- 1Department of Radiation Oncology, Lausanne University Hospital and University of Lausanne, Lausanne, Switzerland
- 2Multidisciplinary Cancer Care Service, Radiation Oncology Unit, Riviera-Chablais Hospital, Rennaz, Switzerland
- 3Heart and Vessel Department, Cardiac MR Center, Lausanne University Hospital and University of Lausanne, Lausanne, Switzerland
- 4Department of Radiation Oncology, Inselspital, Bern University Hospital and University of Bern, Bern, Switzerland
- 5Department of Cardiology, Inselspital, Bern University Hospital and University of Bern, Bern, Switzerland
- 6Department of Radiation Oncology, University Hospital Zurich, Zürich, Switzerland
- 7Department of Cardiology, University Heart Center Zurich, Zürich, Switzerland
- 8Department of Radiation Oncology and Department of Internal Medicine III, Cardiology, Section for Electrophysiology, University Medical Center Schleswig-Holstein, Kiel, Germany
- 9Radio-Oncology Research Laboratory, Lausanne University Hospital and University of Lausanne, Lausanne, Switzerland
- 10Institute of Radiation Physics, Lausanne University Hospital and University of Lausanne, Lausanne, Switzerland
- 11Heart and Vessel Department, Service of Cardiology, Lausanne University Hospital and University of Lausanne, Lausanne, Switzerland
Ventricular tachycardia (VT) caused by myocardial scaring bears a significant risk of mortality and morbidity. Antiarrhythmic drug therapy (AAD) and catheter ablation remain the cornerstone of VT management, but both treatments have limited efficacy and potential adverse effects. Stereotactic body radiotherapy (SBRT) is routinely used in oncology to treat non-invasively solid tumors with high precision and efficacy. Recently, this technology has been evaluated for the treatment of VT. This review presents the basic underlying principles, proof of concept, and main results of trials and case series that used SBRT for the treatment of VT refractory to AAD and catheter ablation.
Introduction
Ventricular tachycardia (VT), an important cause of mortality and morbidity (1), commonly occurs in the context of structural heart diseases (e.g., post-myocardial infarction). Recent progress in cardiac imaging and electroanatomic mapping (EAM) techniques have prompted the use of catheter ablation (CA) for VT substrates delineation and ablation (2, 3). Antiarrhythmic drug therapy (AAD) and CA are the cornerstone of VT management, but both treatments have limited efficacy and potential adverse effects (4, 5). Additionally, despite significant progress in CA efficacy, the recurrence rate after a first VT ablation is about 50% (6), exposing patients to multiple CA procedures (7) and implantable cardioverter-defibrillator (ICD) shocks (8).
Stereotactic radiotherapy (RT), routinely used in the realm of oncology to non-invasively treat solid tumors with high precision and efficacy, appears as a new tool in VT management (9). We sought to review the current literature in order to summarize data and perspectives of this innovative technique in the management of VT.
Ventricular Tachycardia: Pathophysiology, Current Management, and Limitations
VT may be idiopathic or caused by an underlying myocardial substrate that initiates the arrhythmia (e.g., ventricular premature contraction, VPC) and/or maintains the re-entrant circuit. These abnormal anatomical structures are often the result of pathologic changes like post-myocardial infarction scars or post-inflammatory scars. Re-entry is the most common mechanism of VT in this setting (Figure 1). Re-entrant circuits utilize surviving myocytes within or at the border zone of scars resulting in an isthmus (Figure 1) of slow electrical conducting fibers of variable refractoriness (10). Connection of the isthmus to the healthy myocardium determines the site of initial ventricular activation (i.e., exit site, Figure 1) that drives the ECG appearance of the VT. Cardiac inflammatory diseases and post-inflammatory remodeling may also lead to scar and VT susceptibility (11).
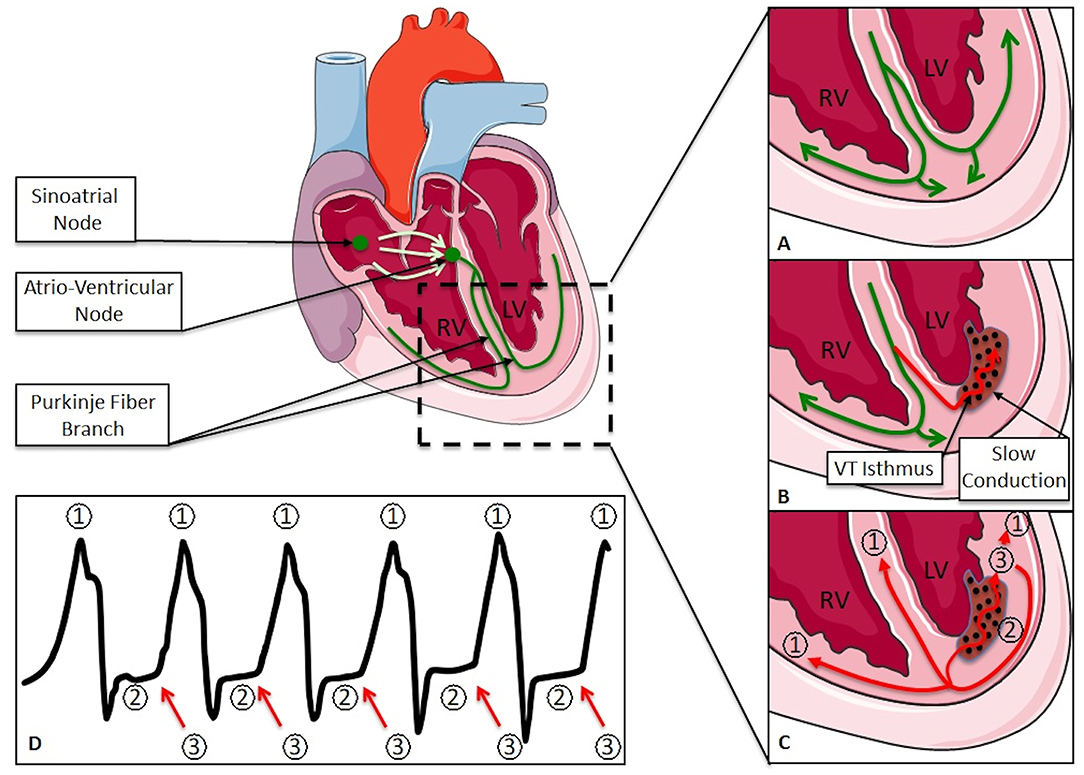
Figure 1. (A) Normal progression of the activation wavefront; (B) slow conduction after myocardial infarction; (C) re-entrant circuit; (D) ECG of a ventricular tachycardia: (1) depolarizing wavefront; (2) slow conduction; (3) exit site. RV, Right Ventricule; LV, Left Ventricule.
Developed in the late 70's, ICDs have revolutionized the approach to the prevention of sudden cardiac death after myocardial infarction (12). ICDs are nowadays commonly used to detect and promptly treat malignant ventricular arrhythmias (13). The benefit of ICDs derives from the ability to interrupt re-entrant VT by anti-tachycardia pacing or shocks. Although these therapies may be life saving, ICD shocks may be traumatic and decrease quality of life (14). Importantly, ICDs are very effective in treating VT episodes but lack any preventive effect.
AAD to supress VT have poor efficacy and multiple side effects that limited their use (4). Interventional therapies, such as CA that disrupts or alters the VT substrate have become the standard of care (6). Most CA techniques currently utilize radiofrequency energy to heat cardiac tissue leading to tissue necrosis, and consequently to the disruption of the VT substrate and circuit. Two approaches have been used over the last decade. The first targets the isthmus of the VT in order to interrupt the re-entrant circuit. The second one, named substrate modification, targets in sinus rhythm any surviving myocardial fibers within or at the border zone of the scar that could serve as potential VT isthmuses because of their slow conducting properties. Fractionated potentials and late activated ventricular potentials are typical examples. Cryoablation, which utilizes a freezant to destroy the VT substrate, has gained increased utilization recently (15). Although CA techniques can be performed for most tachyarrhythmias, their use are also limited by incomplete efficacy, unfavorable side effects, and procedural risks (16). The recurrence rate after CA is about 50% at 2 years for ischemic VT, independently of the chosen approach (substrate modification vs. VT isthmus ablation), with even higher recurrence rates for intramural (e.g., in the interventricular septum) and non-ischemic VTs (17).
For patients with VT refractory to standard treatments, new techniques are described in the literature such as surgical epicardial ablation, video-assisted thoracoscopic cardiac sympathetic denervation (CSD), or intracoronary ethanol infusion (18) and other means under extensive study such as bipolar radiofrequency ablation, half-normal saline irrigation, or needle electrodes (19). Despite limited series, CSD might be an interesting option in patients with sustained VT who have failed both antiarrhythmic medication and catheter ablation for refractory VT (20, 21). However, the antiarrhythmic properties of CSD on VTs arising from structural heart disease need to be deciphered and documented on larger series. To date, there are no data on the combination of alternative techniques with SBRT. The development of non-invasive complementary therapies such as SBRT appears to be an alternative for the most fragile patients and/or those with slow VT.
From Conventional Radiotherapy to Stereotactic Body Radiotherapy
RT utilizes high energy X-rays from linear accelerators (linac) to destroy the targeted tissue, most commonly cancer (Figures 2–4). Historically, conventional RT as exclusive treatment of cancer consisted of multiple daily fractions of irradiation over 4–8 weeks at a dose of 1.8–2 Gy per fraction, leading to high cure rates of various cancers (e.g., prostate, lung, head, and neck). The therapeutic effect of RT is influenced by the dose per fraction and the cumulative total radiation dose, the number of fractions, and the total delivery time. However, the impact on healthy tissues is usually the limiting factor in the total dose delivered to a target tissue (usually the tumor). During the last decades, several technical innovations were introduced in radiation oncology. Modern RT techniques, thanks to on-board imaging systems that check the position of the target and organs at risk (OARs) before treatment, allowed to increase dose delivery to the target volume, while reducing the dose to OARs, and consequently acute and late toxicities (22, 23).
In parallel, a change in the fractionated RT treatment paradigm arose with the advent of brain radiosurgery (24). Radiosurgery usually consists of 1–5 fractions of very high-dose RT (>6 Gy/fraction) delivered with stereotactic accuracy (25). The application of radiosurgical principles outside the brain is termed stereotactic ablative radiotherapy (SABR) stereotactic body radiotherapy (SBRT). Compared to conventional RT, SBRT ablates the targeted tissue with a minimum of fractions. This is supported by radiobiologic studies showing that tumor response is improved with larger doses of radiation delivered in fewer fractions (26, 27). Consequently, due to the high doses also delivered to nearby OARs, SBRT is adapted to small volume targets.
To achieve the necessary high level of accuracy for SBRT dedicated RT devices have been specifically developed for stereotactic treatments at various cancer sites (28). The TrueBeam® system (Varian Medical Systems, Palo Alto, CA), based on a linac, provides image-guided SBRT thank to the on-board cone beam CT and the 6-dimensional couch. Alternatively, the CyberKnife® system (Accuray, Sunnyvale, CA, USA) is an image-guided device dedicated to radiosurgery and SBRT (Figure 4) (29). This device is mounted on a robotic arm to deliver radiation to a tumor from different trajectories, while minimizing dosage to adjacent normal tissue. The CyberKnife® is also able to track tumors directly or alternatively fiducial markers placed in the vicinity of the tumor to deliver highly accurate treatments in order to minimize the dose to surrounding tissues and OARs (30). Recently, RT devices combining linac and medical resonance imaging (MRI) system have been developed fort SBRT treatments (31).
Radiosurgery for non-oncologic diseases is most commonly performed for neurologic disorders or benign central nervous system tumors (32). The most extensively developed data for radiosurgical treatments have pertained to treatment of vestibular schwannoma (33), meningioma of the skull base (34) or seizure (35). Radiosurgery has the advantage of being delivered on an outpatient basis, and has become an excellent alternative to invasive neurosurgery for organ preservation in selected patients.
Heart and Radiotherapy: A Complex Combination
Providing RT for a non-oncologic disease, such as within the heart, may appear paradoxical given the well-known long-term side effects of RT on cardiac tissue. It is important to note that age and pre-existing heart conditions are well-established risk factors for cardiotoxicity of any kind, including radiation-induced cardiac toxicity. Therefore, patients undergoing SBRT for refractory VT are likely to be a risk category (36). Radiation induced cardiac toxicity (RICT) is a late complication of RT, with increasing risk over years after treatment (37, 38). The use of RT contributed to significant survival improvements for patients with breast, lung, esophageal, lymphoma, and thymic cancers. These successes resulted in large cohorts of cancer survivors, who were subject to late or very late complications from RT (39–43). Depending on indication, dose and RT techniques, any subparts of the heart can be damaged such as the pericardium, myocardium, heart valves, coronary arteries, capillaries, and conduction system.
The pathophysiological pathway that leads to RICT suggests that radiation causes both microvascular and macrovascular damages (44). The microvascular injury is characterized by a decrease in capillary density, causing myocardial ischemia and fibrosis. Fibrosis can lead to several consequences: valves dysfunction, pericardial fibrosis (45), and/or effusion, arrhythmia (46, 47), and loss of cardiac compliance leading to diastolic dysfunction (48). Macrovascular injury may manifest as accelerated coronary atherosclerosis (49).
With the emergence of modern RT techniques, heart structures can be much better spared during treatment. Additionally, once RICT was recognized, treatment techniques were modified to minimize cardiac irradiation such as the development of deep inspiration breath-hold techniques during RT for left breast cancer (50). Although there is no minimal safe radiation dose, more recent data suggest a decrease of the dose received by the heart with modern RT techniques, reducing most probably RICT incidence (51).
Currently, heart dose constraints during a thoracic RT are based on the dose received by the whole cardiac volume. To date, there are limited data on the correlation between the dose received by cardiac substructures (valves, coronary arteries, etc.) and potential side effects, and no specific dose constraints validated for each of these substructures. Hahn et al. (52) observed in patients who received mediastinal RT for Hodgkin lymphoma that the risk of late ischemic cardiac events is correlated with the dose received by the coronary arteries such as: volume of left anterior descending artery receiving 5 Gy and volume of left circumflex artery receiving 20 Gy in conventional fractionation (1.8–2 Gy per fraction).
SBRT appears as a more attractive modality to irradiate the heart than conventional RT. Thanks to the highly accurate targeting provided by detailed electroanatomical mapping of the arrhythmia, a better dose fall-off in all directions compared to conventional RT and the possibility to spare cardiac substructures, acute, and long-term toxicities may be minimized (Figure 5) (53). An additional difficulty for cardiac SBRT, compared to the field of neurology, is the presence of a moving target. Indeed, the heart is submitted to its own internal movements and also to breathing. These movements can be taken into account by using RT devices equipped with a tracking system or by the addition of margins corresponding to internal movements (Internal Target Volume, ITV) for linac-based systems.
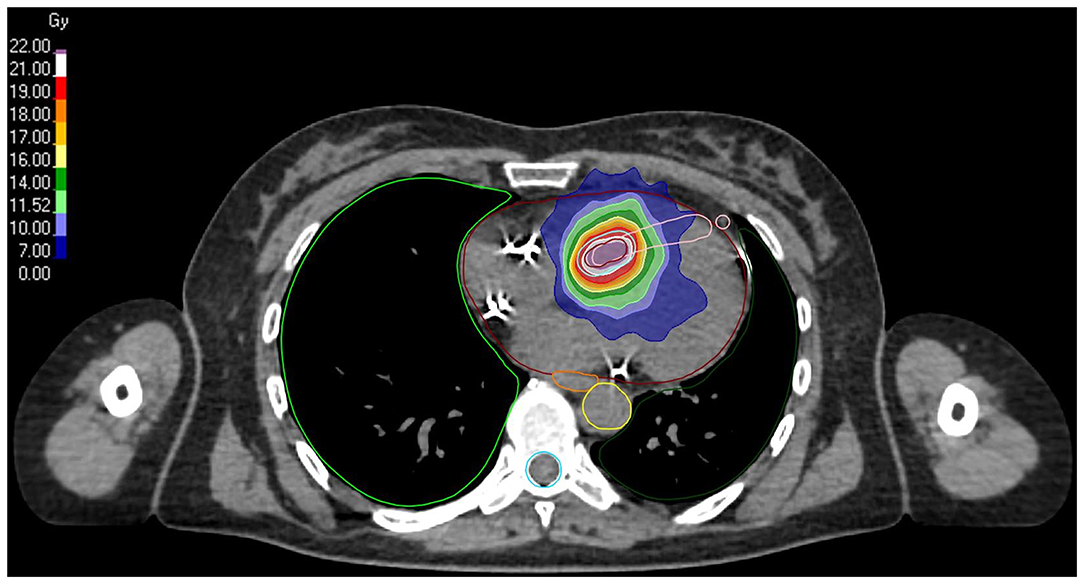
Figure 5. Stereotactic body radiotherapy (SBRT) plan for a ventricular tachycardia (VT) arising from the interventricular septum. The planning target volume is in light blue, the heart is in dark red, the right lung is in green, the left lung is in dark green, the esophagus is in orange, the spinal canal is in blue, the interventricular septum is in pink and the aorta is in yellow.
From Pre-Clinical Data to First Patients
In preclinical studies in healthy animals, the proof-of-principle was demonstrated by irradiating heart tissue to create fibrosis similar to CA. Sharma et al. (54) suggested that a single dose of at least 25 Gy on cavo-tricuspid isthmus, AV node, or pulmonary veins is needed to create a lesion that alters electrophysiological properties. The timeline showed an electrophysiologic effect consistently over 90 days. In 2011, Maguire et al. (55) showed that a single fraction of 25–35 Gy on mini pig pulmonary veins was able to create transmural fibrosis that resulted in electrical isolation 6 months after irradiation. Similarly, Blanck et al. (56) showed that doses >32.5 Gy in the healthy pig heart can induce transmural scarring of cardiac tissue (electrophysiology study 6 months after irradiation). Yet, the underlying electrophysiologic effects of the fibroting and sub-fibroting doses (e.g., <30 Gy) remained unknown beyond healthy animal models. Some additional data could be obtained from pre-clinical studies with carbon ions or protons whose radiobiology might be slightly different as compared to photons (57). Of note, pre-clinical data about scar development at the ventricular level (temporal onset and dose-response relationship) after high dose irradiation are very limited since most studies reported data about atrial tissue (pulmonary veins or cavo-tricuspid isthmus) or AV node irradiation.
To date, clinical outcome of SBRT for cardiac arrhythmias is limited to only small prospective and retrospective case series (Table 1) and case reports. Loo et al. (58) reported the first patient treated with SBRT for AAD-refractory VT after myocardial infarction. The VT substrate was made of surviving myocardial fibers within the scar according to the PET-CT. The treatment was delivered with the CyberKnife® with a temporary pacing wire placed at the right ventricular apex to ensure accuracy (i.e., function as fiducial marker). A similar report by Cvek et al. (59) described the first procedure using the CyberKnife® system in Europe in a patient suffering from a dilated cardiomyopathy. The VT substrate location was based on an electrophysiological (EP) study using an EAM system (CARTO3, Biosense Webster, Irvine, CA, USA). Both treatments were successful and delivered 25 Gy in a single fraction, in imitation of the lowest dose with any effect from the preclinical studies.
The first systematically investigated patients cohort was reported by Cuculich et al. (60) in 2017. In a series of five patients, they reported a strong VT burden reduction of 99.9% after a 6-week blanking period. To determine the location of the VT substrate they used a completely non-invasive mapping method combining a high density surface electrocardiographic imaging technique (ECG-vest with 252 electrodes) that targeted the exit site of the VT and the surrounding ischemic substrate (i.e., infarction and its border zone), merged with a chest CT. All patients received 25 Gy in a single fraction (mean ablation volume of 49 cc) delivered using a linac dedicated for SBRT.
Cuculich et al. then initiated a prospective phase I/II study: ENCORE-VT (NCT02919618) aimed to primarily demonstrate short-term safety and secondarily preliminary efficacy of SBRT for patients with life-threatening, AAD-refractory VT. Mapping of the VT substrate, SBRT delivery, and dose prescription (25 Gy) were similar as for their first patients (60). The main efficacy endpoint was any reduction in VT episodes or any reduction in premature ventricular contractions burden during the 6 months before and after treatment (with a 6-week blanking period after SBRT). First results of this trial with a median follow-up of 13 months have been recently published by Robinson et al. (61). A total of 19 patients were enrolled, with remarkable efficacy in VT burden reduction. The median VT substrate volume (i.e., Gross Target Volume, GTV) was 25.4 cc, the median ITV volume (taking movements into account) was 31 cc and the median final ablation volume (i.e., Planning Target Volume, PTV) was 98.9 cc. The median number of VT episodes decreased from 119 (range, 4–292) in the 6 months before to 3 (range, 0–31) in the 6 months after SBRT. ICD shocks were also significantly reduced from a median of 4 (range, 0–30) to 0 (range, 0–7). Regarding toxicity, 2 patients (10.5%) experienced grade 3 treatment-related adverse events (heart failure and pericarditis) within 90 days of SBRT delivery. In addition, six patients (28%) had treatment-related pericardial effusions including one grade 3 and one grade 4; 11% of patients had pneumonitis that resolved with steroids. The authors recently presented longer-term results showing a persistent effect of SBRT 2 years after treatment in most patients. Additionally, serious toxicity was low, but may occur after 2 years: two grade 3 pericardial effusions and one grade 4 gastro-pericardial fistula (62).
Furthermore, Neuwirth et al. (63) published a larger retrospective case series on SBRT in VT patients refractory to CA with longer term follow-up. Mapping of the VT substrate was based on an electrophysiological (EP) study using an EAM system (CARTO3, Biosense Webster, Israel) as for their first patient (59) and SBRT was delivered using the CyberKnife® system with a single fraction of 25 Gy (mean PTV of 22.2 cc). Results of this series have been recently published at a median follow-up of 28 months. Ten patients were treated, with a VT burden reduction of 87.5% compared to baseline, however, after a 3-month blanking period, VT recurred in 8/10 patients. Finally, they report one possible treatment-related toxicity (mild nausea) and a possible grade 3 late treatment related toxicity (progression of mitral regurgitation 17 months after SBRT). To further study the long-term safety and efficacy of SBRT for TV, the authors have initiated a multicenter prospective study (NCT03819504) (64).
A case series has been published by Lloyd et al. (65) showing the interest of SBRT for refractory VT in advanced heart failure patients. A total of 10 patients with VT refractory to standard treatments were included and received a single fraction of 25 Gy using a linac system dedicated for SBRT with a mean PTV of 81.4 cc. Among eight patients with available ICD data, the total reduction in seconds of detected VT was 69% and the reduction in total ICD shocks after SBRT was 68%. It should be noticed that in this study no blanking-period was considered. They concluded that SBRT for refractory VT was feasible and modestly effective at reducing VT burden in advanced heart failure patients. Interestingly, three patients had post-SBRT histology since they received heart transplant after treatment. Microscopic analyses of the treated regions showed oedema and vacuolization of endothelial cells with mild fibrosis. Electron microscopy of one sample revealed disruption of intercalated disc/gap junction area.
Our group described the first immediate and durable response to cardiac SBRT in an intensive care patient suffering from an electrical storm (ES) due to incessant VT unresponsive to CA and AADs (66). An EP study performed with an EAM was used to delineate the VT substrate location. The right ventricular ICD lead served as a fiducial marker for tracking with the CyberKnife® system. A total dose of 25 Gy in a single fraction was delivered, while the patient was intubated and sedated in the treatment room. Surprisingly, the SBRT rapidly controlled the ES, allowing the extubation of the patient 3 days after the procedure without any recurrence thereafter. To date, our group has treated 10 patients with VT refractory to AAD and CA (67). Eight patients were elective, while the other two (one corresponding to the previously cited patient) were hospitalized in the intensive care unit. All patients had an EAM prior SBRT to define the VT substrate. The mean dose of 22 Gy (range, 20–25) was delivered to the VT substrate (mean PTV of 23 cc) using the CyberKnife® system. At a median follow-up of 6 months (range, 1–14), the elective patients did not experience any sustained VT recurrence or ICD shock. Importantly, no detectable severe adverse events related to SBRT occurred (Table 1).
Recently, another prospective study published by Gianni et al. (68) conducted on five patients mitigates these results. Indeed, the 1-year follow-up reports a recurrence of ventricular arrhythmias in all patients, despite an initial reduction observed in the first 6 months post-ablation. Interestingly, three patients had a redo procedure, which showed surviving bundles of cardiomyocytes within the putative PTV.
Although clinical outcomes of 20–25 Gy single-dose SBRT for refractory VT remain limited to small series and few case reports, results of this new technique are very promising. A longer follow-up on larger cohorts is warranted to assess the efficacy and safety of this technique.
Perspectives
The efficacy of cardiac SBRT has been attributed to radiation-induced fibrosis (Figure 2) that creates conduction blocks within the heart. However, the reduction of VT episodes within a couple of days after a dose of 25 Gy in the rescue procedure reported by Jumeau et al. (66) suggests that the mechanisms of action may not only be attributed to radiation-induced fibrosis. Similarly, in the series by Cuculich et al. (60), all patients presented a strong reduction in VT episodes within the first month after SBRT, which may not only be attributed to late radiation-induced fibrosis which is usually observed months later. These data support an immediate benefit of SBRT on the VT substrate by other mechanisms, which is consistent with preclinical data showing that single fraction doses above 30 Gy are necessary to create dense, and transmural fibrosis in the heart (56). In vivo data by Fajardo and Stewart (69) in animal models showed the presence of inflammatory cells in heart tissue within hours after heart irradiation that could explain this early response. Another interesting experimental finding is that RT might be antiarrhythmic by restoring localization Connexin 43 at intercalated disks both in normal and post-mocardial infarction tissue, which was associated with improved conduction properties and reduced repolarization dispersion (70). There is therefore a need to better understand the pathophysiological mechanisms of SBRT on heart tissue and more specifically on the VT substrate.
While clearly promising, these first steps represent only the beginning of a journey to introduce SBRT into the treatment of cardiac arrhythmias. It is essential to make the technique as safe as possible to avoid toxicities by minimizing unnecessary irradiation of the heart and surrounding tissues. The two main parameters that influence this “unnecessary” irradiation are the ablation volume (i.e., VT substrate) and the prescribed dose. Therefore, there is a need to determine the minimal radiation dose level capable to maintain the efficacy of SBRT for the treatment of cardiac arrhythmias. Importantly, the dose of 25 Gy used so far in cardiac SBRT for VT ablation is relatively high compared to other benign diseases: 18–20 Gy in a single fraction for an arterio-venous malformation (71) or 18 Gy for seizure (35). By reducing the target dose to a minimal effective level, the dose to the surrounding tissue will be reduced, which will lower the probability of long-term complications.
It is also important to keep in mind that the VT substrate is related to the underlying heart disease, e.g., in ischemic VT the substrate is usually delineated, confluent, and limited to the distribution area of a coronary epicardial vessel. In non-ischemic dilated cardiomyopathy, arrhythmogenic cardiomyopathy, or myocarditis/sarcoidosis, the substrate is usually scattered and involves a larger area of the heart. Additionally, the ablation volume is not only related to the VT substrate volume, but also to the SBRT technique. In fact, depending on the SBRT device or on the tracking method, additional geometric safety margins for uncertainty are needed that increase the final ablation volume (i.e., planning target volume, PTV). This has been well-observed in the series of Cuculich and Robinson et al. (60, 61) where the non-invasive mapping of the VT exit (and not of the VT isthmus) and of the ischemic substrate combined with a free-breathing linac based treatment resulted in high PTV dimensions. This has been investigated by Knutson et al. (72) in a dosimetric analysis from ENCORE-VT trial that showed a decrease of PTV between the first and the last treated patient (i.e., learning curve). Hence, wider dose spreading (Figure 1) to OARs could promote pneumonitis and pericarditis.
Recent advances in medical imaging and RT have improved target definition and tracking accuracy. New image-guided RT techniques are currently emerging, including MRI-linac that can combine the possibility of continuous non-ionizing imaging with direct target tracking (73). In the future there is probably a lot to expect from protons and carbon ions as many pre-clinical studies are based on these particle beams (74); a first VT patient has been recently treated using protons (75).
Finally, as with any novel technological advance, radiation therapy for the treatment of cardiac arrhythmias will ultimately have to be tested in well-controlled clinical trials in order to adequately assess the benefits and risks associated with this promising approach. A multi-center, multi-platform clinical feasibility trial on the initial safety profile of radiosurgery for ventricular tachycardia (RAVENTA, NCT03867747) is now recruiting in Germany. To date, several studies have also begun or are in preparation in Milan, Italy (NCT04066517); Calgary, Canada (NCT04065802) or Amsterdam, The Netherlands (NL7510). Before results of these trials with clearly defined protocols become available, this therapy needs to be limited to large centers with collaborative network knowledge in order to optimize patient benefit and safety.
Conclusions
Cardiac SBRT only recently emerged as a promising treatment option for the management of refractory VT. It appears to be an effective and non-invasive option. Given the recentness of this technology and the scarcity of prospective clinical data with limited long-term follow-up, further research and clinical experience are warranted within prospective clinical trials.
Author Contributions
RJ and EP: conception and design. All authors: administrative support and final approval. RJ, MO, EP, and JB: collection and assembly of data. RJ, OB, and EP: data analysis and interpretation.
Conflict of Interest
OE reports personal fees from Merck Serono, personal fees from MSD Global, personal fees from AstraZeneca, outside the submitted work. TR reports grants and personal fees from Biosense Webster, grants from Boston Scientific, grants from Biotronik, grants and personal fees from Medtronic, grants from Abbott/SJM, personal fees from Daiichi, personal fees from BMS-Pfizer, outside the submitted work. JSt reports personal fees from Amgen, personal fees from Astra Zeneca, grants and personal fees from Bayer Healthcare, personal fees from Boehringer-Ingelheim, grants and personal fees from Biosense Webster, grants and personal fees from Boston Scientifc, personal fees from Bristol-Myers Squibb, grants and personal fees from Daiichi-Sankyo, grants and personal fees from Medtronic, personal fees from Novartis, personal fees from Pfizer, personal fees from Sanofi-Aventis, grants and personal fees from St. Jude Medical/Abbott, personal fees from Zoll, other from CorXL (ownership), grants and personal fees from Biotronik, personal fees from Atricure, personal fees from Medscape, personal fees from WebMD, personal fees from Merck/MSD, outside the submitted work. OB reports to have been an employee of CyberHeart Inc. (Sunnyvale, CA, USA) from 2008 to 2010, though he reports no financial ties or obligations or conflict of interest to or with the company or its legal predecessor Varian Inc. (Sunnyvale, CA, USA). CH-S reports personal fees from Biosense Webster, outside the submitted work. AS received educational grants from Abbott, Bayer Healthcare, Biosense Webster, Biotronik, Boston Scientific, BMS/Pfizer, and Medtronic. He owns shares from Gilead Sciences.
The remaining authors declare that the research was conducted in the absence of any commercial or financial relationships that could be construed as a potential conflict of interest.
References
1. Tang PT, Shenasa M, Boyle NG. Ventricular arrhythmias and sudden cardiac death. Card Electrophysiol Clin. (2017) 9:693–708. doi: 10.1016/j.ccep.2017.08.004
2. Cochet H, Komatsu Y, Sacher F, Jadidi AS, Scherr D, Riffaud M, et al. Integration of merged delayed-enhanced magnetic resonance imaging and multidetector computed tomography for the guidance of ventricular tachycardia ablation: a pilot study. J Cardiovasc Electrophysiol. (2013) 24:419–26. doi: 10.1111/jce.12052
3. Dickfeld T, Tian J, Ahmad G, Jimenez A, Turgeman A, Kuk R, et al. MRI-guided ventricular tachycardia ablation: integration of late gadolinium-enhanced 3D scar in patients with implantable cardioverter-defibrillators. Circ Arrhythm Electrophysiol. (2011) 4:172–84. doi: 10.1161/CIRCEP.110.958744
4. Antiarrhythmics versus Implantable Defibrillators (AVID) Investigators. A comparison of antiarrhythmic-drug therapy with implantable defibrillators in patients resuscitated from near-fatal ventricular arrhythmias. N Engl J Med. (1997) 337:1576–83. doi: 10.1056/NEJM199711273372202
5. Connolly SJ, Dorian P, Roberts RS, Gent M, Bailin S, Fain ES, et al. Optimal pharmacological therapy in cardioverter defibrillator patients (OPTIC) investigators. Comparison of beta-blockers, amiodarone plus beta-blockers, or sotalol for prevention of shocks from implantable cardioverter defibrillators: the OPTIC Study: a randomized trial. JAMA. (2006) 295:165–71. doi: 10.1001/jama.295.2.165
6. Sapp JL, Wells GA, Parkash R, Stevenson WG, Blier L, Sarrazin J-F, et al. Ventricular tachycardia ablation versus escalation of antiarrhythmic drugs. N Engl J Med. (2016) 375:111–21. doi: 10.1056/NEJMoa1513614
7. Aliot EM, Stevenson WG, Almendral-Garrote JM, Bogun F, Calkins CH, Delacretaz E, et al. European Society of Cardiology, Heart Rhythm Society. EHRA/HRS Expert Consensus on Catheter Ablation of Ventricular Arrhythmias: developed in a partnership with the European Heart Rhythm Association (EHRA), a Registered Branch of the European Society of Cardiology (ESC), and the Heart Rhythm Society (HRS); in collaboration with the American College of Cardiology (ACC) and the American Heart Association (AHA). Europace. (2009) 11:771–817. doi: 10.1093/europace/eup098
8. Poole JE, Johnson GW, Hellkamp AS, Anderson J, Callans DJ, Raitt MH, et al. Prognostic importance of defibrillator shocks in patients with heart failure. N Engl J Med. (2008) 359:1009–17. doi: 10.1056/NEJMoa071098
9. Zei PC, Soltys S. Ablative radiotherapy as a noninvasive alternative to catheter ablation for cardiac arrhythmias. Curr Cardiol Rep. (2017) 19:79. doi: 10.1007/s11886-017-0886-2
10. MacIntyre CJ, Sapp JL. Treatment of persistent ventricular tachycardia: Drugs or ablation? Trends Cardiovasc Med. (2017) 27:506–13. doi: 10.1016/j.tcm.2017.05.004
11. Baldinger SH, Stevenson WG, John RM. Ablation of ischemic ventricular tachycardia: evidence, techniques, results, and future directions. Curr Opin Cardiol. (2016) 31:29–36. doi: 10.1097/HCO.0000000000000237
12. Mirowski M, Reid PR, Mower MM, Watkins L, Gott VL, Schauble JF, et al. Termination of malignant ventricular arrhythmias with an implanted automatic defibrillator in human beings. N Engl J Med. (1980) 303:322–4. doi: 10.1056/NEJM198008073030607
13. Borne RT, Varosy PD, Masoudi FA. Implantable cardioverter-defibrillator shocks: epidemiology, outcomes, and therapeutic approaches. JAMA Intern Med. (2013) 173:859–65. doi: 10.1001/jamainternmed.2013.428
14. Ahmad M, Bloomstein L, Roelke M, Bernstein AD, Parsonnet V. Patients' attitudes toward implanted defibrillator shocks. Pacing Clin Electrophysiol. (2000) 23:934–8. doi: 10.1111/j.1540-8159.2000.tb00877.x
15. Rivera S, Ricapito Mde L, Tomas L, Parodi J, Bardera Molina G, Banega R, et al. Results of Cryoenergy and Radiofrequency-Based Catheter Ablation for Treating Ventricular Arrhythmias Arising From the Papillary Muscles of the Left Ventricle, Guided by Intracardiac Echocardiography and Image Integration. Circ Arrhythm Electrophysiol. (2016) 9:e003874. doi: 10.1161/CIRCEP.115.003874
16. Santangeli P, Muser D, Maeda S, Filtz A, Zado ES, Frankel DS, et al. Comparative effectiveness of antiarrhythmic drugs and catheter ablation for the prevention of recurrent ventricular tachycardia in patients with implantable cardioverter-defibrillators: a systematic review and meta-analysis of randomized controlled trials. Heart Rhythm. (2016) 13:1552–9 doi: 10.1016/j.hrthm.2016.03.004
17. Piers SRD, Leong DP, van Huls van Taxis CFB, Tayyebi M, Trines SA, Pijnappels DA, et al. Outcome of ventricular tachycardia ablation in patients with nonischemic cardiomyopathy: the impact of noninducibility. Circ Arrhythm Electrophysiol. (2013) 6:513–21. doi: 10.1161/CIRCEP.113.000089
18. Cronin EM, Bogun FM, Maury P, Peichl P, Chen M, Namboodiri N, et al. 2019 HRS/EHRA/APHRS/LAHRS expert consensus statement on catheter ablation of ventricular arrhythmias. J Arrhythmia. (2019) 35:323–484. doi: 10.1002/joa3.12185
19. Bhaskaran A, Tung R, Stevenson WG, Kumar S. Catheter ablation of VT in non-ischaemic cardiomyopathies: endocardial, epicardial and intramural approaches. Heart Lung Circ. (2019) 28:84–101. doi: 10.1016/j.hlc.2018.10.007
20. Vaseghi M, Gima J, Kanaan C, Ajijola OA, Marmureanu A, Mahajan A, et al. Cardiac sympathetic denervation in patients with refractory ventricular arrhythmias or electrical storm: intermediate and long-term follow-up. Heart Rhythm. (2014) 11:360–6. doi: 10.1016/j.hrthm.2013.11.028
21. Vaseghi M, Barwad P, Malavassi Corrales FJ, Tandri H, Mathuria N, et al. Cardiac sympathetic denervation for refractory ventricular arrhythmias. J Am Coll Cardiol. (2017) 69:3070–80. doi: 10.1016/j.jacc.2017.04.035
22. Reese AS, Lu W, Regine WF. Utilization of intensity-modulated radiation therapy and image-guided radiation therapy in pancreatic cancer: is it beneficial? Semin Radiat Oncol. (2014) 24:132–9. doi: 10.1016/j.semradonc.2013.11.003
23. Bockbrader M, Kim E. Role of intensity-modulated radiation therapy in gastrointestinal cancer. Expert Rev Anticancer Ther. (2009) 9:637–47. doi: 10.1586/era.09.16
24. Leksell L. The stereotaxic method and radiosurgery of the brain. Acta Chir Scand. (1951) 102:316–9.
25. Kollar L, Rengan R. Stereotactic body radiotherapy. Semin Oncol. (2014) 41:776–89. doi: 10.1053/j.seminoncol.2014.09.022
26. Kirkpatrick JP, Kelsey CR, Palta M, Cabrera AR, Salama JK, Patel P, et al. Stereotactic body radiotherapy: a critical review for nonradiation oncologists. Cancer. (2014) 120:942–54. doi: 10.1002/cncr.28515
27. Ruggieri R, Stavrev P, Naccarato S, Stavreva N, Alongi F, Nahum AE. Optimal dose and fraction number in SBRT of lung tumours: a radiobiological analysis. Phys Med. (2017) 44:188–95. doi: 10.1016/j.ejmp.2016.12.012
28. Timmerman RD, Kavanagh BD, Cho LC, Papiez L, Xing L. Stereotactic body radiation therapy in multiple organ sites. J Clin Oncol. (2007) 25:947–52. doi: 10.1200/JCO.2006.09.7469
29. Adler JR, Chang SD, Murphy MJ, Doty J, Geis P, Hancock SL. The Cyberknife: a frameless robotic system for radiosurgery. Stereotact Funct Neurosurg. (1997) 69(1–4 Pt 2):124–8. doi: 10.1159/000099863
30. Whyte RI, Crownover R, Murphy MJ, Martin DP, Rice TW, DeCamp MM, et al. Stereotactic radiosurgery for lung tumors: preliminary report of a phase I trial. Ann Thorac Surg. (2003) 75:1097–101. doi: 10.1016/S0003-4975(02)04681-7
31. Lagendijk JJW, Raaymakers BW, van Vulpen M. The magnetic resonance imaging-linac system. Semin Radiat Oncol. (2014) 24:207–9. doi: 10.1016/j.semradonc.2014.02.009
32. Chen JCT, Girvigian MR. Stereotactic radiosurgery: indications and results - part 2. Perm J. (2006) 10:9–15. doi: 10.7812/TPP/04-076
33. Pellet W, Regis J, Roche PH, Delsanti C. Relative indications for radiosurgery and microsurgery for acoustic schwannoma. Adv Tech Stand Neurosurg. (2003) 28:227–82; discussion 282–4. doi: 10.1007/978-3-7091-0641-9_4
34. Nicolato A, Foroni R, Alessandrini F, Maluta S, Bricolo A, Gerosa M. The role of Gamma Knife radiosurgery in the management of cavernous sinus meningiomas. Int J Radiat Oncol Biol Phys. (2002) 53:992–1000. doi: 10.1016/S0360-3016(02)02802-X
35. Abla AA, Shetter AG, Chang SW, Wait SD, Brachman DG, Ng Y-T, et al. Gamma Knife surgery for hypothalamic hamartomas and epilepsy: patient selection and outcomes. J Neurosurg. (2010) 113(Suppl):207–14. doi: 10.3171/2010.8.GKS101027
36. Haque R, Prout M, Geiger AM, Kamineni A, Thwin SS, Avila C, et al. Comorbidities and cardiovascular disease risk in older breast cancer survivors. Am J Manag Care. (2014) 20:86–92. Available online at: https://www.ajmc.com/journals/issue/2014/2014-vol20-n1/comorbidities-and-cardiovascular-disease-risk-in-older-breast-cancer-survivors
37. Clarke M, Collins R, Darby S, Davies C, Elphinstone P, Evans E, et al. Effects of radiotherapy and of differences in the extent of surgery for early breast cancer on local recurrence and 15-year survival: an overview of the randomised trials. Lancet. (2005) 366:2087–106. doi: 10.1016/S0140-6736(05)67887-7
38. Hooning MJ, Botma A, Aleman BMP, Baaijens MHA, Bartelink H, Klijn JGM, et al. Long-term risk of cardiovascular disease in 10-year survivors of breast cancer. J Natl Cancer Inst. (2007) 99:365–75. doi: 10.1093/jnci/djk064
39. Darby SC, Ewertz M, McGale P, Bennet AM, Blom-Goldman U, Brønnum D, et al. Risk of ischemic heart disease in women after radiotherapy for breast cancer. N Engl J Med. (2013) 368:987–98. doi: 10.1056/NEJMoa1209825
40. Hancock SL, Tucker MA, Hoppe RT. Factors affecting late mortality from heart disease after treatment of Hodgkin's disease. JAMA. (1993) 270:1949–55. doi: 10.1001/jama.270.16.1949
41. Boerma M, Sridharan V, Mao X-W, Nelson GA, Cheema AK, Koturbash I, et al. Effects of ionizing radiation on the heart. Mutat Res. (2016) 770(Pt B):319–27. doi: 10.1016/j.mrrev.2016.07.003
42. Mansouri I, Allodji RS, Hill C, El-Fayech C, Pein F, Diallo S, et al. The role of irradiated heart and left ventricular volumes in heart failure occurrence after childhood cancer. Eur J Heart Fail. (2019) 21:509–18. doi: 10.1002/ejhf.1376
43. Haddy N, Diallo S, El-Fayech C, Schwartz B, Pein F, Hawkins M, et al. Cardiac diseases following childhood cancer treatment: cohort study. Circulation. (2016) 133:31–8. doi: 10.1161/CIRCULATIONAHA.115.016686
44. Darby SC, Cutter DJ, Boerma M, Constine LS, Fajardo LF, Kodama K, et al. Radiation-related heart disease: current knowledge and future prospects. Int J Radiat Oncol. (2010) 76:656–65. doi: 10.1016/j.ijrobp.2009.09.064
45. Hooning MJ, Aleman BMP, van Rosmalen AJM, Kuenen MA, Klijn JGM, van Leeuwen FE. Cause-specific mortality in long-term survivors of breast cancer: a 25-year follow-up study. Int J Radiat Oncol Biol Phys. (2006) 64:1081–91. doi: 10.1016/j.ijrobp.2005.10.022
46. Orzan F, Brusca A, Gaita F, Giustetto C, Figliomeni MC, Libero L. Associated cardiac lesions in patients with radiation-induced complete heart block. Int J Cardiol. (1993) 39:151–6. doi: 10.1016/0167-5273(93)90027-E
47. Larsen RL, Jakacki RI, Vetter VL, Meadows AT, Silber JH, Barber G. Electrocardiographic changes and arrhythmias after cancer therapy in children and young adults. Am J Cardiol. (1992) 70:73–7. doi: 10.1016/0002-9149(92)91393-I
48. Heidenreich PA, Hancock SL, Vagelos RH, Lee BK, Schnittger I. Diastolic dysfunction after mediastinal irradiation. Am Heart J. (2005) 150:977–82. doi: 10.1016/j.ahj.2004.12.026
49. Cuzick J, Stewart H, Rutqvist L, Houghton J, Edwards R, Redmond C, et al. Cause-specific mortality in long-term survivors of breast cancer who participated in trials of radiotherapy. J Clin Oncol. (1994) 12:447–53. doi: 10.1200/JCO.1994.12.3.447
50. Boda-Heggemann J, Knopf A-C, Simeonova-Chergou A, Wertz H, Stieler F, Jahnke A, et al. Deep inspiration breath hold-based radiation therapy: a clinical review. Int J Radiat Oncol Biol Phys. (2016) 94:478–92. doi: 10.1016/j.ijrobp.2015.11.049
51. Shah C, Badiyan S, Berry S, Khan AJ, Goyal S, Schulte K, et al. Cardiac dose sparing and avoidance techniques in breast cancer radiotherapy. Radiother Oncol. (2014) 112:9–16. doi: 10.1016/j.radonc.2014.04.009
52. Hahn E, Jiang H, Ng A, Bashir S, Ahmed S, Tsang R, et al. Late cardiac toxicity after mediastinal radiation therapy for hodgkin lymphoma: contributions of coronary artery and whole heart dose-volume variables to risk prediction. Int J Radiat Oncol Biol Phys. (2017) 98:1116–23. doi: 10.1016/j.ijrobp.2017.03.026
53. Spartalis M, Spartalis E, Athanasiou A, Nikiteas N. Safety concerns regarding ablative radiotherapy for ventricular tachycardia. Radiother Oncol. (2018) 128:387. doi: 10.1016/j.radonc.2018.06.009
54. Sharma A, Wong D, Weidlich G, Fogarty T, Jack A, Sumanaweera T, et al. Noninvasive stereotactic radiosurgery (CyberHeart) for creation of ablation lesions in the atrium. Heart Rhythm. (2010) 7:802–10. doi: 10.1016/j.hrthm.2010.02.010
55. Maguire PJ, Gardner E, Jack AB, Zei P, Al-Ahmad A, Fajardo L, et al. Cardiac radiosurgery (CyberHeart™) for treatment of arrhythmia: physiologic and histopathologic correlation in the porcine model. Cureus. (2011) 3:e32. doi: 10.7759/cureus.32
56. Blanck O, Bode F, Gebhard M, Hunold P, Brandt S, Bruder R, et al. Dose-escalation study for cardiac radiosurgery in a porcine model. Int J Radiat Oncol Biol Phys. (2014) 89:590–8. doi: 10.1016/j.ijrobp.2014.02.036
57. van der Ree MH, Blanck O, Limpens J, Lee CH, Balgobind BV, Dieleman EMT, et al. Cardiac radioablation-a systematic review. Heart Rhythm. (2020). doi: 10.1016/j.hrthm.2020.03.013. [Epub ahead of print].
58. Loo BW, Soltys SG, Wang L, Lo A, Fahimian BP, Iagaru A, et al. Stereotactic ablative radiotherapy for the treatment of refractory cardiac ventricular arrhythmia. Circ Arrhythm Electrophysiol. (2015) 8:748–50. doi: 10.1161/CIRCEP.115.002765
59. Cvek J, Neuwirth R, Knybel L, Molenda L, Otahal B, Pindor J, et al. Cardiac radiosurgery for malignant ventricular tachycardia. Cureus. (2014) 6:e190. doi: 10.7759/cureus.190
60. Cuculich PS, Schill MR, Kashani R, Mutic S, Lang A, Cooper D, et al. Noninvasive cardiac radiation for ablation of ventricular tachycardia. N Engl J Med. (2017) 377:2325–36. doi: 10.1056/NEJMoa1613773
61. Robinson CG, Samson PP, Moore KMS, Hugo GD, Knutson N, Mutic S, et al. Phase I/II Trial of Electrophysiology-Guided Noninvasive Cardiac Radioablation for Ventricular Tachycardia. Circulation. (2018) 139:313–21. doi: 10.1161/CIRCULATIONAHA.118.038261
62. Robinson CG, Samson P, Moore KMS, Hugo GD, Knutson N, Mutic S, et al. Longer term results from a phase i/ii study of EP-guided noninvasive cardiac radioablation for treatment of ventricular tachycardia (ENCORE-VT). Int J Radiat Oncol. (2019) 105:682. doi: 10.1016/j.ijrobp.2019.08.032
63. Neuwirth R, Cvek J, Knybel L, Jiravsky O, Molenda L, Kodaj M, et al. Stereotactic radiosurgery for ablation of ventricular tachycardia. Europace. (2019) 21:1088–95. doi: 10.1093/europace/euz133
64. Cvek J, Knybel L, Neuwirth R, Jiravsky O, Sramko M, Peichel P, et al. 3256 Long-term safety of stereotactic body radiotherapy for ablation of ventricular tachycardia: a multicentric study. Eur Heart J. (2019) 40(Supp1):ehz745.0048. doi: 10.1093/eurheartj/ehz745.0048
65. Lloyd MS, Wight J, Schneider F, Hoskins M, Attia T, Escott C, et al. Clinical experience of stereotactic body radiation for refractory ventricular tachycardia in advanced heart failure patients. Heart Rhythm. (2020) 17:415–22. doi: 10.1016/j.hrthm.2019.09.028
66. Jumeau R, Ozsahin M, Schwitter J, Vallet V, Duclos F, Zeverino M, et al. Rescue procedure for an electrical storm using robotic non-invasive cardiac radio-ablation. Radiother Oncol. (2018) 128:189–91. doi: 10.1016/j.radonc.2018.04.025
67. Jumeau R, Ozsahin M, Schwitter J, Duclos F, Vallet V, Zeverino M, et al. Stereotactic body radiotherapy for refractory ventricular tachycardia: clinical outcomes. Radiother Oncol. (2019) 133:S469. doi: 10.1016/S0167-8140(19)31308-8
68. Gianni C, Rivera D, Burkhardt JD, Pollard B, Gardner E, Maguire P, et al. Stereotactic arrhythmia radioablation for refractory scar-related ventricular tachycardia. Heart Rhythm. (2020). doi: 10.1016/j.hrthm.2020.02.036. [Epub ahead of print].
69. Fajardo LF, Stewart JR. Experimental radiation-induced heart disease. I. Light microscopic studies. Am J Pathol. (1970) 59:299–316.
70. Amino M, Yoshioka K, Tanabe T, Tanaka E, Mori H, Furusawa Y, et al. Heavy ion radiation up-regulates Cx43 and ameliorates arrhythmogenic substrates in hearts after myocardial infarction. Cardiovasc Res. (2006) 72:412–21. doi: 10.1016/j.cardiores.2006.09.010
71. Oermann EK, Murthy N, Chen V, Baimeedi A, Sasaki-Adams D, McGrail K, et al. A multicenter retrospective study of frameless robotic radiosurgery for intracranial arteriovenous malformation. Front Oncol. (2014) 4:298. doi: 10.3389/fonc.2014.00298
72. Knutson NC, Samson PP, Hugo GD, et al. Radiation Therapy Workflow and Dosimetric Analysis from a Phase 1/2 Trial of Noninvasive Cardiac Radioablation for Ventricular Tachycardia. Int J Radiat Oncol Biol Phys. (2019) 104:1114–23. doi: 10.1016/j.ijrobp.2019.04.005
73. Mayinger M, Kovacs B, Tanadini-Lang S, Ehrbar S, Wilke L, Chamberlain M, et al. First magnetic resonance imaging-guided cardiac radioablation of sustained ventricular tachycardia. Radiother Oncol. (2020). doi: 10.1016/j.radonc.2020.01.008. [Epub ahead of print].
74. Graeff C, Bert C. Noninvasive cardiac arrhythmia ablation with particle beams. Med Phys. (2018) 45:e1024–35. doi: 10.1002/mp.12595
Keywords: ventricular tachycardia, radiotherapy, stereotactic radiotherapy, ablation, noninvasive
Citation: Jumeau R, Ozsahin M, Schwitter J, Elicin O, Reichlin T, Roten L, Andratschke N, Mayinger M, Saguner AM, Steffel J, Blanck O, Vozenin M-C, Moeckli R, Zeverino M, Vallet V, Herrera-Siklody C, Pascale P, Bourhis J and Pruvot E (2020) Stereotactic Radiotherapy for the Management of Refractory Ventricular Tachycardia: Promise and Future Directions. Front. Cardiovasc. Med. 7:108. doi: 10.3389/fcvm.2020.00108
Received: 23 March 2020; Accepted: 22 May 2020;
Published: 25 June 2020.
Edited by:
Matteo Anselmino, University of Turin, ItalyCopyright © 2020 Jumeau, Ozsahin, Schwitter, Elicin, Reichlin, Roten, Andratschke, Mayinger, Saguner, Steffel, Blanck, Vozenin, Moeckli, Zeverino, Vallet, Herrera-Siklody, Pascale, Bourhis and Pruvot. This is an open-access article distributed under the terms of the Creative Commons Attribution License (CC BY). The use, distribution or reproduction in other forums is permitted, provided the original author(s) and the copyright owner(s) are credited and that the original publication in this journal is cited, in accordance with accepted academic practice. No use, distribution or reproduction is permitted which does not comply with these terms.
*Correspondence: Etienne Pruvot, ZXRpZW5uZS5wcnV2b3QmI3gwMDA0MDtjaHV2LmNo
†These authors have contributed equally to this work