- 1Department of Integrative Biology, University of Guelph, Guelph, ON, Canada
- 2Bird Studies Canada, Delta, BC, Canada
- 3Environment and Climate Change Canada, Saskatoon, SK, Canada
- 4Department of Ecology and Evolutionary Biology, Museum of Vertebrates, Cornell University, Ithaca, NY, United States
- 5Département de Biologie, Université de Sherbrooke, Sherbrooke, QC, Canada
- 6Ecosystem Science and Management Program, University of Northern British Columbia, Prince George, BC, Canada
- 7Alaska Songbird Institute, Fairbanks, AK, United States
- 8Oklahoma Biological Survey, University of Oklahoma, Norman, OK, United States
- 9Department of Biology, Dalhousie University, Halifax, NS, Canada
- 10Behavioral and Molecular Ecology Group, Department of Biological Sciences, University of Wisconsin-Milwaukee, Milwaukee, WI, United States
- 11Beaverhill Bird Observatory, Edmonton, AB, Canada
- 12Ontario Ministry of Natural Resources, Peterborough, ON, Canada
- 13Department of Biological Sciences, Simon Fraser University, Burnaby, BC, Canada
- 14Department of Environmental Studies, University of North Carolina Asheville, Asheville, NC, United States
- 15Department of Biology, Acadia University, Wolfville, NS, Canada
- 16Biology Department, Appalachian State University, Boone, NC, United States
- 17Department of Ecology and Evolutionary Biology, Tulane University, New Orleans, LA, United States
- 18Department of Ecology, Evolution and Organismal Biology, Iowa State University, Ames, IA, United States
During migration, animals may experience high rates of mortality, but costs of migration could also be manifested through non-lethal carry-over effects that influence individual success in subsequent periods of the annual cycle. Using tracking data collected from light-level geolocators, we estimated total spring migration distance (from the last wintering sites to breeding sites) of tree swallows (Tachycineta bicolor) within three major North American flyways. Using path analysis, we then assessed direct and indirect effects of spring migration distance on reproductive performance of individuals of both sexes. When these data were standardized by flyway, females fledged 1.3 fewer young for every 1,017 km they traveled, whereas there was no effect of migration distance on reproductive success in males. In comparison, when these data were standardized across all individuals and not by flyway, longer migrations were associated with 0.74 more young fledged for every 1,017 km traveled by females and 0.26 more young fledged for every 1,186 km migrated by males. Our results suggest that migration distance carries over to negatively influence female reproductive success within flyways but the overall positive effect of migration distance across flyways likely reflects broader life-history differences that occur among breeding populations across the tree swallow range.
Introduction
Migration is widespread throughout the animal kingdom (reviewed in Newton, 2008) and likely evolved as an adaptation to optimize resource use (Alerstam et al., 2003; Alerstam, 2011). However, traveling between locations, many of which are thousands of kilometers apart, is also considered to be costly (Wikelski et al., 2003). Such costs are primarily thought to be “direct” in the form of higher mortality when compared to non-migratory periods of the annual cycle (Lok et al., 2014), though the migratory period may not be the only period with the highest mortality rates (Leyrer et al., 2013; Rakhimberdiev et al., 2015a; Senner et al., 2019). Among those individuals that survive migration, the cost of traveling such long distances may also carry over to influence reproductive success the following season (Harrison et al., 2011). Determining the existence and strength of these carry-over effects and how they may vary within and among populations will contribute to our understanding of long-term population dynamics and how life-history trade-offs shape broad-scale migration patterns (Norris and Marra, 2007; Harrison et al., 2011; Betini et al., 2013).
Many species of birds migrate different distances, even within a single breeding population (Fraser et al., 2012; McKinnon et al., 2013; Knight et al., 2018; McKinnon and Love, 2018). Only a few avian studies have examined carry-over effects of migration distance on individual reproductive success following a breeding season, and most have focused on whether migration distance is related to timing of arrival at a breeding site (Hötker, 2002; Bregnballe et al., 2006; Gunnarsson et al., 2006; Alves et al., 2012; Briedis et al., 2019), the start of breeding (Lok et al., 2016; Kentie et al., 2017), and breeding productivity (Bearhop et al., 2005; Bregnballe et al., 2006; Lok et al., 2016; Kentie et al., 2017). For example, in great cormorants, Phalacrocorax carbo (Bregnballe et al., 2006), and pied avocets, Recurvirostra avosetta (Hötker, 2002), birds wintering farther south arrived later at breeding sites. For pied avocets, early arrival led to higher breeding success (Hötker, 2002), whereas this relationship was not observed in great cormorants (Bregnballe et al., 2006). More southerly wintering black-tailed godwits, Limosa limosa that crossed the Sahara, started breeding earlier than those wintering farther north that did not cross the Sahara, but there was little effect of migration distance on reproductive success (Kentie et al., 2017). Male Eurasian spoonbills, Platalea leucorodia, that migrated longer distances began breeding later and subsequently produced fewer and lower quality chicks, and recruited fewer young (Lok et al., 2016). Similarly, in a study on songbirds, European blackcaps, Sylvia atricapilla, wintering farther north, as estimated from stable isotopes, produced larger clutches and fledged more young compared to those wintering farther south (Bearhop et al., 2005). Collectively these studies suggest that non-lethal effects of migration distance on reproduction might depend on the species or ecological context, and strongly emphasize that further study is needed across a wider range of taxa and among multiple populations.
Recently, we described a migratory network based on year-round movements of tree swallows (Tachycineta bicolor) originating from 12 breeding populations across their range (Knight et al., 2018). In addition to linking breeding populations with stopover and wintering sites, we also identified three distinct migratory flyways (Knight et al., 2018). In the Western flyway, tree swallows breeding west of the Rockies migrated primarily to western Mexico, those in the Central flyway bred in central Canada or the U.S and either crossed the Gulf of Mexico to wintering sites in eastern Mexico, or wintered in Louisiana, Mississippi, or Florida (Central flyway). The Eastern flyway consisted of tree swallows that bred in eastern North America and primarily used wintering sites in Florida, the Caribbean Islands, or Cuba (Eastern flyway; Figure 1; Knight et al., 2018). In a subsequent study, the timing of arrival on the breeding grounds appeared to be most strongly influenced by both the latitude from which the birds departed and the latitude of the breeding site (Gow et al., 2019), suggesting that the distance an individual traveled could influence the timing of breeding. Whether and how spring migration distance could carry over to influence subsequent reproductive success is not known.
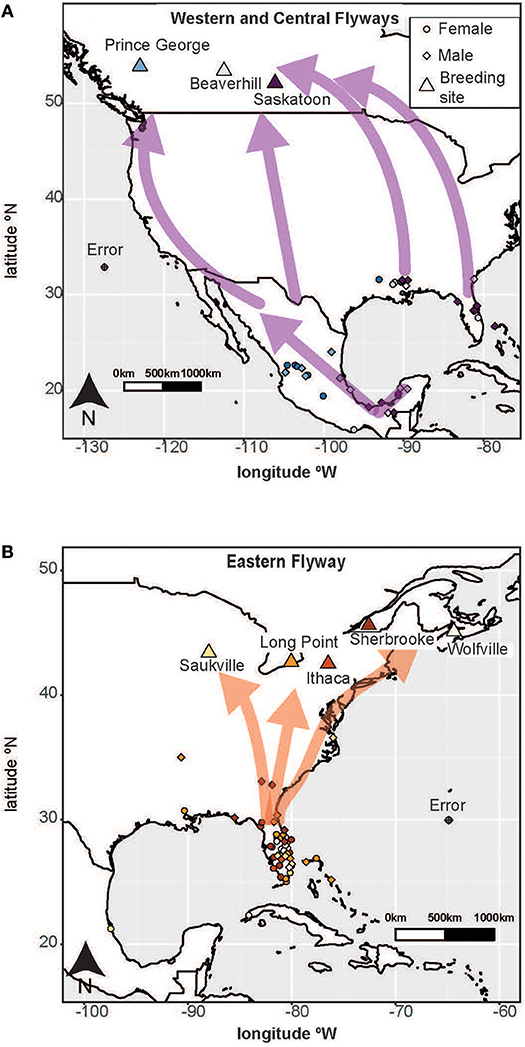
Figure 1. The breeding (triangles) and wintering (females: circles; males: diamonds) locations of tree swallows from populations in (A) Western and Central flyways (B) Eastern flyway. Each circle (female) or diamond (male) is an individual tree swallow on its primary wintering grounds and the colors indicate breeding origin. The arrows in both panels depict the general spring migration routes from wintering to breeding locations (based on Bradley et al., 2014; Knight et al., 2018). These routes were used to calculate migration distance. Map is drawn using the default latitude/longitude projection. The size of each individual circle or diamond represents the approximate error associated with geolocators in this study. Sample sizes are: N = 33 Eastern flyway females; N = 20 Eastern flyway males; N = 13 Central flyway females; and N = 23 Central flyway males.
There are several factors that influence reproductive performance (i.e., number of young fledged), and ultimately population dynamics (Cox et al., 2018). These include reproductive traits, such as clutch size (Dunn et al., 2000; Millet et al., 2015), timing of events such as arrival at breeding locations (e.g., Norris et al., 2004), or start of breeding (i.e., first egg dates; Hochachka, 1990; Verhulst and Nilsson, 2008; Millet et al., 2015). Understanding the relationships among these factors and how they directly or indirectly affect reproductive success could provide mechanisms through which spring migration distance and the duration of spring migration may influence productivity. Here, we evaluated (1) the potential for spring migration distance to affect reproductive performance among populations, (2) how this may vary between major flyways, and (3) whether migration distance had different effects on each sex. We used path analysis (Shipley, 2009) to first quantify the direct effects of spring migration distance on timing of arrival to breeding sites, first egg date, clutch size, and the number of young fledged, and then estimated possible indirect effects of spring migration distance on the number of young fledged via the other reproductive metrics. We evaluated these effects by sex controlling for flyway (identified in Knight et al., 2018), and then compared these results to analyses when data from all flyways were combined. From the direct and indirect effects estimates from the path models, we then generated predictions of the total effect of migration distance on the number of young fledged.
Materials and Methods
Study Sites and Data Collection
Between 2010 and 2014, we equipped 561 adult tree swallows with an archival light-level geolocator (hereafter referred to as “geolocators”) at the 12 breeding sites (Fairbanks, Alaska, 65.90°N, 147.70°W; Vancouver, British Columbia, 49.21°N, 123.18°W; Prince George, British Columbia, 53.85°N, 123.02°W; Beaverhill, Alberta, 53.40°N, 112.50°W, Saskatoon, Saskatchewan, 52.17°N, 106.10°W; Ames, Iowa, 42.11°N, 93.59°W; Saukville, Wisconsin, 43.40°N, 88.00°W; Boone, North Carolina, 36.21°N, 81.67°W; Long Point, Ontario, 42.62°N, 80.46°W; Ithaca, New York, 42.50°N, 76.50°W; Sherbrooke, Québec, 45.55°N, 72.60°W; Wolfville, Nova Scotia, 45.10°N, 64.39°W). Overall, we retrieved 161 geolocators, 133 of which were free from malfunctions. Each bird was tracked for a 1-year period. Of these 133, we obtained reproductive history from 105 individuals. Birds from Vancouver, Alaska, Iowa, and North Carolina were not included in this study, either because of small sample size or because individuals at these sites were outliers based on migration distances within their respective flyway. Eighty-nine individuals (46 females and 43 males) were therefore used in this study.
Geolocator Analysis and Definition of the Last Wintering Site
Light data from geolocators were analyzed using the BAStag package 0.1.3 (Wotherspoon et al., 2013) and FlightR package version 0.3.6 (Rakhimberdiev et al., 2015b) with R version 3.2.3 (R Core Development Team, 2016). The FlightR package works well for open area birds, and it uses a state-space hidden Markov model to estimate daily locations. For step-by-step details about geolocator deployment and analysis see Knight et al. (2018). Given the well-defined light transitions, geolocator error was minimal (46 ± 90 km in latitude and 52 ± 90 km in longitude; Gow et al., 2019). Geolocator error was calculated based on averaged location estimates from the breeding site (Gow et al., 2019). We followed the definitions in Gow et al. (2019) for determining wintering site, and identifying departure and arrival at sites. Briefly, locations were determined by calculating the mean location of all daily locations from a stationary period. We considered birds to have departed from the wintering site if they made a large (≥ 250 km) northward movement, lasting for at least 2 d, away from a stationary position. Given that tree swallows may use more than one wintering site (Knight et al., 2018), the last wintering site was defined as the last location that a tree swallow spent at least 28 d. Breeding arrival date was defined as the first day tree swallows had location estimates consistently matching those of the known breeding site.
Definitions of Reproductive Metrics and Flyways
Tree swallows breed in natural tree cavities or nest boxes throughout the southern half of Canada and the north/central United States; they generally have clutches of 4–7 eggs (Winkler et al., 2011). All tree swallows in our study were single-brooded, although some females attempted a second clutch if their first nest was depredated. Tree swallow clutch sizes increase with breeding latitude (Dunn et al., 2000). At each study site, nest boxes were checked every 1–7 days (with most sites checking nests every 1–3 days) to obtain the following breeding information from individuals in the year following geolocator deployment: first egg date (date on which the first egg of the first clutch of the season was laid), clutch size (number of eggs laid in the first clutch of the season), and number of young fledged (number of young estimated to have survived to fledging). All failed nests were counted as zero in our analyses. We determined breeding arrival date (date bird first arrived at the breeding site), and spring migration distance for each tree swallow carrying a geolocator. We calculated spring migration distance as the great circle distance between the last overwintering site and the breeding site (i.e., spring migration distance; Figure 1; Bradley et al., 2014). The arrows in Figure 1 show the general pathways used to calculate migration distance for each population. Most tree swallows migrated during the spring equinox making it difficult to estimate the true travel route. Thus, for consistency among individuals we calculated migration distance using several points along the migration pathways for tree swallows defined by Bradley et al. (2014; Figure 1). The connections between wintering and breeding sites are available in Knight et al. (2018).
We interpreted clutch size and the number of young fledged differently between sexes. For females, clutch size and the number of young fledged are direct measures of reproductive success. However, because there is rampant extra-pair paternity in tree swallows (e.g., 50–89%; Lifjeld et al., 1993; Barber et al., 1996; Kempenaers et al., 2001; Whittingham and Dunn, 2001; O'Brien and Dawson, 2007), the number of young fledged likely does not represent realized (i.e., genetic) reproductive success of males. Realized reproductive success would account for both a male's potential paternity lost within his nest and potential paternity gained via extra-pair fertilizations outside the social pair bond. Given that we did not have genetic paternity data from our sites, we could not include realized reproductive success of males in our study. Thus, for males, the number of young fledged within the social pair bond (the variable we measured and included in our study) more accurately reflects the quality of the social partner rather than true reproductive success in a given season. First egg dates and clutch size also mainly reflect the quality of the social partner rather than true reproductive parameters for males, meaning that the only reproductive variable that is solely influenced by males was breeding arrival date. Breeding arrival date may have subsequent carry-over effects that may influence the quality of the social mates that a male is able to acquire.
A network analysis by Knight et al. (2018) showed how breeding populations segmented into three migratory flyways: Western, Central, and Eastern. We chose to combine the Western and Central flyways because of the small sample of birds in the Western flyway (n = 11) and because these two flyways had similar means and standard deviations (s.d.) of migration distance (Western: 3,833 ± 795 km; Central: 4,217 ± 1,082 km; Table 1). We eliminated 4 populations from our analyses that had a small sample size (Vancouver, BC and Ames, IA) and/or represented extreme southern and northern regions of the tree swallows' range (Fairbanks, AK and Boone, SC). The populations we included from the Western and Central flyways (hereafter referred to as the Central flyway for simplicity) were Prince George, BC, Beaverhill, AB, and Saskatoon, SK (N = 36; female = 13; male = 23; Figure 1A), and those in the Eastern flyway were Saukville, WI, Long Point, ON, Ithaca, NY, Sherbrooke, QC, and Wolfville, NS (N = 53; female = 33; male = 20; Figure 1B).
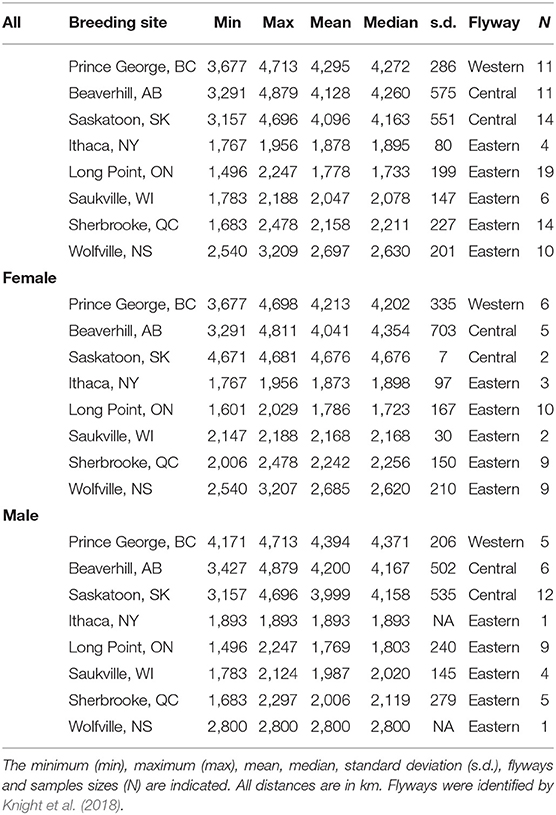
Table 1. Summary of migration distances among breeding sites across sexes and separated by females and males.
Path Analysis
Prior to executing the path analysis, we undertook two types of data standardizations to help separate the potential effects of breeding location on life-history variation from the effects on individuals migrating farther than other individuals within their flyway. First, we standardized each variable by flyway (sexes pooled) by subtracting the mean then dividing by the standard deviation (i.e., z-transformation). This allowed us to control for flyway effects. Second, we z-transformed these data across all individuals independent of flyway. If there was a positive effect of migration distance on first egg date, clutch size, or young fledged when the dataset was standardized across all individuals, then this may indicate the relationship was due to life-history factors rather than migration distance per se. In contrast, when standardizing by flyway, if there is a negative relationship between migration distance on first egg date, clutch size or young fledged, then this may suggest a potential carry-over effect of migration distance.
Following this standardization, we evaluated the direct and indirect effects of migration duration, migration distance, breeding arrival date, first egg date, and clutch size on the number of young fledged using a multi-level path modeling framework (Shipley, 2000, 2009). We included a random effect of “breeding site” to account for local-level effects across the 8 breeding populations. All mixed effects models were fitted with a Gaussian distribution, as the response variables best fit this distribution, using the nlme package (Pinheiro et al., 2018) in R 3.5.2 (R Core Development Team, 2018). We identified the most parsimonious path model based on Akaike's Information Criterion corrected for sample size (AICc; Shipley, 2000, 2009, 2013). We evaluated four different sets of path models, separated by sex and standardization method (i.e., standardized by flyway or standardized across individuals).
We structured the path models based on previous knowledge of tree swallow ecology. For each set of path models, we started by fitting a global model, which included direct effects of spring migration duration, spring migration distance, breeding arrival date, first egg date, and clutch size on the number of young fledged. We removed terms associated with uninformative estimates for young fledged first, followed by those with uninformative estimates for clutch size, first egg date, breeding arrival date, and migration distance. We determined the order of deletion using AICc to assess the terms with the least support in each submodel using maximum likelihood estimation (MuMIn package; Barton, 2016; see Figure S1 for path analysis sub-models). We removed terms from the path model if their deletion did not increase the AIC by at least two units (Table S1). Models were not averaged because top models were all nested within preceding models (Arnold, 2010).
From the path analysis, we calculated direct effects of one variable on another as well as indirect effects (Mitchell, 1993). Indirect effects were calculated by taking the product of all possible pathways (path coefficients) from one variable to another. Direct effects occur between variables and are generated by path coefficients (regression beta coefficients). Because we standardized the dataset prior to conducting the path analysis we did not need to standardize the path coefficients. The total effect was calculated as the sum of all indirect and direct effects from one variable to another.
We compared migration distances between sex and standardization type using a linear mixed effects model (LMM). We included breeding site as a random effect. We used post-hoc pairwise differences to compare migration distances between the sexes standardized by flyway or across individuals.
Predicted Effects of Migration Distance on Young Fledged
Because total effect values summarize all direct and indirect pathways between migration distance to the number of young fledged, we also produced a predictive model. This model involved first summarizing the effect of migration distance on the number of young fledged using the total effect values and standard deviations (s.d.) for each variable (Bart and Earnst, 1999). For example, the effect of migration distance on number of young fledged was expressed as: s.d. (migration distance) = s.d. (young fledged*total effect). We took the mean migration distance and used it to predict differences in the number of young fledged across different migration distances. We only developed predictive models for the sex and standardizations in which there was at least one effect of migration distance on a reproductive metric.
This study was carried out in accordance with the principles of Animal Utilization Protocols or Animal Care Protocols and was approved by each University of the primary researcher for each field site.
Results
Variation of Spring Migration Distance Between Flyways and Sexes
Tree swallows migrated on average 2,930 ± 1,110 km (range: 1,496–4,879 km). While males migrated farther and showed more variation in migration distances than females (males: 3,110 ± 1,187 km; females: 2,761 ± 1,017 km), there was no evidence the sexes arose from different distributions (LMM: β = −61.89 ± 82.44, t = −0.75, p = 0.45; Table 1). Females migrated significantly farther and had different distributions in their migration distances in the Central flyway than the Eastern flyway (LMM: β = 2,129 ± 261, t = 8.14, p < 0.001; Table 1), and a similar pattern was observed for males (LMM: β = 2,201 ± 161, t = 13.66, p < 0.001; Table 1). Males spent more time on average migrating than females but this difference was not significant (LMM: β = 5.58 ± 4.67, t = 1.19, p = 0.24; Table 2). In the Central flyway, females spent significantly more time on spring migration compared to the Eastern flyway (LMM: β = 15.58 ± 6.0, t = 2.60, p < 0.01; Table 2). Although males in the Central flyway spent more days migrating in the spring than in the Eastern flyway, this was not significantly different (LMM: β = 13.13 ± 7.24, t = 1.81, p = 0.14; Table 2).
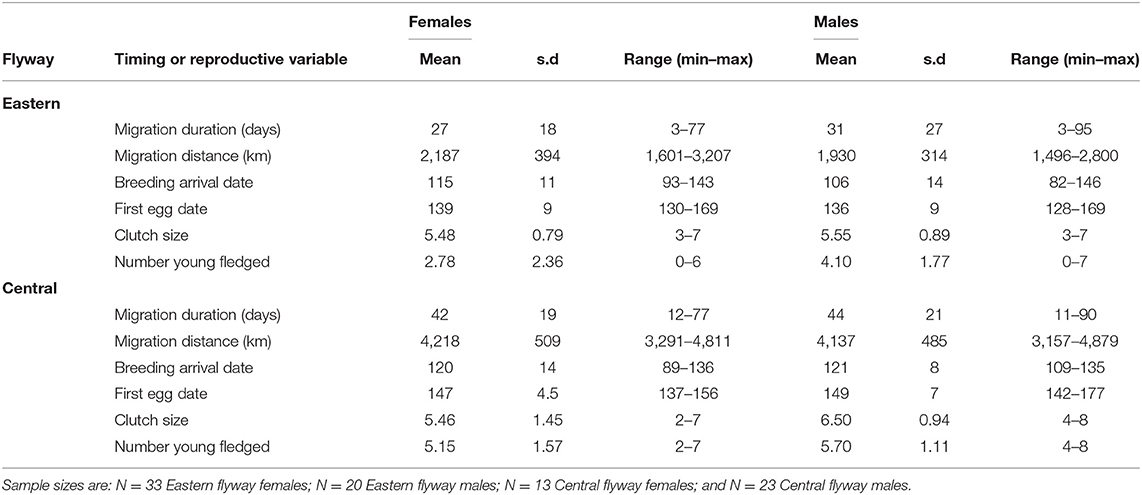
Table 2. Summary of the means, standard deviations (s.d.) and range (minimum and maximum) of migration distance and duration, timing (breeding arrival date, first egg date) events, and reproductive variables (clutch size and number of young fledged) for females and males within each flyway.
Effect of Migration Distance on Reproductive Performance: Variables Standardized by Flyway
For females, migration distance negatively affected the number of young fledged (Figure 2). Females migrating the shortest distances within their flyway fledged more young compared to those migrating the farthest distances, suggesting a direct carry-over effect of migration distance on young fledged. In contrast, for males there was no effect of migration distance on clutch size or young fledged, but males that spent longer migrating in the spring arrived later to the breeding site. Breeding arrival date positively influenced first egg dates in females, but males that arrived later had social mates that laid their first clutches earlier. For females, first egg dates did not influence clutch size. Earlier breeding males had social mates that produced larger clutches than later breeding males. For females, clutch size positively affected the number of young fledged, and males mated to females that laid large clutches also fledged more young. Overall, the total effect of migration distance on number of young fledged for females was −0.55 ± 0.33, resulting in a predicted 1.33 fewer young for every 1,017 km they migrated (Figure 3).
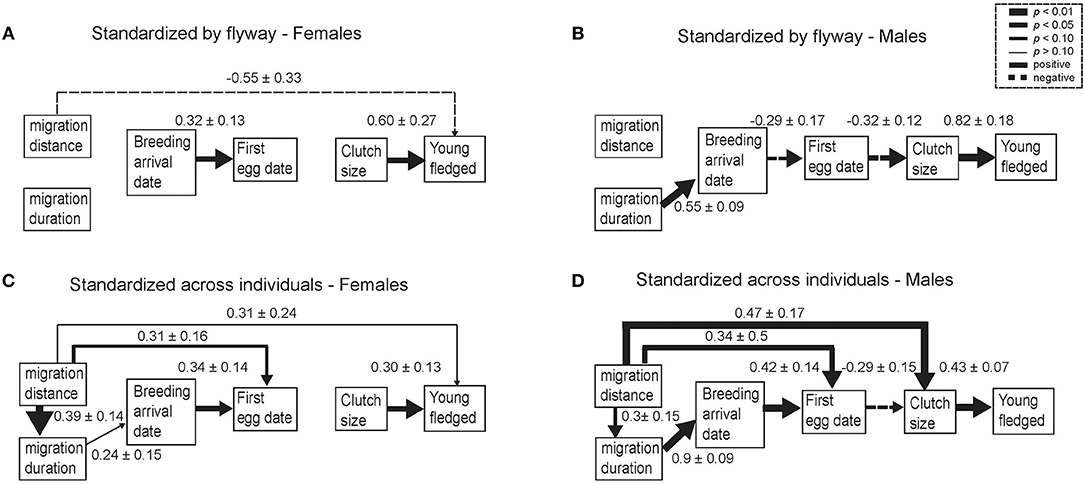
Figure 2. Path diagrams for the factors that influence the number of young fledged for female and male tree swallows using variables standardized by flyway (A,B) and standardized across individuals (C,D). Path models represent the most parsimonious models as determined using information theory. The thickness of each arrow is scaled to reflect the magnitude of the partial regression coefficients and the corresponding levels of significance (see legend on figure). Solid lines indicate positive relationships and dashed lines indicate negative relationships. All data were standardized prior to analysis and all partial regression coefficients are comparable across all relationships. Sample sizes are: N = 33 Eastern flyway females; N = 20 Eastern flyway males; N = 13 Central flyway females; and N = 23 Central flyway males.
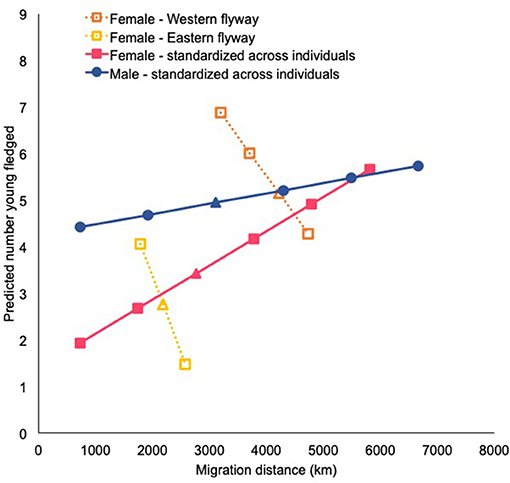
Figure 3. Predicted number of young fledged by tree swallows based on migration distance. Predicted relationships from the path model standardized by flyway for females are shown with open squares and dashed lines, with the flyways separated (western = orange; eastern = yellow). Relationships from the path model standardized across individuals for females is indicated by the solid pink squares and lines. Males from the path model standardized across individuals are represented by filled blue circles and solid lines. Relationships were based on the path analyses (Figure 2), and subsequent changes in young fledged (y-axis) are based on total effects of migration distance (x-axis) calculated from the path analyses. The triangle indicates the mean value of young fledged and migration distance in which each predicted relationship is based off.
Effect of Migration Distance on Reproductive Performance: Variables Standardized Across All Individuals
Migration distance in females directly and positively affected migration duration, first egg dates, and young fledged (Figure 2). Given the later first egg dates and higher number of young fledged in the Central flyway than the Eastern flyway (Table 2), results from the path analysis showed that females migrating the farthest distances (most birds in the Central flyway) fledged more young and also began breeding later than females migrating shorter distances (most birds in the Eastern flyway). For males, migration distance had a positive direct effect on migration duration, and the male's social mate's first egg date and clutch size. Furthermore, males traveling the farthest distances (typically in the Central flyway) began breeding later and their social mate had larger clutches than those migrating shorter distances (typically the Eastern flyway). But the negative relationship between first egg date and clutch size suggested that males with social mates that began breeding later had smaller clutches. Overall, the total effect of migration distance on young fledged for females was 0.31, which implied that females fledged 0.74 more young for every 1,017 km farther they migrated. For males, the total effect of migration distance on young fledged was 0.16, which implied that males fledged 0.26 more young for every 1,186 km farther they migrated (Figure 3).
Discussion
Collectively, previous research suggests that non-lethal effects of migration distance on reproduction might be species or context specific (Hötker, 2002; Bregnballe et al., 2006; Gunnarsson et al., 2006; Alves et al., 2012; Lok et al., 2016; Kentie et al., 2017), but if migration distance is energetically costly then individuals that migrate farther distances may experience carry-over effects into stationary periods of the annual cycle (Harrison et al., 2011 but see Conklin et al., 2017). Our results suggest that variation in migration distance within a flyway with individuals breeding at similar latitudes, had a negative effect on the number of young fledged for females. For males, there were no effects of migration distance when data were standardized by flyway, but males that migrated faster arrived earlier, and were mated to females that began breeding later. This suggests males migrating at faster paces may experience a cost in their ability to acquire an early breeding mate, even though they arrived early to the breeding site. In contrast, when data were standardized across all individuals, we show that overall migration distance is positively associated with fledging success, and thus, may be representing broader life-history differences.
It is possible that the negative relationship between migration distance on young fledged in females was the result of location-specific life history variation rather than a carry-over effect. However, we argue that this is unlikely for several reasons. First, breeding sites within the Central and Eastern Flyways varied little in latitude within their flyways (i.e., 1.68°N in the Central flyway and 3.05°N in Eastern flyway), suggesting any such effects of migration distance were likely unrelated to latitudinal variation in the breeding location. Second, within breeding sites there was often large variation in migration distance (Table 1). This was especially true of birds in the Central flyway as individuals migrated to Mexico, the Gulf of Mexico, and in some cases, Florida resulting in migration distances within sites that varied by over 1,000 km (Figure 1). Thus, by standardizing within flyways we were able to separate the potential effects of breeding location from migration on reproductive variables. However, future research that includes additional sites and individuals within a migratory network would likely provide a clearer picture of the effect of migration distance on reproduction.
In contrast, when standardizing across all individuals, males and females that migrated the farthest distances fledged the most young. We argue that this result was likely driven by broader life-history differences related to breeding latitude (Ricklefs, 1980; Dunn et al., 2000; Jetz et al., 2008) rather than migration distance, per se. Tree swallows wintered within a narrow band ranging ~11° of latitude whereas their breeding range covers ~34° of latitude (Winkler et al., 2011), leading to spring migration distance, when examined across the entire network, being positively correlated with breeding latitude (see also Gow et al., 2019). Previously, we provided evidence that tree swallows breeding at higher latitudes arrived later and began breeding later (Gow et al., 2019), similar to the positive effects of migration distance we observed on first egg date of males and females in this study when we standardized across individuals.
One reason we observed a negative effect of migration distance on young fledged in females but not males (when data were standardized within flyways) may be related to how the sexes differ in their sensitivity to the energetic or physiological costs of migration distance. Under the reproductive stress hypothesis, the sex with the higher reproductive demands will be more sensitive to their energetic or physiological state when investing in reproduction (Nagy et al., 2007; Gow et al., 2013; Gow and Wiebe, 2014). In tree swallows, females invest in reproduction through nest building, egg laying, incubation, feeding young and intense female-female competition. Males only engage in feeding young, but also invest in male-male competition, pursuit of extra-pair fertilizations, and securing and defending nest sites (Winkler et al., 2011). Thus, female tree swallows are the sex investing more heavily in the production of young. Experimental studies manipulating female quality or timing of breeding (Winkler and Allen, 1995; Dawson, 2008; Harriman et al., 2016) suggest a female's quality may affect her ability to produce and care for offspring. In this way, it is possible that migration distance influenced the number of young fledged by affecting female condition, but did not affect whether a male was capable of mating with a female that produced more young.
Male tree swallows that migrated faster relative to other individuals in their flyway arrived earlier to the breeding site. However, arriving earlier to the breeding grounds may not necessarily be beneficial if it leaves an individual in a poorer condition (e.g., González-Prieto and Hobson, 2013), unless they acquired larger reserves prior to migration (Bayly et al., 2016). Arriving to the breeding grounds in a poor physiological state may be particularly detrimental for male tree swallows given their reliance on aerial insects. Interestingly, male tree swallows that arrived early to the breeding grounds (when standardizing by flyway) seemed to be mated to females that began breeding relatively late. One reason for this negative relationship may be related to how breeding tree swallows are impacted by poor weather (Weegman et al., 2017; Cox et al., 2019) and low insect abundances (McCarty and Winkler, 1999; Imlay et al., 2017). Both poor weather and low insect abundances may impair reproductive performance via their effects on the timing of breeding (Dawson, 2008; Harriman et al., 2016), and female body condition (Winkler and Allen, 1995; Paquette et al., 2014). These factors may also differ among flyways. Populations in the Central and Western flyways appear to be more strongly affected by timing of breeding and insect abundances during egg laying (Dawson, 2008; Harriman et al., 2016), while those in the Eastern flyway may experience more negative effects from poor weather conditions (Weegman et al., 2017; Cox et al., 2019) rather than variation in insect abundance (McCarty and Winkler, 1999; Imlay et al., 2017). This difference may explain why when we did not standardize by flyway and instead standardized across all individuals faster arriving males arrived earlier (similar to within flyway standardization). These early arriving males mated with females whom also began breeding earlier, demonstrating an overall positive benefit to migrating faster and arriving early across the range.
Our findings provide valuable insight into how migration distance may influence current and future population declines of tree swallows, as well as other species. Many tree swallow populations in the northeastern parts of their range have experienced declines over the past couple of decades (Shutler et al., 2012). The cause(s) of these declines are unclear, but one possibility is that deterioration of overwintering habitat quality influences survival and carries over to influence reproductive performance. Another mechanism for population declines in this species, and potentially others, may occur if individuals are forced to migrate farther. Individuals may migrate farther distances if there is a reduction in habitat quality, which may reduce the carry-capacity of those sites (e.g., Stutchbury et al., 2016), forcing individuals to seek alternative roosting sites farther south. For swallows breeding in the Western or Central flyways, this may mean either crossing the Gulf of Mexico or moving to areas farther south in Central America, whereas those breeding in the Eastern flyway may be forced to seek habitat on Caribbean Islands or travel even farther distances to Mexico. Alternatively, with the globally rising temperatures, suitable habitat for tree swallows may be available farther north. The geographic differences among flyways may affect the potential distances some individual migrate, which in turn may affect the number of young fledged, and contribute to population declines.
Data Availability Statement
All datasets generated for this study are included in the manuscript/Supplementary Files.
Ethics Statement
This study was carried out in accordance with the principles of Animal Utilization Protocols or Animal Care Protocols and was approved by each University of the primary researcher for each field site.
Author Contributions
EG and DN designed the research and wrote the manuscript. EG analyzed these data. SK processed the geolocator data. All other authors conducted fieldwork and helped with manuscript revisions.
Funding
Funding was provided by Leaders Opportunity Fund Grants from the Canadian Foundation for Innovation (DN and RD), Natural Sciences and Engineering Research Council of Canada (NSERC) Discovery Grants (DN, RC, RD, MB, DG, FP, and ML), an NSERC Research Tools and Instruments Grant (DN, MB, RD, DG, ML, FP, and DS), an NSERC Industrial Research and Development Fellowship (DB), an NSERC Alexander Graham Bell Canada Graduate Scholarship (LLB), the NSERC Canada Research Chairs Program (MB and FP), the University of Guelph (DN), Environment and Climate Change Canada (RC and OL), Bird Studies Canada (DB and DH), the University of Northern British Columbia (RD), the British Columbia Knowledge Development Fund (RD), the Skaggs Foundation (TB), a National Science Foundation Grant DEB-0933602 (CT), a National Science Foundation Grant IOS-0745156 (CV and DV), National Science Foundation Grants DEB-0717021 and DEB-1242573 (DW), Fonds de Recherche du Québec – Nature et Technologies (MB, DG, and FP), the James S. McDonnell foundation (CT), the Alberta Conservation Association (GH and HT), TD Friends of the Environment (GH and HT), the Shell Environmental Fund (GH and HT), and Nature Canada's Charles Labatiuk Nature Endowment Fund (GH and HT). The development and analysis of some of the geolocators were supported by the National Science Foundation Grants Nos. IDBR 1152356 and DEB 0946685 (EB), IDBR 1152131 (DW).
Conflict of Interest
The authors declare that the research was conducted in the absence of any commercial or financial relationships that could be construed as a potential conflict of interest.
The reviewer, MH, declared past collaborations with several of the authors, DN, DW, CT, and EB, to the handling editor.
Acknowledgments
We thank the numerous field assistants, graduate students, undergraduate students, and volunteers who assisted with fieldwork at all breeding sites used in this study.
Supplementary Material
The Supplementary Material for this article can be found online at: https://www.frontiersin.org/articles/10.3389/fevo.2019.00380/full#supplementary-material
References
Alerstam, T. (2011). Optimal bird migration revisited. J. Ornithol. 152, S5–S23. doi: 10.1007/s10336-011-0694-1
Alerstam, T., Hedernström, A., and Åkesson, S. (2003). Long-distance migration: evolution and determinants. Oikos 103, 247–260. doi: 10.1034/j.1600-0706.2003.12559.x
Alves, J. A., Gunnarsson, T. G., Potts, P. M., Gélinaud, G., Sutherland, W. J., and Gill, J. A. (2012). Overtaking on migration: does longer distance migration always incur a penalty. Oikos 121, 464–470. doi: 10.1111/j.1600-0706.2011.19678.x
Arnold, T. W. (2010). Uninformative parameters and model selection using Akaike's Information Criterion. J. Wildl. Manage. 74, 1175–1178. doi: 10.1111/j.1937-2817.2010.tb01236.x
Barber, C. A., Robertson, R. J., and Boag, P. T. (1996). The high frequency of extra-pair paternity in tree swallows is not an artefact of nestboxes. Behav. Ecol. Sociobiol. 38, 425–430. doi: 10.1007/s002650050260
Bart, J., and Earnst, S. L. (1999). Relative importance of male territory quality in pairing success of male rock ptarmigan (Lagopus mutus). Behav. Ecol. Sociobiol. 45, 355–359. doi: 10.1007/s002650050571
Barton, K. (2016). MuMIn: Multi-Model Inference. R package. Available online at: https://CRAN.R-project.org/package=MuMIn.
Bayly, N., Gómez, C., Hobson, K., and Rosenberg, K. (2016). Prioritizing tropical habitats for long-distance migratory songbirds: an assessment of habitat quality at a stopover site in Colombia. Avian Conserv. Ecol. 11:5. doi: 10.5751/ACE-00873-110205
Bearhop, S., Fiedler, W., Furness, R. W., Votier, S. C., Waldron, S., Newton, J., et al. (2005). Assortative mating as a mechanism for rapid evolution of a migratory divide. Science 310, 502–504. doi: 10.1126/science.1115661
Betini, G. S., Griswold, C. K., and Norris, D. R. (2013). Carry-over effects, sequential density dependence and the dynamics of populations in a seasonal environment. Proc. R. Soc. B 280:20130110. doi: 10.1098/rspb.2013.0110
Bradley, D. W., Clark, R. G., Dunn, P. O., Laughlin, A. J., Taylor, C. M., Vleck, C. M., et al. (2014). Trans-Gulf of Mexico loop migration of tree swallows revealed by solar geolocation. Curr. Zool. 60, 653–659. doi: 10.1093/czoolo/60.5.653
Bregnballe, T., Fredericksen, M., and Gregersen, J. (2006). Effects of distance to wintering area on arrival date and breeding performance in Great Cormorants Phalacrocorax carbo. Ardea 94, 619–630.
Briedis, M., Bauer, S., Adamík, P., Alves, J. A., Costa, J. S., Emmenegger, T., et al. (2019). A full annual perspective on sex-biased migration timing in long-distance migratory birds. Proc. R. Soc. B 286:20182821. doi: 10.1098/rspb.2018.2821
Conklin, J. R., Senner, N. R., Battley, P. F., and Theunis, P. (2017). Extreme migration and the individual quality spectrum. J. Avian. Biol. 48, 19–36. doi: 10.1111/jav.01316
Cox, A. R., Robertson, R. J., Lendvai, Á. Z., Everitt, K., and Bonier, F. (2019). Rainy springs linked to poor nestling growth in a declining avian aerial insectivore. Proc. R. Soc. B 286:20190018. doi: 10.1098/rspb.2019.0018
Cox, A. S., Robertson, R. J., Fedy, B. C., Rendell, W. B., and Bonier, F. (2018). Demographic drivers of local population declines in tree swallows (Tachycineta bicolor) in Ontario, Canada. Condor 120, 842–851. doi: 10.1650/CONDOR-18-42.1
Dawson, R. D. (2008). Timing of breeding and environmental factors as determinants of reproductive performance of tree swallows. Can. J. Zool. 86, 843–850. doi: 10.1139/Z08-065
Dunn, P. O., Thusius, K. J., Kimber, K., and Winkler, D. W. (2000). Geographic and ecological variation in clutch size of tree swallows. Auk 117, 215–221. doi: 10.1093/auk/117.1.215
Fraser, K. C., Stutchbury, B. J. M., Silverio, C., Kramer, P. M., Barrow, J., Newstead, D., et al. (2012). Continent-wide tracking to determine migratory connectivity and tropical habitat association of a declining aerial insectivore. Proc. R. Soc. Lond. B 279, 4901–4906. doi: 10.1098/rspb.2012.2207
González-Prieto, A. M., and Hobson, K. A. (2013). Environmental conditions on wintering grounds and during migration influence spring nutritional condition and arrival phenology of Neotropical migrants at a northern stopover site. J Ornithol. 154, 1067–1078. doi: 10.1007/s10336-013-0975-y
Gow, E. A., Burke, L., Winkler, D. W., Knight, S. M., Bradley, D. W., Clark, R. G., et al. (2019). A range-wide domino effect and resetting of the annual cycle in a migratory songbird. Proc. R. Soc. B 286:20181916. doi: 10.1098/rspb.2018.1916
Gow, E. A., Musgrove, A. B., and Wiebe, K. L. (2013). Brood age and size influence sex-specific parental provisioning patterns in a sex-role reversed species. J. Ornithol. 154, 525–535. doi: 10.1007/s10336-012-0923-2
Gow, E. A., and Wiebe, K. L. (2014). Males migrate farther than females in a differential migrant: an examination of the fasting endurance hypothesis. R. Soc. Open Sci. 1:140346. doi: 10.1098/rsos.140346
Gunnarsson, T. G., Gill, J. A., Atkinson, P. W., Gélinaud, G., Potts, P. M., Croger, R. E., et al. (2006). Population-scale drivers of individual arrival times in migratory birds. J. Anim. Ecol. 75, 1119–1127. doi: 10.1111/j.1365-2656.2006.01131.x
Harriman, V. B., Dawson, R. D., Bortolotti, L. E., and Clark, R. G. (2016). Seasonal patterns in reproductive success of temperate-breeding birds: experimental tests of the date and quality hypothesis. Ecol. Evol. 7, 2122–2132. doi: 10.1002/ece3.2815
Harrison, X. A., Blount, J. D., Inger, R., Norris, D. R., and Bearhop, S. (2011). Carry-over effects as drivers of fitness differences in animals. J. Anim. Ecol. 80, 4–18. doi: 10.1111/j.1365-2656.2010.01740.x
Hochachka, W. (1990). Seasonal decline in reproductive performance of song sparrows. Ecology 71, 1279–1288. doi: 10.2307/1938265
Hötker, H. (2002). Arrival of pied avocets Recurvirostra avosetta at the breeding site: effects of winter quarters and consequences for reproductive success. Ardea 90, 379–387.
Imlay, T. L., Mann, H. A. R., and Leonard, M. L. (2017). No effect of insect abundance on nestling survival or mass for three aerial insectivores. Avian Conserv. Ecol. 12:19. doi: 10.5751/ACE-01092-120219
Jetz, W., Sekercioglu, C. H., and Böhning-Gaese, K. (2008). The worldwide variation in avian clutch size across species and space. PLoS Biol. 6:e303. doi: 10.1371/journal.pbio.0060303
Kempenaers, B., Everding, S., Bishop, C., Boag, P., and Robertson, R. J. (2001). Extra-pair paternity and the reproductive role of male floaters in the tree swallow (Tachycineta bicolor). Behav. Ecol. Sociobiol. 49, 251–259. doi: 10.1007/s002650000305
Kentie, R., Marquez-Ferrando, R., Figuerola, J., Gangoso, L., Hooijmeijer, J. C. E. W., Loonstra, A. H. J., et al. (2017). Does wintering north or south of the Sahara correlate with timing and breeding performance in black-tailed godwits. Ecol. Evol. 7, 2812–2820. doi: 10.1002/ece3.2879
Knight, S. M., Bradley, D. W., Clark, R. G., Gow, E. A., Bélisle, M., Berzins, L., et al. (2018). Constructing and evaluating a continent-wide migratory songbird network across the annual cycle. Ecol. Monogr. 88, 445–460. doi: 10.1002/ecm.1298
Leyrer, J., Lok, T., Brugge, M., Spaans, B., Sandercock, B. K., and Piersma, T. (2013). Mortality within the annual cycle: seasonal survival patterns in Afro-Siberian red knots Calidris canutus canutus. J. Ornithol. 154, 933–943. doi: 10.1007/s10336-013-0959-y
Lifjeld, J. T., Dunn, P. O., Robertson, R. J., and Boag, P. T. (1993). Extra-pair paternity in monogamous tree swallows. Anim. Behav. 45, 213–229. doi: 10.1006/anbe.1993.1028
Lok, T., Overdijk, O., and Piersma, T. (2014). The cost of migration: spoonbills suffer higher mortality during trans-Saharan spring migrations only. Biol. Lett. 11:20140944. doi: 10.1098/rsbl.2014.0944
Lok, T., Veldhoen, L., Overdijk, O., Tinbergen, J. M., and Piersma, T. (2016). An age-dependent fitness cost of migration? Old trans-Saharan migrating spoonbills breed later than those staying in Europe, and late breeders have lower recruitment. J. Anim. Ecol. 86, 998–1009. doi: 10.1111/1365-2656.12706
McCarty, J. P., and Winkler, D. W. (1999). Relative importance of environmental variables in determining the growth of nestling tree swallows Tachycineta bicolor. Ibis 141, 286–296. doi: 10.1111/j.1474-919X.1999.tb07551.x
McKinnon, E. A., Fraser, K. C., and Stutchbury, B. J. M. (2013). New discoveries in landbird migration using geolocators, and a flight plan for the future. Auk 130, 211–222. doi: 10.1525/auk.2013.12226
McKinnon, E. A., and Love, O. P. (2018). Ten years tracking the migrations of small landbirds: lessons learned in the golden age of bio-logging. Auk 135, 834–856. doi: 10.1642/AUK-17-202.1
Millet, A., Pelletier, F., Bélisle, M., and Garant, D. (2015). Patterns of fluctuating selection on morphological and reproductive traits in female tree swallows (Tachycineta bicolor). Evol. Biol. 42, 349–358. doi: 10.1007/s11692-015-9333-8
Mitchell, R. J. (1993). “Path analysis: pollination,” in Design and Analysis of Ecological Experiments, eds S. M. Scheimer and J. Gurevitch (New York, NY: Oxford University Press), 211–231.
Nagy, L. R., Stanculescu, D., and Holmes, R. T. (2007). Mass loss by breeding female songbirds: food supplementation supports energetic stress hypothesis in black-throated blyue warblers. Condor 109, 304–311. doi: 10.1093/condor/109.2.304
Norris, D. R., Marra, P., Montgomerie, R., Kyser, T., and Ratcliffe, L. M. (2004). Reproductive effort, molting latitude, and feather color in a migratory songbird. Science 306, 2249–2250. doi: 10.1126/science.1103542
Norris, D. R., and Marra, P. P. (2007). Seasonal interactions, habitat quality, and population dynamics in migratory birds. Condor 109, 535–547. doi: 10.1093/condor/109.3.535
O'Brien, E. L., and Dawson, R. D. (2007). Context-dependent genetic benefits of extra-pair mate choice in a socially monogamous passerine. Behav. Ecol. Sociobiol. 61, 775–782. doi: 10.1007/s00265-006-0308-8
Paquette, S. R., Pelletier, F., Garant, D., and Bélisle, M. (2014). Severe recent decrease of adult body mass in a declining insectivorous bird population. Proc. R. Soc. B 281:20140649. doi: 10.1098/rspb.2014.0649
Pinheiro, J., Bates, D., Debroy, S., Sarkar, D., and R Core Team (2018). Nlme: Linear and Nonlinear Mixed Effects Models. R package. Available online at: https://CRAN.R-project.org/package=nlme.
R Core Development Team (2016). R: A Language and Environment for Statistical Computing. Vienna: R Foundation for Statistic Computing. Available online at: http://www.R-project.org.
R Core Development Team (2018). R: A Language and Environment for Statistical Computing. Vienna: R Foundation for Statistial Computing. Available online at: http://www.R-project.org.
Rakhimberdiev, E., van den Hout, P. J., Brugge, M., Spaans, B., and Piersma, T. (2015a). Seasonal mortality and sequential density dependence in a migratory bird. J. Avian Biol. 46, 332–341. doi: 10.1111/jav.00701
Rakhimberdiev, E., Winkler, D. W., Bridge, E., Seavy, N. E., Sheldon, D., Piersma, T., et al. (2015b). A hidden Markov model for reconstructing animal paths from solar geolocation loggers using templates for light intensity. Mov. Ecol. 3, 1–15. doi: 10.1186/s40462-015-0062-5
Ricklefs, R. E. (1980). Geographical variation in clutch size among passerine birds: Ashmole's hypothesis. Auk 97, 38–49.
Senner, N. R., Verhoeven, M. A., Abad-Gómez, J. M., Alves, J. A., Hooijmeijer, J. C. E. W., Howison, R. A., et al. (2019). High migratory survival and highly variable migratory behavior in black-tailed godwits. Front. Ecol. Evol. 7:96. doi: 10.3389/fevo.2019.00096
Shipley, B. (2000). Cause and Correlation in Biology: A User's Guide to Path Analysis, Structural Equations and Causual Interence. Cambridge: Cambridge University Press. doi: 10.1017/CBO9780511605949
Shipley, B. (2009). Confirmatory path analysis in a generalized multilevel context. Ecology 90, 363–368. doi: 10.1890/08-1034.1
Shipley, B. (2013). The AIC model selection method applied to path analytic models compared using a d-separation test. Ecology 94, 560–564. doi: 10.1890/12-0976.1
Shutler, D., Hussell, D., Norris, D., Winkler, D., Robertson, R., Bonier, F., et al. (2012). Spatiotemporal patterns in nest box occupancy by tree swallows across North America. Avian Conserv. Ecol. 7:3. doi: 10.5751/ACE-00517-070103
Stutchbury, B. J., Siddiqui, R., Applegate, K., Hvenegaard, G. T., Mammenga, P., Mickle, N., et al. (2016). Ecological causes and consequences of intratropical migration in temperate-breeding migratory birds. Am. Nat. 188, S28–S40. doi: 10.1086/687531
Verhulst, S., and Nilsson, J. (2008). The timing of birds' breeding seasons: a review of experiments that manipulated timing of breeding. Philos. Trans. R. Soc. B 363, 399–410. doi: 10.1098/rstb.2007.2146
Weegman, M. D., Arnold, T. W., Dawson, R. D., Winkler, D. W., and Clark, R. G. (2017). Integrated population models reveal local weather conditions are the key drivers of population dynamics in an aerial insectivore. Oecologia 185, 119–130. doi: 10.1007/s00442-017-3890-8
Whittingham, L. A., and Dunn, P. O. (2001). Survival of extrapair and within-apir young in tree swallows. Behav. Ecol. 12, 496–500. doi: 10.1093/beheco/12.4.496
Wikelski, M., Tarlow, E. M., Raim, A., Diehl, R. H., Larkin, R. P., and Visser, G. H. (2003). Costs of migration in free-flying songbirds. Nature 423:704. doi: 10.1038/423704a
Winkler, D. W., and Allen, P. E. (1995). Effects of handicapping on female condition and reproduction in tree swallows. Auk 112, 737–747.
Winkler, D. W., Hallinger, K. K., Ardia, D. R., Robertson, R. J., Stutchbury, B. J. M., and Cohen, R. R. (2011). “Tree swallow (Tachycineta bicolor),” in The Birds of North America, ed P. G. Rodewald (Ithaca, NY: Cornell Lab of Ornithology).
Keywords: tree swallow, migration, geolocation, migration distance, path analysis, young fledged
Citation: Gow EA, Knight SM, Bradley DW, Clark RG, Winkler DW, Bélisle M, Berzins LL, Blake T, Bridge ES, Burke L, Dawson RD, Dunn PO, Garant D, Holroyd G, Horn AG, Hussell DJT, Lansdorp O, Laughlin AJ, Leonard ML, Pelletier F, Shutler D, Siefferman L, Taylor CM, Trefry H, Vleck CM, Vleck D, Whittingham LA and Norris DR (2019) Effects of Spring Migration Distance on Tree Swallow Reproductive Success Within and Among Flyways. Front. Ecol. Evol. 7:380. doi: 10.3389/fevo.2019.00380
Received: 25 April 2019; Accepted: 23 September 2019;
Published: 11 October 2019.
Edited by:
Nathan R. Senner, University of South Carolina, United StatesReviewed by:
Emily Anne McKinnon, University of Manitoba, CanadaRose J. Swift, Northern Prairie Wildlife Research Center (United States Geological Survey), United States
Michael T. Hallworth, Northeast Climate Adaptation Science Center, United States
Copyright © 2019 Gow, Knight, Bradley, Clark, Winkler, Bélisle, Berzins, Blake, Bridge, Burke, Dawson, Dunn, Garant, Holroyd, Horn, Hussell, Lansdorp, Laughlin, Leonard, Pelletier, Shutler, Siefferman, Taylor, Trefry, Vleck, Vleck, Whittingham and Norris. This is an open-access article distributed under the terms of the Creative Commons Attribution License (CC BY). The use, distribution or reproduction in other forums is permitted, provided the original author(s) and the copyright owner(s) are credited and that the original publication in this journal is cited, in accordance with accepted academic practice. No use, distribution or reproduction is permitted which does not comply with these terms.
*Correspondence: Elizabeth A. Gow, egow@uoguelph.ca
†Deceased