave a site when exposed to anthropogenic activities, this may not equate to their utilizing lower-quality habitats or experiencing long-term, broad-scale displacement (Thompson et al., 2013). Recently, several studies have attempted to investigate biological significance using advanced mathematical models that allow for complexity of animal behavior, motivational state, social structure, and exposure to anthropogenic activities (e.g., New et al., 2013). Unfortunately, ground-truthing the outcomes is logistically challenging, requiring long-term studies at the individual- and population-level. Therefore, most behavioral studies are still restricted to establishing links between short-term measures and long-term population consequences (New et al., 2014).
Research Needs
As can be seen from Supplementary Table S1, research on the potential impacts of watercraft on marine mammals has been patchy—in terms of its coverage of species, geographic areas, vessel type, and type of impact. As a result, there are a number of knowledge gaps resulting in several obvious research needs.
Species Coverage
The Society for Marine Mammalogy currently recognizes 126 extant species of cetaceans, pinnipeds and sirenians. While 47 of these species have been studied regarding the impacts of vessel noise, the vast majority have received no attention or at maximum, one publication. More than half (64%) of the mysticete species have at least been the topic of a publication once, as have about half (46%) of the delphinid (Family Delphinidae) and half (43%) of the porpoise (Family Phocoenidae) species. However, of all the river dolphins (Families Iniidae, Platanistidae, Pontoporiidae, and Lipotidae—noting that the latter was declared possibly extinct in 2006), only one publication was found. All of the 22 species of beaked whales (Family Ziphiidae) are deep-diving pelagic species and rather cryptic, and so only two have been studied with regard to noise impacts. In terms of sirenians, only the Florida manatee appears in the literature on vessel noise impacts. Out of the pinnipeds, four of 18 phocids (true seals) and one otariid (i.e., eared seals) have been included in publications on responses to vessel noise at sea. Note that we did not review publications on the potential effects of approaching vessels on hauled-out pinnipeds, as underwater noise would not have been the cause.
The most-commonly studied species identified in this review were bottlenose dolphins and humpback whales. Ease of access might have played a role, as these species are widespread and at times exceptionally coastal. Thus their popularity as a target species for vessel noise impact studies does not necessarily reflect their being a research priority, although many populations do inarguably experience high levels of vessel traffic and noise. In comparison, given that river dolphins experience a multitude of anthropogenic stressors, including often-chronic noise from boats, it is perhaps surprising that these species have not had greater research focus. Rivers are among the most threatened ecosystems in the world (Tockner et al., 2010); but these systems represent problematic study sites for cetacean research. For example, the Indus River dolphin (Platanista gangetica minor) historically occurred in approximately 3,400 km of the Indus River and its tributaries; surveying this extensive, narrow and convoluted system is logistically challenging (Braulik, 2006; Jensen et al., 2013). Finding river dolphins and tracking them during response studies is difficult. The literature thus far has consequently focused on abundance estimates and status assessments, as well as documenting and mitigating immediately lethal threats (e.g., bycatch; Smith and Smith, 1998), as opposed to potentially less-obvious threats such as disturbance from vessels and noise. Similarly, the potential impacts on cryptic species like deep-diving, pelagic beaked whales are perhaps not always apparent or easy to study. But impacts could be biologically significant, given the sheer volume and density of ocean traffic, coupled with a vertically downward focused sound radiation pattern and a deep-ocean sound propagation environment that enables very long propagation distances.
Non-cetacean species received considerably less research attention. Sirenians are predominantly found in coastal areas, whereas pinnipeds are tied to land; both these characteristics mean these animals inevitably have high habitat overlap with human activities. Yet the impacts of those activities in terms of their physical presence and associated noise remain poorly understood.
Geographic Area
Another group of species that has been under-represented are those utilizing Antarctic waters. Annually migrating mysticetes critically depend on the Antarctic Ocean in the austral summer for feeding, as they do not feed while on their tropical breeding grounds in the austral winter. Some of the phocid species are truly Antarctic in the sense that they are present there all year round. Antarctica is predominantly governed by high-income countries, and thus might be expected to receive higher levels of research attention. Ship noise, in particular, is rapidly increasing off Antarctica due to booming tourism and heightened fisheries effort (Erbe et al., 2019). While Arctic marine mammals were first studied several decades ago, at a time when industrial development (i.e., mostly offshore oil and gas) was expected to grow rapidly, no such impetus has yielded a research increase in Antarctica. In fact, not a single publication has addressed the potential effects of watercraft noise on marine mammals in Antarctic waters, perhaps because of an absence of oil and gas exploration (as prohibited under the Antarctic Treaty) and the associated funding that accompanies such work. However, the expanding tourism and fishing industries may offer opportunities for future research work.
Not all areas have such opportunities. Marine mammal conservation at a global scale is challenged by a lack of basic information on species presence, but this is particularly true in the developing world (Braulik et al., 2018). For instance, as noted above, river ecosystems have received relatively little research attention. However, in addition to being logistically challenging study areas, those utilized by river dolphins are all located in developing countries, and so local researchers also experience considerable socio-economic challenges when conducting even baseline research. Overall, the majority of publications identified in this review originated from developed countries. Although this likely in part reflects funding or resource availability, it could also reflect publishing practices. For example, in this review only English-publishing journals were included. Furthermore, whilst studies may have taken place on the impacts of noise on marine mammals in developing countries, this research may not have reached the international, peer-reviewed publication stage. It is likely that this information is available, but difficult to access or not publically available (e.g., internal reports, environmental impact assessments, or local conservation and management plans). Therefore, there is a need not only for greater research in particular geographic areas, but also for sharing of research outcomes with a global audience.
Vessel Type
Vessels ranging from small, rigid-hulled inflatable whale-watching boats to large, powerful icebreakers have been investigated with regards to their potential impacts on certain species of marine mammal. Some combinations of vessel type and marine mammal species are more common than others in the literature. For example, the effects of cetacean-watching tourism vessels have most commonly been studied on bottlenose dolphins, then killer whales, humpback whales, and beluga whales. As tourism vessels are directly targeting marine mammals, it is reasonable to be concerned about the impacts these may have on the animals of interest. This is particularly true in areas where multiple trips occur each day or multiple tourism vessels are in operation, as this could lead to cumulative exposure and impacts. Additionally, cetacean-tourism vessels can also act as platforms of opportunity, allowing researchers the chance to study these animals from the tourism vessel itself rather than a dedicated research vessel. However, whilst there are many studies investigating the impacts of cetacean tourism, few specifically consider noise from tourism vessels.
In comparison, small recreational watercraft, such as jetskis, have received relatively little attention. Recreational watercraft may also have cumulative impacts on marine mammals, with an individual animal potentially encountering a multitude of vessels each day. Personal watercraft are considerably more challenging to document than tour vessels, but, given the continual increase in personal watercraft ownership, these vessels are of increasing concern with regards to noise impacts on marine mammals.
Type of Impact
The types of noise impacts that have been studied are as patchy as the coverage of species, areas, and vessels. Risk assessments are often based on the assumption that affected animals will leave the area. However, as summarized above, there is overwhelming evidence that marine mammals can display a wide range of behavioral responses, ranging from the obvious (e.g., area avoidance) to the subtle (e.g., shifts in acoustic behavior or raised cortisol levels). Measuring these responses comes with a number of logistical challenges; consequently, many studies have historically focused on the former, easier-to-identify response types. Recent technological developments have facilitated a rise in the number of studies targeting subtler types of impact, which will undoubtedly continue over coming years. However, there is still a need for integrative studies that simultaneously consider multiple response types in order to capture the variation associated with different species, populations, cohorts, and individuals.
One obvious pattern is that the effects of noise on the vocalizations of dolphins have been studied more than on those of other marine mammals. Perhaps this is due to the ease at which coastal dolphins can be recorded these days and due to the stereotypical nature of their vocalizations. This does not imply that acoustic communication is more important (and hence of more concern) in dolphins than other species. In fact, a range of responses can be evidence of disturbance, and more studies simultaneously looking at both physical and acoustical behavior are needed.
A significant gap in our knowledge is our lack of understanding of the potential long-term and population-level impacts and the corresponding biological significance. It could be argued that if a response does not equate to having biological significance, then it is of least concern; such conclusions would have obvious regulatory and management implications, but require considerable ground-truthing. This emphasizes the need for long-term, broad-scale studies targeting a range of response types to examine their consequences at the individual and population level. Physical and vocal behavioral changes impact an individual’s energetic costs (Noren et al., 2013; Holt et al., 2015, 2016; Williams et al., 2017), but knowledge on how these costs affect other biologically important functions (e.g., growth and reproduction) is currently absent. Even if population consequences could be ascertained, the question remains how these consequences affect the structure, function, and stability of the ecosystem of which the population is a part (Wong and Candolin, 2015). Recent research has focused on developing a framework for assessing the population consequences of disturbance (PCoD) using sparsely available data, supplementing it with expert elicitation to link changes in individual behavior or physiology to vital rates, and incorporating these into stochastic population models (King et al., 2015; Harwood et al., 2016). This methodology has the benefit of being able to model population consequences on the best available data, identifying gaps in understanding to focus research efforts, and being able to be updated as more data becomes available.
Discussion
The potential for watercraft noise to impact marine mammals is considerable. Some interactions have received particular attention, such as small boats affecting coastal dolphins; cetacean-watching boats affecting the specific populations of whales, dolphins, and porpoises that they target; large commercial ships affecting threatened species such as gray and southern resident killer whales; and icebreakers affecting Arctic mysticetes and odontocetes. Reasons for these specific combinations of vessel type and species include spatio-temporal overlap in presence, identified research needs (such as an expected rise in industrialization of the Arctic due to climate change), conservation urgency (as in the case of the southern resident killer whale), and ease of access (such as coastal and tourism-targeted species).
Other patterns, in addition to specific species-vessel combinations, emerge. For example, research looking at the effects of small vessels is primarily related to vessel behavior without mentioning noise produced by these vessels. This is in contrast to larger vessels, where the noise factor is more often taken into account. Overall, our understanding of the potential effects of watercraft noise on marine mammals exhibits a number of ‘holes.’
In this article, we have summarized the information available in the literature, highlighted some of the data gaps, and identified common problems. Standards are needed for both physical and biological aspects of study design, data collection (including recording of vessel noise and animal responses), data analysis, modeling, and reporting to avoid common mistakes and make results comparable and synthesisable (Erbe et al., 2016a). Given the inter-disciplinary nature of the field of noise impacts on marine fauna, multi-disciplinary teams are needed to ensure consistent quality of outcomes.
While this article focused on the impacts of ship noise on marine mammals, ship noise also impacts other marine fauna such as fish (e.g., Slabbekoorn et al., 2010; Simpson et al., 2016) and crustaceans (e.g., Wale et al., 2013). The potential bioacoustic impacts on these species have been of concern for as long as those on marine mammals (Myrberg, 1978). However, despite the longevity of these concerns, there remains an information paucity for many species, populations, and cohorts in terms of the impacts of noise, responses invoked, and biological significance of disturbance. As well as being a concern in its own right, this topic also has biological significance for marine mammals in terms of impacts on their prey species.
Overall, ship traffic is expected to keep increasing by approximately 4% per year over the coming five years, with different rates predicted for different ship types (United Nations Conference on Trade and Development [UNCTAD], 2018). Ship noise is a loss in energy, and vibrating propellers, appendages, and cavities are a structural risk; therefore, there is a natural incentive for the shipping industry to maintain its vessels and thus reduce noise (Leaper and Renilson, 2012; Leaper et al., 2014). Reducing ship noise for environmental reasons has also been on the agenda of the International Maritime Organization (IMO) publishing guidelines on quieting technologies and methods for newly built, as well as existing, vessels (International Maritime Organization [IMO], 2014). Particularly quiet vessels have been designed for defense and research applications, demonstrating that significant reductions in a ship’s noise footprint are achievable (Mitson, 1995; Fischer and Brown, 2005; Bahtiarian and Fischer, 2006; De Robertis et al., 2013; Palomo et al., 2014). The conundrum remains though, whether quieter vessels pose a higher risk of collision with marine mammals.
Conclusion
The impacts of ship noise on marine mammals continue to be of great concern. Despite this and increasing research attention over recent years, a number of common problems exist in terms of both the physics and biology of this inter-disciplinary issue. Consequently, a number of knowledge gaps remain. However, growing awareness, improving technology, increasing availability of multi-variate data streams, and analytical advancements have started to provide much-needed context for impact assessments. The continuing growth of long-term data sets is enabling much-needed assessments of chronic exposures at the individual and population level of marine mammals. As a scientific community, we should endeavor to address the gaps highlighted in this review to strategically target under-represented species, geographic areas, vessel types, and types of impact.
Author Contributions
CE conceived, designed, and led the study, collected and analyzed the data, prepared the figures and table, authored and reviewed the drafts of the manuscript, and approved the final manuscript. SM and RS contributed to the design of the study, collected the data, prepared the figures, authored and reviewed the drafts of the manuscript, and approved the final manuscript. JS, LT, and CE collected the data, authored and reviewed the drafts of the manuscript, and approved the final manuscript.
Conflict of Interest
The authors declare that the research was conducted in the absence of any commercial or financial relationships that could be construed as a potential conflict of interest.
Supplementary Material
The Supplementary Material for this article can be found online at: https://www.frontiersin.org/articles/10.3389/fmars.2019.00606/full#supplementary-material
TABLE S1 | Publications on the effects of ship noise on marine mammals: Reference, vessel type, species, study location, latitude, longitude, study objectives, study design, range to vessel, types of responses, received level, anthropogenic covariates, environmental covariates, biological covariates, and sample size.
Footnotes
- ^ In the case of ship noise, source levels (SL) are typically given as a root-mean-square (rms) sound pressure level (SPL). The sound pressure is recorded at some distance (i.e., in the far-field) from the vessel, and the root-mean-square is computed (i.e., literally squaring the pressure samples, summing, dividing by their number, and taking the square-root). Applying “20 log10()” converts the rms sound pressure to a level quantity (i.e., SPL) in the far-field. Propagation loss is typically modeled and a propagation loss term is added, yielding a (monopole) SL referenced to a distance of 1 m from the source. SPL and SL are thus expressed in dB relative to 1 μPa and 1 μPa m, respectively. Note that the notation of ‘@ 1 m’ is common in the literature but deprecated by the ISO (International Organization for Standardization, 2016, 2017).
- ^ Web of Science search information: Search string: TS = (ship$ OR boat$ OR vessel$) AND TS = noise AND TS = (marine mammal$ OR whale$ OR porpoise$ OR dolphin$ OR seal$ OR sea lion$ OR sealion$ OR dugong$ OR manatee$). Years searched: 1972–2019. Number of returned articles: 504.
- ^ The sound exposure level (SEL) is a measure of the total noise energy over time. It is computed as the time-integral of the squared pressure, before applying 10 log10(), and it is expressed in dB relative to 1 μPa2s (International Organization for Standardization, 2017).
References
Accardo, C. M., Ganley, L. C., Brown, M. W., Duley, P. A., George, J. C., Reeves, R. R., et al. (2018). Sightings of a bowhead whale (Balaena mysticetus) in the Gulf of Maine and its interactions with other baleen whales. J. Cetacean Res. Manag. 19, 23–30.
Ackerman, B. B., Wright, S. D., Bonde, R. K., Odell, D. K., and Banowetz, D. J. (1992). “Trends and patterns in manatee mortality in Florida, 1974-1991,” in Interim report of the technical workshop on manatee population biology (Manatee Population Research Report No.10), eds T. J. O’Shea, B. B. Ackerman, and H. F. Percival (Gainesville, FL: Florida Cooperative Fish and Wildlife Research Unit).
Aguilar Soto, N., Johnson, M., Madsen, P. T., Tyack, P. L., Bocconcelli, A., and Borsani, J. F. (2006). Does intense ship noise disrupt foraging in deep-diving Cuvier’s beaked whales (Ziphius cavirostris)? Mar. Mamm. Sci. 22, 690–699. doi: 10.1111/j.1748-7692.2006.00044.x
Ainslie, M. A., Dahl, P. H., and de Jong, C. A. F. (2014). “Practical spreading laws: the snakes and ladders of shallow water acoustics,” in Paper Presented at the 2nd International Conference and Exhibition on Underwater Acoustics, (Island of Rhodes).
Akamatsu, T., Nakazawa, I., Tsuchiyama, T., and Kimura, N. (2008). Evidence of night time movement of finless porpoises through Kanmon Strait monitored using a stationary acoustic recording device. Fish. Sci. 74, 970–975. doi: 10.1111/j.1444-2906.2008.01614.x
Akkaya Bas, A., Christiansen, F., Amaha Öztürk, A., Öztürk, B., and McIntosh, C. (2017). The effects of marine traffic on the behaviour of Black Sea harbour porpoises (Phocoena phocoena relicta) within the Istanbul Strait, Turkey. PLoS One 12:e0172970. doi: 10.1371/journal.pone.0172970
Andersen, S., Teilmann, J., Dietz, R., Schmidt, N., and Miller, L. (2012). Behavioural responses of harbour seals to human-induced disturbances. Aquat. Conserv. Mar. Freshw. Ecosyst. 22, 113–121. doi: 10.1002/aqc.1244
Anderwald, P., Brandecker, A., Coleman, M., Collins, C., Denniston, H., Haberlin, M. D., et al. (2013). Displacement responses of a mysticete, an odontocete, and a phocid seal to construction-related vessel traffic. Endang. Species Res. 21, 231–240. doi: 10.3354/esr00523
André, M., Caballe, A., Van der Schaar, M., Solsona, A., Houegnigan, L., Zaugg, S., et al. (2017). Sperm whale long-range echolocation sounds revealed by ANTARES, a deep-sea neutrino telescope. Sci. Rep. 7:45517. doi: 10.1038/srep45517
Andrew, R., Bruce, M. H., and James, A. M. (2002). Ocean ambient sound: comparing the 1960s with the 1990s for a receiver off the California coast. Acoust. Res. Lett. Online 3, 65–70. doi: 10.1121/1.1461915
Andrew, R. K., Howe, B. M., and Mercer, J. A. (2011). Long-time trends in ship traffic noise for four sites off the North American West Coast. J. Acoust. Soc. Am. 129, 642–651. doi: 10.1121/1.3518770
Arcangeli, A., and Crosti, R. (2009). The short-term impact of dolphin-watching on the behaviour of bottlenose dolphins (Tursiops truncatus) in Western Australia. J. Mar. Anim. Ecol. 2, 3–9.
Arveson, P. T., and Vendittis, D. J. (2000). Radiated noise characteristics of a modern cargo ship. J. Acoust. Soc. Am. 107, 118–129. doi: 10.1121/1.428344
Au, D., and Perryman, W. (1982). Movement and speed of dolphin schools responding to an approaching ship. Fish. Bull. 80, 371–379.
Au, W. W. L., and Green, M. (2000). Acoustic interaction of humpback whales and whale-watching boats. Mar. Environ. Res. 49, 469–481. doi: 10.1016/s0141-1136(99)00086-0
Azzara, A. J., von Zharen, W. M., and Newcomb, J. J. (2013). Mixed-methods analytic approach for determining potential impacts of vessel noise on sperm whale click behavior. J. Acoust. Soc. Am. 134, 4566–4574. doi: 10.1121/1.4828819
Bagočius, D. (2014). Potential masking of the Baltic grey seal vocalisations by underwater shipping noise in the Lithuanian area of the Baltic Sea. Environ. Res. Eng. Manag. 70, 66–72. doi: 10.5755/j01.erem.70.4.6913
Bahtiarian, M., and Fischer, R. (2006). Underwater radiated noise of the NOAA ship Oscar Dyson. Noise Control Eng. J. 54, 224–235. doi: 10.3397/1.2219891
Baker, C. S., and Herman, L. M. (1989). Behavioural Responses of Summering Humpback Whales To Vessel Traffic: Experimental and Opportunistic Observations (NTIS: PB90-198409). Honolulu, HI: University of Hawaii.
Bashir, T., Khan, A., Behera, S. K., and Gautam, P. (2013). Time dependent activity pattern of Ganges River dolphin Platanista gangetica gangetica and its response to human presence in Upper Ganges River, India. Mamm. Study 38, 9–17. doi: 10.3106/041.038.0110
Bejder, L., Samuels, A., Whitehead, H., and Gales, N. (2006). Interpreting short-term behavioural responses to disturbance within a longitudinal perspective. Anim. Behav. 72, 1149–1158. doi: 10.1016/j.anbehav.2006.04.003
Blair, H. B., Merchant, N. D., Friedlaender, A. S., Wiley, D. N., and Parks, S. E. (2016). Evidence for ship noise impacts on humpback whale foraging behaviour. Biol. Lett. 12:20160005. doi: 10.1098/rsbl.2016.0005
Blane, J. M., and Jaakson, R. (1994). The impact of ecotourism boats on the St Lawrence beluga whales. Environ. Conserv. 21, 267–269. doi: 10.1017/S0376892900033282
Blundell, G. M., and Pendleton, G. W. (2015). Factors affecting haul-out behavior of harbor seals (Phoca vitulina) in tidewater glacier inlets in Alaska: can tourism vessels and seals coexist? PLoS One 10:e0125486. doi: 10.1371/journal.pone.0125486
Braulik, G. T. (2006). Status assessment of the Indus River dolphin, Platanista gangetica minor, March–April 2001. Biol. Conserv. 129, 579–590. doi: 10.1016/j.biocon.2005.11.026
Braulik, G. T., Kasuga, M., Wittich, A., Kiszka, J. J., MacCaulay, J., Gillespie, D., et al. (2018). Cetacean rapid assessment: an approach to fill knowledge gaps and target conservation across large data deficient areas. Aquat. Conserv. Mar. Freshw. Ecosyst. 28, 216–230. doi: 10.1002/aqc.2833
Buck, B. M., and Greene, C. R. (1980). A two-hydrophone method of eliminating the effects of nonacoustic noise interference in measurements of infrasonic ambient noise levels. J. Acoust. Soc. Am. 68, 1306–1308. doi: 10.1121/1.385097
Buckstaff, K. C. (2004). Effects of watercraft noise on the acoustic behaviour of bottlenose dolphins, Tursiops truncatus, in Sarasota Bay, Florida. Mar. Mamm. Sci. 20, 709–725. doi: 10.1111/j.1748-7692.2004.tb01189.x
Burgess, W. C., Tyack, P. L., Le Boeuf, B. J., and Costa, D. P. (1998). A programmable acoustic recording tag and first results from free-ranging northern elephant seals. Deep Sea Res. Part II-Top. Stud. Oceanogr. 45, 1327–1351. doi: 10.1016/S0967-0645(98)00032-0
Burnham, R., Duffus, D., and Mouy, X. (2018). Gray Whale (Eschrichtius robustus) call types recorded during migration off the West Coast of Vancouver Island. [Original Research]. Front. Mar. Sci. 5:329. doi: 10.3389/fmars.2018.00329
Calleson, C. S., and Kipp Frohlich, R. (2007). Slower boat speeds reduce risks to manatees. Endanger. Species Res. 3, 295–304. doi: 10.3354/esr00056
Castellote, M., Clark, C., and Lammers, M. (2012). Acoustic and behavioural changes by fin whales (Balaenoptera physalus) in response to shipping and airgun noise. Biol. Conserv. 147, 115–122. doi: 10.1016/j.biocon.2011.12.021
Cato, D. H. (2008). “Ocean ambient noise: its measurement and its significance to marine animals,” in Paper Presented at the Proceedings of the Institute of Acoustics - Underwater Noise Measurement, Impact and Mitigation, (Southampton).
Chapman, N. R., and Price, A. (2011). Low frequency deep ocean ambient noise trend in the Northeast Pacific Ocean. J. Acoust. Soc. Am. 129, EL161–EL165. doi: 10.1121/1.3567084
Chen, F., Shapiro, G. I., Bennett, K. A., Ingram, S. N., Thompson, D., Vincent, C., et al. (2017). Shipping noise in a dynamic sea: a case study of grey seals in the Celtic Sea. Mar. Pollut. Bull. 114, 372–383. doi: 10.1016/j.marpolbul.2016.09.054
Cholewiak, D., Clark, C. W., Ponirakis, D., Frankel, A., Hatch, L. T., Risch, D., et al. (2018). Communicating amidst the noise: modeling the aggregate influence of ambient and vessel noise on baleen whale communication space in a national marine sanctuary. Endanger. Species Res. 36, 59–75. doi: 10.3354/esr00875
Christiansen, F., Lusseau, D., Stensland, E., and Berggren, P. (2010). Effects of tourist boats on the behaviour of Indo-Pacific bottlenose dolphins off the south coast of Zanzibar. Endanger. Spec. Res. 11, 91–99. doi: 10.3354/esr00265
Clark, C. W., Ellison, W. T., Southall, B. L., Hatch, L., Van Parijs, S. M., Frankel, A., et al. (2009). Acoustic masking in marine ecosystems: intuitions, analysis, and implication. Mar. Ecol. Prog. Ser. 395, 201–222. doi: 10.3354/Meps08402
Cole, B. F., and Podeszwa, E. M. (1967). Shallow-water propagation under downward-refraction conditions. J. Acoust. Soc. Am. 41, 1479–1484. doi: 10.1121/1.1910510
Cominelli, S., Devillers, R., Yurk, H., MacGillivray, A., McWhinnie, L., and Canessa, R. (2018). Noise exposure from commercial shipping for the southern resident killer whale population. Mar. Pollut. Bull. 136, 177–200. doi: 10.1016/j.marpolbul.2018.08.050
Constantine, R., Brunton, D. H., and Dennis, T. (2004). Dolphin-watching tour boats change bottlenose dolphin (Tursiops truncatus) behaviour. Biol. Conserv. 117, 299–307. doi: 10.1016/j.biocon.2003.12.009
Cosens, S. E., and Dueck, L. P. (1988). “Responses of migrating narwhal and beluga to icebreaker traffic at the Admiralty Inlet ice-edge, N.W.T. in 1986,” in Port and Ocean Engineering Under Arctic Conditions, eds W. M. Sackinger, and M. O. Jeffries (Fairbanks, AK: Geophysical Institute, University of Alaska), 39–54.
Cox, T. M., Read, A. J., Solow, A., and Tregenza, N. (2001). Will harbour porpoises (Phocoena phocoena) habituate to pingers? J. Cetacean Res. Manag. 3, 81–86.
Cranford, T. W., and Krysl, P. (2015). Fin whale sound reception mechanisms: skull vibration enables low-frequency hearing. PLoS One 10:e0116222. doi: 10.1371/journal.pone.0116222
Cummings, W. C., and Thompson, P. O. (1971). Underwater sounds from the blue whale, Balaenoptera musculus. J. Acoust. Soc. Am. 50, 1193–1198. doi: 10.1121/1.1912752
Cunningham, K. A., and Mountain, D. C. (2014). Simulated masking of right whale sounds by shipping noise: incorporating a model of the auditory periphery. J. Acoust. Soc. Am. 135, 1632–1640. doi: 10.1121/1.4864470
Curé, C., Isojunno, S., Visser, F., Wensveen, P. J., Sivle, L. D., Kvadsheim, P. H., et al. (2016). Biological significance of sperm whale responses to sonar: comparison with anti-predator responses. Endanger. Spec. Res. 31, 89–102. doi: 10.3354/esr00748
Dahlheim, M., and Castellote, M. (2016). Changes in the acoustic behavior of gray whales Eschrichtius robustus in response to noise. Endanger. Species Res. 31, 227–242. doi: 10.3354/esr00759
Dahlheim, M. E. (1987). Bio-acoustics of the Gray Whale (Eschrichtius robustus). Ph.D. Thesis, University of British Columbia: Vancouver, BC.
Dahlheim, M. E., Fisher, H. D., and Schempp, J. D. (1984). “Sound production by the gray whale and ambient noise levels in Laguna San Ignacio, Baja California Sur, Mexico,” in The Gray Whale Eschrichtius robustus, eds M. L. Jones, S. L. Swartz, and S. Leatherwood (New York, NY: Academic Press), 511–541. doi: 10.1016/b978-0-08-092372-7.50028-5
De Robertis, A., Wilson, C. D., Furnish, S. R., and Dahl, P. H. (2013). Underwater radiated noise measurements of a noise-reduced fisheries research vessel. Ices J. Mar. Sci. 70, 480–484. doi: 10.1121/1.3660550
de Sá Alves, L. C. P., Orams, M., Andriolo, A., and de Freitas Azevedo, A. (2012). The growth of ‘botos feeding tourism’, a new tourism industry based on the boto (Amazon river dolphin) Inia geoffrensis in the Amazonas State, Brazil. Sitientibus Série Ciências Biológicas 11, 8–15. doi: 10.13102/scb140
Dell, R. B., Holleran, S., and Ramakrishnan, R. (2002). Sample size determination. ILAR J. 43, 207–213. doi: 10.1093/ilar.43.4.207
DeRuiter, S. L., Southall, B. L., Calambokidis, J., Zimmer, W. M. X., Sadykova, D., Falcone, E. A., et al. (2013). First direct measurements of behavioural responses by Cuvier’s beaked whales to mid-frequency active sonar. Biol. Lett. 9:20130223. doi: 10.1098/rsbl.2013.0223
Dong, S.-Y., Dong, L.-J., Li, S.-H., Kimura, S., and Akamatsu, T. (2012). Effects of vessel traffic on the acoustic behavior of Yangtze finless porpoises (Neophocaena phocaenoides asiaeorientalis) in the confluence of Poyang lake and the Yangtze River using fixed passive acoustic observation methods. Acta Hydrobiol. Sin. 36, 246–254.
Dunlop, R. A. (2016a). Changes in vocal parameters with social context in humpback whales: considering the effect of bystanders. Behav. Ecol. Sociobiol. 70, 857–870. doi: 10.1007/s00265-016-2108-0
Dunlop, R. A. (2016b). The effect of vessel noise on humpback whale, Megaptera novaeangliae, communication behaviour. Anim. Behav. 111, 13–21. doi: 10.1016/j.anbehav.2015.10.002
Dunlop, R. A., Noad, M. J., McCauley, R. D., Kniest, E., Paton, D., and Cato, D. H. (2015). The behavioural response of humpback whales (Megaptera novaeangliae) to a 20 cubic inch air gun. Aquat. Mamm. 41, 412–433. doi: 10.1578/AM.41.4.2015.412
Dunlop, R. A., Noad, M. J., McCauley, R. D., Kniest, E., Slade, R., Paton, D., et al. (2016). Response of humpback whales (Megaptera novaeangliae) to ramp-up of a small experimental air gun array. Mar. Pollut. Bull. 103, 72–83. doi: 10.1016/j.marpolbul.2015.12.044
Dunlop, R. A., Noad, M. J., McCauley, R. D., Kniest, E., Slade, R., Paton, D., et al. (2017a). The behavioural response of migrating humpback whales to a full seismic airgun array. Proc. R. Soc. B Biol. Sci. 284:20171901. doi: 10.1098/rspb.2017.1901
Dunlop, R. A., Noad, M. J., McCauley, R. D., Scott-Hayward, L., Kniest, E., Slade, R., et al. (2017b). Determining the behavioural dose–response relationship of marine mammals to air gun noise and source proximity. J. Exp. Biol. 220, 2878–2886. doi: 10.1242/jeb.160192
Dunlop, R. A., Noad, M. J., McCauley, R. D., Kniest, E., Slade, R., Paton, D., et al. (2018). A behavioural dose-response model for migrating humpback whales and seismic air gun noise. Mar. Pollut. Bull. 133, 506–516. doi: 10.1016/j.marpolbul.2018.06.009
Dyndo, M., Wiśniewska, D. M., Rojano-Doñate, L., and Madsen, P. T. (2015). Harbour porpoises react to low levels of high frequency vessel noise. Sci. Rep. 5:11083. doi: 10.1038/srep11083
Eberhardt, R. L., and Evans, W. E. (1962). Sound activity of the California gray whale (Eschrichtius glaucus). J. Audio Eng. Soc. 10, 324–328.
Ellison, W., Southall, B., Clark, C., and Frankel, A. (2012). A new context-based approach to assess marine mammal behavioral responses to anthropogenic sounds. Conserv. Biol. 26, 21–28. doi: 10.1111/j.1523-1739.2011.01803.x
Erbe, C. (2002). Underwater noise of whale-watching boats and its effects on killer whales (Orcinus orca). Mar. Mamm. Sci. 18, 394–418. doi: 10.1111/j.1748-7692.2002.tb01045.x
Erbe, C. (2013). Underwater noise of small personal watercraft (jet skis). J. Acoust. Soc. Am. 133, EL326–EL330. doi: 10.1121/1.4795220
Erbe, C., Ainslie, M. A., de Jong, C. A. F., Racca, R., and Stocker, M. (2016a). “The need for protocols and standards in research on underwater noise impacts on marine life,” in The Effects of Noise on Aquatic Life II, eds A. N. Popper, and A. Hawkins (New York, NY: Springer Verlag), 1265–1271. doi: 10.1007/978-1-4939-2981-8_159
Erbe, C., Liong, S., Koessler, M. W., Duncan, A. J., and Gourlay, T. (2016b). Underwater sound of rigid-hulled inflatable boats. J. Acoust. Soc. Am. 139, EL223–EL227. doi: 10.1121/1.4954411
Erbe, C., McCauley, R., Gavrilov, A., Madhusudhana, S., and Verma, A. (2016c). The underwater soundscape around Australia. Proc. Acoust. 2016, 9–11. doi: 10.1371/journal.pone.0089820
Erbe, C., Dähne, M., Gordon, J., Herata, H., Houser, D. S., Koschinski, S., et al. (2019). Managing the effects of noise from ship traffic, seismic surveying and construction on marine mammals in Antarctica. Front. Mar. Sci. doi: 10.3389/fmars.2019.00647
Erbe, C., Dunlop, R., and Dolman, S. (2018). “Effects of noise on marine mammals,” in Effects of Anthropogenic Noise on Animals, eds H. Slabbekoorn, R. J. Dooling, A. N. Popper, and R. R. Fay (New York, NY: Springer), 277–309. doi: 10.1007/978-1-4939-8574-6_10
Erbe, C., and Farmer, D. M. (2000). Zones of impact around icebreakers affecting beluga whales in the Beaufort Sea. J. Acoust. Soc. Am. 108, 1332–1340. doi: 10.1121/1.1288938
Erbe, C., MacGillivray, A. O., and Williams, R. (2012). Mapping cumulative noise from shipping to inform marine spatial planning. J. Acoust. Soc. Am. 132, EL 423–EL 428. doi: 10.1121/1.4758779
Erbe, C., McCauley, R. D., McPherson, C., and Gavrilov, A. (2013). Underwater noise from offshore oil production vessels. J. Acoust. Soc. Am. 133, EL 465–EL 470. doi: 10.1121/1.4802183
Erbe, C., Verma, A., McCauley, R., Gavrilov, A., and Parnum, I. (2015). The marine soundscape of the Perth Canyon. Prog. Oceanogr. 137, 38–51. doi: 10.1016/j.pocean.2015.05.015
Erbe, C., Williams, R., Sandilands, D., and Ashe, E. (2014). Identifying modelled ship noise hotspots for marine mammals of Canada’s Pacific region. PLoS One 9:e89820. doi: 10.1371/journal.pone.0089820
Etter, P. C. (2003). Underwater Acoustic Modeling and Simulation, 3rd Edn. New York, NY: Spon Press.
Finley, K. J., Miller, G. W., Davis, R. A., and Greene, C. R. (1990). Reactions of belugas, Delphinapterus leucas, and narwhals, Monodon monoceros, to ice-breaking ships in the Canadian high arctic. Can. Bull. Fish. Aquat. Sci. 224, 97–117.
Fischer, R. W., and Brown, N. A. (2005). “Factors affecting the underwater noise of commercial vessels operating in environmentally sensitive areas,” Paper Presented at the IEEE Oceans 2005 Conference, Washington, DC.
Fletcher, S., LeBoeuf, B. J., Costa, D. P., Tyack, P. L., and Blackwell, S. B. (1996). Onboard acoustic recording from diving northern elephant seals. J. Acoust. Soc. Am. 100, 2531–2539. doi: 10.1121/1.417361
Foote, A. D., Osborne, R. W., and Hoelzel, A. R. (2004). Whale-call response to masking boat noise. Nature 428:910. doi: 10.1038/428910a
Fournet, M. E. H., Matthews, L. P., Gabriele, C. M., Haver, S., Mellinger, D. K., and Klinck, H. (2018). Humpback whales Megaptera novaeangliae alter calling behavior in response to natural sounds and vessel noise. Mar. Ecol. Prog. Ser. 607, 251–268. doi: 10.3354/meps12784
Frankel, A. S., and Clark, C. W. (2002). ATOC and other factors affecting the distribution and abundance of humpback whales (Megaptera novaeangliae) off the north shore of Kauai. Mar. Mamm. Sci. 18, 644–662. doi: 10.1111/j.1748-7692.2002.tb01064.x
Frankel, A. S., and Gabriele, C. M. (2017). Predicting the acoustic exposure of humpback whales from cruise and tour vessel noise in Glacier Bay, Alaska, under different management strategies. Endanger. Spec. Res. 34, 397–415. doi: 10.3354/esr00857
Frisk, G. (2012). Noiseonomics: the relationship between ambient noise levels in the sea and global economic trends. Sci. Rep. 2:437. doi: 10.1038/srep00437
Gabriele, C. M., Ponirakis, D. W., Clark, C. W., Womble, J. N., and Vanselow, P. B. S. (2018). Underwater acoustic ecology metrics in an Alaska marine protected area reveal marine mammal communication masking and management alternatives. Front. Mar. Sci. 5:270. doi: 10.3389/fmars.2018.00270
Gaspard, C. J., Bauer, G. B., Reep, R. L., Dziuk, K., Cardwell, A., Read, L., et al. (2012). Audiogram and auditory critical ratios of two Florida manatees (Trichechus manatus latirostris). J. Exp. Biol. 215, 1442–1447. doi: 10.1242/jeb.089201
Gassmann, M., Wiggins, S. M., and Hildebrand, J. A. (2017). Deep-water measurements of container ship radiated noise signatures and directionality. J. Acoust. Soc. Am. 142, 1563–1574. doi: 10.1121/1.5001063
Geraci, J. R., and St Aubin, D. J. (1980). Offshore petroleum resource development and marine mammals: a review and research recommendations. Mar. Fish. Rev. 42, 1–12.
Gerstein, E. R., Blue, J. E., and Forysthe, S. E. (2005). “The acoustics of vessel collisions with marine mammals,” in Proceedings of the Oceans 2005 MTS/IEEE, Washington, DC.
Gerstein, E. R., Gerstein, L., Forsythe, S. E., and Blue, J. E. (1999). The underwater audiogram of the West Indian manatee (Trichechus manatus). J. Acoust. Soc. Am. 105, 3575–3583. doi: 10.1121/1.424681
Goldbogen, J. A., Southall, B. L., DeRuiter, S. L., Calambokidis, J., Friedlaender, A. S., Hazen, E. L., et al. (2013). Blue whales respond to simulated mid-frequency military sonar. Proc. R. Soc. B 280:20130657. doi: 10.1098/rspb.2013.0657
Gordon, J., Leaper, R., Hartley, F. G., and Chappell, O. (1992). Effects of whale-watching vessels on the surface and underwater acoustic behaviour of sperm whales off Kaikoura, New Zealand. Conserv. Te Papa Atawhai 48, 1–64.
Greene, C. R. (1985). “Characteristics of waterborne industrial noise,” in Behavior, Disturbance Responses and Distribution of Bowhead Whales Balaena mysticetus in the Beaufort Sea, 1980-84 (NTIS PB87-124376; MMS 85-0034), ed. W. J. Richardson (Washington, DC: LGL Ecology Research Association Inc.).
Guerra, M., Dawson, S. M., Brough, T. E., and Rayment, W. J. (2014). Effects of boats on the surface and acoustic behaviour of an endangered population of bottlenose dolphins. Endanger. Species Res. 24, 221–236. doi: 10.3354/esr00598
Halpern, B. S., Frazier, M., Potapenko, J., Casey, K. S., Koenig, K., Longo, C., et al. (2015). Spatial and temporal changes in cumulative human impacts on the world’s ocean. Nat. Commun. 6:7615. doi: 10.1038/ncomms8615
Hamilton, E. L. (1980). Geoacoustic modelling of the sea floor. J. Acoust. Soc. Am. 68, 1313–1340. doi: 10.1121/1.385100
Hampton, J. O., MacKenzie, D. I., and Forsyth, D. M. (2019). How many to sample? Statistical guidelines for monitoring animal welfare outcomes. PLoS One 14:e0211417. doi: 10.1371/journal.pone.0211417
Hamson, R. M. (1997). The modelling of ambient noise due to shipping and wind sources in complex environments. Appl. Acoust. 51, 251–287. doi: 10.1016/S0003-682X(97)00003-0
Harwood, J., King, S., Booth, C., Donovan, C., Schick, R. S., Thomas, L., et al. (2016). “Understanding the population consequences of acoustic disturbance for marine mammals,” in The Effects of Noise on Aquatic Life II, eds A. N. Popper, and A. Hawkins, (New York, NY: Springer Verlag), 417–423. doi: 10.1007/978-1-4939-2981-8_49
Hastie, G. D., Wilson, B., Tufft, L. H., and Thompson, P. M. (2003). Bottlenose dolphins increase breathing synchrony in response to boat traffic. Mar. Mamm. Sci. 19, 74–84. doi: 10.1111/j.1748-7692.2003.tb01093.x
Heiler, J., Elwen, S. H., Kriesell, H. J., and Gridley, T. (2016). Changes in bottlenose dolphin whistle parameters related to vessel presence, surface behaviour and group composition. Anim. Behav. 117, 167–177. doi: 10.1016/j.anbehav.2016.04.014
Henry, E., and Hammill, M. O. (2001). Impact of small boats on the haulout activity of harbour seals (Phoca vitulina) in Metis Bay, Saint Lawrence Estuary, Quebec, Canada. Aquat. Mamm. 27, 140–148.
Hermannsen, L., Beedholm, K., Tougaard, J., and Madsen, P. T. (2014). High frequency components of ship noise in shallow water with a discussion of implications for harbor porpoises (Phocoena phocoena). J. Acoust. Soc. Am. 136, 1640–1653. doi: 10.1121/1.4893908
Hodgson, A. J., and Marsh, H. (2007). Response of dugongs to boat traffic: the risk of disturbance and displacement. J. Exp. Mar. Biol. Ecol. 340, 50–61. doi: 10.1016/j.jembe.2006.08.006
Holt, M., Noren, D., and Emmons, C. (2011). Effects of noise levels and call types on the source levels of killer whale calls. J. Acoust. Soc. Am. 130, 3100–3106. doi: 10.1121/1.3641446
Holt, M. M., Noren, D. P., Dunkin, R. C., and Williams, T. M. (2015). Vocal performance affects metabolic rate in dolphins: implications for animals communicating in noisy environments. J. Exp. Biol. 218, 1647–1654. doi: 10.1242/jeb.122424
Holt, M. M., Noren, D. P., Dunkin, R. C., and Williams, T. M. (2016). Comparing the metabolic costs of different sound types in bottlenose dolphins. Proc. Meet. Acoust. 27:010019. doi: 10.1121/2.0000274
Holt, M. M., Noren, D. P., Veirs, V., Emmons, C. K., and Veirs, S. (2009). Speaking up: Killer whales (Orcinus orca) increase their call amplitude in response to vessel noise. J. Acoust. Soc. Am. 125, El27–El32. doi: 10.1121/1.3040028
Houser, D. S., and Finneran, J. J. (2006). A comparison of underwater hearing sensitivity in bottlenose dolphins (Tursiops truncatus) determined by electrophysiological and behavioral methods. J. Acoust. Soc. Am. 120, 1713–1722. doi: 10.1121/1.2229286
International Maritime Organization [IMO] (2014). Guidelines. (for)the Reduction of Underwater Noise From Commercial Shipping to Address Adverse Impacts on Marine Life (Circulation No. MEPC.1/Circ.833). London: IMO.
International Organization for Standardization (2016). Underwater Acoustics—Quantities and Procedures for Description and Measurement of Underwater Sound From Ships—Part 1: Requirements for Precision Measurements in Deep Water Used for Comparison Purposes (ISO 17208-1). Geneva: International Organization for Standardization.
International Organization for Standardization (2017). Underwater Acoustics–Terminology (ISO 18405). Geneva: International Organization for Standardization.
International Organization for Standardization (2019). Underwater Acoustics—Quantities and Procedures for Description and Measurement of Underwater Sound From Ships—Part 2: Determination of Source Levels From Deep Water Measurements (ISO 17208-2). Geneva: International Organization for Standardization.
Jahoda, M., Lafortuna, C. L., Biassoni, N., Almirante, C., Azzellino, A., Panigada, S., et al. (2003). Mediterranean fin whale’s (Balaenoptera physalus) response to small vessels and biopsy sampling assessed through passive tracking and timing of respiration. Mar. Mamm. Sci. 19, 96–110. doi: 10.1111/j.1748-7692.2003.tb01095
Janik, V. M., and Thompson, P. M. (1996). Changes in surfacing patterns of bottlenose dolphins in response to boat traffic. Mar. Mamm. Sci. 12, 597–602. doi: 10.1111/j.1748-7692.1996.tb00073.x
Jansen, J. K., Boveng, P. L., Dahle, S. P., and Bengtson, J. L. (2010). Reaction of harbor seals to cruise ships. J. Wildl. Manag. 74, 1186–1194. doi: 10.1111/j.1937-2817.2010.tb01239.x
Jensen, F. B., Kuperman, W. A., Porter, M. B., and Schmidt, H. (2011). Computational Ocean Acoustics, 2nd Edn. New York, NY: Springer-Verlag.
Jensen, F. H., Rocco, A., Mansur, R. M., Smith, B. D., Janik, V. M., and Madsen, P. T. (2013). Clicking in shallow rivers: short-range echolocation of Irrawaddy and Ganges river dolphins in a shallow, acoustically complex habitat. PLoS One 8:e59284. doi: 10.1371/journal.pone.0059284
Johnson, P. D., and Besselsen, D. G. (2002). Practical aspects of experimental design in animal research. ILAR J. 43, 202–206. doi: 10.1093/ilar.43.4.202
Johnson, S. R., Greene, C. R., Davis, R. A., and Richardson, W. J. (1986). Bowhead Whales and Underwater Noise Near the Sandpiper Island Drillsite, Alaskan Beaufort Sea, Autumn 1985 (Report for Shell Western Exploration and Production). King City, ON: LGL Ltd. Environmental Research Associates and Greeneridge Sciences.
Jones, E. L., Hastie, G. D., Smout, S., Onoufriou, J., Merchant, N. D., Brookes, K. L., et al. (2017). Seals and shipping: quantifying population risk and individual exposure to vessel noise. J. Appl. Ecol. 54, 1930–1940. doi: 10.1111/1365-2664.12911
Joy, R., Tollit, D., Wood, J., MacGillivray, A., Li, Z., Trounce, K., et al. (2019). Potential benefits of vessel slowdowns on endangered Southern Resident Killer Whales. Front. Mar. Sci. 6:344. doi: 10.3389/fmars.2019.00344
Kastak, D., and Schusterman, R. J. (1998). Low-frequency amphibious hearing in pinnipeds: methods, measurements, noise, and ecology. J. Acoust. Soc. Am. 103, 2216–2228. doi: 10.1121/1.421367
King, S. L., Schick, R. S., Donovan, C., Booth, C. G., Burgman, M., Thomas, L., et al. (2015). An interim framework for assessing the population consequences of disturbance. Methods Ecol. Evol. 6, 1150–1158. doi: 10.1111/2041-210X.12411
Klishin, V. O., Diaz, R. P., Popov, V. V., and Supin, A. Y. (1990). Some characteristics of hearing of the Brazilian manatee, Trichechus inunguis. Aquat. Mamm. 16, 139–144.
Koper, R. P., Erbe, C., Preez, D. R. D., and Plön, S. (2016). Comparison of soundscape contributors between two neighboring southern right whale nursing areas along the South African coast. Proc. Meet. Acoust. 27:070014. doi: 10.1121/2.0000303
Koper, R. P., and Plön, S. (2016). Interspecific interactions between cetacean species in Algoa Bay, South Africa. Aquat. Mamm. 42, 454–461. doi: 10.1578/AM.42.4.2016.454
Kraus, S. D., Brown, M. W., Caswell, H., Clark, C. W., Fujiwara, M., Hamilton, P. K., et al. (2005). North Atlantic right whales in crisis. Science 309, 561–562. doi: 10.1126/science.1111200
Kreb, D., and Rahadi, K. D. (2004). Living under an aquatic freeway: effects of boats on Irrawaddy dolphins (Orcaella brevirostris) in a coastal and riverine environment in Indonesia. Aquat. Mamm. 30, 363–375. doi: 10.1578/AM.30.3.2004.363
Kvadsheim, P. H., DeRuiter, S., Sivle, L. D., Goldbogen, J., Roland-Hansen, R., Miller, P. J. O., et al. (2017). Avoidance responses of minke whales to 1-4 kHz naval sonar. Mar. Pollut. Bull. 121, 60–68. doi: 10.1016/j.marpolbul.2017.05.037
Laist, D. W., Knowlton, A. R., Mead, J. G., Collet, A. S., and Podesta, M. (2001). Collisions between ships and whales. Mar. Mamm. Sci. 17, 35–75. doi: 10.1111/j.1748-7692.2001.tb00980.x
Leaper, R., and Renilson, M. (2012). A review of practical methods for reducing underwater noise pollution from large commercial vessels. Int. J. Maritime Eng. 154, A79–A88. doi: 10.3940/rina.ijme.2012.a2.227
Leaper, R., Renilson, M., and Ryan, C. (2014). Reducing underwater noise from large commercial ships: current status and future directions. J. Ocean Technol. 9, 50–69.
Lemon, M., Lynch, T. P., Cato, D. H., and Harcourt, R. G. (2006). Response of travelling bottlenose dolphins (Tursiops aduncus) to experimental approaches by a powerboat in Jervis Bay, New South Wales, Australia. Biol. Conserv. 127, 363–372. doi: 10.1016/j.biocon.2005.08.016
Lesage, V., Barrette, C., Kingsley, M. C. S., and Sjare, B. (1999). The effect of vessel noise on the vocal behaviour of belugas in the St. Lawrence River Estuary, Canada. Mar. Mamm. Sci. 15, 65–84. doi: 10.1111/j.1748-7692.1999.tb00782.x
LGL Ltd. (1986). Reactions of Beluga Whales and Narwhals to Ship Traffic and Icebreaking Along Ice Edges in the Eastern Canadian High Arctic: 1982-1984. Ottawa, ON: Indian and Northern Affairs Canada.
Lightsey, J. D., Rommel, S. A., Costidis, A. M., and Pitchford, T. D. (2006). Methods used during gross necropsy to determine watercraft-related mortality in the Florida manatee (Trichechus manatus latirostris). J. Zoo Wildl. Med. 37, 262–276. doi: 10.1638/04-095.1
Lusseau, D. (2003a). Effects of tour boats on the behaviour of bottlenose dolphins: using markov chains to model anthropogenic impacts. Conserv. Biol. 17, 1785–1793. doi: 10.1111/j.1523-1739.2003.00054.x
Lusseau, D. (2003b). Male and female bottlenose dolphins Tursiops spp. have different strategies to avoid interactions with tour boats in Doubtful Sound, New Zealand. Mar. Ecol. Prog. Ser. 257, 267–274. doi: 10.3354/meps257267
Lusseau, D. (2005). Residency pattern of bottlenose dolphins Tursiops spp. in Milford Sound, New Zealand, is related to boat traffic. Mar. Ecol. Prog. Ser. 295, 265–272. doi: 10.3354/meps295265
Lusseau, D. (2006). The short-term behavioural reactions of bottlenose dolphins to interactions with boats in Doubtful Sound, New Zealand. Mar. Mamm. Sci. 22, 802–818. doi: 10.1111/j.1748-7692.2006.00052.x
Lusseau, D., Bain, D. E., Williams, R., and Smith, J. C. (2009). Vessel traffic disrupts the foraging behavior of southern resident killer whales Orcinus orca. Endanger. Species Res. 6, 211–221. doi: 10.3354/esr006211
Lusseau, D., and Higham, J. E. S. (2004). Managing the impacts of dolphin-based tourism through the definition of critical habitats: the case of bottlenose dolphins (Tursiops spp.) in Doubtful Sound, New Zealand. Tour. Manag. 25, 657–667. doi: 10.1016/j.tourman.2003.08.012
Mannocci, L., Boustany, A. M., Roberts, J. J., Palacios, D. M., Dunn, D. C., Halpin, P. N., et al. (2017). Temporal resolutions in species distribution models of highly mobile marine animals: recommendations for ecologists and managers. Divers. Distrib. 23, 1098–1109. doi: 10.1111/ddi.12609
Marley, S. A., Salgado-Kent, C. P., Erbe, C., and Parnum, I. (2017a). Effects of vessel traffic and underwater noise on the movement, behaviour and vocalisations of bottlenose dolphins in an urbanised estuary. Sci. Rep. 7:13437. doi: 10.1038/s41598-017-13252-z
Marley, S. A., Salgado Kent, C. P., Erbe, C., and Thiele, D. (2017b). A tale of two soundscapes: comparing the acoustic characteristics of urban versus pristine coastal dolphin habitats in Western Australia. Acoust. Austr. 45, 159–178. doi: 10.1007/s40857-017-0106-7
Marsh, H., Penrose, H., Eros, C., and Hugues, J. (2001). Dugong: Status Report and Action Plans for Countries and Territories (Early Warning and Assessment Report Series No. UNEP/DEWA/RS.02-1). Nairobi: United Nations Environment Programme.
Martins, D. T. L., Rossi-Santos, M. R., and Silva, F. J. D. (2018). Effects of anthropogenic noise on the acoustic behaviour of Sotalia guianensis (Van Beneden, 1864) in Pipa, North-eastern Brazil. J. Mar. Biol. Assoc. U. K. 98, 215–222. doi: 10.1017/S0025315416001338
Mattson, M. C., Thomas, J. A., and St Aubin, D. (2005). Effects of boat activity on the behavior of bottlenose dolphins (Tursiops truncatus) in waters surrounding Hilton Head Island, South Carolina. Aquat. Mamm. 31, 133–140. doi: 10.1578/AM.31.1.2005.133
May-Collado, L. J., and Quinones-Lebron, S. G. (2014). Dolphin changes in whistle structure with watercraft activity depends on their behavioral state. J. Acoust. Soc. Am. 135, EL193–EL198. doi: 10.1121/1.4869255
May-Collado, L. J., and Wartzok, D. (2008). A comparison of bottlenose dolphin whistles in the Atlantic Ocean: factors promoting whistle variation. J. Mammal. 89, 1229–1240. doi: 10.1644/07-MAMM-A-310.1
McCauley, R. D., Thomas, F., Parsons, M. J. G., Erbe, C., Cato, D., Duncan, A. J., et al. (2017). Developing an underwater sound recorder: the long and short (Time) of it. Acoust. Austr. 45, 301–311. doi: 10.1007/s40857-017-0113-118
McKenna, M., Ross, D., Wiggins, S., and Hildebrand, J. (2012). Underwater radiated noise from modern commercial ships. J. Acoust. Soc. Am. 131, 92–103. doi: 10.1121/1.3364100
Mikkelsen, L., Johnson, M., Wisniewska, D. M., van Neer, A., Siebert, U., Madsen, P. T., et al. (2019). Long-term sound and movement recording tags to study natural behavior and reaction to ship noise of seals. Ecol. Evol. 9, 2588–2601. doi: 10.1002/ece3.4923
Miksis-Olds, J. L., Bradley, D. L., and Niu, X. M. (2013). Decadal trends in Indian Ocean ambient sound. J. Acoust. Soc. Am. 134, 3464–3475. doi: 10.1121/1.4821537
Miksis-Olds, J. L., Donaghay, P. L., Miller, J. H., Tyack, P. L., and Nystuen, J. A. (2007a). Noise level correlates with manatee use of foraging habitats. J. Acoust. Soc. Am. 121, 3011–3020. doi: 10.1121/1.2713555
Miksis-Olds, J. L., Donaghay, P. L., Miller, J. H., Tyack, P. L., and Reynolds, J. E. (2007b). Simulated vessel approaches elicit differential responses from manatees. Mar. Mamm. Sci. 23, 629–649. doi: 10.1111/j.1748-7692.2007.00133.x
Miksis-Olds, J. L., and Nichols, S. M. (2016). Is low frequency ocean sound increasing globally? J. Acoust. Soc. Am. 139, 501–511. doi: 10.1121/1.4938237
Mitson, R. B. (1995). Underwater Noise of Research Vessels (Cooperative Res. Report No. 209). Copenhagen: International Council for the Exploration of the Sea.
Moore, S. E., and Ljungblad, D. K. (1984). Gray whales in the Beaufort, Chukchi, and Bering Seas: distribution and sound production. The Gray Whale Eschrichtius robustus 29, 543–559. doi: 10.1016/b978-0-08-092372-7.50029-7
Morete, M. E., Bisi, T. L., and Rosso, S. (2007). Mother and calf humpback whale responses to vessels around the Abrolhos Archipelago, Bahia, Brazil. J. Cetacean Res. Manag. 9, 241–248.
Morisaka, T., Shinohara, M., Nakahara, F., and Akamatsu, T. (2005). Effects of ambient noise on the whistles of Indo-Pacific bottlenose dolphin populations. J. Mammal. 86, 541–546. doi: 10.1644/1545-1542(2005)86%5B541:eoanot%5D2.0.co;2
Myrberg, A. A. (1978). “Ocean noise and the behavior of marine animals: relationships and implications,” Effects of Noise on Wildlife, eds J. L. Fletcher and R. G. Busnel (San Diego: Academic Press), 169–208.
Nabe-Nielsen, J., Sibly, R. M., Tougaard, J., Teilmann, J., and Sveegaard, S. (2014). “Effects of noise and by-catch on a Danish harbour porpoise population,” in Effects of Noise on Wildlife, Vol. 272, eds J. L. Fletcher and R. G. Busnel, (New York, NY: Academic Press), 242–251. doi: 10.1016/j.ecolmodel.2013.09.025
New, L. F., Clark, J. S., Costa, D. P., Fleishman, E., Hindell, M. A., Klanjcek, T., et al. (2014). Using short-term measures of behaviour to estimate long-term fitness of southern elephant seals. Mar. Ecol. Prog. Ser. 496, 99–108. doi: 10.3354/meps10547
New, L. F., Harwood, J., Thomas, L., Donovan, C., Clark, J. S., Hastie, G., et al. (2013). Modelling the biological significance of behavioural change in coastal bottlenose dolphins in response to disturbance. Funct. Ecol. 27, 314–322. doi: 10.1111/1365-2435.12052
Niemi, M., Auttila, M., Valtonen, A., Viljanen, M., and Kunnasranta, M. (2013). Haulout patterns of Saimaa ringed seals and their response to boat traffic during the moulting season. Endanger. Species Res. 22, 115–124. doi: 10.3354/esr00541
Noren, D., Johnson, A., Rehder, D., and Larson, A. (2009). Close approaches by vessels elicit surface active behaviors by southern resident killer whales. Endanger. Species Res. 8, 179–192. doi: 10.3354/esr00205
Noren, D. P., Holt, M. M., Dunkin, R. C., and Williams, T. M. (2013). The metabolic cost of communicative sound production in bottlenose dolphins (Tursiops truncatus). J. Exp. Biol. 216, 1624–1629. doi: 10.1242/jeb.083212
Nowacek, D. P., Johnson, M. P., and Tyack, P. L. (2004). North Atlantic right whales (Eubalaena glacialis) ignore ships but respond to alerting stimuli. Proc. R. Soc. Lond. Ser. B Biol. Sci. 271, 227–231. doi: 10.1098/rspb.2003.2570
Nowacek, S. M., Wells, R. S., Owen, E. C. G., Speakman, T. R., Flamm, R. O., and Nowacek, D. P. (2004). Florida manatees, Trichechus manatus latirostris, respond to approaching vessels. Biol. Conserv. 119, 517–523. doi: 10.1016/j.biocon.2003.11.020
Nowacek, D. P., Thorne, L. H., Johnston, D. W., and Tyack, P. L. (2007). Responses of cetaceans to anthropogenic noise. Mamm. Rev. 37, 81–115. doi: 10.1111/j.1365-2907.2007.00104.x
Nowacek, S. M., Wells, R. S., and Solow, A. R. (2001). Short-term effects of boat traffic on bottlenose dolphins, Tursiops truncatus, in Sarasota Bay, Florida. Mar. Mamm. Sci. 17, 673–688. doi: 10.1111/j.1748-7692.2001.tb01292.x
Nsw Government Maritime (2010). NSW Boat Ownership and Storage: Growth Forecasts to 2026. Sydney, NSW: NSW Government Maritime.
Øren, K., Kovacs, K. M., Yoccoz, N. G., and Lydersen, C. (2018). Assessing site-use and sources of disturbance at walrus haul-outs using monitoring cameras. Polar Biol. 41, 1737–1750. doi: 10.1007/s00300-018-2313-6
O’Shea, T. J. (1995). “Waterborne recreation and the Florida manatee,” in Wildlife and Recreationists: Coexistence Through Management and Research, eds R. L. Knight, and K. J. Gutzwiller (Washington, DC: Island Press), 297–312.
Osterrieder, S. K., Kent, C. S., and Robinson, R. W. (2017). Responses of Australian sea lions, Neophoca cinerea, to anthropogenic activities in the Perth metropolitan area, Western Australia. Aquat. Conserv. Mar. Freshw. Ecosyst. 27, 414–435. doi: 10.1002/aqc.2668
Palka, D. L., and Hammond, P. S. (2001). Accounting for responsive movement in line transect estimates of abundance. Can. J. Fish. Aquat. Sci. 58, 777–787. doi: 10.1139/f01-024
Palomo, P. B., Mullor, R. S., and Rodriguez, A. M. (2014). “Reduction of the underwater radiated noise by ships: new shipbuilding challenge. The vessels “Ramoìn Margalef” and “Aìngeles Alvarinþo” as technological references of how to build silent vessels,” in Paper Presented at the Transport Research Arena 2014 Conference, Paris.
Papale, E., Gamba, M., Perez-Gil, M., Martin, V. M., and Giacoma, C. (2015). Dolphins adjust species-specific frequency parameters to compensate for increasing background noise. PLoS One 10:e0121711. doi: 10.1371/journal.pone.0121711
Parks, S. E., Clark, C. W., and Tyack, P. L. (2007a). Short- and long-term changes in right whale calling behavior: the potential effects of noise on acoustic communication. J. Acoust. Soc. Am. 122, 3725–3731. doi: 10.1121/1.2799904
Parks, S. E., Ketten, D. R., O’Malley, J. T., and Arruda, J. (2007b). Anatomical predictions of hearing in the north Atlantic right whale. Anat. Rec. Adv. Integ. Anat. Evol. Biol. 290, 734–744. doi: 10.1002/Ar.20527
Parks, S. E., Johnson, M., Nowacek, D., and Tyack, P. L. (2011). Individual right whales call louder in increased environmental noise. Biol. Lett. 7, 33–35. doi: 10.1098/rsbl.2010.0451
Parks, S. E., Urazghildiiev, I., and Clark, C. W. (2009). Variability in ambient noise levels and call parameters of North Atlantic right whales in three habitat areas. J. Acoust. Soc. Am. 125, 1230–1239. doi: 10.1121/1.3050282
Payne, R., and Webb, D. (1971). Orientation by means of long range acoustic signaling in baleen whales. Ann. N. Y. Acad. Sci. 188, 110–141. doi: 10.1111/j.1749-6632.1971.tb13093.x
Pérez-Jorge, S., Gomes, I., Hayes, K., Corti, G., Louzao, M., Genovart, M., et al. (2016). Effects of nature-based tourism and environmental drivers on the demography of a small dolphin population. Biol. Conserv. 197, 200–208. doi: 10.1016/j.biocon.2016.03.006
Pirotta, E., Harwood, J., Thompson, P. M., New, L., Cheney, B., Arso, M., et al. (2015a). Predicting the effects of human developments on individual dolphins to understand potential long-term population consequences. Proc. R. Soc. Lond. Ser. B Biol. Sci. 282:20152109. doi: 10.1098/rspb.2015.2109
Pirotta, E., Merchant, N. D., Thompson, P. M., Barton, T. R., and Lusseau, D. (2015b). Quantifying the effect of boat disturbance on bottlenose dolphin foraging activity. Biol. Conserv. 181, 82–89. doi: 10.1016/j.biocon.2014.11.003
Pirotta, E., Milor, R., Quick, N., Moretti, D., Di Marzio, N., Tyack, P., et al. (2012). Vessel noise affects beaked whale behavior: results of a dedicated acoustic response study. PLoS One 7:e42535. doi: 10.1371/journal.pone.0042535
Pirotta, E., New, L., Harwood, J., and Lusseau, D. (2014). Activities, motivations and disturbance: an agent-based model of bottlenose dolphin behavioral dynamics and interactions with tourism in Doubtful Sound, New Zealand. Ecol. Model. 282, 44–58. doi: 10.1016/j.ecolmodel.2014.03.009
Popov, V. V., and Supin, A. Y. (1990). “Electrophysiological studies on hearing in some cetaceans and a manatee,” in Sensory Abilities of Cetaceans: Laboratory and Field Evidence, eds J. A. Thomas, and R. A. Kastelein (New York, NY: Plenum Press), 405–415. doi: 10.1007/978-1-4899-0858-2_27
Putland, R. L., Merchant, N. D., Farcas, A., and Radford, C. A. (2018). Vessel noise cuts down communication space for vocalizing fish and marine mammals. Glob. Change Biol. 24, 1708–1721. doi: 10.1111/gcb.13996
Rako, N., Fortuna, C. M., Holcer, D., Mackelworth, P., Nimak-Wood, M., Pleslić, G., et al. (2013). Leisure boating noise as a trigger for the displacement of the bottlenose dolphins of the Cres–Lošinj archipelago (northern Adriatic Sea, Croatia). Mar. Pollut. Bull. 68, 77–84. doi: 10.1016/j.marpolbul.2012.12.019
Rako Gospić, N., and Picciulin, M. (2016). Changes in whistle structure of resident bottlenose dolphins in relation to underwater noise and boat traffic. Mar. Pollut. Bull. 105, 193–198. doi: 10.1016/j.marpolbul.2016.02.030
Richardson, J. W., Fraker, M. A., Würsig, B., and Wells, R. S. (1985). Behaviour of bowhead whales Balaena mysticetus summering in the Beaufort Sea: reactions to industrial activities. Biol. Conserv. 32, 195–230. doi: 10.1016/0006-3207(85)90111-9
Richardson, W. J., Buchanan, R. A., Clark, C. W., Dorsey, E. M., and Fraker, M. A. (1982). Behavior, Disturbance Responses, and Feeding of Bowhead Whales Balaena mysticetus in the Beaufort Sea, 1980-1981 (No. PB-86-152170/XAB United States NTIS, PC A20/MF A01. GRA English). Bryan, TX: LGL Ecological Research Associates, Inc.
Richardson, W. J., Greene, C. R., Malme, C. I., and Thomson, D. H. (1995). Marine Mammals and Noise. San Diego, CA: Academic Press.
Richardson, W. J., Greene, C. R. J., Koski, W. R., Malme, C. I., Miller, G. W., and Smultea, M. A. (1990). Acoustic Effects of Oil Production Activities on Bowhead and White Whales Visible During Spring Migration Near Pt. Barrow, Alaska—1989 Phase. Herndon, VA: LGL Ltd.
Rolland, R. M., Parks, S. E., Hunt, K. E., Castellote, M., Corkeron, P. J., Nowacek, D. P., et al. (2012). Evidence that ship noise increases stress in right whales. Proc. R. Soc. Lond. Ser. B Biol. Sci. 279, 2363–2368. doi: 10.1098/rspb.2011.2429
Rycyk, A. M., Deutsch, C. J., Barlas, M. E., Hardy, S. K., Frisch, K., Leone, E. H., et al. (2018). Manatee behavioral response to boats. Mar. Mamm. Sci. 34, 924–962. doi: 10.1111/mms.12491
Scarpaci, C., Bigger, S. W., Corkeron, P., and Nugegoda, D. (2000). Bottlenose dolphins (Tursiops truncatus) increase whistling in the presence of ‘swim-with-dolphin’ tour operations. J. Cetacean Res. Manag. 2, 183–185.
Scheifele, P. M., Andrew, S., Cooper, R. A., Darre, M., Musiek, F. E., and Max, L. (2005). Indication of a Lombard vocal response in the St. Lawrence River beluga. J. Acoust. Soc. Am. 117, 1486–1492. doi: 10.1121/1.1835508
Schorr, G. S., Falcone, E. A., Moretti, D. J., and Andrews, R. D. (2014). First long-term behavioral records from Cuvier’s beaked whales (Ziphius cavirostris) reveal record-breaking dives. PLoS One 9:e92633. doi: 10.1371/journal.pone.0092633
Scrimger, P., and Heitmeyer, R. M. (1991). Acoustic source-level measurements for a variety of merchant ships. J. Acoust. Soc. Am. 89, 691–699. doi: 10.1121/1.1894628
Shockley, R. C., Northrop, J., Hansen, P. G., and Hartdegen, C. (1982). SOFAR propagation paths from Australia to Bermuda: comparison of signal speed algorithms and experiments. J. Acoust. Soc. Am. 71, 51–60. doi: 10.1121/1.387250
Sidman, C. F., and Fik, T. J. (2005). Modeling spatial patterns of recreational boaters: vessel, behavioral, and geographic considerations. Leisure Sci. 27, 175–189. doi: 10.1080/01490400590912079
Simard, Y., Roy, N., Gervaise, C., and Giard, S. (2016). Analysis and modeling of 255 source levels of merchant ships from an acoustic observatory along St. Lawrence Seaway. J. Acoust. Soc. Am. 140, 2002–2018. doi: 10.1121/1.4962557
Simpson, S. D., Radford, A. N., Nedelec, S. L., Ferrari, M. C. O., Chivers, D. P., McCormick, M. I., et al. (2016). Anthropogenic noise increases fish mortality by predation. Nat. Commun. 7:10544. doi: 10.1038/ncomms10544
Sivle, L. D., Kvadsheim, P. H., Cure, C., Isojunno, S., Wensveen, P. J., Lam, F.-P. A., et al. (2015). Severity of expert-identified behavioural responses of Humpback Whale, Minke Whale, and Northern Bottlenose Whale to naval sonar. Aquat. Mamm. 41, 469–502. doi: 10.1578/AM.41.4.2015.469
Sivle, L. D., Wensveen, P. J., Kvadsheim, P. H., Lam, F. P. A., Visser, F., Curé, C., et al. (2016). Naval sonar disrupts foraging in humpback whales. Mar. Ecol. Prog. Ser. 562, 211–220. doi: 10.3354/meps11969
Slabbekoorn, H., Bouton, N., van Opzeeland, I., Coers, A., ten Cate, C., and Popper, A. N. (2010). A noisy spring: the impact of globally rising underwater sound levels on fish. Trends Ecol. Evol. 25, 419–427. doi: 10.1016/j.tree.2010.04.005
Smith, A. M., and Smith, B. D. (1998). Review of status and threats to river cetaceans and recommendations for their conservation. Environ. Rev. 6, 189–206. doi: 10.1139/a99-002
Southall, B. L., Bowles, A. E., Ellison, W. T., Finneran, J. J., Gentry, R. L., Greene, C. R. J., et al. (2007). Marine mammal noise exposure criteria: initial scientific recommendations. Aquat. Mamm. 33, 411–521. doi: 10.1080/09524622.2008.9753846
Southall, B. L., Finneran, J. J., Reichmuth, C., Nachtigall, P. E., Ketten, D. R., Bowles, A. E., et al. (2019). Marine mammal noise exposure criteria: updated scientific recommendations for residual hearing effects. Aquat. Mamm. 45, 125–232. doi: 10.1578/AM.45.2.2019.125
Stafford-Bell, R., Scarr, M., and Scarpaci, C. (2012). Behavioural responses of the Australian Fur Seal (Arctocephalus pusillus doriferus) to vessel traffic and presence of swimmers in Port Phillip Bay, Victoria, Australia. Aquat. Mamm. 38, 241–249. doi: 10.1578/AM.38.3.2012.241
Stamation, K. A., Croft, D. B., Shaughnessy, P. D., Waples, K. A., and Briggs, S. V. (2010). Behavioral responses of humpback whales (Megaptera novaeangliae) to whale-watching vessels on the southeastern coast of Australia. Mar. Mamm. Sci. 26, 98–122. doi: 10.1111/j.1748-7692.2009.00320.x
Steckenreuter, A., Möller, L., and Harcourt, R. (2012). How does Australia’s largest dolphin-watching industry affect the behaviour of Indo-Pacific bottlenose dolphins? J. Environ. Manag. 97, 14–21. doi: 10.1016/j.jenvman.2011.11.002
Stensland, E., and Berggren, P. (2007). Behavioural changes in female Indo-Pacific bottlenose dolphins in response to boat-based tourism. Mar. Ecol. Prog. Ser. 332, 225–234. doi: 10.3354/meps332225
Strasberg, M. (1979). Nonacoustic noise interference in measurements of infrasonic ambient noise. J. Acoust. Soc. Am. 66, 1487–1493. doi: 10.1121/1.383543
Symons, J., Pirotta, E., and Lusseau, D. (2014). Sex differences in risk perception in deep-diving bottlenose dolphins leads to decreased foraging efficiency when exposed to human disturbance. J. Appl. Ecol. 51, 1584–1592. doi: 10.1111/1365-2664.12337
Tennessen, J. B., and Parks, S. E. (2016). Acoustic propagation modeling indicates vocal compensation in noise improves communication range for North Atlantic right whales. Endanger. Species Res. 30, 225–237. doi: 10.3354/esr00738
Terhune, J. M., Stewart, R. E. A., and Ronald, K. (1979). Influence of vessel noises on underwater vocal activity of harp seals. Can. J. Zool. 57, 1337–1338. doi: 10.1139/z79-170
Thode, A., Straley, J., Tiemann, C. O., Folkert, K., and O’Connell, V. (2007). Observations of potential acoustic cues that attract sperm whales to longline fishing in the Gulf of Alaska. J. Acoust. Soc. Am. 122, 1265–1277. doi: 10.1121/1.2749450
Thompson, P. M., Brookes, K. L., Graham, I. M., Barton, T. R., Needham, K., Bradbury, G., et al. (2013). Short-term disturbance by a commercial two-dimensional seismic survey does not lead to long-term displacement of harbour porpoises. Proc. R. Soc. Lond. Ser. B Biol. Sci. 280:20132001. doi: 10.1098/rspb.2013.2001
Tockner, K., Pusch, M., Borchardt, D., and Lorang, M. S. (2010). Multiple stressors in coupled river–floodplain ecosystems. Freshw. Biol. 55, 135–151. doi: 10.1111/j.1365-2427.2009.02371.x
Tournadre, J. (2014). Anthropogenic pressure on the open ocean: the growth of ship traffic revealed by altimeter data analysis. Geophys. Res. Lett. 41, 7924–7932. doi: 10.1002/2014gl061786
Trevorrow, M. V., Vasiliev, B., and Vagle, S. (2008). Directionality and maneuvering effects on a surface ship underwater acoustic signature. J. Acoust. Soc. Am. 124, 767–778. doi: 10.1121/1.2939128
Tripovich, J. S., Hall-Aspland, S., Charrier, I., and Arnould, J. P. Y. (2012). The behavioural response of Australian Fur seals to motor boat noise. PLoS One 7:e37228. doi: 10.1371/journal.pone.0037228
Tsujii, K., Akamatsu, T., Okamoto, R., Mori, K., Mitani, Y., and Umeda, N. (2018). Change in singing behavior of humpback whales caused by shipping noise. PLoS One 13:e0204112. doi: 10.1371/journal.pone.0204112
U.S. Department of Homeland Security (2018). 2017 Recreational Boating Statistics (Report No. COMDTPUB P16754.31). Washington, DC: U.S. Department of Homeland Security.
United Nations Conference on Trade and Development [UNCTAD] (2018). Review of Maritime Transport 2018 (No. 9211128412). Geneva: United Nations Conference on Trade and Development.
Van Parijs, S. M., and Corkeron, P. (2001). Boat traffic affects the acoustic behaviour of Pacific humpback dolphins, Sousa chinensis. J. Mar. Biol. Assoc. U. K. 81, 533–538. doi: 10.1017/S0025315401004180
Veirs, S., Veirs, V., and Wood, J. D. (2016). Ship noise extends to frequencies used for echolocation by endangered killer whales. PeerJ 4:e1657. doi: 10.7717/peerj.1657
Wale, M. A., Simpson, S. D., and Radford, A. N. (2013). Size-dependent physiological responses of shore crabs to single and repeated playback of ship noise. Biol. Lett. 9:20121194. doi: 10.1098/rsbl.2012.1194
Wang, Z., Akamatsu, T., Mei, Z., Dong, L., Imaizumi, T., Wang, K., et al. (2015). Frequent and prolonged nocturnal occupation of port areas by Yangtze finless porpoises (Neophocaena asiaeorientalis): forced choice for feeding? Integ. Zool. 10, 122–132. doi: 10.1111/1749-4877.12102
Wang, Z., Akamatsu, T., Wang, K., and Wang, D. (2014). The diel rhythms of biosonar behavior in the Yangtze finless porpoise (Neophocaena asiaeorientalis asiaeorientalis) in the Port of the Yangtze River: the correlation between prey availability and boat traffic. PLoS One 9:e97907. doi: 10.1371/journal.pone.0097907
Wensveen, P. J., Kvadsheim, P. H., Lam, F.-P. A., von Benda-Beckmann, A. M., Sivle, L. D., Visser, F., et al. (2017). Lack of behavioural responses of humpback whales (Megaptera novaeangliae) indicate limited effectiveness of sonar mitigation. J. Exp. Biol. 220, 4150–4161. doi: 10.1242/jeb.161232
Widmer, W. M., and Underwood, A. J. (2004). Factors affecting traffic and anchoring patterns of recreational boats in Sydney Harbour, Australia. Landscape Urban Plann. 66, 173–183. doi: 10.1016/S0169-2046(03)00099-9
Williams, A. O., and Horne, W. (1967). Axial focusing of sound in the SOFAR channel. J. Acoust. Soc. Am. 41, 189–198. doi: 10.1121/1.1910316
Williams, R., Erbe, C., Ashe, E., Beerman, A., and Smith, J. (2014). Severity of killer whale behavioural responses to ship noise: a dose-response study. Mar. Pollut. Bull. 79, 254–260. doi: 10.1016/j.marpolbul.2013.12.004
Williams, R., Erbe, C., Ashe, E., and Clark, C. W. (2015). Quiet(er) marine protected areas. Mar. Pollut. Bull. 100, 154–161. doi: 10.1016/j.marpolbul.2015.09.012
Williams, R., Trites, A. W., and Bain, D. E. (2002). Behavioural responses of killer whales (Orcinus orca) to whale-watching boats: opportunistic observations and experimental approaches. J. Zool. 256, 255–270. doi: 10.1017/S0952836902000298
Williams, T. M., Kendall, T. L., Richter, B. P., Ribeiro-French, C. R., John, J. S., Odell, K. L., et al. (2017). Swimming and diving energetics in dolphins: a stroke-by-stroke analysis for predicting the cost of flight responses in wild odontocetes. J. Exp. Biol. 220, 1135–1145. doi: 10.1242/jeb.154245
Wisniewska, D. M., Johnson, M., Teilmann, J., Rojano-Doñate, L., Shearer, J., Sveegaard, S., et al. (2016). Ultra-high foraging rates of harbor porpoises make them vulnerable to anthropogenic disturbance. Curr. Biol. 26, 1441–1446. doi: 10.1016/j.cub.2016.03.069
Wisniewska, D. M., Johnson, M., Teilmann, J., Siebert, U., Galatius, A., Dietz, R., et al. (2018). High rates of vessel noise disrupt foraging in wild harbour porpoises (Phocoena phocoena). Proc. R. Soc. B Biol. Sci. 285:20172314. doi: 10.1098/rspb.2017.2314
Wong, B. B. M., and Candolin, U. (2015). Behavioral responses to changing environments. Behav. Ecol. 26, 665–673. doi: 10.1093/beheco/aru183
Keywords: auditory masking, chronic noise exposure, marine watercraft, ship noise emission, commercial shipping, marine mammal, behavioral response
Citation: Erbe C, Marley SA, Schoeman RP, Smith JN, Trigg LE and Embling CB (2019) The Effects of Ship Noise on Marine Mammals—A Review. Front. Mar. Sci. 6:606. doi: 10.3389/fmars.2019.00606
Received: 05 June 2019; Accepted: 11 September 2019;
Published: 11 October 2019.
Edited by:
Annette Breckwoldt, Leibniz Centre for Tropical Marine Research (LG), GermanyReviewed by:
Frants Havmand Jensen, Woods Hole Oceanographic Institution, United StatesCraig Aaron Radford, The University of Auckland, New Zealand
Copyright © 2019 Erbe, Marley, Schoeman, Smith, Trigg and Embling. This is an open-access article distributed under the terms of the Creative Commons Attribution License (CC BY). The use, distribution or reproduction in other forums is permitted, provided the original author(s) and the copyright owner(s) are credited and that the original publication in this journal is cited, in accordance with accepted academic practice. No use, distribution or reproduction is permitted which does not comply with these terms.
*Correspondence: Christine Erbe, c.erbe@curtin.edu.au