- 1Guanghua School of Stomatology, Hospital of Stomatology, Sun Yat-Sen University, Guangzhou, China
- 2Guangdong Provincial Key Laboratory of Stomatology, Guangzhou, China
- 3Department of Microbiology and Immunology, Vagelos College of Physicians and Surgeons, Columbia University, New York, NY, United States
- 4Department of Medicine, Milton S. Hershey Medical Center at Penn State University, Pennsylvania, PA, United States
- 5Center for Clinic Immunology, Third Affiliated Hospital at Sun Yat-Sen University, Guangzhou, China
Tetraspanins are transmembrane proteins that modulate multiple diverse biological processes, including signal transduction, cell–cell communication, immunoregulation, tumorigenesis, cell adhesion, migration, and growth and differentiation. Here, we provide a systematic review of the involvement of tetraspanins and their partners in the regulation and function of B cells, including mechanisms associated with antigen presentation, antibody production, cytokine secretion, co-stimulator expression, and immunosuppression. Finally, we direct our focus to the signaling mechanisms, evolutionary conservation aspects, expression, and potential therapeutic strategies that could be based on tetraspanins and their interacting partners.
Origin, Development, Features, and Functions of B Cells
Origin, Subtypes, and Development of B Cells
Conventional B cells—a type of white blood cell—were first defined in 1965 by Cooper (1). They originate from hematopoietic stem cells in mammalian bone marrow or in the bursa of Fabricius of birds, where they pass through several developmental stages and become IgM+ immature B cells capable of recognizing antigen (1, 2). The immature IgM+ B cells subsequently migrate to secondary lymphoid tissues and develop into three groups of mature naïve B cells: follicular B cells, marginal zone B cells (MZB), and B-1b cells. When bound with antigen, mature naïve B cells are activated, selected, and differentiated into plasmablasts and then antibody producing plasma B cells. These are conventional B cells and also named as B-2 cells. There are additional B cell populations (named B-1 cells) generated in the fetal liver or spleen which undergo self-renewal in the periphery and secrete IgM and IgG3 natural antibodies to facilitate immune responses. B-1 cells have a distinct developmental lineage from B-2 cells. The exact origin and development of B-1 cells is uncertain (3). Accumulated evidence indicates the existence of yet other B cells, named regulatory B cells (Breg), and associates their function with suppression of immune responses. Whether Breg is a distinct lineage of B cells is still unknown (4). More details of B cell development stages and B cell subsets are summarized in Figure 1.
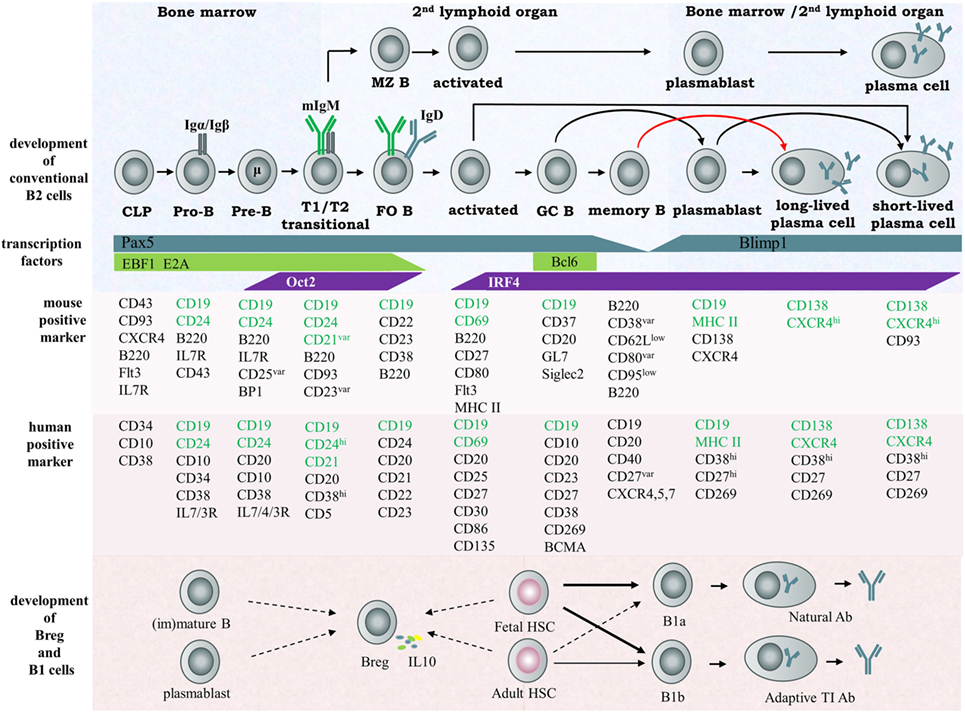
Figure 1. Scheme of B cell development and subsets in humans and mice. According to their individualized origin, surface marker, anatomic localization and functional property, B lymphocytes can be divided into several subsets, including B-1a cell, 1b cell, Breg cell, and B2 cell, the latter considered the conventional B cell. In the early stage, B cells differentiate from hematopoietic precursors into pro-B, pre-B within the bone marrow, then migrate to the spleen and progress through the transitional T1 and T2 stages. These immature cells then differentiate into FO or MZ naïve B cells depending on their special B cell receptor. MZ B cells rapidly develop into plasma cells secreting IgM during the early stage of pathogen infection and function as the first defense line against blood-borne pathogens. FO B cells enter germinal centers and undergo class switch recombination (CSR), somatic hypermuation (SHM), and affinity maturation and terminally differentiate into memory B cells or plasma cells. The important transcription factors and surface markers in human or murine involved in conventional B cell development are shown. The origin of regulatory B cells and B1 cells is still not identified. Here, the solid arrows represent known developmental routes while the dashed arrows represent possible development directions. Abbreviation: CLP, common lymphoid progenitor.
Functions of B Cells
B cells play pivotal roles in the immune system. As outlined in Figure 2, B cells can promote an immune response through presentation of antigens and production of diverse antibodies, proinflammatory cytokines, and co-stimulators (5). B cells can also suppress immune responses through a variety of mechanisms, such as production of IL-10, IL-35, and TGFb1, induction of regulatory T cells, and clearance of auto antigens (4). Many cell surface molecules are involved in B cell development and function. Tetraspanins are one such important family of molecules.
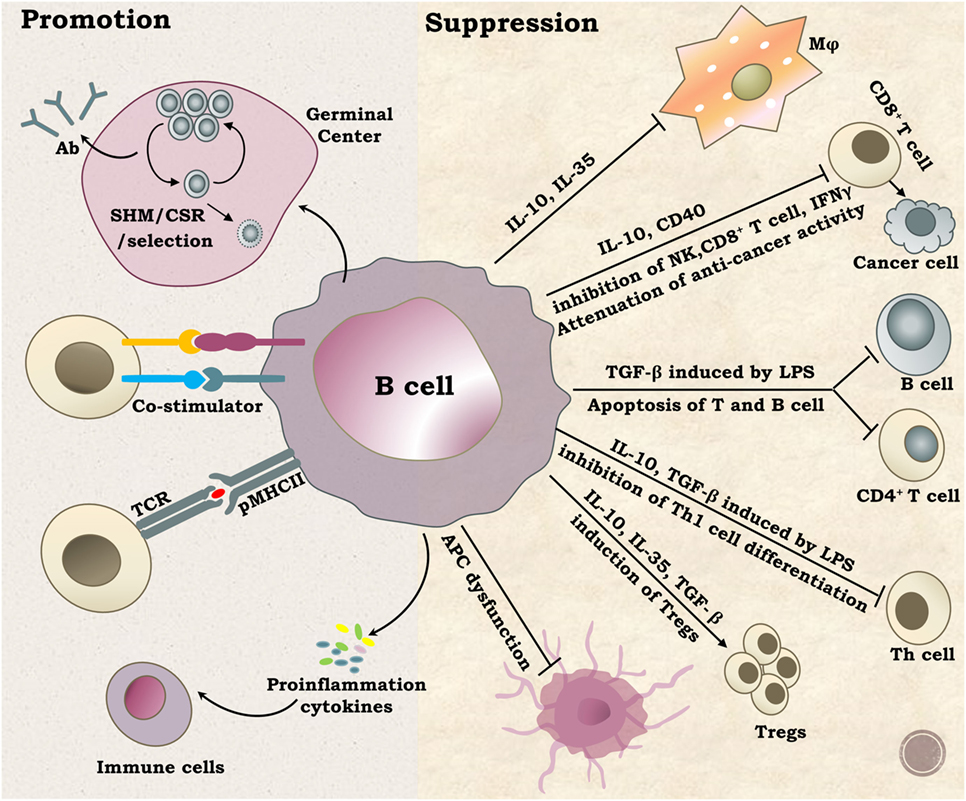
Figure 2. Functions of B cells. B lymphocytes perform diverse and complex roles in vivo mainly through promotion or suppression of immune responses. The well-known function of B cells is antibody production by plasma cells after SHM selection and CSR. B cells can also activate other immune cells by providing co-stimulation signals, serving as antigen-presenting cells or secreting multiple proinflammation cytokines, such as IL2, IL4, IL6, TNF-α, and INF-γ. On the other hand, B cells can suppress immune responses by regulating certain types of immune cells through multiple ways. Abbreviations: SHM, somatic hypermutation; CSR, class switch recombination; Ab, antibody.
General Features and Functions of Tetraspanins
Structure and Evolutionary Conservation of Tetraspanins
Tetraspanins belong to a protein family in which members contain intracellular N- and C-termini, two extracellular domains (EC1 and EC2), and specifically four transmembrane domains (Figure 3A; 6, 7). Each phylum has evolved its own particular tetraspanins with distinction in the variety and abundance in different species. Despite this, the chemical composition of tetraspanins is highly conserved among species with four or more cysteine residues in a highly conserved “CCG” motif in the EC2 domain (8). There are 33 tetraspanins found in humans (Tables 1 and 2) and most of them preserve the characteristics of the ancient sequence in domain EC2.
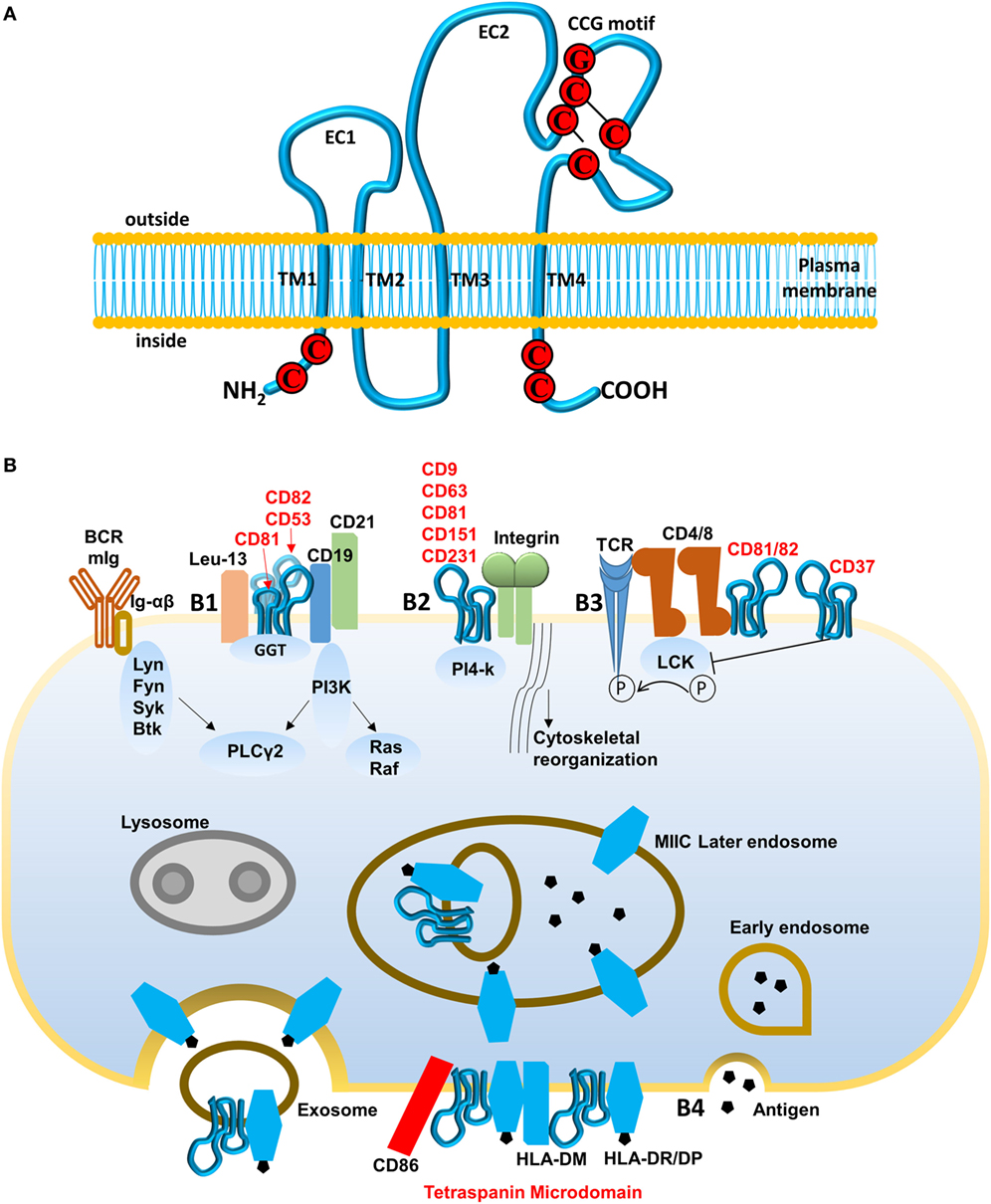
Figure 3. Structure of tetraspanin and pathways regulated by tetraspanins. (A) Schematic diagram of tetraspanins. Tetraspanins present four transmembrane domains (TMs) intracellular N- and C-termini and two extracellular domains (EC1 and EC2). CCG motif is formed with cysteine–cysteine–glycine (marked by red) and two disulfide bonds (marked by black line). (B) Pathways regulated by tetraspanins. (B1) B cell receptor (BCR) activation mediated by CD19–CD81–CD21 complex. Ig-α/β receive signals and are phosphorylated by Src kinase (Lyn, Fyn, or Btk), then recruit Syk kinase for initiating downstream signal pathway PLCγ2, Ras/Raf. Tetraspanin CD81, associated with CD52 and CD82, binds C19/CD21/Leu-13 signal-transducing complex and actives PLCγ2 through PI3K, which lowers the threshold for BCR signaling. (B2) Integrin-mediated cell adhesion. PI4-k, associated with various tetraspanins (CD9, CD63, CD81, CD151, and CD231), interacts with and promotes integrins to modulate cell spread and migration. (B3) T cell-B cell contact (TCR) pathway mediated by tetraspanins CD81, CD82, and CD37. CD4 and CD8 associate with Lck kinase to activate TCR signaling but their interaction with CD81, CD82, and CD37 interferes with phosphorylation of Lck kinase and may inhibit TCR signaling. (B4) Endocytic pathway for antigen presentation. Recognized antigens are internalized, processed, and loaded onto MHC class II molecules during the late endosome stage. Major histocompatibility complex class II mediates transport to the cell surface and the release of exosomes. Tetraspanin microdomains in antigen-presenting cell membranes are enriched for specific peptide–MHC class II complexes, peptide editor human leukocyte antigen-DM, and CD86 among other proteins. This selecting domain probably facilitates antigen presentation and T-cell activation, increasing MHC avidity.
General Interactions Among Tetraspanins and Their Partners
Tetraspanins act as scaffold proteins to anchor multiple proteins—including other tetraspanins, partners of tetraspanins, and other proteins—to one area of the cell membrane, and form a tetraspanin-enriched microdomain (TEM) or tetraspanins web (10, 11). A recent study with super resolution microscopy provided a close view of TEM and demonstrated that TEM is composed of individual nanoclusters (<120 nm). There are no more than 10 CD53 molecules in a single tetraspanin cluster of CD53. The study also evaluated the distances between the individual clusters, including CD53, CD37, CD81, CD82, and the tetraspanin partners such as CD19 and major histocompatibility complex class II (MHC II) (12). Based on the sensitivity and stringency to different detergents, the interactions of tetraspanins and partners in TEM were classified into three categories (13, 14). This model allows for dynamic and adaptable interactions between tetraspanins and other surface proteins based on a descriptive categorization without correlation to functionality in the living cell. A recent review proposed a new applaudable classification of tetraspanin interactions based on their function in the formation of TEM: interactions (a) necessary to maintain tetraspanin structure, (b) that support tetraspanin web formation, (c) that add functional partners to the web, and (d) that facilitate intracellular events (6).
Three hypothetical models could be postulated to decipher the ways that tetraspanin microdomains enhance or regulate cellular signals and exert effects on fundamental biological processes. One model is that tetraspanins be considered a transmembrane linker connecting and augmenting signal transduction between membrane partners and intracellular-signaling proteins (15). Another model could propose that tetraspanins are involved in gathering partner membrane proteins which subsequently result in increased avidity and/or enhanced interaction with their ligands (16). The third hypothesis is that tetraspanins function as regulators by sequestering partners from signal transduction (17) thus preventing inappropriate signals and responses in resting cells. Without favoring any of these models at the present time, we now direct our attention to signal transduction and/or regulation by tetraspanins in immune cells (Figure 3B).
Interaction of Tetraspanin CD81 and Its Partners in B Cell Receptor (BCR) Activation Pathway
In B cells, tetraspanins CD81 interacts with the CD19/CD21 signal-transducing complex to lower the threshold for BCR signaling (Figure 3B1). The multiprotein complex BCR consists of two parts: membrane immunoglobulin (Ig) with integral membrane domain, and signal transduction moiety Ig-α/Ig-β (also known as CD79A/CD79B) heterodimer tethered by disulfide bridges (18). When antigen binds to Ig, Src family kinase-like Lyn phosphorylates immuno-receptor tyrosine-based activation motif residues on the cytoplasmic tails of Ig-α/Ig-β, sequentially recruiting and activating Syk and Btk kinases, then initiating downstream signaling cascades of the Ras–MAPK pathway and PLCγ2 (19). The CD19/CD21 complex is thought to augment BCR signaling by decreasing the signaling threshold for B cell activation (20). Indeed, the direct association of tetraspanin CD81 with CD19 as a part of the CD19/CD21/Leu-13 complex is critical for both assembly and localization of this complex (20) and CD81-deficient B cells have been found to have reduced expression of CD19 and impaired B cell signaling (21). Furthermore, probably through interaction of tetraspanins CD9, CD53, CD82 with CD81, the CD19/CD21 complex has an additional layer of control over B cell signal regulation through formation of a CD19/CD21 complex with additional functional proteins, such as glutathione and oxidative homeostasis-related enzyme γ-glutamyl transpeptidase GGT (22).
Function of Tetraspanin/Integrin Complexes in Signaling Pathway for Cell Migration and Adhesion
Tetraspanins associating with and forming tetraspanin/kinase-integrin complexes are implicated in both leukocyte and cell–cell adhesion (Figure 3B2) by causing signal activation and cytoskeletal reorganization. In B cells, by enhancing tyrosine phosphorylation levels, tetraspanin CD9 promotes β1 integrin-dependent mobility (23). In addition, tetraspanins CD9 as well as CD63, CD81 have been documented to associate with both PI4-kinase and integrin α3β1 in lymphoid cell lines (24). Finally, tetraspanins also have been found to enhance the avidity of integrins for neutrophil motility and T cell–B cell contact (25).
Function of Tetraspanin CD37 and Its Partners in T Cell–B Cell Contact (TCR) Activation Pathway
Tetraspanins are implicated in TCR-induced activation and proliferation (Figure 3B3). Interaction of peptide with the MHC activates the TCR and initiates the downstream signaling cascade of Src kinases Fyn and Lck. Lck subsequently activates the functional proteins involved in T cell activation and proliferation. Interaction of Lck with CD4/CD8 plays crucial roles in this pathway (20); should CD4 associate with tetraspanins CD81/82 then Lck is sequestered from the TCR signaling pathway (26). Additional evidence shows that tetraspanin CD37 is coupled to TCR signal transduction mostly by influencing the dynamics of CD4-Lck distribution to TCR signal associated microdomains (27). Thus, tetraspanins regulate the T cell biologic process by influencing the TCR-CD4/CD8 cascade proximal to Lck mobilization.
Functions of Tetraspanins and Their Partners in Antigen-Presenting Processes
MHC avidity and facilitation of T cell activation is also mediated by tetraspanins (Figure 3B4). Tetraspanins function in antigen-presenting cells (APCs) to assist in the presentation of the MHC–peptide complex to T cells. Tetraspanins CD81, CD37, CD82, CD53, and CD63, tether with MHC and associate with stimulators on exosome vesicles which are MHC II-enriched compartments. After the cell membrane is fused with MHC, the exosomes are released and can act as stimuli for T-cell proliferation (28). But there is an additional way in which tetraspanins work with MHC. Tetraspanin microdomains are enriched for MHC II, CD86, and the class II editor human leukocyte antigen in the membrane of APCs. This complex is referred to as the “CDw78+ microdomain” involved in T cell activation (20).
Through the above-enumerated regulatory pathways, the TEMs form a web for signal transduction from extracellular stimuli to intracellular-signaling components and ultimately regulate multiple biological processes, including cell activation, proliferation, adhesion, migration, and communication, as well as involvement in pathological conditions, such as autoimmune diseases, metastasis, and viral infection (Table S2 in Supplementary Material).
Expression Profiles of Tetraspanins and their Partners on B Cells
Uniquely expressed molecules in certain B cell subsets may serve as markers of the subset or have special function for that particular subpopulation. Systematic analysis of expression of tetraspanins and partners of tetraspanins on B cells may facilitate an understanding of their biological involvement in B cell biology including B cell development and function.
Expression of Tetraspanins on the Surface of B Cells
Most tetraspanins are expressed on B cells but differ in abundance in various B cell subsets at different developmental stages (Figure 4). mRNA transcripts of Tspan2-8, 31, 33, CD9, and CD63 are expressed at high levels in mouse progenitor B cells in the bone marrow but at very low levels, except for CD9 and Tspan31, in other B cell subsets (which mainly exist in periphery lymphoid organs). In contradistinction, CD37, CD53, CD82, and Tspan32 all show similar expression patterns of low level expression in mouse pro-B cells but high level in other B cell subpopulations. On tested human B cells (CD38− naïve B cells, isotype switch memory B cells, and memory B cells), TSPAN3, TSPAN13, CD53, CD37, CD82, CD81, CD63, and TSPAN33 show relatively high levels of mRNA (TPM > 10). TSPAN2, 5, 10, 14, 17, 31, and 32, and UPK1B, ROM1, CD151, and CD9 have detectable mRNA transcripts. But the remaining tetraspanins have no detectable mRNA. In addition, the expression of all tetraspanins except TSPAN19 is detectable in at least one strain of B cell lymphoma cell lines. More expression profiles of tetraspanins can be found in Figure 4 and Table 2.
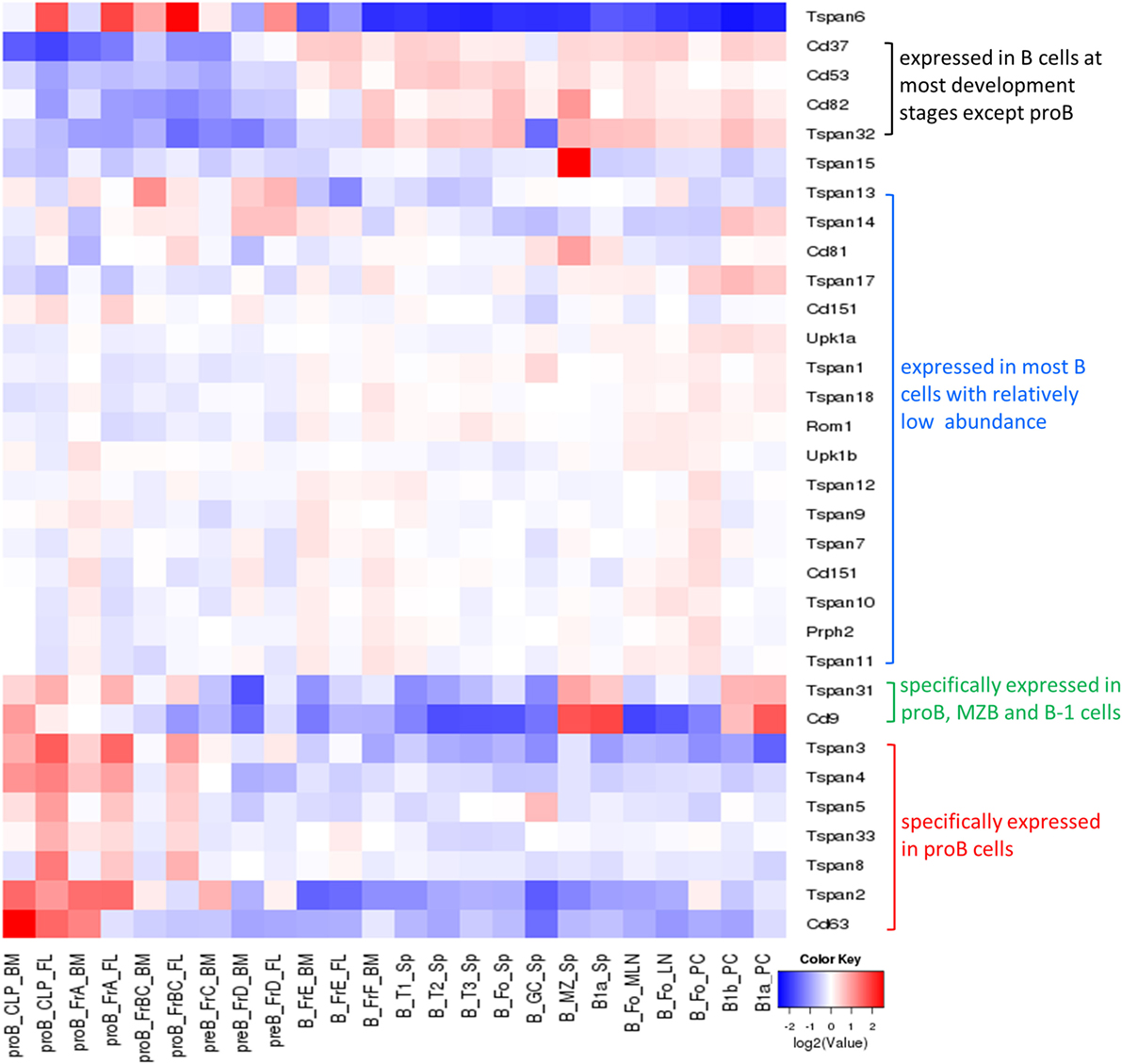
Figure 4. Expression of tetraspanins in murine B cell subsets. The heat map was obtained from http://www.immgen.org/by inputting the list of tetraspanins in “My GeneSet” and choosing B cells as the populations of interest. The gene expression level is determined by Affymetrix microarrays (GEO: GSE15907).
Expression of Tetraspanin Partners on the Surface of B Cells
Affinity capture assays, protein-fragment complementation assays, and two-hybrid tests in the databases of BioGRID (Table S3 in Supplementary Material) and ingenuity pathway analysis (Table 1; Table S2 in Supplementary Material) have allowed for the identification of hundreds of tetraspanin interacting partners. The main cell surface partner proteins of tetraspanins are other tetraspanins, integrins, G-protein coupled receptors, and transmembrane receptors like CD19. After removal of the partners expressed in the cytoplasm and the nucleus, there are 93 membrane proteins which potentially interact with extracellular tetraspanins or tetraspanins on the same membrane (Table S3 in Supplementary Material). Some of the tetraspanins which interact with other tetraspanins include CD151, CD37, CD53, CD63, CD81, CD82, CD9, ROM1, TSPAN2, TSPAN3, and TSPAN12 (Table S3 in Supplementary Material). Most of the partners show high levels of expression in more than one mouse B cell subset (Figure 5). In tested human B cells (CD38− naïve B cells, isotype switched memory B cells, and memory B cells), EZR, ADGRE5, ARF6, MSN, ITGB1, ITGA4, CD44, REEP5, EPN1, CR2, MET, ATP1A1, CD1D, ADAM10, APP, IGSF8, TNFRSF10B, and LGALS9 all show relatively high levels of mRNA (TPM > 10). More data can be found in Figure 5.
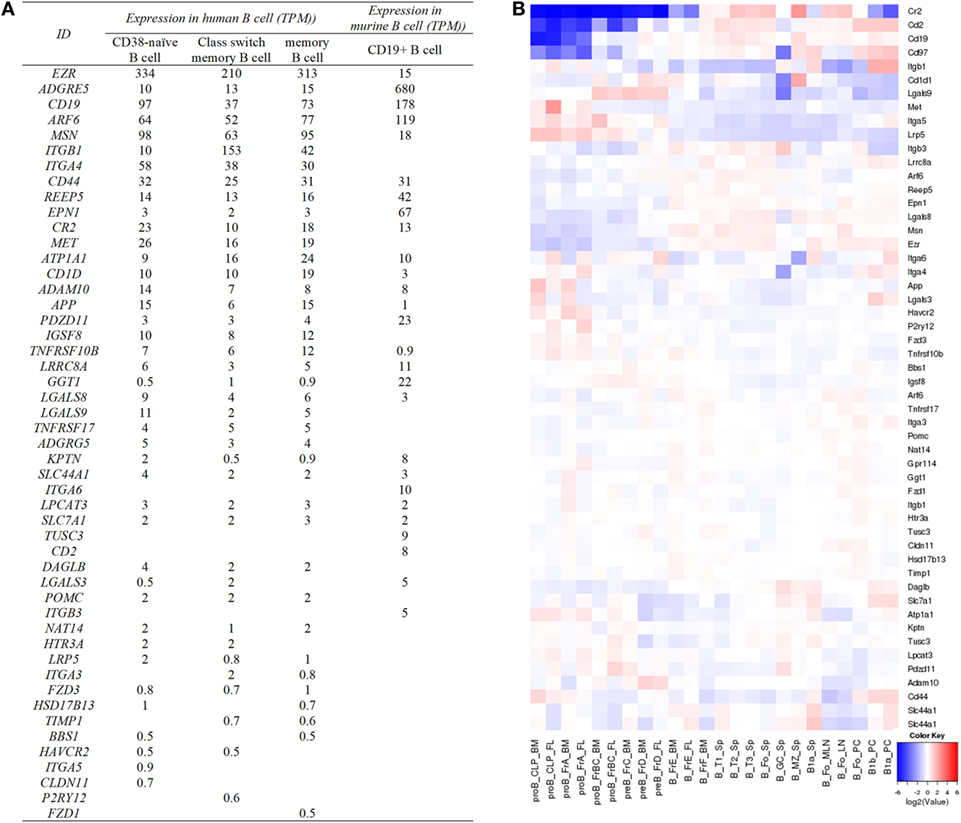
Figure 5. Expression of tetraspanin partners in human and murine B cell subsets. (A) The expression of cell surface partners of human and murine tetraspanins on specific B cells. The partners without TPM values are not listed in the table. Human data are determined by the RNA-seq data generated by the Blueprint Consortium, and murine data are from RNA-Seq CAGE (Cap Analysis of Gene Expression) analysis of mouse cells in RIKEN FANTOM5 project. (B) Expression of tetraspanin partners listed in (A) in murine B cell subsets. The heat map was obtained from http://www.immgen.org/ by inputting the list of cell surface partners of tetraspanin partners in “My GeneSet” and choosing B cells as the populations of interest. Based on the database, ADGRE5, ADGRG5, and CD1D in (A) are shown as Cd97, Gpr114, and Cd1d1 in (B), respectively.
Functions of Tetraspanins in B Cells
Tetraspanins modulate cell adhesion, migration, and invasion which are strongly involved in cancer development and progression (29). The association between tetraspanin expression and cancer prognostic is found in many kinds of cancers (Table 1). In B lymphoma, aberrant expression of CD9, CD81, and CD82 was linked to B-acute lymphoblastic leukemia (30–32). Increased CD37 expression was found in B cell malignancies and thus CD37 antibodies were developed to deplete malignant B cells for the treatment of chronic lymphocytic leukemia (33). The correlation of tumorigenesis and tetraspanins is discussed in another submission for this topic, so here we focus on the functions of tetraspanins and their partners on normal B cell biology without any further discussion of B cell malignancy. Functions of tetraspanins are summarized in Figure 6 and Table 3.
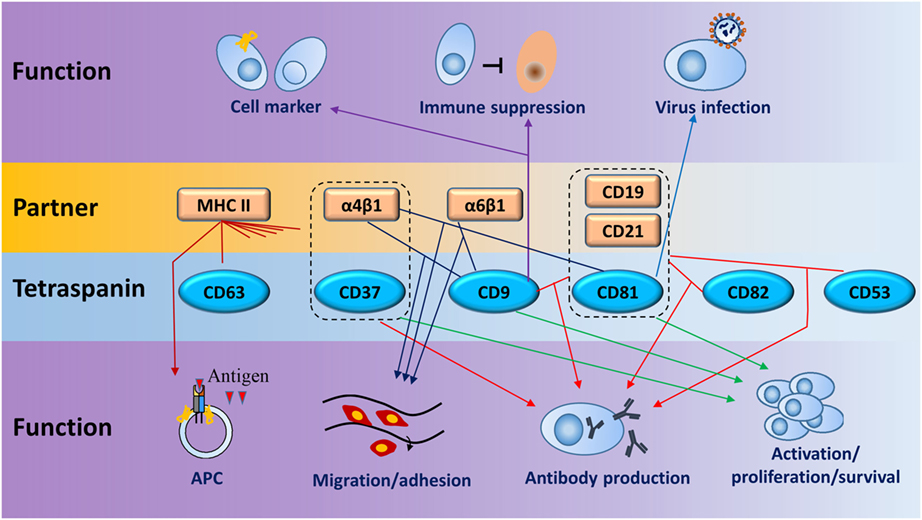
Figure 6. Schematic diagram for main functions of tetraspanins and their partners in B cells. Dotted lines enclose coreceptors formed by tetraspanins and partners. Lines between tetraspanins and partners or coreceptors indicate the interactions which have functions in B cells (line with arrow) in seven biologic processes, such as cell activation, antigen presentation, and antibody production. One tetraspanin or partner can have one or more functions on B cells. Same colored lines with arrows point to similar function or regulation.
Act as Markers Identifying B Cell Subsets
As discussed above (Figure 4; Table 2), since some tetraspanins are enriched in specific B cell subsets, they may be used as markers to identify B cell subsets or diagnostic markers for certain diseases. CD9 is reported to be a special shared marker by B-1 cells, MZB cells, and plasma cells in mice. A study demonstrated that CD9 is expressed by plasma cells in response to antigens independent or dependent on T cells, suggesting CD9 is acquired by T cell dependent plasma cells (34). Another study demonstrated that CD9 is a cell surface marker for precursors of human plasma cells in germinal centers. It is based on the evidence that (1) compared to CD9− cells, CD9+ B cells show higher Blimp-1 but lower Bcl-6 and Pax-5 protein levels, and a faster process of differentiation into plasmablasts in the presence of PC-generating cytokines; (2) expression of CD9 was induced and gradually increased in CD9− GC-B cells under PC generating condition (35). A recent study showed that murine CD9 is a unique cell surface marker identifying IL-10 competent Bregs and their progenitors (36).
Roles in Cell Activation, Proliferation, Survival, and Development
The CD21/CD19/CD81 complex modulates signal transduction events pivotal for development of B lymphocyte and the normal function of humoral immunity. As shown in Figure 1, CD19, a hallmark of B cells, is sustained in its presence on B cells from the earliest pro-B cell stage to plasmablasts during development. CD19 functions as a co receptor of B cells in association with CD21 and CD81. In the complex, CD19 is the signaling molecule bound by Src-family kinases and PI-3 kinase, CD21 binds opsonized foreign antigens, and CD81 is associated with other tetraspanins including CD82, CD63, CD53, and CD9 to enable formation of TEMs (43). CoIP experiments demonstrated association of CD9, CD81, and CD82 with CD19 and digitonin treatment disrupted the CD9/CD19 and CD9/CD81 associations but not the CD81/CD19 association, implying that the association of CD9 with CD19 is through CD81 instead of another molecule. Different proteins including CD19 could be tyrosine phosphorylated which is induced by engagement of CD9, suggesting that CD9 involves B cell activation and differentiation (37). CD81 is one of the key proteins participating in controlling homeostasis of lymphocytes through modulating their proliferation. CD81 KO mice show reduced B1 cells and CD19 expression on B cells although the development of T cells and conventional B cells is normal. Moreover, in CD81 KO mice, the proliferative response of T cells is enhanced following TCR engagement, while proliferation of B cell responding to BCR cross-linking is severely impaired (21). Engagement of CD81 with hepatitis C virus (HCV) envelope protein E2 could protect B cells from apoptosis (56), and induce B cell activation (57, 58) and VH hypermutation (59).
CD9 appears to function in B cell activation and differentiation based on its expression in specific B cell subsets and its functional interaction with CD19. CD9 is reported as a cell surface marker of B-1 B cells, MZ B cells, and plasma cells, but the development of these B cell subsets as well as the humoral immune responses to antigens appear to be normal when CD9 is knocked out (60). A recent study also confirmed that most of the tested markers expressed on total B cells are not significantly altered when CD9 is mutated. Interestingly, however, the frequency of occurrence of IL10 competent Breg (B10 cells) is increased and CD23 expression is reduced on B10 cells when CD9 is knocked out (36). Moreover, CD9 is reported to facilitate interacting with human follicular dendritic cells through the VLA4/VCAM-1 axis and contribute to the survival of germinal center B cells (38).
In addition to its involvement in Ig production, CD53 also contributes to B cell differentiation. A study demonstrated that CD53 is a direct genetic target of EBF-1, a critical transcription factor in early B lymphocyte development. CD53 has functional binding sites for EBF-1 in its promoter elements and can be induced by ectopic expression of EBF-1 (45). CD53 transcripts are enhanced significantly by mitogenic stimulation, implying that CD53 may be involved in the transport of signals important for cell proliferation. Under conditions of serum deprivation, ligation of CD53 in B lymphoma cells triggers an AKT-mediated survival response and prompts a significant reduction in caspase activation and the number of cells that enter apoptosis (61). By using live-cell imaging and gene knockout mice, a recent study demonstrated that CD53 is specifically required for the recruitment of PKCβ (the protein kinase C family member) from cytosol to CD53-enriched TEMs on the plasma membrane to activate PKCβ for antigen-dependent BCR activation, suggesting that TEMs act as signaling hotspots (46).
The tetraspanin CD37 has profound roles in B cell proliferation and survival. CD37 regulates the plasma membrane distribution of α(4)β(1) integrins by controlling their mobility and clustering, a necessary step in activating Akt survival pathways. It is reported that the number of IgG-secreting plasma cells is reduced in lymphoid organs when CD37 is knocked out in mice, possibly due to the impaired association of VCAM-1 to the α(4)β(1) integrin for the Akt survival pathway with the corollary of increased apoptosis of plasma cells in germinal centers (51). In a recent study, CD37 knockout in mice can drive B cell lymphoma progression through constitutive activation of the IL6 pathway by losing the control of suppressor of cytokine signaling 3 (54). Although CD37 is crucial for B cells to survive and provide long lasting immune protection, another study reported that CD37 may trigger a cascade of events resulting in apoptosis when it is tyrosine phosphorylated and binds with signaling factors. The study also found that CD37 mediates SHP1-dependent death via its N-terminal domain, whereas it antagonizes death signals through the C-terminal domain by mediating PI3K-dependent survival (52).
CD82 associates with MHC-I at the cell surface of B cells and could interfere with the capacity of the MHC-I complex to protect targets from NK-mediated cytotoxicity (55). CD63 is reported as a suppressor of exosome production and could regulate exosome-mediated MHC II-dependent T-cell stimulation (48).
Roles in Antibody Production
In addition to its role in B cell proliferation and selection of IgG+ plasma cells, CD37 promotes IgG1 production while inhibiting IgA immune responses in vivo. CD37 deficiency causes a reduction of serum IgG1 levels and alters B cell responses to T cell-dependent antigen under suboptimal costimulatory conditions (62). Besides the reduction in serum IgG1 levels, CD37 deficiency in B cells causes high levels of IL-6 and is directly responsible for the increased IgA+ plasma cell numbers and IgA production levels in CD37−/− mice. CD37−/− mice are better protected from infection by Candida albicans than WT mice due to the increased C. albicans-specific IgA antibody levels. Neutralization of IL-6 in vivo could reverse the enhanced IgA response in CD37−/− mice (63). Therefore, it is not surprising to find that CD37 protects against the development of IgA nephropathy by controlling the formation and deposit of IgA–antigen complexes in the glomerulus (53).
The absence of CD81 on murine B cells causes a defect of antibody responses to T cell-dependent protein antigens and reduces the production of IL-4 that is specific to antigens in both spleens and lymph nodes. A putative ligand on a subpopulation of B and T cells may interact with CD81 to signal IL-4 production (41). The function of CD81 was confirmed in a patient carrying a homozygous mutation of the CD81 gene which caused the syndrome of antibody deficiency by disrupting the CD19 complex in B cells and impairing BCR activation although the CD19 alleles in the patient are normal. Otherwise, the patient had neither overt T cell subset nor functional defects, similar to CD19-deficient patients. Further study revealed that CD19 membrane expression critically depends on CD81 and no cell surface CD19 could be observed on B cells from the patient who had the mutated CD81 (42).
Besides the above proteins, CD53 also plays an important role in activation and differentiation of B cells. CD53 engagement with both the MEM-53 antibody against CD53 and a polyclonal anti-mouse immunoglobulin promotes B cell activation from resting status into the G1 phase, and induces Ig production when treated with T cell supernatant (47).
Immune Suppression
A study has shown that CD9 is a unique cell surface marker for murine B10 cells and their progenitor cells. Moreover, CD9+ B cells are capable of inhibiting contact hypersensitivity mediated by Th1 cells in vivo. Further ex vivo assays demonstrated that CD9 is involved in cross-talk between B cells and T cells, which is required for IL10+ B cells to suppress proliferation of T cells (36). Another study also indicated that IL-10+ Bregs are enriched in a CD9+ B cell subset and their homeostasis is altered by induced allergic asthma. Adoptive transfer of CD9+ B cells in asthmatic mice normalizes lung function in an IL-10-dependent manner via inhibiting inflammation driven by Th2 and Th17 cells (39).
Roles in Virus Infection
Both CD9 and CD63 were identified and found to be transcribed by IgM+ cells in different tissues of rainbow trout (Oncorhynchus mykiss). And the abundance of CD9 transcripts decreased significantly in IgM+ splenocytes when the cells were exposed in vitro to viral hemorrhagic septicemia virus (64). Overexpression of CD9 caused a significantly higher yield of extracellular vesicles and improved the speed and efficiency of lentiviral gene delivery into T and B cells with the lentivirus produced in the CD9 high cells, although the virus titers were not increased. The study indicates an important role for CD9 in membrane fusion, virus infection, and information transfer mediated by extracellular microvesicles (40).
Viral oncogene latent membrane protein 1 (LMP1) accumulates within intraluminal vesicles to avoid degradation and thus constitutively activates NF-κB which is important for EBV persistence in B cells. CD63 associates with LMP1 and facilitates the inclusion of LMP1 into vesicles lacking MHC II. Preclusion of LMP1 assembly within CD63-enriched domains by C-terminal modifications of LMP1 leads to NF-κB overstimulation. Interference through shRNAs against CD63 causes redistribution of LMP1 and leads to a dramatic increase in LMP1-induced NF-κB activity, indicating that CD63 is sensitized to and controls LMP1-mediated NF-κB activation (49).
CD81 plays important roles in HCV infection by acting as a HCV entry factor (65), promoting HCV RNA replication (66), and reducing HCV-induced immune responses (44). B cells expressing CD81 can be infected by HCV and serve as reservoirs for chronic HCV infection (67).
Cell Migration, Adhesion
CD63 plays important roles in cell migration as it can affect the abundance of CXCR4 on the cell surface through IL-21-stimulated endocytosis and endosomal recruitment. Restimulation of activated B cells with T cell-produced IL-21 accelerates CXCR4 internalization by inducing endocytosis-related GRK6 expression. The level of CD63 is strikingly elevated in activated Bcl6-deficient B cells and downregulation of CD63 mRNA with siRNAs upregulates CXCR4 expression on the B cells. Activated B cells treated with Bcl6 inhibitor have a similar phenotype to Bcl6-deficient B cells: increased CD63 mRNA expression and downregulated CXCR4 expression (50). It is reported that CD53 plays an important role in homotypic cell aggregation of lymphocytes and may interfere with lymphocyte activation and cell adhesion. HI29, an anti-CD53 monoclonal antibody, was able to induce homotypic cell aggregation in a B cell strain from a leukocyte adhesion deficiency patient. Moreover, pre-incubation with MEM53, another antibody against CD53, can block such aggregation but anti-CD44 or anti-CD49d mAbs have no blocking effects. Tetraspanins also interact with integrins which function within the area of cellular motility. Ectopic expression of CD9 has been reported to enhance B cell migration via interacting with integrins α6β1 and α4β1 (23). α4β1 on B cells can also be associated with CD81 (68).
Functions of Tetraspanin Partners Expressed on B Cells
The partners of tetraspanins have multiple functions in B cells—including regulation of B cell activation, survival, development, antibody production, virus infection, and signal transduction—through mechanisms which may not be correlated with the interaction between tetraspanins and the partners. More details can be found in Table 4.
Therapy Strategies for Immune Diseases Correlated with Tetraspanins and their Partners
Most studies on employing tetraspanins and their partners as therapy target of diseases involve cancer treatments, a subject outside the scope of this review. For other diseases, there are some strategies using tetraspanins and their partners on B cells as therapy targets, as we explain below.
Depletion of B Cell Subsets, Blockade of Receptors or Crosslinking With Antibodies Against Certain Tetraspanins or Partners
CD21 can be used as a target for depletion of EBV positive B cells as it is a receptor for EBV on B cells. CD19 is a hallmark of B cells and could be used as a target for B cell depletion in the treatment of autoimmune diseases, such as multiple sclerosis, rheumatoid arthritis, and systemic lupus erythematosus (SLE). There is a phase I clinical trial (Identifier: NCT00639834, ClinicalTrials.gov) using anti-CD19 antibody MDX-1342 together with methotrexate for the treatment of patients with rheumatoid arthritis.
CD81 is an entry factor for HCV infection. Monoclonal antibodies with high affinity to CD81 are generated for prevention of HCV infection (108).
TNFRSF17/BCMA is preferentially expressed in CD180− B cells which produce autoantibodies and are significantly increased in SLE (104, 105, 109). TNFRSF17/BCMA and CD180− B cell subsets would be ideal targets for SLE treatment.
CD44 engagement could control CD40L-mediated polyclonal B cell activation (88). Cross-linking of CD53 with antibodies against CD53 promotes activation of resting B cells, speeds up the entrance into the G1 phase of cell cycle, and induces Ig production during the incubation with T cell supernatant (47).
Reduction of Protein Abundance With shRNAs or siRNAs Against Certain Tetraspanins or Partners
Interference through shRNAs against CD63 causes redistribution of LMP1, leads to a dramatic increase in LMP1-induced NF-κB activity, and would benefit treatment of EBV infection (49).
Overexpression or Delivery of Certain Tetraspanins or Partners in B Cells
CD9 expression increases exosome production and promotes lentivirus infection (40), thus CD9 could be overexpressed in the engineered cells producing therapeutic exosomes to enhance the yield of exosomes and the delivery efficiency of exosomes.
Interference of Tetraspanins or Partners With Small Molecules, Inhibitors, or Stimulators of Diseases
ADAM10 improves IgE production via its sheddase activity on CD23, an IgE receptor with low affinity (70). Adam10 increases in the B cells of allergic patients and Th2 prone mice (71) and would help diagnostically in predicting Th2 disease susceptibility. ADAM10 inhibitors could be used for attenuating allergic diseases.
Immunotherapy With Certain B Cell Subsets Defined by Specific Tetraspanins or Partners
IL-10 secreting Breg defined by CD19 and CD9 in mouse (36) or CD19, CD27, and CD38 in human (4) could be enriched, expanded, and then adoptively transferred for treatments of autoimmune diseases.
Author Contributions
FZ, XW, and XH summarized the literature, wrote the manuscript, and prepared figures. GR, SZ, and UB provided critical comments and wrote part of the manuscript. JS supervised all the work and wrote the manuscript.
Conflict of Interest Statement
The authors declare that the research was conducted in the absence of any commercial or financial relationships that could be construed as a potential conflict of interest.
Funding
This research is supported by the Program for Guangdong Introducing Innovative and Entrepreneurial Teams (2016ZT06S252) to JS and SZ; and by NIH/NIAID grants (R01AI099195 and R01AI134988), Leukemia and Lymphoma Society of America award, and Pershing Square Sohn Cancer Research Alliance award to UB.
Supplementary Material
The Supplementary Material for this article can be found online at https://www.frontiersin.org/articles/10.3389/fimmu.2018.01606/full#supplementary-material.
References
2. Melchers F. Checkpoints that control B cell development. J Clin Invest (2015) 125:2203–10. doi:10.1172/JCI78083
3. Montecino-Rodriguez E, Dorshkind K. B-1 B cell development in the fetus and adult. Immunity (2012) 36:13–21. doi:10.1016/j.immuni.2011.11.017
4. Rosser EC, Mauri C. Regulatory B cells: origin, phenotype, and function. Immunity (2015) 42:607–12. doi:10.1016/j.immuni.2015.04.005
5. Shimabukuro-Vornhagen A, Hallek MJ, Storb RF, von Bergwelt-Baildon MS. The role of B cells in the pathogenesis of graft-versus-host disease. Blood (2009) 114:4919–27. doi:10.1182/blood-2008-10-161638
6. van Deventer SJ, Dunlock VE, van Spriel AB. Molecular interactions shaping the tetraspanin web. Biochem Soc Trans (2017) 45:741–50. doi:10.1042/BST20160284
7. Yanez-Mo M, Barreiro O, Gordon-Alonso M, Sala-Valdes M, Sanchez-Madrid F. Tetraspanin-enriched microdomains: a functional unit in cell plasma membranes. Trends Cell Biol (2009) 19:434–46. doi:10.1016/j.tcb.2009.06.004
8. Huang S, Yuan S, Dong M, Su J, Yu C, Shen Y, et al. The phylogenetic analysis of tetraspanins projects the evolution of cell-cell interactions from unicellular to multicellular organisms. Genomics (2005) 86:674–84. doi:10.1016/j.ygeno.2005.08.004
9. Pérez-Martínez CA, Maravillas-Montero JL, Meza-Herrera I, Vences-Catalán F, Zlotnik A, Santos-Argumedo L. Tspan33 is expressed in transitional and memory B cells, but is not responsible for high ADAM10 expression. Scand J Immunol (2017) 86:23–30. doi:10.1111/sji.12559
10. Levy S, Shoham T. The tetraspanin web modulates immune-signalling complexes. Nat Rev Immunol (2005) 5:136–48. doi:10.1038/nri1548
11. Zuidscherwoude M, de Winde CM, Cambi A, van Spriel AB. Microdomains in the membrane landscape shape antigen-presenting cell function. J Leukoc Biol (2014) 95:251–63. doi:10.1189/jlb.0813440
12. Zuidscherwoude M, Göttfert F, Dunlock VM, Figdor CG, van den Bogaart G, van Spriel AB. The tetraspanin web revisited by super-resolution microscopy. Sci Rep (2015) 5:12201. doi:10.1038/srep12201
13. Tarrant JM, Robb L, van Spriel AB, Wright MD. Tetraspanins: molecular organisers of the leukocyte surface. Trends Immunol (2003) 24:610–7. doi:10.1016/j.it.2003.09.011
14. Hemler ME. Tetraspanin functions and associated microdomains. Nat Rev Mol Cell Biol (2005) 6:801–11. doi:10.1038/nrm1736
15. Lammerding J, Kazarov AR, Huang H, Lee RT, Hemler ME. Tetraspanin CD151 regulates alpha6beta1 integrin adhesion strengthening. Proc Natl Acad Sci U S A (2003) 100:7616–21. doi:10.1073/pnas.1337546100
16. Kropshofer H, Spindeldreher S, Röhn TA, Platania N, Grygar C, Daniel N, et al. Tetraspan microdomains distinct from lipid rafts enrich select peptide-MHC class II complexes. Nat Immunol (2002) 3:61–8. doi:10.1038/ni750
17. Odintsova E, Voortman J, Gilbert E, Berditchevski F. Tetraspanin CD82 regulates compartmentalisation and ligand-induced dimerization of EGFR. J Cell Sci (2003) 116:4557–66. doi:10.1242/jcs.00793
18. Chu PG, Arber D. A. CD79: a review. Appl Immunohistochem Mol Morphol (2001) 9:97–106. doi:10.1097/00129039-200106000-00001
19. Burger JA, Wiestner A. Targeting B cell receptor signalling in cancer: preclinical and clinical advances. Nat Rev Cancer (2018) 18:148–67. doi:10.1038/nrc.2017.121
20. Wright MD, Moseley GW, van Spriel AB. Tetraspanin microdomains in immune cell signalling and malignant disease. Tissue Antigens (2004) 64:533–42. doi:10.1111/j.1399-0039.2004.00321.x
21. Miyazaki T, Muller U, Campbell KS. Normal development but differentially altered proliferative responses of lymphocytes in mice lacking CD81. EMBO J (1997) 16:4217–25. doi:10.1093/emboj/16.14.4217
22. Nichols TC, Guthridge JM, Karp DR, Molina H, Fletcher DR, Holers VM. γ-glutamyl transpeptidase, an ecto-enzyme regulator of intracellular redox potential, is a component of TM4 signal transduction complexes. Eur J Immunol (1998) 28:4123–9. doi:10.1002/(SICI)1521-4141(199812)28:12<4123::AID-IMMU4123>3.0.CO;2-G
23. Shaw AR, Domanska A, Mak A, Gilchrist A, Dobler K, Visser L, et al. Ectopic expression of human and feline CD9 in a human B cell line confers beta 1 integrin-dependent motility on fibronectin and laminin substrates and enhanced tyrosine phosphorylation. J Biol Chem (1995) 270:24092–9. doi:10.1074/jbc.270.41.24092
24. Yauch RL, Hemler ME. Specific interactions among transmembrane 4 superfamily (TM4SF) proteins and phosphoinositide 4-kinase. Biochem J (2000) 351:629–37. doi:10.1042/bj3510629
25. VanCompernolle SE, Levy S, Todd SC. Anti-CD81 activates LFA-1 on T cells and promotes T cell-B cell collaboration. Eur J Immunol (2001) 31:823–31. doi:10.1002/1521-4141(200103)31:3<823::AID-IMMU823>3.0.CO;2-D
26. Imai T, Yoshie O. C33 antigen and M38 antigen recognized by monoclonal antibodies inhibitory to syncytium formation by human T cell leukemia virus type 1 are both members of the transmembrane 4 superfamily and associate with each other and with CD4 or CD8 in T cells. J Immunol (1993) 151:6470–81.
27. van Spriel AB, Puls KL, Sofi M, Pouniotis D, Hochrein H, Orinska Z, et al. A regulatory role for CD37 in T cell proliferation. J Immunol (2004) 172:2953–61. doi:10.4049/jimmunol.172.5.2953
28. Escola JM, Kleijmeer MJ, Stoorvogel W, Griffith JM, Yoshie O, Geuze HJ. Selective enrichment of tetraspan proteins on the internal vesicles of multivesicular endosomes and on exosomes secreted by human B-lymphocytes. J Biol Chem (1998) 273:20121–7. doi:10.1074/jbc.273.32.20121
29. Hemler ME. Tetraspanin proteins promote multiple cancer stages. Nat Rev Cancer (2014) 14:49–60. doi:10.1038/nrc3640
30. Liang P, Miao M, Liu Z, Wang H, Jiang W, Ma S, et al. CD9 expression indicates a poor outcome in acute lymphoblastic leukemia. Cancer Biomark (2018) 21:781–6. doi:10.3233/CBM-170422
31. Muzzafar T, Medeiros LJ, Wang SA, Brahmandam A, Thomas DA, Jorgensen JL. Aberrant underexpression of CD81 in precursor B-cell acute lymphoblastic leukemia: utility in detection of minimal residual disease by flow cytometry. Am J Clin Pathol (2009) 132:692–8. doi:10.1309/AJCP02RPVOKTNWEC
32. Ji H, Chen L, Dai Y, Sun X, Li X, Wang Q, et al. Aberrant expression of CD133 and CD82 in patients with pediatric acute lymphoblastic leukemia and the clinical significance. Oncol Lett (2017) 14:5811–8. doi:10.3892/ol.2017.6981
33. Robak T, Robak P. Anti-CD37 antibodies for chronic lymphocytic leukemia. Expert Opin Biol Ther (2014) 14:651–61. doi:10.1517/14712598.2014.890182
34. Won WJ, Kearney JF. CD9 is a unique marker for marginal zone B cells, B1 cells, and plasma cells in mice. J Immunol (2002) 168:5605–11. doi:10.4049/jimmunol.168.11.5605
35. Yoon SO, Zhang X, Lee IY, Spencer N, Vo P, Choi YS. CD9 is a novel marker for plasma cell precursors in human germinal centers. Biochem Biophys Res Commun (2013) 431:41–6. doi:10.1016/j.bbrc.2012.12.102
36. Sun J, Wang J, Pefanis E, Chao J, Rothschild G, Tachibana I, et al. Transcriptomics identify CD9 as a marker of murine IL-10-competent regulatory B cells. Cell Rep (2015) 13:1110–7. doi:10.1016/j.celrep.2015.09.070
37. Horváth G, Serru V, Clay D, Billard M, Boucheix C, Rubinstein E. CD19 is linked to the integrin-associated tetraspans CD9, CD81, and CD82. J Biol Chem (1998) 273:30537–43. doi:10.1074/jbc.273.46.30537
38. Yoon SO, Lee IY, Zhang X, Zapata MC, Choi YS. CD9 may contribute to the survival of human germinal center B cells by facilitating the interaction with follicular dendritic cells. FEBS Open Bio (2014) 4:370–6. doi:10.1016/j.fob.2014.04.001
39. Braza F, Chesne J, Durand M, Dirou S, Brosseau C, Mahay G, et al. A regulatory CD9(+) B-cell subset inhibits HDM-induced allergic airway inflammation. Allergy (2015) 70:1421–31. doi:10.1111/all.12697
40. Böker KO, Lemus-Diaz N, Rinaldi Ferreira R, Schiller L, Schneider S, Gruber J. The impact of the CD9 tetraspanin on lentivirus infectivity and exosome secretion. Mol Ther (2018) 26:634–47. doi:10.1016/j.ymthe.2017.11.008
41. Maecker HT, Do MS, Levy S. CD81 on B cells promotes interleukin 4 secretion and antibody production during T helper type 2 immune responses. Proc Natl Acad Sci U S A (1998) 95:2458–62. doi:10.1073/pnas.95.5.2458
42. van Zelm MC, Smet J, Adams B, Mascart F, Schandené L, Janssen F, et al. CD81 gene defect in humans disrupts CD19 complex formation and leads to antibody deficiency. J Clin Invest (2010) 120:1265–74. doi:10.1172/JCI39748
43. Levy S. Function of the tetraspanin molecule CD81 in B and T cells. Immunol Res (2014) 58:179–85. doi:10.1007/s12026-014-8490-7
44. Feneant L, Levy S, Cocquerel L. CD81 and hepatitis C virus (HCV) infection. Viruses (2014) 6:535–72. doi:10.3390/v6020535
45. Mansson R, Lagergren A, Hansson F, Smith E, Sigvardsson M. The CD53 and CEACAM-1 genes are genetic targets for early B cell factor. Eur J Immunol (2007) 37:1365–76. doi:10.1002/eji.200636642
46. Zuidscherwoude M, Dunlock VE, van den Bogaart G, van Deventer SJ, van der Schaaf A, van Oostrum J, et al. Tetraspanin microdomains control localized protein kinase C signaling in B cells. Sci Signal (2017) 10:eaag2755. doi:10.1126/scisignal.aag2755
47. Rasmussen AM, Blomhoff HK, Stokke T, Horejsi V, Smeland EB. Cross-linking of CD53 promotes activation of resting human B lymphocytes. J Immunol (1994) 153:4997–5007.
48. Petersen SH, Odintsova E, Haigh TA, Rickinson AB, Taylor GS, Berditchevski F. The role of tetraspanin CD63 in antigen presentation via MHC class II. Eur J Immunol (2011) 41:2556–61. doi:10.1002/eji.201141438
49. Verweij FJ, van Eijndhoven MA, Hopmans ES, Vendrig T, Wurdinger T, Cahir-McFarland E, et al. LMP1 association with CD63 in endosomes and secretion via exosomes limits constitutive NF-kappaB activation. EMBO J (2011) 30:2115–29. doi:10.1038/emboj.2011.123
50. Yoshida N, Kitayama D, Arima M, Sakamoto A, Inamine A, Watanabe-Takano H, et al. CXCR4 expression on activated B cells is downregulated by CD63 and IL-21. J Immunol (2011) 186:2800–8. doi:10.4049/jimmunol.1003401
51. van Spriel AB, de Keijzer S, van der Schaaf A, Gartlan KH, Sofi M, Light A, et al. The tetraspanin CD37 orchestrates the alpha(4)beta(1) integrin-Akt signaling axis and supports long-lived plasma cell survival. Sci Signal (2012) 5:ra82. doi:10.1126/scisignal.2003113
52. Lapalombella R, Yeh YY, Wang L, Ramanunni A, Rafiq S, Jha S, et al. Tetraspanin CD37 directly mediates transduction of survival and apoptotic signals. Cancer Cell (2012) 21:694–708. doi:10.1016/j.ccr.2012.03.040
53. Rops AL, Figdor CG, van der Schaaf A, Tamboer WP, Bakker MA, Berden JH, et al. The tetraspanin CD37 protects against glomerular IgA deposition and renal pathology. Am J Pathol (2010) 176:2188–97. doi:10.2353/ajpath.2010.090770
54. de Winde CM, Veenbergen S, Young KH, Xu-Monette ZY, Wang XX, Xia Y, et al. Tetraspanin CD37 protects against the development of B cell lymphoma. J Clin Invest (2016) 126:653–66. doi:10.1172/JCI81041
55. Lagaudrière-Gesbert C, Lebel-Binay S, Wiertz E, Ploegh HL, Fradelizi D, Conjeaud H. The tetraspanin protein CD82 associates with both free HLA class I heavy chain and heterodimeric beta 2-microglobulin complexes. J Immunol (1997) 158:2790–7.
56. Chen ZH, Zhu Y, Ren Y, Tong Y, Hua X, Zhu F, et al. Hepatitis C virus protects human B lymphocytes from fas-mediated apoptosis via E2-CD81 engagement. PLoS One (2011) 6:e18933. doi:10.1371/journal.pone.0018933
57. Rosa D, Saletti G, De Gregorio E, Zorat F, Comar C, D’Oro U, et al. Activation of naive B lymphocytes via CD81, a pathogenetic mechanism for hepatitis C virus-associated B lymphocyte disorders. Proc Natl Acad Sci U S A (2005) 102:18544–9. doi:10.1073/pnas.0509402102
58. Rosa D, Saletti G, Nuti S, Galli-Stampino L, Galli G, Pozzato G, et al. BCR-independent activation of naive B cell via CD81: possible pathogenic mechanism of HCV associated B-lymphocyte disorders. J Hepatol (2004) 40:23–23. doi:10.1016/S0168-8278(04)90061-X
59. Machida K, Cheng KTH, Pavio N, Sung VMH, Lai MMC. Hepatitis C virus E2-CD81 interaction induces hypermutation of the immunoglobulin gene in B cells. J Virol (2005) 79:8079–89. doi:10.1128/JVI.79.13.8079-8089.2005
60. Cariappa A, Shoham T, Liu H, Levy S, Boucheix C, Pillai S. The CD9 tetraspanin is not required for the development of peripheral B cells or for humoral immunity. J Immunol (2005) 175:2925–30. doi:10.4049/jimmunol.175.5.2925
61. Yunta M, Lazo PA. Apoptosis protection and survival signal by the CD53 tetraspanin antigen. Oncogene (2003) 22:1219–24. doi:10.1038/sj.onc.1206183
62. Knobeloch KP, Wright MD, Ochsenbein AF, Liesenfeld O, Löhler J, Zinkernagel RM, et al. Targeted inactivation of the tetraspanin CD37 impairs T-cell-dependent B-cell response under suboptimal costimulatory conditions. Mol Cell Biol (2000) 20:5363–9. doi:10.1128/MCB.20.15.5363-5369.2000
63. van Spriel AB, Sofi M, Gartlan KH, van der Schaaf A, Verschueren I, Torensma R, et al. The Tetraspanin protein CD37 regulates IgA responses and anti-fungal immunity. PLoS Pathog (2009) 5:e1000338. doi:10.1371/journal.ppat.1000338
64. Castro R, Abós B, González L, Aquilino C, Pignatelli J, Tafalla C. Molecular characterization of CD9 and CD63, two tetraspanin family members expressed in trout B lymphocytes. Dev Comp Immunol (2015) 51:116–25. doi:10.1016/j.dci.2015.03.002
65. Bartosch B, Vitelli A, Granier C, Goujon C, Dubuisson J, Pascale S, et al. Cell entry of hepatitis C virus requires a set of co-receptors that include the CD81 tetraspanin and the SR-B1 scavenger receptor. J Biol Chem (2003) 278:41624–30. doi:10.1074/jbc.M305289200
66. Zhang YY, Zhang BH, Ishii K, Liang TJ. Novel function of CD81 in controlling hepatitis C virus replication. J Virol (2010) 84:3396–407. doi:10.1128/JVI.02391-09
67. Ito M, Masumi A, Mochida K, Kukihara H, Moriishi K, Matsuura Y, et al. Peripheral B cells may serve as a reservoir for persistent hepatitis C virus infection. J Innate Immun (2010) 2:607–17. doi:10.1159/000317690
68. Feigelson SW, Grabovsky V, Shamri R, Levy S, Alon R. The CD81 tetraspanin facilitates instantaneous leukocyte VLA-4 adhesion strengthening to vascular cell adhesion molecule 1 (VCAM-1) under shear flow. J Biol Chem (2003) 278:51203–12. doi:10.1074/jbc.M303601200
69. Hammad H, Vanderkerken M, Pouliot P, Deswarte K, Toussaint W, Vergote K, et al. Transitional B cells commit to marginal zone B cell fate by Taok3-mediated surface expression of ADAM10. Nat Immunol (2017) 18:313–20. doi:10.1038/ni.3657
70. Cooley LF, Martin RK, Zellner HB, Irani AM, Uram-Tuculescu C, El Shikh ME, et al. Increased B cell ADAM10 in allergic patients and Th2 prone mice. PLoS One (2015) 10:e0124331. doi:10.1371/journal.pone.0124331
71. Hoffmann FS, Kuhn PH, Laurent SA, Hauck SM, Berer K, Wendlinger SA, et al. The immunoregulator soluble TACI is released by ADAM10 and reflects B cell activation in autoimmunity. J Immunol (2015) 194:542–52. doi:10.4049/jimmunol.1402070
72. Mathews JA, Gibb DR, Chen B-H, Scherle P, Conrad DH. CD23 Sheddase A disintegrin and metalloproteinase 10 (ADAM10) is also required for CD23 sorting into B cell-derived exosomes. J Biol Chem (2010) 285:37531–41. doi:10.1074/jbc.M110.141556
73. Del Nagro CJ, Otero DC, Anzelon AN, Omori SA, Kolla RV, Rickert RC. CD19 function in central and peripheral B-cell development. Immunol Res (2005) 31:119–31. doi:10.1385/IR:31:2:119
74. Carter RH, Wang Y, Brooks S. Role of CD19 signal transduction in B cell biology. Immunol Res (2002) 26:45–54. doi:10.1385/IR:26:1-3:045
75. Aiba Y, Kameyama M, Yamazaki T, Tedder TF, Kurosaki T. Regulation of B-cell development by BCAP and CD19 through their binding to phosphoinositide 3-kinase. Blood (2008) 111:1497–503. doi:10.1182/blood-2007-08-109769
76. Poe JC, Hasegawa M, Tedder TF. CD19, CD21, and CD22: multifaceted response regulators of B lymphocyte signal transduction. Int Rev Immunol (2001) 20:739–62. doi:10.3109/08830180109045588
77. Fujimoto M, Poe JC, Hasegawa M, Tedder TF. CD19 regulates intrinsic B lymphocyte signal transduction and activation through a novel mechanism of processive amplification. Immunol Res (2000) 22:281–98. doi:10.1385/IR:22:2-3:281
78. Liu S, Kandeva T, Tchervenkov J. CD1d-mediated interaction between activated T cells and B cells is essential to B-cell proliferation and anti-alpha-Gal antibody production. Transplant Proc (2009) 41:398–402. doi:10.1016/j.transproceed.2008.10.054
79. Barral P, Eckl-Dorna J, Harwood NE, De Santo C, Salio M, Illarionov P, et al. B cell receptor-mediated uptake of CD1d-restricted antigen augments antibody responses by recruiting invariant NKT cell help in vivo. Proc Natl Acad Sci U S A (2008) 105:8345–50. doi:10.1073/pnas.0802968105
80. Belperron AA, Dailey CM, Bockenstedt LK. Infection-induced marginal zone B cell production of Borrelia hermsii-specific antibody is impaired in the absence of CD1d. J Immunol (2005) 174:5681–6. doi:10.4049/jimmunol.174.9.5681
81. Mizoguchi A, Mizoguchi E, Takedatsu H, Blumberg RS, Bhan AK. Chronic intestinal inflammatory condition generates IL-10-producing regulatory B cell subset characterized by CD1d upregulation. Immunity (2002) 16:219–30. doi:10.1016/S1074-7613(02)00274-1
82. Punnonen J, de Vries JE. Characterization of a novel CD2+ human thymic B cell subset. J Immunol (1993) 151:100–10.
83. Li M, Yang J, Zhang H, Shao JF, Su N, Liu SP. Enhancement of B cell responses by the interaction of CD2 with LFA-3. J Tongji Med Univ (1992) 12:71–4. doi:10.1007/BF02887783
84. Stohl W, Crow MK. Inhibition by anti-CD2 monoclonal antibodies of anti-CD3-induced T cell-dependent B cell activation. Cell Immunol (1990) 130:257–70. doi:10.1016/0008-8749(90)90269-W
85. Won W-J, Bachmann MF, Kearney JF. CD36 is differentially expressed on B cell subsets during development and in responses to antigen. J Immunol (2008) 180:230–7. doi:10.4049/jimmunol.180.1.230
86. Konig H, Pfisterer P, Corcoran LM, Wirth T. Identification of CD36 as the first gene dependent on the B-cell differentiation factor Oct-2. Genes Dev (1995) 9:1598–607. doi:10.1101/gad.9.13.1598
87. Gore Y, Starlets D, Maharshak N, Becker-Herman S, Kaneyuki U, Leng L, et al. Macrophage migration inhibitory factor induces B cell survival by activation of a CD74-CD44 receptor complex. J Biol Chem (2008) 283:2784–92. doi:10.1074/jbc.M703265200
88. Wyant TL, Fisher MT, McKallip RJ, Nagarkatti PS, Nagarkatti M, Conrad DH. Mouse B cell activation is inhibited by CD44 cross-linking. Immunol Invest (2005) 34:399–416. doi:10.1080/08820130500265406
89. Rosel M, Foger N, Zoller M. Involvement of CD44 exon v10 in B-cell activation. Tissue Antigens (1998) 52:99–113. doi:10.1111/j.1399-0039.1998.tb02273.x
90. Rafi A, Nagarkatti M, Nagarkatti PS. Hyaluronate-CD44 interactions can induce murine B-cell activation. Blood (1997) 89:2901–8.
91. Arredouani MS, Bhasin MK, Sage DR, Dunn LK, Gill MB, Agnani D, et al. Analysis of host gene expression changes reveals distinct roles for the cytoplasmic domain of the Epstein-Barr virus receptor/CD21 in B-cell maturation, activation, and initiation of virus infection. J Virol (2014) 88:5559–77. doi:10.1128/JVI.03099-13
92. Kim D, Niewiesk S. Synergistic induction of interferon alpha through TLR-3 and TLR-9 agonists identifies CD21 as interferon alpha receptor for the B cell response. PLoS Pathog (2013) 9:e1003233. doi:10.1371/journal.ppat.1003233
93. Farjam M, Ebrahimpour A, Fakhraei B. CD21 positive B cell: a novel target for treatment of multiple sclerosis. Med Hypotheses (2013) 80:556–7. doi:10.1016/j.mehy.2013.01.016
94. Busse C, Feederle R, Schnölzer M, Behrends U, Mautner J, Delecluse HJ. Epstein-Barr viruses that express a CD21 antibody provide evidence that gp350’s functions extend beyond B-cell surface binding. J Virol (2010) 84:1139–47. doi:10.1128/JVI.01953-09
95. Mitrevski M, Marrapodi R, Camponeschi A, Lazzeri C, Todi L, Quinti I, et al. Intravenous immunoglobulin replacement therapy in common variable immunodeficiency induces B cell depletion through differentiation into apoptosis-prone CD21(low) B cells. Immunol Res (2014) 60:330–8. doi:10.1007/s12026-014-8599-8
96. Barrington RA, Schneider TJ, Pitcher LA, Mempel TR, Ma M, Barteneva NS, et al. Uncoupling CD21 and CD19 of the B-cell coreceptor. Proc Natl Acad Sci U S A (2009) 106:14490–5. doi:10.1073/pnas.0903477106
97. Twohig JP, Pappworth IY, Sivasankar B, Kulik L, Bull M, Holers VM, et al. Defective B cell ontogeny and humoral immune response in mice prematurely expressing human complement receptor 2 (CR2, CD21) is similar to that seen in aging wild type mice. Mol Immunol (2009) 46:2002–13. doi:10.1016/j.molimm.2009.03.007
98. Pappworth IY, Kulik L, Haluszczak C, Reuter JW, Holers VM, Marchbank KJ. Increased B cell deletion and significantly reduced auto-antibody titre due to premature expression of human complement receptor 2 (CR2, CD21). Mol Immunol (2009) 46:1042–9. doi:10.1016/j.molimm.2008.08.273
99. Barrington RA, Zhang M, Zhong X, Jonsson H, Holodick N, Cherukuri A, et al. CD21/CD19 coreceptor signaling promotes B cell survival during primary immune responses. J Immunol (2005) 175:2859–67. doi:10.4049/jimmunol.175.5.2859
100. Cherukuri A, Shoham T, Sohn HW, Levy S, Brooks S, Carter R, et al. The tetraspanin CD81 is necessary for partitioning of coligated CD19/CD21-B cell antigen receptor complexes into signaling-active lipid rafts. J Immunol (2004) 172:370–80. doi:10.4049/jimmunol.172.1.370
101. Mongini PKA, Jackson AE, Tolani S, Fattah RJ, Inman JK. Role of complement-binding CD21/CD19/CD81 in enhancing human B cell protection from Fas-mediated apoptosis. J Immunol (2003) 171:5244–54. doi:10.4049/jimmunol.171.10.5244
102. Gordin M, Tesio M, Cohen S, Gore Y, Lantner F, Leng L, et al. c-Met and its ligand hepatocyte growth factor/scatter factor regulate mature B cell survival in a pathway induced by CD74. J Immunol (2010) 185:2020–31. doi:10.4049/jimmunol.0902566
103. van der Voort R, Taher TE, Keehnen RM, Smit L, Groenink M, Pals ST. Paracrine regulation of germinal center B cell adhesion through the c-met-hepatocyte growth factor/scatter factor pathway. J Exp Med (1997) 185:2121–31. doi:10.1084/jem.185.12.2121
104. Salazar-Camarena DC, Ortiz-Lazareno PC, Cruz A, Oregon-Romero E, Machado-Contreras JR, Muñoz-Valle JF, et al. Association of BAFF, APRIL serum levels, BAFF-R, TACI and BCMA expression on peripheral B-cell subsets with clinical manifestations in systemic lupus erythematosus. Lupus (2016) 25:582–92. doi:10.1177/0961203315608254
105. Koarada S, Tashiro S, Suematsu R, Inoue H, Ohta A, Tada Y. [BCMA and autoantibody-producing RP105 B cells; possible new targets of B cell therapy in systemic lupus erythematosus]. Nihon Rinsho Meneki Gakkai Kaishi (2012) 35:38–45. doi:10.2177/jsci.35.38
106. Hatzoglou A, Roussel J, Bourgeade MF, Rogier E, Madry C, Inoue J, et al. TNF receptor family member BCMA (B cell maturation) associates with TNF receptor-associated factor (TRAF) 1, TRAF2, and TRAF3 and activates NF-kappa B, elk-1, c-Jun N-terminal kinase, and p38 mitogen-activated protein kinase. J Immunol (2000) 165:1322–30. doi:10.4049/jimmunol.165.3.1322
107. Gross JA, Johnston J, Mudri S, Enselman R, Dillon SR, Madden K, et al. TACI and BCMA are receptors for a TNF homologue implicated in B-cell autoimmune disease. Nature (2000) 404:995–9. doi:10.1038/35010115
108. Ji C, Liu Y, Pamulapati C, Bohini S, Fertig G, Schraeml M, et al. Prevention of hepatitis C virus infection and spread in human liver chimeric mice by an anti-CD81 monoclonal antibody. Hepatology (2015) 61:1136–44. doi:10.1002/hep.27603
109. Koarada S, Tada Y, Sohma Y, Haruta Y, Suematsu R, Mitamura M, et al. Autoantibody-producing RP105(-) B cells, from patients with systemic lupus erythematosus, showed more preferential expression of BCMA compared with BAFF-R than normal subjects. Rheumatology (Oxford) (2010) 49:662–70. doi:10.1093/rheumatology/kep437
Keywords: tetraspanin, B cell, partner, immune regulation, therapy strategy
Citation: Zou F, Wang X, Han X, Rothschild G, Zheng SG, Basu U and Sun J (2018) Expression and Function of Tetraspanins and Their Interacting Partners in B Cells. Front. Immunol. 9:1606. doi: 10.3389/fimmu.2018.01606
Received: 30 March 2018; Accepted: 27 June 2018;
Published: 18 July 2018
Edited by:
Annemiek van Spriel, Radboud University Nijmegen Medical Centre, NetherlandsReviewed by:
Roberta Pelanda, University of Colorado, United StatesShoshana Levy, Stanford University, United States
Copyright: © 2018 Zou, Wang, Han, Rothschild, Zheng, Basu and Sun. This is an open-access article distributed under the terms of the Creative Commons Attribution License (CC BY). The use, distribution or reproduction in other forums is permitted, provided the original author(s) and the copyright owner(s) are credited and that the original publication in this journal is cited, in accordance with accepted academic practice. No use, distribution or reproduction is permitted which does not comply with these terms.
*Correspondence: Uttiya Basu, ub2121@cumc.columbia.edu;
Jianbo Sun, sunjb3@mail.sysu.edu.cn
†These authors have contributed equally to this work.