- 1Department of Rural Engineering, Plant Health and Soils (DEFERS), São Paulo State University, São Paulo, Brazil
- 2Departament of Soil Science, University of São Paulo, São Paulo, Brazil
Sugarcane holds the prominent position in global economy. Extensive research is needed to enhance the efficiency of phosphorous fertilizers which increase the cost of sugarcane production more specifically in the less fertile soils which are weathered and phosphorous deficit as tropical region soils. We are currently living in a transitional period called the “Green Micro-revolution,” in which the use of plant growth-promoting bacteria (PGPB) is justified and although bacteria such as Azospirillum brasilense, Bacillus, and Pseudomonas fluorescens in other crops are recognized for increasing production however, little is known about the effects of these microorganisms on sugarcane. The current study aimed to evaluate the effect of inoculation with three species of plant growth promoting bacteria (A. brasilense, Bacillus subtilis, and P. fluorescens) without or with combine application of reduced doses of phosphate fertilizer, in the P soil available, P leaf concentration, shoot yield and P accumulation in sugarcane (cane-plant) at the end of the cycle. The experiment was carried out with a sugarcane crop in a Hapludox Rhodic with low available P content, in a randomized block design with 8 × 5 factorial scheme, being eight inoculations and five P doses (0, 45, 90, 135, and 180 kg ha−1 P2O5) as triple superphosphate. The bacterial inoculations influenced the leaf P content, so that inoculation with B. subtilis + P. fluorescens provided the highest concentration of phosphorus in the sugarcane leaf. Both the layers of the soil were differently influenced by inoculation and P doses whereas inoculation with A. brasilense + P. fluorescens at 135 kg P2O5 ha−1 provided the highest soil P content in the soil layer of 0–0.25 m. Although, maximum stalk yield was obtained with inoculation of B. subtilis + P. fluorescens at the dose of 135 kg ha−1 P2O5. The inoculation of A. brasilense + B. subtilis with application of 45 kg P2O5 ha−1 improved dry matter, total P accumulation and stalk production by 38% in sugarcane variety (RB92579) and reduced P fertilization by 75% for the same variety grown in low-P soil.
Introduction
Brazil has the title of world's largest producer of sugarcane, followed by India and China and accounts for 28% of ethanol and 16% of sugar consumed worldwide (RFA, 2019; USDA, 2019). This follow-up only drives the country's agribusiness forward, as the global demand for ethanol is growing every day and the soil and climatic conditions of the region are very favorable to the cultivation of this of cane crop, along with the availability of extensive agricultural areas (CONAB, 2019). In the last harvest of sugarcane (2018/2019) with cultivated of 8.5 million hectare, the country hit production of 633.3 million tons with an average yield of 72.2 t ha−1. The state of São Paulo was occupying 51% of the sugarcane cultivated area and is responsible for 53% of national production (CONAB, 2019).
One of the main limitations is the poor and inadequate fertile soils which fail to meet the nutritional and growth requirements and hence unable to achieve high production. Phosphorus (P) is the most critical element with high interaction with soil (Raij, 2011), required in a small quantity by sugarcane in comparison of N and K but still plays an essential role in the development of tillering and root system (Kingston, 2014) and greatly influence the longevity. Most of Brazilian soils (tropical and weathered) are highly deficient in phosphorus. Even though the soils are efficient with total P but still a tiny fraction of P is available to plants (Sampaio, 2011). The low availability of phosphorus is due to low P in the source material, clay adsorption and its precipitation with oxides and hydroxides of Fe and Al (Caione et al., 2015). Therefore, a huge quantity of P fertilizers is applied which significantly adds to the cost of production of cane crop. In addition, phosphate fertilizers are produced by phosphate rock and therefore, Morocco being responsible for 85% of the known active mining reserves (Campos et al., 2018) and it is estimated that it will be diminish among 200 to 300 years (Sattari et al., 2012). Around 10 to 30% of the phosphorus fertilizer applied in the first year is absorbed by roots of cane crop whereas an extensive amount accumulates in the soil as fixed P, not available to plants (Syers et al., 2008). It is necessary to find alternatives to reduce the use of phosphate fertilizers. Since every day looking to a more sustainable agriculture combined with increase in productivity and economically viable.
The use of plant growth promoting bacteria (PGPB) is a promising alternative with low environmental impact to increase the efficiency of use of mineral fertilizers, including phosphate, providing high cost-effective yields (Spolaor et al., 2016). The PGPB promote plant growth due to various mechanisms such as biosynthesis of phytohormones and secondary metabolites (Duca et al., 2014; Tahir et al., 2017), biological nitrogen fixation (Li et al., 2017), induction of resistance to biotic stresses (phytopathogen biocontrol) and abiotic stresses (drought and salinity) (Yan et al., 2016; Takishita et al., 2018) production of siderophores metal accumulator (Ali et al., 2014) and soil nutrient solubilization such as phosphorus and potassium (Gupta et al., 2015; Shen et al., 2016; Patel and Archana, 2017). Bacteria with combination of these mechanisms, promote and increase the productivity of several crops (Kumar et al., 2014; Smith et al., 2015; Galindo et al., 2016).
Some of these microorganisms are being studied together to enhance P utilization which is unavailable/immobilized in the soil. Hereby theses microbes try to make P available to the plants and reduce the application of P which certainly decrease the hazardous environmental impact caused by the application of these fertilizers. Phosphate solubilization or increased inorganic P availability by PGBP in vitro is observed by proton production, organic acid binders and phytate (organic P) mobilization probably due to phytase production mobilization phytate (organic P) probably by the production of phytase (Jorquera et al., 2008; Hinsinger et al., 2011) provided by these microorganisms.
Several field and greenhouse researches with phosphate fertilizer in sugar cane (Calheiros et al., 2012; Caione et al., 2015; Albuquerque et al., 2016; Borges et al., 2019) however, they did not work with inoculation of phosphate-solubilizing bacteria (alone or in combination). Plant growth-promoting bacteria (PGPB) are playing a key role in plant health and growth and can be promisingly applied in agricultural production to reduce the use of chemical fertilizers. This manuscript evaluated the effect of inoculation with three PGPB species and five P doses in sugarcane. Inoculation can play a fundamental role in cultivation, generating great benefits to the crop and saving fertilizers cost for the producers. The results revealed a combination of Azospirillum brasilense and Bacillus subtilis allied to the low dose of P2O5 was the best fertilizer management in the sugarcane, which is meaningful for production practice of sugarcane. Seeking for further study with PGPB and its ability to solubilize inorganic phosphate, this study aimed to assess the effect of inoculation with three species of plant growth-promoting or phosphate solubilizing bacteria associated with or without reduced phosphate fertilizer application on soil P content, leaf P concentration, stalk yield (kg ha−1), dry matter and P accumulation in sugarcane at the end of cycle.
Materials and Methods
Location of the Experimental Area
The research was conducted in the field with sugar cane crop (crop year 2017/18) in Ilha Solteira in area belonging to one of sugar and ethanol industry, located at the northwest state of São Paulo—Brazil. Geographically, the area is located at the latitude 20° 21′ S, longitude 51° 04′ W and an altitude of 371 meters. The area was being cultivated with Urochloa brizantha for the last 10 years which was grazed and pasture degraded. The soil of the area is a Dystrophic Red Latosol with a medium to coarse texture, classified according to criteria established by Brazilian Society of Soil Science (Santos et al., 2018), and as a Rhodic Hapludox according to the Soil Survey Staff (2014), with a particle size of 777, 98, 125 and 747, 88, 165 g kg−1 of sand, silt and clay at depths of 0.25-0.50 m and from 0.00 to 0.25, respectively. The soil was chemically analyzed before experiment implantation according to the methodology proposed by van Raij et al. (2001) and described in Table 1.
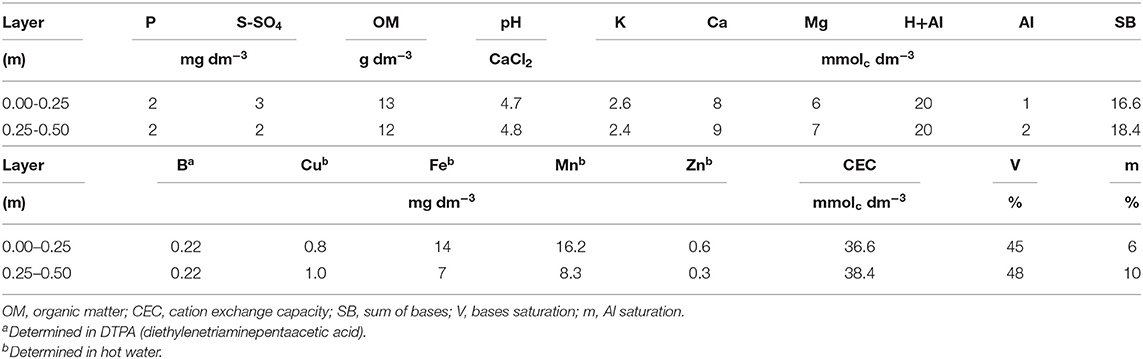
Table 1. Pre-experiment soil chemical characterizations in the soil layers of 0.00–0.25 and 0.25–0.50 m.
According to Koppen, the climate type of the region is classified as Aw which was defined as humid tropical with the rainy season in summer and dry in winter. Climatological data were recorded throughout the experimental period (Figure 1).
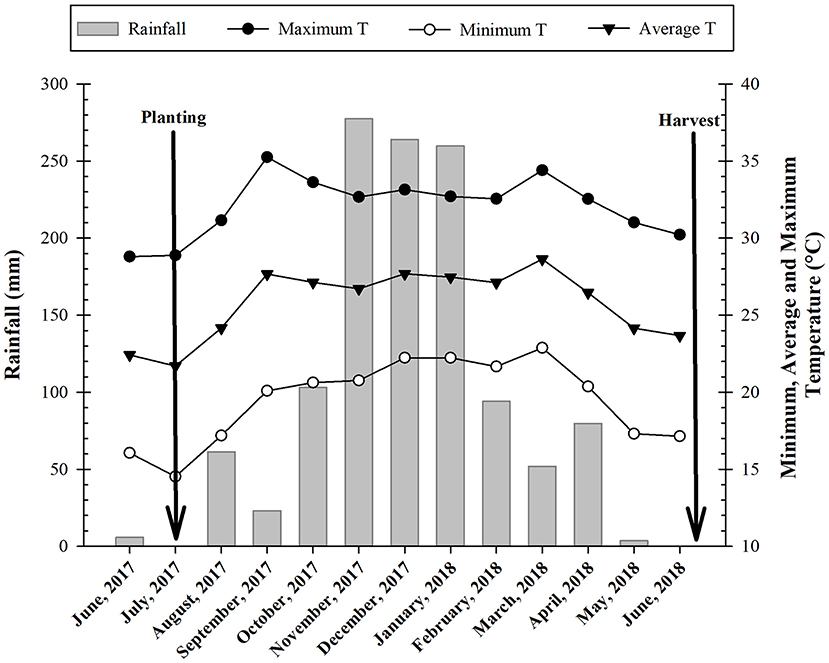
Figure 1. Monthly averages of precipitation (mm), maximum, average, and minimum temperatures recorded during the period in which the experiment was conducted. Ilha Solteira, state of São Paulo, Brazil, 2017/2018.
Experimental Design and Treatments
The experimental design was a randomized block with three replicates, arranged in 8 × 5 factorial scheme, comprised of eight inoculations (1) Without inoculation—control (W.I.), (2) Inoculation with Azospirillum brasilense (Azo), (3) Inoculation with Bacillus subtilis (Bac), (4) Inoculation with Pseudomonas fluorescens (Pseud), (5) Inoculation with A. brasilense + B. subtilis (Azo + Bac), (6) Inoculation with A. brasilense + P. fluorescens (Azo + Pseud), (7) Inoculation with B. subtilis + P. fluorescens (Bac + Pseud), (8) Inoculation with A. brasilense + B. subtilis + P. fluorescens (Azo + Bac + Pseud), and five phosphorus doses at planting (0, 45, 90, 135, and 180 kg ha−1 P2O5) as a source of triple superphosphate, corresponding to 0, 25, 50, 75, and 100% of the recommended dose for sugarcane crop according to Raij and Cantarella (1997).
Establishment and Management of the Experiment
After pre-experiment soil chemical characterization and 15 days before planting, soil profile prepared with application of 1 t ha−1 of limestone (PRNT 85%) in order to obtain 60% saturation of bases and 1 t ha−1 of gypsum to increase the sulfur (S), as recommended by Raij and Cantarella (1997) for sugarcane crop. Three gradings and subsoiling were carried out, after which the soil was furrowed at 0.40 m depth and insecticide fipronil (180 g ha−1 of the active ingredient—ai) + fungicide pyraclostrobin (125 g ha−1 of ai) were applied in the planting furrow. The sugarcane variety RB92579 was grown on 11th July, 2017 by adapting manual planting system. One-meter long channel of cane was having around 22 buds, cut and placed within the planting furrows. The plots were 5 m long with five rows, spaced 1.5 m, whereas central three rows were used for data collection.
The following doses of liquid inoculants were used for bacterial inoculations: A. brasilense [strains Ab-V5 and Ab-V6 with guarantee of 2 × 108 colony forming units (CFU) ml−1] was applied at the dose of 1.0 L ha−1; B. subtilis (strain CCTB04, with guarantee of 1 × 108 CFU ml−1) and P. fluorescens (strain CCTB03, with guarantee of 2 × 108 CFU mL−1) were applied at the dose of 0.5 L ha−1, based on the manufacturer's recommendation. The spray volume was 200 L ha−1 (for three bacterial inoculations) applied by backpack sprayer pump spraying in the furrow of sugarcane plant. The spray was applied in the late afternoon to prolong the influence of bacterial inoculation with lower temperature.
The recommended doses of nitrogen and potassium was applied to all the treatment beyond the levels of phosphorus at the time of sowing. Nitrogen was applied at the rate of 30 kg N ha−1 from a source of ammonium nitrate and potassium was applied at the rate of 120 kg K2O ha−1 from the source of potassium chloride based on soil analysis and utilization of fertilizer by the plant. The experiment was irrigated with sprinkler irrigation system (30 mm slide) soon after 5 days of sugarcane sowing due to low precipitation period. The plots were applied with 5 kg Zn ha−1 from zinc sulfate at the time of tillering. This application was done due to the reasons that experimental soil was deficient with micronutrients and phosphate was used as main factor which in excess can lead to zinc deficiency due to antagonistic effect, in which phosphate react with Zn and make it less soluble for absorption by plants.
Throughout cane life cycle different chemicals were applied for the control weeds, pests, and diseases: pre-emergence (24 days after planting—DAP) the hexazinone herbicide (320 g ai ha−1) + 2.4 D (967 g ai ha−1) + tebuthiuron (1,000 g ai ha−1). At 110 DAP, in the lion breaking 2.4 D (967 g ai ha−1) + Amicarbazone (840 g ai ha−1) + S-metolachlor (2,400 g ai ha−1) were applied. At 101 and 199 DAP, insecticides chlorantraniliprole (10 g ai ha−1) + lambda-cyhalothrin (5 g ai ha−1) were applied. Biological control of the sugarcane borer (Diatraea saccharalis) was used through the release of Trichogramma galloi (at 142, 148, 213, and 220 DAP, respectively) and Cotesia flavipes (at 192 DAP).
In order to induce the sugarcane to accumulate sucrose, application of maturing agent, trinexapaque-ethyl (275 g ai ha−1) was performed at 301 DAP. The crop was manually harvested after 349 days of planting on 25th June, 2018.
Assessments
To determine the leaf P concentration (Malavolta et al., 1997), at the stage of higher vegetative development of the crop, at 195 DAP, were collected in the morning, the middle third 15 flag leaves (leaf diagnose- highest with visible leaf collar) per share, excluding the central rib, as recommended by Raij and Cantarella (1997).
Five representative plants from each plot were harvested 349 DAP. Its parts were properly separated into stalks s and leaves, dried in air forced desiccator at 65°C until obtaining a constant weight. Each sample was then quantified for dry mass of stalks, leaves, and total dry mass (entire plant), which were converted into kg ha−1.
Then, the same samples were crushed and grinded separately and subjected to analysis for the determination of P concentration, according to the methodology proposed by Malavolta et al. (1997). For subsequent calculation of this macronutrient accumulation in leaves, stalks s and total (entire plant) at the end of the cycle, in kg ha−1.
At the time of harvest (349 DAP), the stalks collected from all the treatment of five lines were weighed for the calculation of sugarcane yield (kg ha−1).
The soil available P content for each treatment in two layers (0–0.25 and 0.25–0.50 m) of the soil was determined after harvesting of sugarcane. The soil was collected from four different planting locations and forming a composite sample, which was subsequently sent to the laboratory for analysis according to the methodology described by van Raij et al. (2001).
Statistical Analysis
The results were submitted to analysis of variance (F test) and Scott-Knott test with probability (p ≤ 0.05) for means comparison of inoculation treatments. Regression equations were adjusted for the effect of P2O5 doses. The collected data were subjected to SISVAR statistical software for analysis interpretation and Sigma-Plot 12.5 software for plotting the graphics.
Results
Phosphorus Content in Soil and Cane Leaf
The overall average available soil P concentrations were 8.11 and 3.70 mg dm−3 in the layers of 0–0.25 and 0.25–0.50 m, respectively (Table 2) which were obviously higher than that of P content obtained in pre-experiment analysis (Table 1). There were significant interactions between phosphorus doses and inoculations with PGPB for available P content in the two soil layers analyzed (Table 2). The highest P available contents in both soil layers were observed with inoculation of A. brasilense + P. fluorescens at a dose of 135 kg P2O5 ha−1 of in the layer of 0–0.25m and A. brasilense inoculation with 180 kg P2O5 ha−1 in the 0.25–0.50 m layer (Table 3).
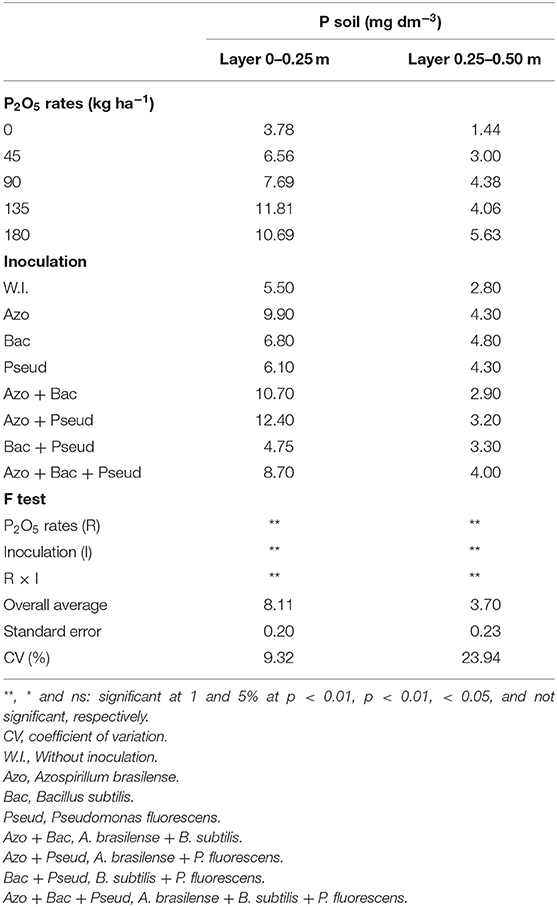
Table 2. Available P contents in soil after cane harvest as influenced by inoculation of PGPB and P doses in sugarcane.
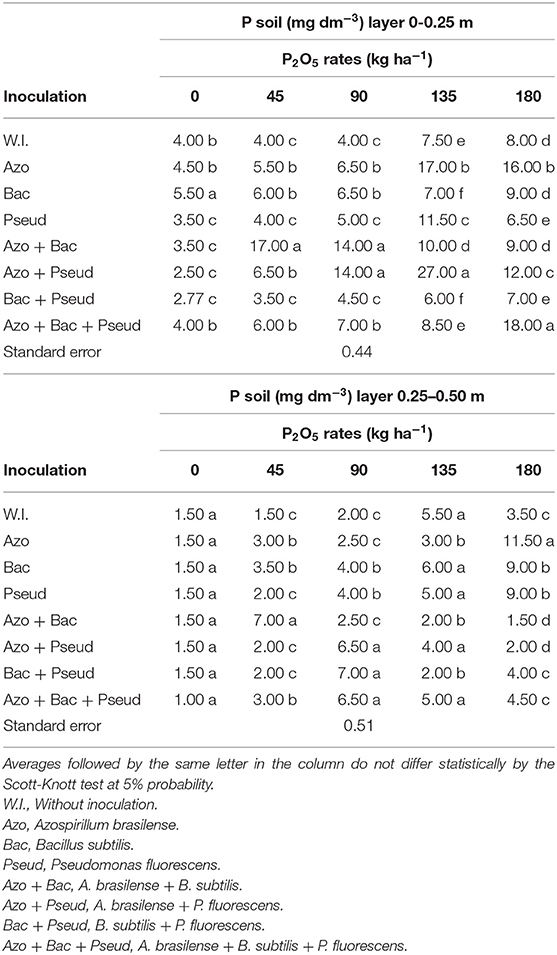
Table 3. Interaction between bacterial inoculations and P2O5 doses for soil available P in the layers of 0-0.25 m and 0.25-0.50 m.
In the 0–0.25 m soil layer, the highest P available contents were observed with lowest soil applied phosphate (45 kg P2O5 ha−1), which was statistically similar to that of the highest applied dose (180 kg P2O5 ha−1 of P2O5 dose commonly used by plant). However, in the 0–0.25 m soil layer, the combined inoculation of A. brasilense + B. subtilis resulted in highest available P contents with the lowest doses of phosphate applied fertilizer (45 and 90 kg ha−1 P2O5) (Figure 2A).
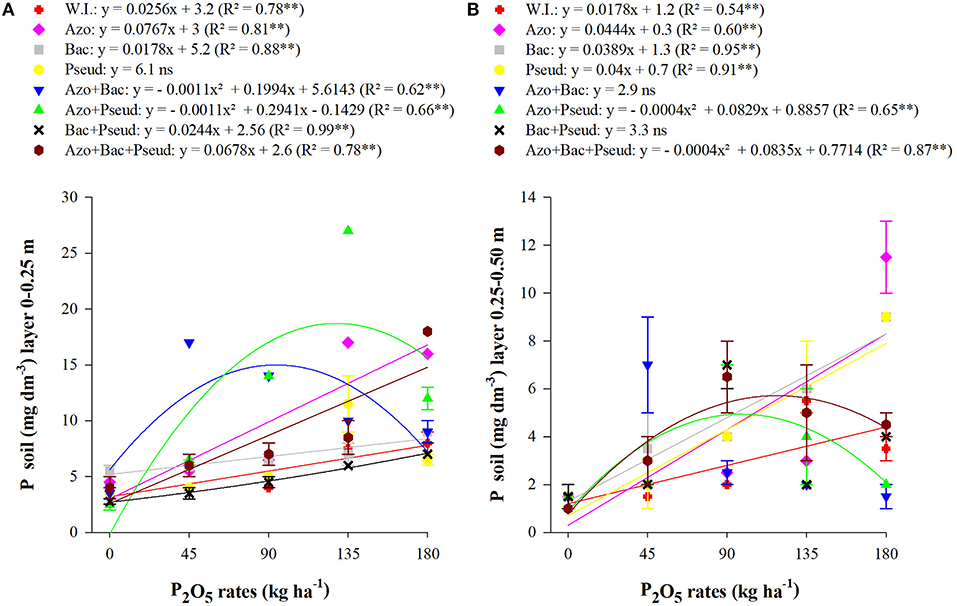
Figure 2. Interactions between bacterial inoculations and P2O5 doses for soil available P in the 0–0.25 m (A) and 0.25–0.50 m layers (B). (Mean values ± standard deviation). ** and ns: significant at 1% at p < 0.01 and not significant, respectively. W.I., without inoculation; Azo, Azospirillum brasilense; Bac, Bacillus subtilis; Pseud, Pseudomonas fluorescens; Azo + Bac, A. brasilense + B. subtilis; Azo + Pseud, A. brasilense + P. fluorescens; Bac + Pseud, B. subtilis + P. fluorescens; Azo + Bac + Pseud, A. brasilense + B. subtilis + P. fluorescens.
In case of interaction PGPB and phosphorus doses available P content in soil layer of 0–0.25 m, the graph trend indicated a linear increase for no-inoculation, A. brasilense, B. subtilis, B. subtilis + P. fluorescens, and A. brasilense + B. subtilis + P. fluorescens (Figure 2A). Quadratic regression for the treatments described that available P contents were improved with inoculation of A. brasilense + B. subtilis up to the dose of 91 kg P2O5 ha−1 and inoculation of A. brasilense + P. fluorescens up to the dose of 134 kg P2O5 ha−1. Similarly, the graph trend for 0.25–0.50 m soil layer indicated that there an increasing linear trend for no-inoculation, A. brasilense, B. subtilis, and P. fluorescens and quadratic adjustments for A. brasilense + P. fluorescens, and A. brasilense + B. subtilis + P. fluorescens up to the dose of 104 kg ha−1 P2O5 (Figure 2B). For leaf P concentration, there was a unique influence of inoculation on leaf P concentrations regardless of applied phosphorus doses. Inoculation with P. fluorescens and other bacteria in combination (except A. brasilense + P. fluorescens) resulted in higher cane leaf P concentration during nutrient analysis whereas the interaction between inoculations and phosphorus doses was non-significant (Table 4).
Dry Matter Partitioning
Different phosphorus (P) doses and bacterial inoculations significantly influenced leaf, stalk and total plant dry matter. Stalk and total dry mass were significantly increased with inoculation of A. brasilense + P. fluorescens and soil applied P doses (90 and 135 kg P2O5 ha−1) which were statistically similar. Leaf dry mass was also increased with A. brasilense + P. fluorescens inoculation P application at the rate of 135 kg P2O5 ha−1 (Table 6).
The inoculation of A. brasilense + B. subtilis combined with the dose of 45 kg P2O5 ha−1 increased the stalk and total dry mass in sugarcane in comparison to other inoculations at the same P dose (Table 6). Inoculation of B. subtilis + P. fluorescens resulted in maximum dry mass for different parts of cane plant in the absence of phosphate fertilizer (Table 6). In general, the influence of P doses (45 and 90 kg ha−1 P2O5) along with inoculation of PGPB were observed more prominent in dry weight of the sugarcane (Table 6).
The interactions between different P doses and bacterial inoculations for leaf, stalk and total dry mass were also found significant (Table 5). The graph trend for leaf dry mass set increasing linear functions in A. brasilense and A. brasilense + P. fluorescens inoculations and a decreasing linear function in inoculations of A. brasilense + B. subtilis and B. subtilis + P. fluorescens whereas the quadratic adjustment in un-inoculated treatments up to the dose of 105 kg P2O5 ha−1 (Figure 3A). Similarly, the stalks dry mass set a decreasing linear function for B. subtilis, P. fluorescens and B. subtilis + P. fluorescens (Figure 3B). The stalks dry mass was set to quadratic functions for A. brasilense and A. brasilense + P. fluorescens up to 120 and 113 kg P2O5 ha−1, respectively, whereas for no-inoculation up to the dose of 115 kg P2O5 ha−1. Hence, by utilizing the above P doses, the estimated stalk dry mass yield could be 60,709, 87,406, and 60,811 kg ha−1 respectively. Furthermore, the graph trend of total dry mass also set decreasing functions for B. subtilis, P. fluorescens and B. subtilis + P. fluorescens (Figure 3C). The graph trend of quadratic functions was set for A. brasilense up to P dose of 128 kg P2O5 ha−1, A. brasilense + P. fluorescens up to the dose of 122 kg P2O5 ha−1 and no-inoculation up to a dose of 111 kg P2O5 ha−1.
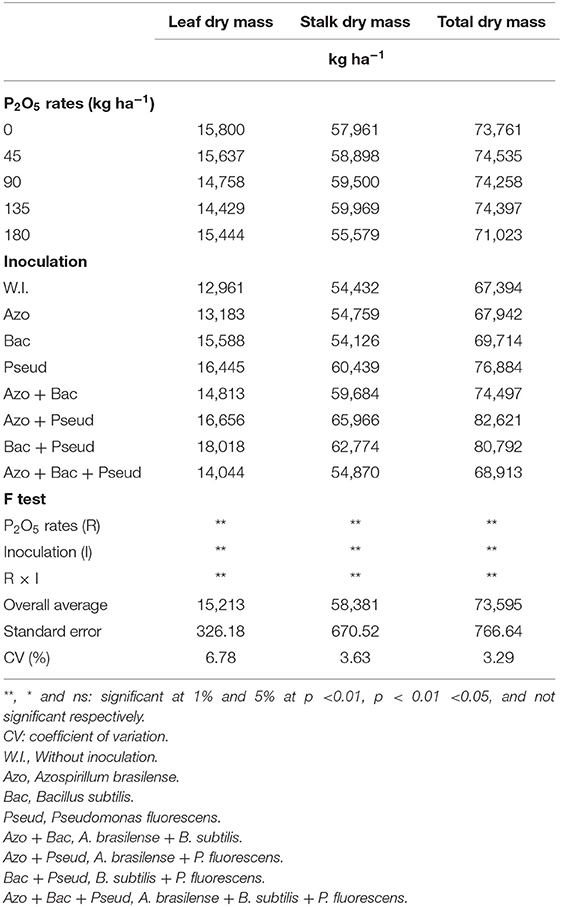
Table 5. Leaf dry mass, stalk dry mass and total dry mass (entire plant) of sugarcane as influenced by different bacterial inoculations and P2O5 doses.
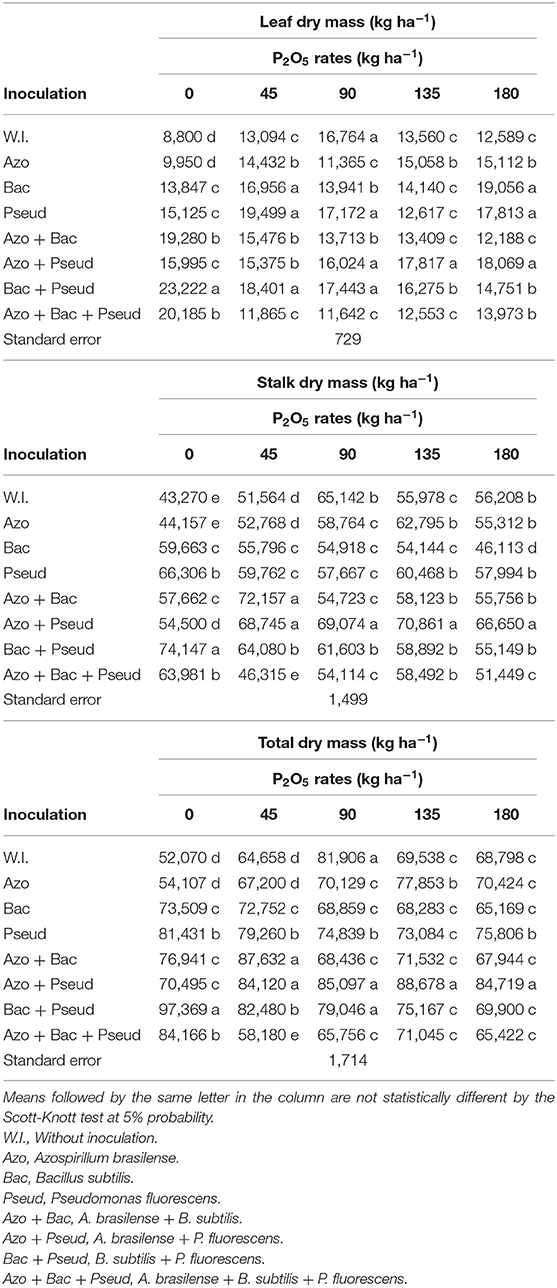
Table 6. Interactions between different bacterial inoculations and P2O5 doses for leaf dry mass, stalk dry mass and total dry mass in sugarcane crop.
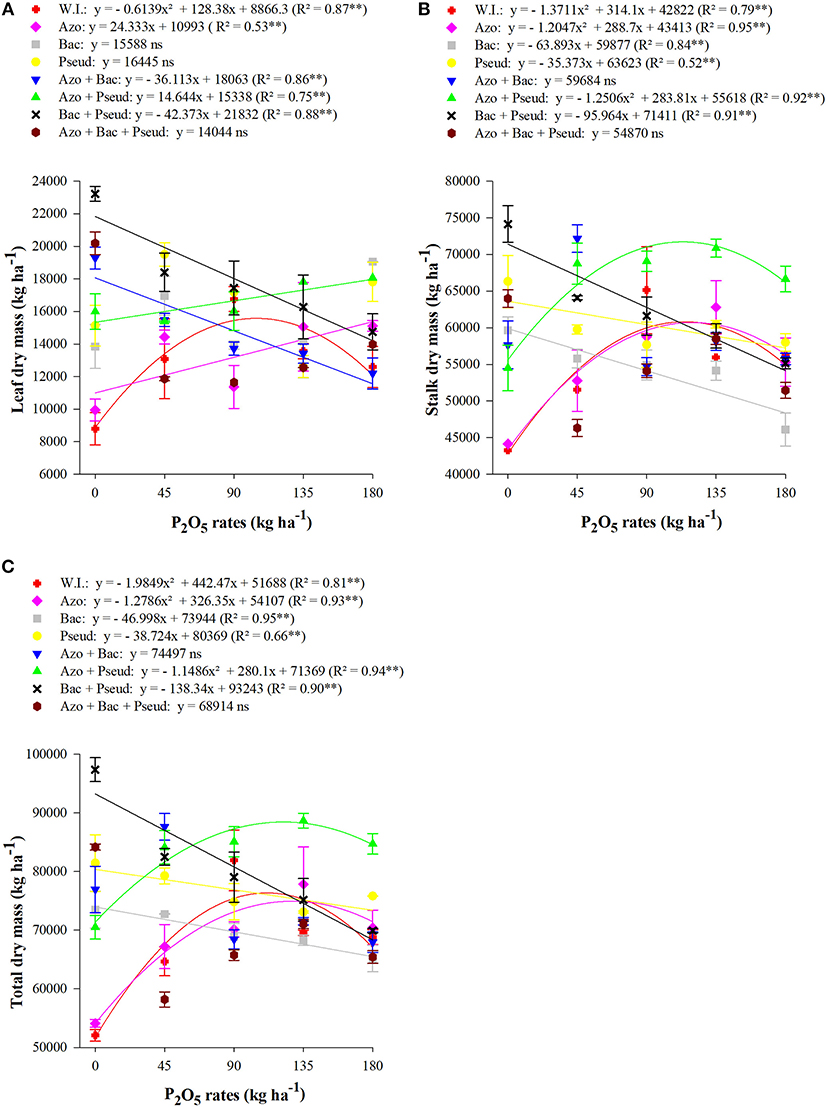
Figure 3. Interactions between different bacterial inoculations and P2O5 doses for leaf dry mass (A), stalk dry mass (B), and total dry mass (C) at the end of the plant-cane cycle, RB92579 variety (Mean values ± standard deviation). ** and ns: significant at 1% at p < 0.01 and not significant, respectively. W.I., without inoculation; Azo, Azospirillum brasilense; Bac, Bacillus subtilis; Pseud, Pseudomonas fluorescens; Azo + Bac, A. brasilense + B. subtilis; Azo + Pseud, A. brasilense + P. fluorescens; Bac + Pseud, B. subtilis + P. fluorescens; Azo + Bac + Pseud, A. brasilense + B. subtilis + P. fluorescens.
Phosphorus Accumulation in Leaf, Stalk, and Entire Plant
The leaf phosphorus (P) accumulation was enhanced with inoculation of B. subtilis + P. fluorescens with soil applied phosphate at the doses of 0.0, 45, 90, and 135 kg P2O5 ha−1.The inoculation of P. fluorescens with at 45 P2O5 kg ha−1 whereas A. brasilense + B. subtilis + P. fluorescens in the absence of phosphate fertilizer improved leaf P accumulation (Table 8). The highest P accumulation in leaves of cane variety (RB92579) was observed at a dose of 180 kg P2O5 ha−1 with inoculation of P. fluorescens. The interaction of inoculation and P doses was also found significant for P accumulation in the cane leaves (Table 7). The graph trend set a linear increment for P leaf accumulation with inoculation of A. brasilense, B. subtilis, A. brasilense + B. subtilis, and A. brasilense + P. fluorescens (Figure 4A) whereas non-inoculated treatments set to a quadratic function up to a dose of 105 kg P2O5 ha−1.
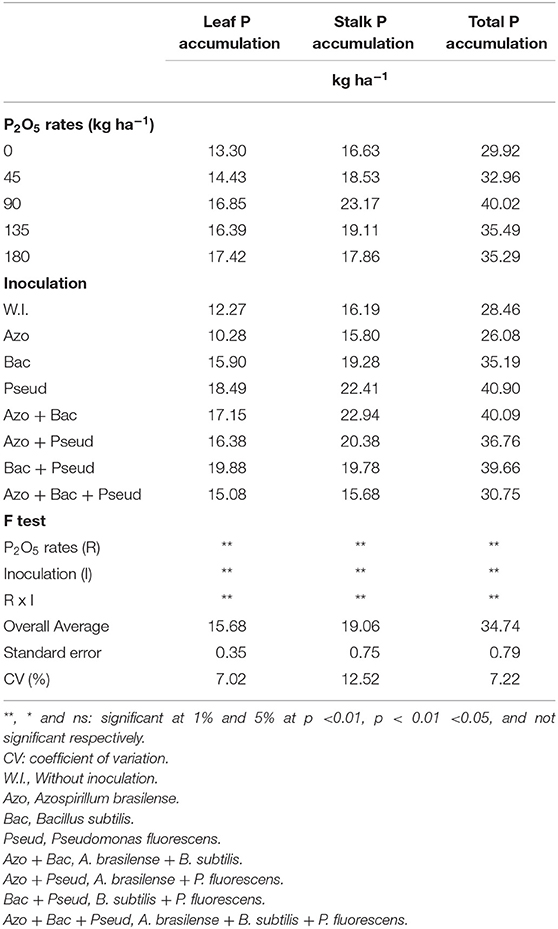
Table 7. Phosphorus (P) accumulation in leaf, stalk, and total plant as influenced by different bacterial inoculations and P2O5 doses in sugarcane crop.
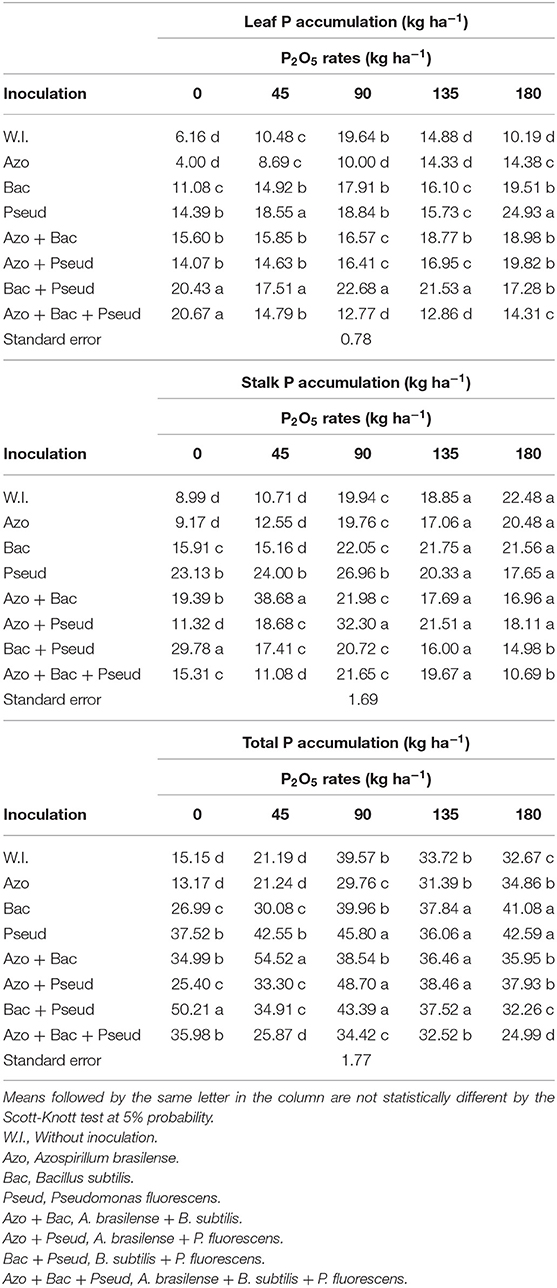
Table 8. Interactions between bacterial inoculations and P2O5 doses for leaf P accumulation, stalk P accumulation and total P accumulation in sugarcane crop.
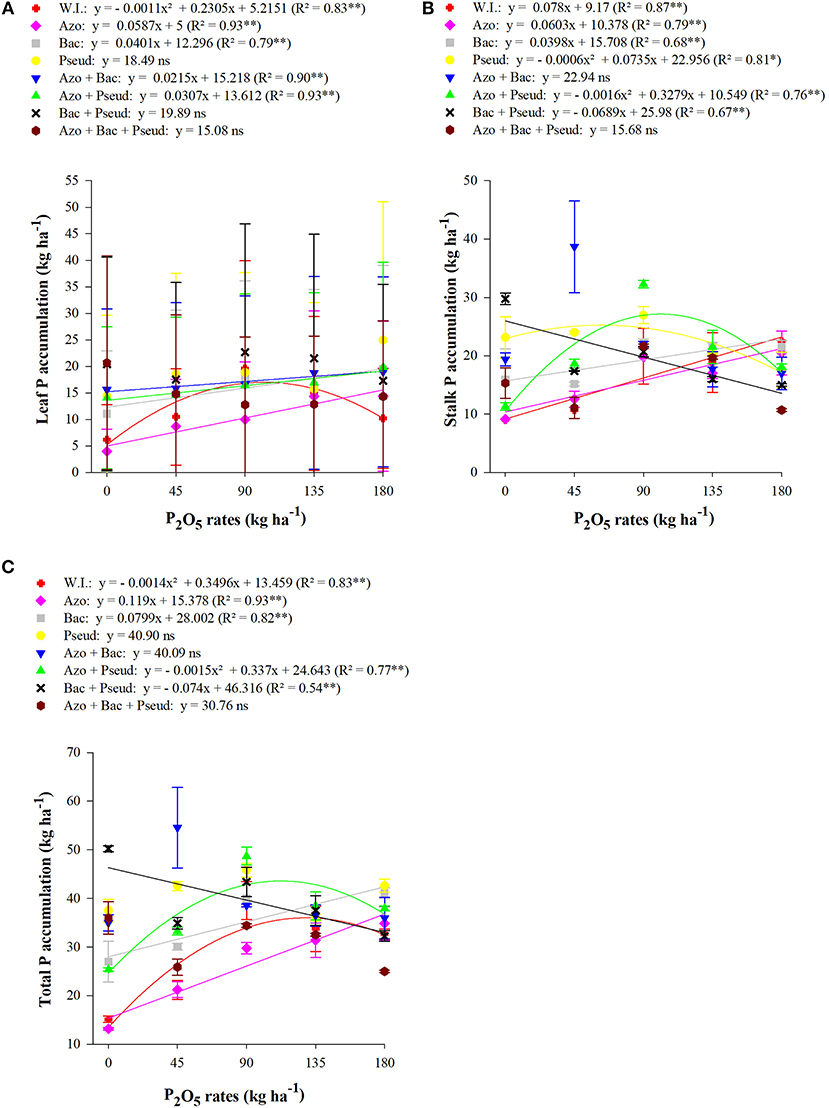
Figure 4. Interactions between different bacterial inoculations and P2O5 doses for leaf P accumulation (A), stalk P accumulation (B) and total P accumulation (C), at the end cycle of the plant-cane, RB92579 variety (Mean values ± standard deviation). **, * and ns: significant at 1 and 5% at p < 0.01, 0.01 < p < 0.05, and not significant, respectively. W.I., without inoculation; Azo, Azospirillum brasilense; Bac, Bacillus subtilis; Pseud, Pseudomonas fluorescens; Azo + Bac, A. brasilense + B. subtilis; Azo + Pseud, A. brasilense + P. fluorescens; Bac + Pseud, B. subtilis + P. fluorescens; Azo + Bac + Pseud, A. brasilense + B. subtilis + P. fluorescens.
The accumulation of P in the stalks of the sugarcane was significantly influenced by the higher doses of soil applied P (135 and 180 kg P2O5 ha−1) (Table 8). However, it was improved with inoculation of A. brasilense + P. fluorescens at a dose of 90 kg P2O5 ha−1 and B. subtilis + P. fluorescens inoculation without phosphorus application. The highest accumulation of P in the stalks was recorded with A. brasilense + B. subtilis inoculation in combination with a dose of 45 kg P2O5 ha−1. The interaction of inoculation and P doses was also found significant for P accumulation in the cane stalks (Table 7). The graph trend indicated that P content in stalks was improved and set a linear function for no-inoculation, A. brasilense and B. subtilis whereas inoculation of B. subtilis + P. fluorescens was set to a decreasing linear function (Figure 4B). Quadratic functions were set by inoculation with Pseud up to the dose of 61 kg ha−1 P2O5 and inoculation with A. brasilense + P. fluorescens up to the dose of 102 kg ha−1 P2O5.
The total P accumulation in the entire plant was also positively influenced by bacterial inoculations and phosphate doses. The inoculation of B. subtilis + P. fluorescens at 0 kg P2O5 ha−1 increased P total in cane plant in comparison to other treatments for the same P dose whereas the highest P total accumulation was observed with inoculation of A. brasilense + B. subtilis treated with 45 kg P2O5 ha−1 (Table 8). Similarly, total P accumulation was prominently influenced by all the inoculations at 135 kg P2O5 ha−1 in comparison to non-inoculated plots whereas inoculation of A. brasilense and triple (A. brasilense + B. subtilis + P. fluorescens) were statistically similar to that of control. The interaction of inoculation and P doses was also found significant for P accumulation in the cane stalks (Table 7). The total P accumulation in the entire plant set an increasing linear function for A. brasilense and B. subtilis inoculations and decreasing linear function for B. subtilis + P. fluorescens (Figure 4C). A. brasilense + P. fluorescens and no-inoculation were set up to quadratic functions for the doses of 112 and 125 kg P2O5 ha−1, respectively.
Sugarcane Yield
The cane yield was positively influenced by inoculations and phosphorus (P) doses (Table 9). Among P doses, the highest stalk yield was observed at 135 kg P2O5 ha−1 with inoculation of B. subtilis + P. fluorescens whereas P applied at the dose of 45 kg P2O5 ha−1 with inoculation of A. brasilense + B. subtilis also resulted in high stalk yield (Table 10). The lower and higher applied doses did not significantly influence stalk yield. P applied at the rates of 45, 90 and 135 kg ha−1 P2O5 with triple inoculation (A. brasilense + B. subtilis + P. fluorescens) resulted in statistically similar yield to control (no-inoculation) (Table 10).
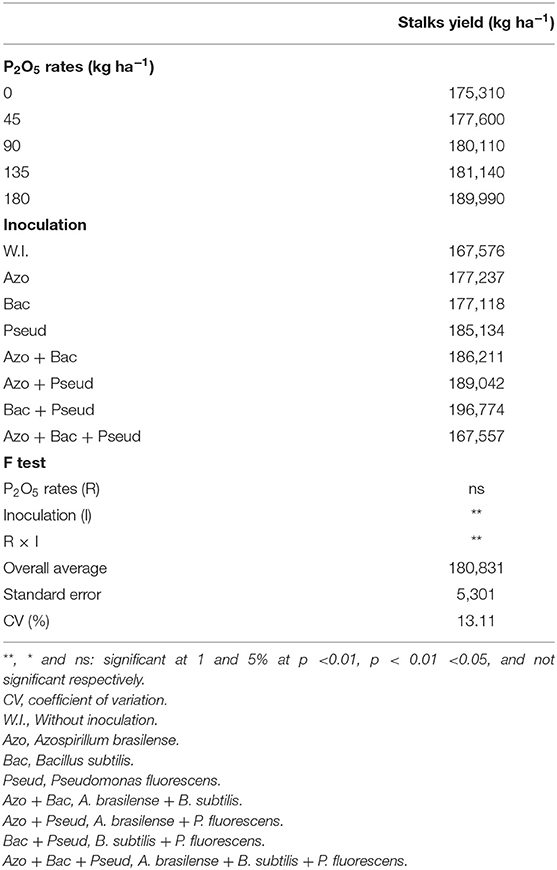
Table 9. Stalks yield of sugarcane as influenced by different bacterial inoculations and P2O5 doses.
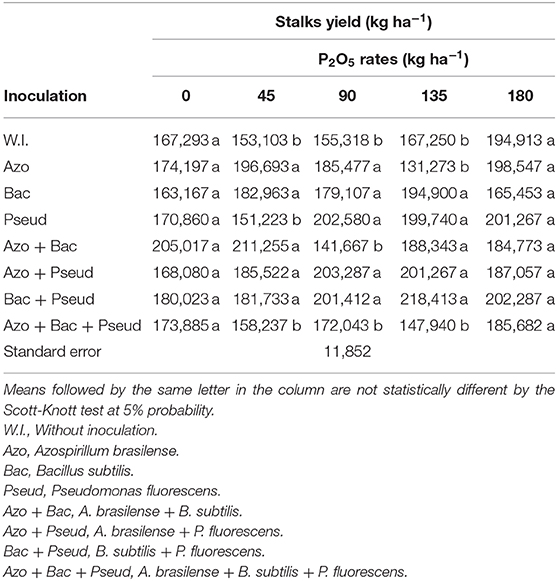
Table 10. Interactions between different bacterial inoculations and P2O5 doses for stalks yield in sugarcane crop.
Interaction between P2O5 doses and inoculation with PGPB was observed for the yield of sugarcane stalks (Table 9). The treatments with no-inoculation, P. fluorescens and B. subtilis + P. fluorescens inoculations set to increasing linear functions (Figure 5). The inoculation of B. subtilis up to a dose of 97 kg P2O5 ha−1 was set to quadratic functions, therefore using this dose, the estimated maximum stalk yield would be 188,502 kg ha−1. The inoculation of A. brasilense + P. fluorescens to the dose of 110 kg P2O5 ha−1 estimated with maximum stalk yield of 202,126 kg ha−1.
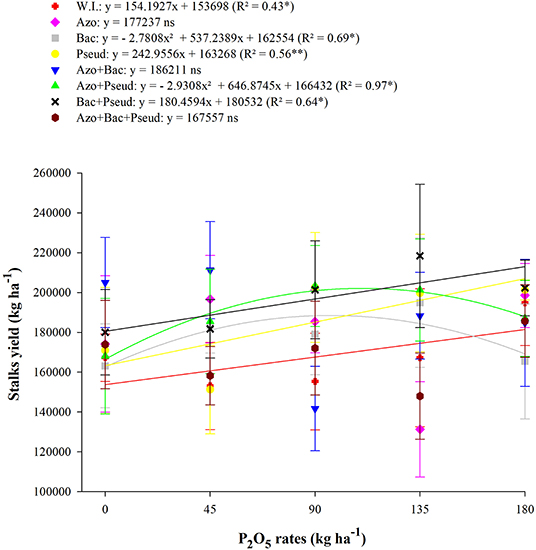
Figure 5. Interactions between different bacterial inoculations and P2O5 doses for stalks yield at the end of the plant-cane, RB92579 variety (Mean values ± standard deviation). **, * and ns: significant at 1 and 5% at p < 0.01, 0.01 < p < 0.05, and not significant, respectively. W.I., without inoculation; Azo, Azospirillum brasilense; Bac, Bacillus subtilis; Pseud, Pseudomonas fluorescens; Azo + Bac, A. brasilense + B. subtilis; Azo + Pseud, A. brasilense + P. fluorescens; Bac + Pseud, B. subtilis + P. fluorescens; Azo + Bac + Pseud, A. brasilense + B. subtilis + P. fluorescens.
Discussion
Phosphorus Content in Soil and Cane Leaf
Agricultural soil holds huge phosphorus reserves but all this phosphorus is not readily available to the plants, especially in acidic and highly weathered soils. In case of application of fertilizer containing phosphate can bind to Fe and Al oxides forming insoluble compounds. Phosphate in alkaline soils can bind with Ca and Mg making it unavailable for the plants to accumulate due to the high reactivity (Santos et al., 2012). Thus, a tiny fraction of P is available to plants for absorption.
The P available content in the soil of experimental area was improved with P fertilization however, there were no much differences among the un-inoculated treatments undergo different P doses (0, 45, and 90 kg P2O5 ha−1) (Table 3). The inoculation of PGPB with different P doses reflected higher variation for available P content. It might indicate that these microorganisms through their mechanisms of solubilizing inorganic P and making it available for the use of plants (Granada et al., 2018).
The soil layer of 0–0.25 m was analyzed after the inoculation of B. subtilis + A. brasilense along with application of 45 kg P2O5 ha−1 resulted in 17 mg dm−3 P. therefore, it was observed that this inoculation improved available P soil content more than four times in comparison of un-inoculated treatments. Thus, in both of soil depths, it is possible to observe that this inoculation increased the available soil P contents more than 4 times in comparison to control treatments. Caione et al. (2015) analyzed a Dystrophic Red Latosol soil submitted to the application of 360 kg P2O5 ha−1 from superphosphate and cultivated with sugarcane for six months cycle. It was reported that the P available content increased to 57 mg dm−3 in 0–0.20 m layer of soil. The P content can be vary depending on the amount of clay and organic matter present in each soil. The phosphorus dynamics in the soil is complex, especially in tropical soils. The lower availability of this nutrient in the soil may be due to the low content of organic matter in the soil. It is also known that soils with low clay content tend to have low levels of organic matter, and consequently, the available phosphorus content is altered by these soil attributes. Which also interfere with the activity of the enzyme phosphatase and microorganisms involved in the solubilization of unavailable phosphate.
Granada et al. (2018) utilized efficient P solubilizing bacterial strains that resulted in an average reduction of 33% P fertilization during the crop cycle. There must be considered an interaction between plant and bacteria, especially the relevance of these microorganisms with studied genotype. Lira-Cadete et al. (2012) studied three sugarcane varieties (RB92579, RB867515, and RB863129) and observed that RB92579 indicated positive interaction with PGPB (high phosphate solubilization rate in the laboratory). Borges et al. (2019) reported that the P use efficiency and dry matter production of sugarcane may increase in weathered soils when organic and inorganic P sources combinedly applied to the soil to meet the demand for this nutrient.
Leaf is the key to the development of the plant. Phosphorous plays an important role in the synthesis of different compounds of cells, phosphate-sugar catalyzes, photosynthesis and respiration, as well as help in the synthesis of cell membrane components (phospholipids), nucleotides (ATP), DNA and RNA (Taiz et al., 2017). Leaf analysis is one of the most commonly used methods to evaluate the demand of nutrients in sugarcane crop and contribution in fertilizers management (Moura Filho et al., 2014). The leaf P concentrations of all treatments (Table 4) are within the range (1.5–3.0 g kg−1), considered appropriate P content for the crop growth according to Raij and Cantarella (1997). Caione et al. (2015) studied P doses from different sources including triple superphosphate and indicated that cane leaf P content after 4 and 8 months of sprouting was significantly influenced by P doses. Hence, regardless of P sources, the obtained leaf P contents (1.4 to 1.8 g kg−1) were slightly lower than present research. Lima (2011) investigated an average P concentration of 1.7 g kg−1 in leaf analysis of sugarcane (variety RB867515) after five-month cycle. Cane variety (SP92 4221) was evaluated after 4 months of plantation resulted in foliar P contents ranging between 0.4 and 0.8 g kg−1 (Nobile et al., 2010). These differences may occur due to the characteristic of each variety and sampling time of each study.
Nobile et al. (2010) conducted a study with medium texture dystrophic Red Latossol soil and indicated that increasing P content in the soil enhances the absorption capacity of the plant and high P content was accumulated in leaf. The results of the present study were not inline to that of the previous studies and indicated that the P content in soil (Table 2) didn't correlate with the P content in leaf (Table 4). The treatments with higher levels of P in soil were not correlated to those with the highest concentration of P in leaf. The bacterial inoculation had differently functioned in the soil phosphate solubilization and hence, inoculation (B. subtilis + P. fluorescens) with the highest P content in leaf had the lowest content of P in soil. Here is the fact that combinations of certain microbes are activated and enabled in result of metabolites and phytohormones production that help in expanding the sugarcane root system and allowing them to achieve greater volume of soil and reach the more distant P, as it is a poorly mobile nutrient (Granada et al., 2018). Solubilization of soil fixed P through microbes making it available for increased plants absorption and its greater expression in leaf concentration.
The plants under soluble phosphate limiting conditions are when inoculated P. fluorescens fluorescens strains producing high amounts of gluconic acid and improving growth capacity due to uptake of solubilized P (Oteino et al., 2015). The primary mechanism for mineral P solubilization is the production of organic acids and acid phosphatases (Illmer and Schinner, 1995). Gluconic acid appears to be the most frequent acid in inorganic phosphate solubilization (Rodriguez et al., 2006). According to some authors, the inoculation with Bacillus sp. (Vardharajula et al., 2011) or A. brasilense (García et al., 2017) improved proline content in plants and make them more resistant to drought stress. The Arabidopsis plants when inoculated with A. brasilense resulted in the production of high level of abscisic acid. This abscisic acid positively influenced Arabidopsis growth by modulating their root architecture with lateral increment, stimulating photosynthetic pigments and reducing water loss through slow stomatal conductance during the drought stress (Cohen et al., 2015).
Dry Matter Partitioning
To satisfy the environmental, agronomic, social and economic issues, manual harvest of sugarcane crop with previous burning was replaced by mechanical harvesting and hereby the leaves and pointers (meristem / apex in development) are currently left on soil surface with amount of 10 to 20 tons ha−1 y−1 of dry biomass (Trivelin et al., 2013). The green sugarcane harvesting system in association with other precise farming equipment and management practices changed the nutritional requirements of the most modern sugarcane varieties due to large amount of straw remaining on the soil of cultivated area (Leite et al., 2016).
Leite et al. (2016) studied three varieties of sugarcane at three different locations and obtained an average leaf dry mass of 9,000 kg ha−1 and stalk dry mass of 37,000 kg ha−1. The present experiment resulted in more leaf dry mass of 15,213 kg ha−1 and stalk dry mass of 58,381 kg ha−1 (Table 5) which are far better than previous experiment. Calheiros et al. (2012) studied the same variety (RB92579) of the present study and found a mean shoot (leaves + stem) dry mass of 35,253 kg ha−1 however the present study resulted in an average shoot dry mass of 73,595 kg ha−1 (Table 5).
The combined dry mass of cane leaf and stalk resulted in maximum total dry mass (entire plant) and likewise happened in most of the inoculated treatments (Table 6). This might be due to the reason that these microorganisms utilize and adapt certain mechanisms which directly contribute and improve plant growth and yield. Inoculatin Vicia faba plants with phosphate solubilizing bacteria, Khalafallah et al. (1982) indicated that phosphorus fertilization was reduced by 50% with inoculation. The plants applied with half dose of fertilizer resulted in similar dry mass to that plant applied with 100% of fertilizers. The combined inoculation of B. subtilis + P. fluorescens with no phosphorus application increased leaf, stalk and total dry masses by 164, 71, and 87% (2.6, 1.7, and 1.9 times) respectively (Table 6). The combined inoculation may result in synergistic effect which had described in the present study for double inoculation. The double bacterial inoculation had distinctive influence than that of individual or triple inoculations in dry mass of sugarcane. Khan and Zaidi (2007) indicated that combine inoculation of Bacillus sp. and Azotobacter chroococcum with arbuscular mycorrhizal fungi (Glomus fasciculatum) significantly increased dry mass by about 2.6 times in comparison to control treatments (uninoculated).
Díaz-Zorita et al. (2012) reported that combine inoculation of A. brasilense with Sinorhizobium meliloti resulted in double dry mass of alfalfa pasture (94% increment). Park et al. (2015) investigated that the effect of volatile compounds produced by P. fluorescens in tobacco plants increased the dry mass up to 9.5 times. Tahir et al. (2017) documented that inoculation of Bacillus subtilis increased the dry mass of tomato by 3.3 times and root-shoot length by 1.4 times each. The authors also reported that the rate of photosynthetic and auxin contents was also enhanced which may promote and assist plant growth. Oliver and Silva (2018) conducted a research on the combined inoculation of PGPB (containing A. brasilense) with N doses in ratoon sugarcane variety (RB92579) and observed that stalk and total dry mass were negatively influenced by bacterial inoculation associated with a maximum N dose (120 kg N ha−1) However, the inoculation associated with intermediate doses of N (60 and 90 kg ha−1) increased stalk and total dry mass reflecting that higher N doses may inhibit the activities of the microorganisms. The same results were observed in the present study for phosphorus application in association with inoculations of B. subtilis and B. subtilis + P. fluorescens (Figures 3B,C).
Analyzing several studies published in the literature, Cassán and Díaz-Zorita (2016) claimed that maize inoculated with A. brasilense in rainfed regions of South America resulted in greater vegetative growth with higher shoot dry mass (8%). However, the same results for wheat and barley crops were reported under different environmental conditions. Although these authors also conducted two experiments at semi-arid and sub-humid regions on Rye crop inoculated with A. brasilense and reported that plant dry matter was increase by 13% (500 kg ha−1) in comparison of non-inoculated treatments. They also reported that such increase inn dry matter was only possible due to the association of microorganisms with N fertilizer and emphasizing the complementary need for both practices (inoculation and fertilization). Naiman et al. (2009) studied N with combined inoculation of A. brasilense or P. fluorescens fluorescens in wheat crop and observed that shoot dry mass did not increase with urea application however, it had positively influenced with bacterial inoculation. Santos et al. (2019) studied the rooting system and growth of pre-germinated seedlings in sugarcane variety (IACSP95-5000) inoculated with PGPB and reported that shoot dry biomass for all the tested strains including A. brasilense was improved.
Inoculation of A. brasilense + B. subtilis at the dose of 45 kg P2O5 ha−1 resulted an increment of 40 and 36% in the stalk and total dry mass, respectively, in comparison to non-inoculated (Table 6). The dry masses were also significantly increased with inoculation of A. brasilense + P. fluorescens in combination with 90 and 135 kg P2O5 ha−1. The increments in dry mass were 31% for leaf, 27% for stalk dry mass and 28% for total dry mass of the sugarcane variety RB92579. This might be due to the reason that plant hormone is responsible for cell division, stomatal conductance and may control the amount of the apical meristem and leaf chlorophyll content in the leaves. Azospirillum brasilense has a role in the synthesis of cytokines which had positive influence on vegetative growth of the plant (Vacheron et al., 2013). Bacillus subtilis under dry soil conditions producing cytokinin which may increase shoot dry mass in lettuce and decreased its root / shoot ratio (Arkhipova et al., 2007). The corn inoculated with B. subtilis and P. fluorescens resulting in production of indole acetic acid (IAA) which in turn increased shoot-root biomass along with water uptake and hereby, ensuring the survival and development of plants during drought (Marulanda et al., 2009).
Phosphorus Accumulation in Leaf, Stalk, and Entire Plant
The mean P accumulation observed at the end of sugarcane cycle in leaves, stalks and total (entire plant) was 15.68, 19.06, and 34.74 kg ha−1 respectively (Table 7). Leite et al. (2016) reported that mean P accumulation in shoot (stalks + leaves) was 32 kg ha−1 which is very similar to that found in the present study. Oliveira et al. (2010) reported that P accumulation in shoot of several sugarcane varieties is ranging from 19 and 30 kg ha−1. The range of P accumulation in shoot for the present study was 13.17 to 54.52 kg ha−1 (Table 8). In another study, Oliveira et al. (2010) studied a cane variety RB92579 and observed an average of 25 kg P ha−1 accumulation in shoots of sugarcane. Calheiros et al. (2012) also studied the same cane variety RB92579 for P accumulation in shoot dry mass and found an average P accumulation of 24.66 kg ha−1.
Caione et al. (2015) studied a cane variety CTC 15 under the application of triple superphosphate and observed that P accumulated in stalks was 25.4 kg ha−1 and straw (leaf + pointer) was 15.3 kg ha−1 which therefore, resulting in total P accumulation of 40.7 kg ha−1. Thus, it was observed that 38% of the plant P was accumulated in straw and 62% was accumulated in the stalks. It was also observed that 30% of P accumulated in the leaves of sugarcane (Trivelin et al., 2013). In case of present study, an average P accumulated in the leaves was 45% and in stalk was 55%.
The P accumulation in the sugarcane aerial parts was positively influenced by bacterial inoculations and P doses. Inoculation of B. subtilis + P. fluorescens had possible positive interaction with P doses (0, 45, 90, and 135 kg P2O5 ha−1) and improved leaf P accumulation. The leaf P accumulation was increased by 15 to 232% (1.2 to 3.3 times) when compared to non-inoculated (control) (Table 8). The same combination (B. subtilis + P. fluorescens) also increased P accumulation in the cane stalks with dose 1 kg P2O5 ha−1 which consequently resulted in the increment of total P accumulation (entire plant) by 231% (3.3 times) for both leaves and stalks in relation to non-inoculated treatment (Table 8). Inoculation of A. brasilense + P. fluorescens with the application of 90 kg P2O5 ha−1 increased P accumulation in the cane stalks by 62% (1.6 times) in comparison to non-inoculated (Table 8). A. brasilense + B. subtilis inoculation associated with 45 kg P2O5 ha−1 increased the P accumulation in the stalks by 261% (3.6 times) and the total P accumulation (entire plant) by 157% (2.6 times) in comparison to control (non-inoculated) (Table 8).
An interesting feature was evaluated that both stalk and total P accumulation was decreased with inoculation of B. subtilis + P. fluorescens and increasing doses of applied phosphate fertilizer (Figure 4B). It indicated that this combination of bacterial inoculation may solubilize the soil attached P because P applied from fertilizer wasn't available for the plant accumulation. As the supply of phosphorus via input increased, bacteria were stagnating P solubilization and may have been harmed by high P doses. The ecto-rhizospheric strains (colonize outside of the roots) and rhizobium endosymbiotic strains (colonize interior of the plants) of P. fluorescens and B. subtilis have been described as the most effective phosphate solubilizers among soil bacterial communities (Igual et al., 2001). Inoculation of PGPB with phosphate solubilization capability directly to the soil may increase P availability to the plants by 15 times in the soil (Seneviratne and Jayasinghearachchi, 2005).
Soil bacteria increase the solubility of calcium phosphate because majority of these microorganisms have the ability to secrete organic acids (carboxylic acid) which help in the reduction of rhizosphere pH by decoupling the connection between calcium and phosphate (Jayakumar et al., 2019). Solubilizing bacteria may also assist in solubilization of aluminum and iron-bound phosphate and release carboxylic acids which promote direct dissolution of Al and Fe bound P by chelating ions associated with phosphate through a linker exchange mechanism (Tomar, 1998).
Sugarcane Yield
Sugarcane yield was prominently improved by phosphorus doses and bacterial inoculation. Mean comparison indicated that stalk yield (180,831 kg ha−1) of cane variety (RB92579) was obtained with P doses (Table 9). Several studies have been conducted to highlight the role of phosphorous in improved production of sugarcane (Tsado et al., 2013; Caione et al., 2015). Albuquerque et al. (2016) described that application of P as triple superphosphate up to 100 kg P2O5 ha−1 in the furrow increases the yield of cane variety RB92579. Application of P in the furrow as triple superphosphate (at the rate of 100 kg P2O5 ha−1) increased the yield of sugarcane by 34% in comparison of control (Caione et al., 2013). Calheiros et al. (2012) performed experiment with same variety (RB92579) under different phosphate fertilizer and observed that greater stalk yield (133,000 kg ha−1) was recorded with application of 90 kg P2O5 ha−1. Stalk yield of 232,000 kg ha−1 was obtained with application of 180 kg P2O5 ha−1 in cane variety CTC 15 (Caione et al., 2015).
Inoculation of B. subtilis + P. fluorescens with application of 135 kg P2O5 ha−1 increased cane yield by 51,163 kg ha−1(31%) in comparison to non-inoculated treatments (Table 10). This might be due to the reason that this combination of bacteria and phosphorus also stood with highest leaf P concentration which possibly contribute to the most of cane yield for all treatments. Inoculation of different PGPB strains resulted an average stalk yield of 101,800 and 108,300 kg ha−1 for the sugarcane varieties RB72454 and RB867515 respectively (Schultz et al., 2014).
A. brasilense + B. subtilis in combination with 45 kg P2O5 ha−1 increased stalk yield by 58,152 kg ha−1 (38%). This combination of bacteria and P demonstrated that it is possible to decrease the amount of phosphate applied fertilizer. Certainly, this treatment resulted in highest stalk and total dry mass, highest stalk and total P accumulation for all treatments and also increase available P content in both soil layers (0–0.25 and 0.25–0.50 m) which in turn resulting into high stalk yield of sugarcane variety RB92579.
The activity of microorganisms may be harmful in combination with a high or with no dose of P. In case of present study, inoculation of PGPB with P doses of 0 and 180 kg P2O5 ha−1 were statistically similar to that of non-inoculated (Table 10). Oliver and Silva (2018) performed an experiment with certain diazotrophic bacteria including A. brasilense and observed that inoculation with combined application of N doses (60 and 90 kg N ha−1) resulted in higher stalk yield in sugarcane variety (RB92579). Oliveira et al. (2017) efficiently inoculated crops (mainly cereals) with A. brasilense and reduced fertilizer application which may not have any negative influence on crop yield. They indicated that inoculation with combination of N fertilizers in wheat crop had greatly influenced the yield which was not statistically different than without N fertilization. Azospirillum brasilense was applied to corn in furrow planting at a dose of 200 ml h−1 in Cerrado region of Brazil resulted in higher grain yield (Morais et al., 2016).
According to Naiman et al. (2009) application of 45 kg ha−1 N with inoculation of A. brasilense and P. fluorescens fluorescens to wheat seeds resulted in an increment of 9 and 10% in the yield of wheat grain, respectively, in comparison to uninoculated treatments. The authors also claimed that farmers could use PGPB to achieve the same increase in wheat yield as achieved with application of 45 kg N ha−1 of urea. Sahandi et al. (2019) performed two field experiments with peppermint (Mentha piperita L.) using phosphorus doses in association with inoculation of phosphate solubilizing bacteria (PSB). They reported that PSB inoculation increased the efficiency of phosphate fertilizers which leads to reduce the consumption of phosphate fertilizers and also increased plant biomass and yield of essential oils. Several studies demonstrated that crop seed inoculation with phosphate solubilizing microorganisms have the potential to reduce phosphate fertilizer application up to 50% without significant changes in yields (Jilani et al., 2007; Yazdani et al., 2009).
Hussain et al. (2013) investigated some strains of Bacillus sp. (PS-12) and P. fluorescens sp. (PS-32 and PS-51) and observed that corn grain yield was increased by 11, 42, and 33%, respectively, in comparison to non-inoculated treatments. Rudresh et al. (2005) reported that chickpea inoculated with Bacillus sp. improved grain yield by twice and also improved grain P concentration.
Lavakush et al. (2014) reported that rice raised in greenhouse inoculated with P solubilizing bacteria (A. brasilense and Pseudomonas spp.) potentially reduced P fertilization by 50%. The inoculated plants showed statistically similar performance in plant height, panicle length, grains panicle−1 and grain yield to the plants fertilized with 30 and 60 kg P2O5 ha−1. Similar results were observed in the present study, the stalk yield obtained with inoculation of P. fluorescens, A. brasilense + P. fluorescens, and B. subtilis + P. fluorescens and P dose (90 kg P2O5 ha−1) was statistically similar to the yield obtained at 180 kg P2O5 ha−1 without inoculation. It was demonstrated that this inoculation combination if applied in planting furrow of sugarcane variety RB92579 could save half of the phosphorus applied in the current study.
Hassan et al. (2010) indicated that Bacillus strains (B. subtillus NH-100 and Bacillus sp. NH-217) had antagonistic interaction with fungus Colletotrichum falcatum to control red rot from sugarcane. The antagonistic strains of Bacillus sp. stand relatively efficient than other biocontrol agents because of their excessive sporulation, prolonged life, improved plant nutrition and potential in controlling plant diseases (Hassan et al., 2010; Patel et al., 2019). Zarei et al. (2019) studied P. fluorescens strains and different irrigation levels in sweet corn. It was reported that P. fluorescens inoculation increased growth and grain yield as well-mitigated the adverse impact of drought stress. It was exhibited that these strains have the ability to solubilize phosphate, increased siderophores production and availability of nutrients such as P and Fe to plants. Schultz et al. (2014) studied sugarcane varieties with five strains of PGPB (including A. brasilense) and observed that the productivity of sugarcane variety RB72454 in the first cane plant and ratoon cane was improved with bacterial inoculation which was statistically similar to the yield obtained with 120 kg N ha−1. It is once again demonstrated that fertilizers could be reduced by utilization of PGPB inoculation.
According to Taulé et al. (2012), potentially N fixing bacteria were more effective in promoting sugarcane growth when they were also phosphate solubilizers and indole acetic acid producers. Phosphate solubilizing and indole acetic acid producing bacteria in sugarcane provided beneficial effect on plant-bacteria interaction (Beneduzi et al., 2013). The appearance of specific features of PGPB indicated that these organisms can promote plant growth by more than one mechanism. Thus, it is critical to characterize bacterial strains that adapted to sugarcane cultivation and are able to excrete substances that promote plant growth (Beneduzi et al., 2013). Hence, further field studies are needed which are carried out over long periods (five or six harvests) with existing strains to confirm whether or not the behavior obtained in this study, contribution of these bacteria in reducing application of phosphate fertilizers in sugarcane crop.
Conclusions
The bacterial inoculation and P doses prominently improved nutrient accumulation, dry matter and yield of sugarcane crop. Bacteria influenced P leaf concentration and higher values were obtained with B. subtilis + P. fluorescens inoculation. The inoculation with Azospirillum brasilense + Pseudomonas fluorescens at the dose 135 kg P2O5 ha−1 stood for the highest available P content in the 0–0.25 m soil layer.
The highest stalks yield, stalk and total dry matter, P accumulation in stalks and P total was obtained with inoculation of A. brasilense + B. subtilis allied to the dose of 45 kg P2O5 ha−1. In addition, the P content available in the soil surface layer after the plant-cane harvesting in this treatment was still within the P average range recommended by the literature, indicating that such a bacterial combination solubilized unavailable part of phosphate in the soil and allowing plant to use it. Thus, the combination of these two bacteria associated with such a dose is recommended in the sugarcane planting in soil with low available P with an increase of 38% in stalk yield and providing 75% of reduction in the phosphate fertilization, enabling the producer to minimize the production costs of sugarcane.
Data Availability Statement
The datasets generated for this study are available on request to the corresponding author.
Author Contributions
PR wrote the manuscript, with contributions from EM and AJ. MT, FG, AJ, and SB corrected and improved the manuscript. PR, EM, GF, and MB conducted the samplings and data collection. PR and EM did the analysis, with the support of MT, SB, FG, and PP.
Funding
This research was funded by CAPES (Coordination for the Improvement of Higher Education Personnel) and CNPq (National Council for Scientific and Technological Development), award number 312359/2017-9.
Conflict of Interest
The authors declare that the research was conducted in the absence of any commercial or financial relationships that could be construed as a potential conflict of interest.
References
Albuquerque, A. W., Sá, L. A., Rodrigues, W. A. R., Moura, A. B., and Oliveira Filho, M. S. (2016). Growth and yield of sugarcane as a function of phosphorus doses and forms of application. Rev. Bras. Eng. Agríc. Ambient. 20, 29–35. doi: 10.1590/1807-1929/agriambi.v20n1p29-35
Ali, S., Charles, T. C., and Glick, B. R. (2014). Amelioration of high salinity stress damage by plant growth-promoting bacterial endophytes that contain ACC deaminase. Plant Physiol. Biochem. 80, 160–167. doi: 10.1016/j.plaphy.2014.04.003
Arkhipova, T. N., Prinsen, E., Veselov, S. U., Martinenko, E. V., Melentiev, A. I., and Kudoyarova, G. R. (2007). Cytokinin producing bacteria enhance plant growth in drying soil. Plant Soil 292, 305–315. doi: 10.1007/s11104-007-9233-5
Beneduzi, A., Moreira, F., Costa, P. B., Vargas, L. K., Lisboa, B. B., Favareto, R., et al. (2013). Diversity and plant growth promoting evaluation abilities of bacteria isolated from sugarcane cultivated in the South of Brazil. Appl. Soil Ecol. 63, 94–104. doi: 10.1016/j.apsoil.2012.08.010
Borges, B. M. M. N., Abdala, D. B., Souza, M. F., Viglio, L. M., Coelho, M. J. A., Pavinato, P. S., et al. (2019). Organomineral phosphate fertilizer from sugarcane byproduct and its effects on soil phosphorus availability and sugarcane yield. Geoderma 339, 20–30. doi: 10.1016/j.geoderma.2018.12.036
Caione, G., Fernandes, F. M., and Lange, A. (2013). Residual effect of phosphorus sources in soil chemical properties, nutrition and biomass productivity of sugarcane. Rev. Bras. Ciênc. Agrár. 8, 189–196. doi: 10.5039/agraria.v8i2a2016
Caione, G., Prado, R. M., Campos, C. N. S., Moda, L. R., Vasconcelos, R. L., and Pizauro Júnior, J. M. (2015). Response of sugarcane in a red ultisol to phosphorus rates, phosphorus sources, and filter cake. Sci. World J. 2015, 1–10. doi: 10.1155/2015/405970
Calheiros, A. S., Oliveira, M. W., Ferreira, V. M., Barbosa, G. V. S., Santiago, A. D., and Santos Aristides, E. V. (2012). Production of biomass, from sugar and protein in function of sugarcane varieties and phosphorous fertilization. Semina Ciênc. Agrár. 33, 809–817. doi: 10.5433/1679-0359.2012v33n2p809
Campos, P., Borie, F., Cornejo, P., López-Ráez, J. A., López-García, A., and Seguel, A. (2018). Phosphorus acquisition efficiency related to root traits: is mycorrhizal symbiosis a key factor to wheat and barley cropping? Front. Plant Sci. 9:752. doi: 10.3389/fpls.2018.00752
Cassán, F., and Díaz-Zorita, M. (2016). Azospirillum sp. in current agriculture: From the laboratory to the field. Soil Biol. Biochem. 103, 117–130. doi: 10.1016/j.soilbio.2016.08.020
Cohen, A. C., Bottini, R., Pontin, M., Berli, F. J., Moreno, D., Boccanlandro, H., et al. (2015). Azospirillum brasilense ameliorates the response of Arabidopsis thaliana to drought mainly via enhancement of aba levels. Physiol. Plant. 153, 79–90. doi: 10.1111/ppl.12221
CONAB (2019). Monitoring of the Brazilian Crop: Sugarcane, First Survey: 2018/19 Crop. Portuguese. Available online at: https://www.conab.gov.br (accessed October 02, 2019).
Díaz-Zorita, M., Baliña, R., and Canigia, M. F. (2012). “Azospirillum brasilense enhances alfalfa productivity: field evaluation,” in 9th International and 1st Latinamerican PGPR Workshop. Quirama (Medellín), 3–8.
Duca, D., Lorv, J., Patten, C. L., Rose, D., and Glick, B. R. (2014). Microbial indole-3-acetic acid and plant growth. J. Microbiol. 106, 85–125. doi: 10.1007/s10482-013-0095-y
Galindo, F. S., Teixeira Filho, M. C. M., Buzetti, S., Santini, J. M. K., Alves, C. J., Nogueira, L. M., et al. (2016). Corn yield and foliar diagnosis affected by nitrogen fertilization and inoculation with Azospirillum brasilense. Rev. Bras. Cienc. Solo 40:e0150364. doi: 10.1590/18069657rbcs20150364
García, J. E., Maroniche, G., Creus, C., Suárez-Rodríguez, R., Ramirez-Trujillo, J. A., and Groppa, M. D. (2017). In vitro PGPR properties and osmotic tolerance of different Azospirillum native strains and their effects on growth of maize under drought stress. Microbiol. Res. 202, 21–29. doi: 10.1016/j.micres.2017.04.007
Granada, C. E., Passaglia, L. M. P., Souza, E. M., and Sperotto, R. A. (2018). Is phosphate solubilization the forgotten child of plant growth-promoting rhizobacteria? Front. Microbiol. 9:2054. doi: 10.3389/fmicb.2018.02054
Gupta, G., Parihar, S. S., Ahirwar, N. K., Snehi, S. K., and Singh, V. (2015). Plant growth promoting rhizobacteria (PGPR): current and future prospects for development of sustainable agriculture. J. Biochem. Technol. 7, 96–102. doi: 10.4172/1948-5948.1000188
Hassan, M. N., Osborn, A. M., and Hafeez, F. Y. (2010). Molecular and biochemical characterization of surfactin producing Bacillus species antagonistic to Colletotrichum falcatum Went causing sugarcane red rot. Afr. J. Microbiol. Res. 4, 2137–2142.
Hinsinger, P., Brauman, A., Devau, N., Gérard, F., Jourdan, C., Laclau, J. P., et al. (2011). Acquisition of phosphorus and other poorly mobile nutrients by roots. Where do plant nutrition models fail? Plant Soil 348:29. doi: 10.1007/s11104-011-0903-y
Hussain, M. I., Asghar, H. N., Akhtar, M. J., and Arshad, M. (2013). Impact of phosphate solubilising bacteria on growth and yield of maize. Soil Environ. 32, 71–78. doi: 10.1007/s11104-015-2588-0
Igual, J., Valverde, A., Cervantes, E., and Velázquez, E. (2001). Phosphate-solubilizing bacteria as inoculants for agriculture: use of updated molecular techniques in their study. Agron. Sustain. Dev. 21, 561–568. doi: 10.1051/agro:2001145
Illmer, P., and Schinner, F. (1995). Phosphate solubilizing microorganisms under non-sterile conditions. Bodenkultur 46, 197–204.
Jayakumar, N., Paulraj, P., Sajeesh, P., Sajna, K., and Zinneera, A. (2019). Application of native phosphate solubilizing bacteria for the use of cheap organic and inorganic phosphate source in agricultural practise of Capsicum annum (Chili)-A pilot scale field study. Mater. Today Proc. 16, 1630–1639. doi: 10.1016/j.matpr.2019.06.028
Jilani, G., Akram, A., Ali, R. M., Hafeez, F. Y., Shams, I. H., Chaudhry, A. N., et al. (2007). Enhancing crop growth, nutrients availability, economics and beneficial rhizosphere microflora through organic and biofertilizers. Ann. Microbiol. 57, 177–183. doi: 10.1007/BF03175204
Jorquera, M., Martínez, O. S. C. A. R., Maruyama, F., Marschner, P., and Mora, M. L. L. (2008). Current and future biotechnological applications of bacterial phytases and phytase-producing bacteria. Microbes Environ. 23, 182–191. doi: 10.1264/jsme2.23.182
Khalafallah, M. A., Saber, M. S. M., and Abd-El-Maksoud, H. K. (1982). Influence of phosphate dissolving bacteria on the efficiency of superphosphate in a calcareous soil cultivated with Vicia faba. Z. Pflanzenernachr. Bodenk. 145, 455–459. doi: 10.1002/jpln.19821450505
Khan, M. S., and Zaidi, A. (2007). Synergistic effects of the inoculation with plant growth-promoting rhizobacteria and an arbuscular mycorrhizal fungus on the performance of wheat. Turk. J. Agric. For. 31, 355–362. doi: 10.1002/jpln.200620602
Kumar, A., Maurya, B. R., and Raghuwanshi, R. (2014). Isolation and characterization of PGPR and their effect on growth, yield and nutrient content in wheat (Triticum aestivum L.). Biocatal. Agric. Biotechnol. 3, 121–128. doi: 10.1016/j.bcab.2014.08.003
Lavakush, Y. J., Verma, J. P., Jaiswal, D. K., and Kumar, A. (2014). Evaluation of PGPR and different concentration of phosphorus level on plant growth, yield and nutrient content of rice (Oryza sativa). Ecol. Eng. 62, 123–128. doi: 10.1016/j.ecoleng.2013.10.013
Leite, J. M., Ciampitti, I. A., Mariano, E., Vieira-Megda, M. X., and Trivelin, P. C. O. (2016). Nutrient partitioning and stoichiometry in unburnt sugarcane ratoon at varying yield levels. Front. Plant Sci. 7:466. doi: 10.3389/fpls.2016.00466
Li, H. B., Singh, R. K., Singh, P., Song, Q. Q., Xing, Y. X., Yang, L. T., et al. (2017). Genetic diversity of nitrogen-fixing and plant growth promoting Pseudomonas species isolated from sugarcane rhizosphere. Front. Microbiol. 8:1268. doi: 10.3389/fmicb.2017.01268
Lima, C. C. (2011). Availability of phosphorus for sugarcane in soil treated with silicon rich organic composts. Rev. Bras. Eng. Agríc. Ambient. 15, 1222–1227. doi: 10.1590/S1415-43662011001200002
Lira-Cadete, L., Farias, A. R. B., Ramos, A. P. S., Costa, D. P., Freire, F. J., and Kuklinsky-Sobral, J. (2012). Genetic variability of sugarcane-associated diazotrophic bacteria capable of inorganic phosphate solubilizing. Biosci. J. 28, 122–129.
Malavolta, E., Vitti, G. C., and Oliveira, S. A. (1997). Evaluation of Plant Nutritional Status: Principles and Applications. Piracicaba: Potafos.
Marulanda, A., Barea, J. M., and Azcón, R. (2009). Stimulation of plant growth and drought tolerance by native microorganisms (AM fungi and bacteria) from dry environments: mechanisms related to bacterial effectiveness. J. Plant Growth Regul. 28, 115–124. doi: 10.1007/s00344-009-9079-6
Morais, T. P., Brito, C. H., Brandão, A. M., and Rezende, W. S. (2016). Inoculation of maize with Azospirillum brasilense in the seed furrow. Rev. Cienc. Agron. 47, 290–298. doi: 10.5935/1806-6690.20160034
Moura Filho, G., Albuquerque, A. W. D., Moura, A. B., Santos, A. C., Oliveira Filho, M. D. S., and Silva, L. C. D. (2014). Nutritional diagnosis and yield of sugarcane varieties in Urtisols. Rev. Bras. Eng. Agríc. Ambient. 18, 1102–1109. doi: 10.1590/1807-1929/agriambi.v18n11p1102-1109
Naiman, A. D., Latrónico, A., and Salamone, I. E. G. (2009). Inoculation of wheat with Azospirillum brasilense and Pseudomonas fluorescens: impact on the production and culturable rhizosphere microflora. Eur. J. Soil Biol. 45, 44–51. doi: 10.1016/j.ejsobi.2008.11.001
Nobile, F. O., Galbiatti, J. A., Muraishi, R., Ribeiro, A. G., and Freddi, O. S. (2010). Nutrient diagnosis of sugarcane in function of residue rates of the bauxite mining. Eng. Agric. 30, 168–178. doi: 10.1590/S0100-69162010000100018
Oliveira, A. L. M., Santos, O. J. A. P., Marcelino, P. R. F., Milani, K. M. L., Zuluaga, M. Y. A., Zucareli, C., et al. (2017). Maize inoculation with Azospirillum brasilense Ab-V5 cells enriched with exopolysaccharides and polyhydroxybutyrate results in high productivity under low N fertilizer input. Front. Microbiol. 8:1873. doi: 10.3389/fmicb.2017.01873
Oliveira, E., Freire, F. J., Oliveira, R., Freire, M., Simões Neto, D. E., and Silva, S. (2010). Nutrient extraction and export by fully irrigated sugarcane varieties. Rev. Bras. Cienc. Solo 34, 1343–1352. doi: 10.1590/S0100-06832010000400031
Oliver, R., and Silva, M. A. (2018). Interaction between diazotrophic bacteria and N-fertilizer doses on sugarcane crop. J. Plant Nutr. 41, 722–736. doi: 10.1080/01904167.2018.1425436
Oteino, N., Lally, R. D., Kiwanuka, S., Lloyd, A., Ryan, D., Germaine, K. J., et al. (2015). Plant growth promotion induced by phosphate solubilizing endophytic Pseudomonas isolates. Front. Microbiol. 6:745. doi: 10.3389/fmicb.2015.00745
Park, Y. S., Dutta, S., Ann, M., Raaijmakers, J. M., and Park, K. (2015). Promotion of plant growth by Pseudomonas fluorescens strain SS101 via novel volatile organic compounds. Biochem. Biophys. Res. Comum. 461, 361–365. doi: 10.1016/j.bbrc.2015.04.039
Patel, J. K., and Archana, G. (2017). Diverse culturable diazotrophic endophytic bacteria from Poaceae plants show cross-colonization and plant growth promotion in wheat. Plant Soil 417, 99–116. doi: 10.1007/s11104-017-3244-7
Patel, P., Shah, R., Joshi, B., Ramar, K., and Natarajan, A. (2019). Molecular identification and biocontrol activity of sugarcane rhizosphere bacteria against red rot pathogen Colletotrichum falcatum. Rep. Biotechnol. 21:e00317. doi: 10.1016/j.btre.2019.e00317
Raij, B. V. (2011). Soil Fertility and Nutrient Management. Piracicabab: International Plant Nutrition Institute.
Raij, B. V., and Cantarella, H. (1997). “Other industrial crops: sugarcane,” in: Technical Bulletin 100: Liming and Fertilizing Recommendations for the State of São Paulo, eds B. V. Raij, H. Cantarella, J. A. Quaggio, and A. M. C. Furlani (Campinas: IAC), 233–239.
RFA (2019). Renewable Fuels Association: Annual World Fuel Ethanol Production. Available online at: https://ethanolrfa.org/statistics/annual-ethanol-production/ (accessed October 02, 2019).
Rodriguez, H., Fraga, R., Gonzalez, T., and Bashan, Y. (2006). Genetics of phosphate solubilisation and its potential applications for improving plant growth-promoting bacteria. Plant Soil 287, 15–21. doi: 10.1007/s11104-006-9056-9
Rudresh, D. L., Shivaprakash, M. K., and Prasad, R. D. (2005). Effect of combined application of Rhizobium, phosphate solubilizing bacterium and Trichoderma spp. on growth, nutrient uptake and yield of chickpea (Cicer aritenium L.). Appl. Soil Ecol. 28, 139–146. doi: 10.1016/j.apsoil.2004.07.005
Sahandi, M. S., Mehrafarin, A., Badi, H. N., Khalighi-Sigaroodi, F., and Sharifi, M. (2019). Improving growth, phytochemical, and antioxidant characteristics of peppermint by phosphate-solubilizing bacteria along with reducing phosphorus fertilizer use. Ind. Crops Prod. 141:111777. doi: 10.1016/j.indcrop.2019.111777
Sampaio, M. S. (2011). “Evaluation of the Spatial Variability Behavior of the Remaining Phosphorus in Coffee Crop Soil,” in 15th Brazilian Symposium on Remote Sensing (Curitiba), 9203–9210.
Santos, H. G., Jacomine, P. K. T., Anjos, L. H. C., Oliveira, V. A., Lumbreras, J. F., Coelho, M. R., et al. (2018). Brazilian System of Soil Classification. Brasília: Embrapa Solos.
Santos, S. G., Chaves, V. A., Ribeiro, F. S., Alves, G. C., and Reis, V. M. (2019). Rooting and growth of pre-germinated sugarcane seedlings inoculated with diazotrophic bacteria. Appl. Soil Ecol. 133, 12–23. doi: 10.1016/j.apsoil.2018.08.015
Santos, I. B., Lima, D. R. M., Barbosa, J. G., Oliveira, J. T. C., Freire, F. J., and Kuklinsky-Sobral, J. (2012). Diazotrophic bacteria associated to roots of sugarcane: inorganic phosphate solubilization and the salinity tolerance. Biosci. J. 28, 142–149.
Sattari, S. Z., Bouwman, A. F., Giller, K. E., and van Ittersum, M. K. (2012). Residual soil phosphorus as the missing piece in the global phosphorus crisis puzzle. Proc. Natl. Acad. Sci.U.S.A. 109, 6348–6353. doi: 10.1073/pnas.1113675109
Schultz, N., Silva, J. A. D., Sousa, J. S., Monteiro, R. C., Oliveira, R. P., Chaves, V. A., et al. (2014). Inoculation of sugarcane with diazotrophic bacteria. Rev. Bras. Cienc. Solo 38, 407–414. doi: 10.1590/S0100-06832014000200005
Seneviratne, G., and Jayasinghearachchi, H. S. (2005). A rhizobial biofilm with nitrogenase activity alters nutrient availability in a soil. Soil Biol. Biochem. 37, 1975–1978. doi: 10.1016/j.soilbio.2005.02.027
Shen, H., He, X., Liu, Y., Chen, Y., Tang, J, and Guo, T. (2016). A complex inoculant of N2-fixing, P- and K-solubilizing bacteria from a purple soil improves the growth of kiwifruit (Actinidia chinensis) plantlets. Front. Microbiol. 7:841. doi: 10.3389/fmicb.2016.00841
Smith, D. L., Subramanian, S., Lamont, J. R., and Bywater-Ekegärd, M. (2015). Signaling in the phytomicrobiome: breadth and potential. Front. Plant Sci. 6:709. doi: 10.3389/fpls.2015.00709
Soil Survey Staff (2014). Keys to Soil Taxonomy. Washington, DC: USDA and Natural Resources Conservation Service.
Spolaor, L. T., Gonçalves, L. S. A., Santos, O. J. A. P. D., Oliveira, A. L. M. D., Scapim, C. A., Bertagna, F. A. B., et al. (2016). Plant growth-promoting bacteria associated with nitrogen fertilization at topdressing in popcorn agronomic performance. Bragantia 75, 33–40. doi: 10.1590/1678-4499.330
Syers, J. K., Johnston, A. E., and Curtin, D. (2008). Efficiency of Soil and Fertilizer Phosphorus Use. Rome: FAO Fertilizer and Plant Nutrition Bulletin.
Tahir, H. A., Gu, Q., Wu, H., Raza, W., Hanif, A., Wu, L., et al. (2017). Plant growth promotion by volatile organic compounds produced by Bacillus subtilis SYST2. Front. Microbiol. 8:171. doi: 10.3389/fmicb.2017.00171
Taiz, L., Zeiger, E., Møller, I. M., and Murphy, A. (2017). Plant Physiology and Development. Porto Alegre: Artmed.
Takishita, Y., Charron, J. B., and Smith, D. L. (2018). Biocontrol rhizobacterium Pseudomonas sp. 23S induces systemic resistance in tomato (Solanum lycopersicum L.) against bacterial canker Clavibacter michiganensis subsp. michiganensis. Front. Microbiol. 9:2119. doi: 10.3389/fmicb.2018.02119
Taulé, C., Mareque, C., Barlocco, C., Hackembruch, F., Reis, V. M., Sicardi, M., et al. (2012). The contribution of nitrogen fixation to sugarcane (Saccharum officinarum L.), and the identification and characterization of part of the associated diazotrophic bacterial community. Plant Soil 356, 35–49. doi: 10.1007/s11104-011-1023-4
Tomar, R. (1998). Effect of phosphate-solubilizing bacteria and farmyard manure on the yield of blackgram (Phaseolus mungo). Indian J. Agr. Sci. 68, 81–83.
Trivelin, P. C. O., Franco, H. C. J., Otto, R., Ferreira, D. A., Vitti, A. C., Fortes, C., et al. (2013). Impact of sugarcane trash on fertilizer requirements for São Paulo, Brazil. Sci. Agric. 70, 345–352. doi: 10.1590/S0103-90162013000500009
Tsado, P. A., Lawal, B. A., Igwe, C. A., Adeboye, M. K. A., Odofin, A. J., and Adekambi, A. A. (2013). Effects of sources and levels of phosphorus on yield and quality of sugarcane in Southern Guinea Savanna Zone of Nigeria. Agric. Sci. Dev. 2, 25–27.
USDA (2019). Sugar: World Markets and Trade. Foreign Agricultural Service. Available online at: https://apps.fas.usda.gov/psdonline/circulars/sugar.pdf (accessed October 02, 2019).
Vacheron, J., Desbrosses, G., Bouffaud, M. L., Touraine, B., Moënne-Loccoz, Y., Muller, D., et al. (2013). Plant growth-promoting rhizobacteria and root system functioning. Front. Plant Sci. 4:356. doi: 10.3389/fpls.2013.00356
van Raij, B., Andrade, J. C., Cantarella, H., and Quaggio, A. J. (2001). Chemical Analysis for Fertility Evaluation of Tropical Soils. Campinas: IAC.
Vardharajula, S., Zulfikar Ali, S., Grover, M., Reddy, G., and Bandi, V. (2011). Drought-tolerant plant growth promoting Bacillus spp.: effect on growth, osmolytes, and antioxidant status of maize under drought stress. J. Plant Interact. 6, 1–14. doi: 10.1080/17429145.2010.535178
Yan, H. X., Liu, L., Li, L., Zhang, P. P., Liang, W. H., and Zhao, H. T. (2016). Research progress on agriculture of plant growth promoting Rhizobacteria. Heilongjiang Agric. Sci. 6, 148–151. doi: 10.11942/j.issn1002-2767.2016.06.0148
Yazdani, M., Bahmanyar, M. A., Pirdashti, H., and Esmaili, M. A. (2009). Effect of phosphate solubilization microorganisms (PSM) and plant growth promoting rhizobacteria (PGPR) on yield and yield components of corn (Zea mays L.). World Acad. Sci. Eng. Technol. 49, 90–92.
Keywords: Azospirillum brasilense, Bacillus subtilis, Pseudomonas fluorescens, grass inoculation, phosphate solubilization, phosphorus concentration
Citation: Rosa PAL, Mortinho ES, Jalal A, Galindo FS, Buzetti S, Fernandes GC, Barco Neto M, Pavinato PS and Teixeira Filho MCM (2020) Inoculation With Growth-Promoting Bacteria Associated With the Reduction of Phosphate Fertilization in Sugarcane. Front. Environ. Sci. 8:32. doi: 10.3389/fenvs.2020.00032
Received: 30 November 2019; Accepted: 03 March 2020;
Published: 25 March 2020.
Edited by:
Dionisios Gasparatos, Agricultural University of Athens, GreeceReviewed by:
Salma Mukhtar, University of the Punjab, PakistanJun Shan, Institute of Soil Science (CAS), China
Copyright © 2020 Rosa, Mortinho, Jalal, Galindo, Buzetti, Fernandes, Barco Neto, Pavinato and Teixeira Filho. This is an open-access article distributed under the terms of the Creative Commons Attribution License (CC BY). The use, distribution or reproduction in other forums is permitted, provided the original author(s) and the copyright owner(s) are credited and that the original publication in this journal is cited, in accordance with accepted academic practice. No use, distribution or reproduction is permitted which does not comply with these terms.
*Correspondence: Marcelo Carvalho Minhoto Teixeira Filho, bWNtLnRlaXhlaXJhLWZpbGhvQHVuZXNwLmJy