- Institute of Interdisciplinary Integrative Medicine Research, Shanghai University of Traditional Chinese Medicine, Shanghai, China
Anoplin is an amphipathic, α-helical bioactive peptide from wasp venom. In recent years, pharmaceutical and organic chemists discovered that anoplin and its derivatives showed multiple pharmacological activities in antibacterial, antitumor, antifungal, and antimalarial activities. Owing to the simple and unique structure and diverse biological activities, anoplin has attracted considerable research interests. This review highlights the advances in structural modification, biological activities, and the outlook of anoplin in order to provide a basis for new drug design and delivery.
Introduction
Wasp venom is the light yellow, transparent liquid secreted by the venom glands and accessory glands of wasp; it is stored in a poison sac and discharged by sting (Monteiro et al., 2009). In general, venom is used as a protective measure against the invaders that threaten their survival or colony (Hou et al., 2014). It is worth mentioning that, for wasps, venom is also used during the hunting of prey (Yan et al., 2011). Wasp venom is mainly composed of a few biological active peptides (like mast cell degranulating peptides and mastoparan) (Argiolas and Pisano, 1985; Monteiro et al., 2009), active enzymes (phospholipase A2 and hyaluronidase) (Tsai et al., 2011), histamine, 5-hydroxytryptamine, choline, glycerin, amino acids, and so on (Schmidt, 1982). Among them, peptides in the wasp venom usually exhibit superior pharmacological effects and a promising clinical value (Arifuzzaman et al., 2019; Li et al., 2019).
As a wasp venom peptide, anoplin (1, Figure 1) was isolated from the venom sac of the Japanese solitary spider wasp Anoplius samariensis (Hisada et al., 2000). Anoplin is the shortest, amphipathic, linear α-helical antimicrobial peptide (AMP) with only 10 residues (Gly-Leu-Leu-Lys-Arg-Ile-Lys-Thr-Leu-Leu-NH2) (Konno et al., 2001; Jittikoon, 2015); it also exhibits a wide range of biological activities including antibacterial (Konno et al., 2001; Monincová et al., 2010), mast cell degranulating (Cabrera et al., 2009), antitumor (Zhu et al., 2013; Da Silva et al., 2018; Kai et al., 2018), antimalarial (Carter et al., 2013), antifungal (Jindrichova et al., 2014), and anti-inflammatory activities (Zhong et al., 2020b). Anoplin exerts its functions by direct interaction with anionic bilayers and biological membranes via ion channels (Cabrera et al., 2008; Leung et al., 2011), selectively binding to the bacterial DNA or inhibiting ATP synthase (Syed et al., 2018). Owing to its extremely simple structure and nonhemolytic toxicity, anoplin exhibits superiority in chemical manipulation, structure–activity relationship studies, mechanisms of action, and medical application, which has a great potential as a novel class of drugs for antibiotics and anticancer applications. Given the fact that there is no report on research progress about structural modification of anoplin so far, we have summarized the advances in structural modification and relevant pharmaceutical activities to push the further application of anoplin.
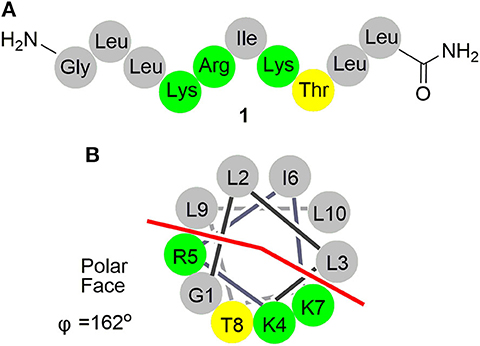
Figure 1. (A) Schematic representation of anoplin (1). (B) Helical wheel diagram of anoplin showing the polar and nonpolar faces of the helix. All residues are drawn in helical configuration. Gray symbols represent hydrophobic residues. Yellow symbols represent polar, uncharged residues. Green symbols represent basic residues.
Structural Modification of Anoplin
Point Mutation and Truncation
The Hansen lab (Ifrah et al., 2005) firstly conducted the structure–function and structure–toxicity relationships research of anoplin by point mutation, C- and N-terminal truncation. Terminus truncations indicated that the full length of decapeptide is necessary for antibacterial activity toward Escherichia coli or Staphylococcus aureus. Then standard Ala-scan revealed Gly-1, Leu-2, Leu-3, Ile-6, Leu-9, and Leu-10 are critical residues. A series of studies of analogs with different residue substitutions showed that hydrophobic anoplin derivatives generally have a lower minimum inhibitory concentration (MIC). On the other hand, it will also have higher hemolytic activity and reduce or reverse the selectivity between E. coli and S. aureus. More importantly, the overall charge of +4 is essential for anoplin to differentiate bacteria and normal cells.
After Meinike et al. verified the substitution of Arg-5 with different hydrophobic amino acids that resulted in potent analogs, they further replaced Arg-5 with peptoid monomers. Among the tests, the therapeutic index (TI; larger values indicate an improved activity specificity) of analogs containing N-(2,2-diphenylethyl) Gly or N-(1-naphthalenemethyl) Gly (2 or 3, Figure 2A) is commendably comparable with that of anoplin, but the selectivity was reversed toward E. coli and S. aureus (Meinike and Hansen, 2009).
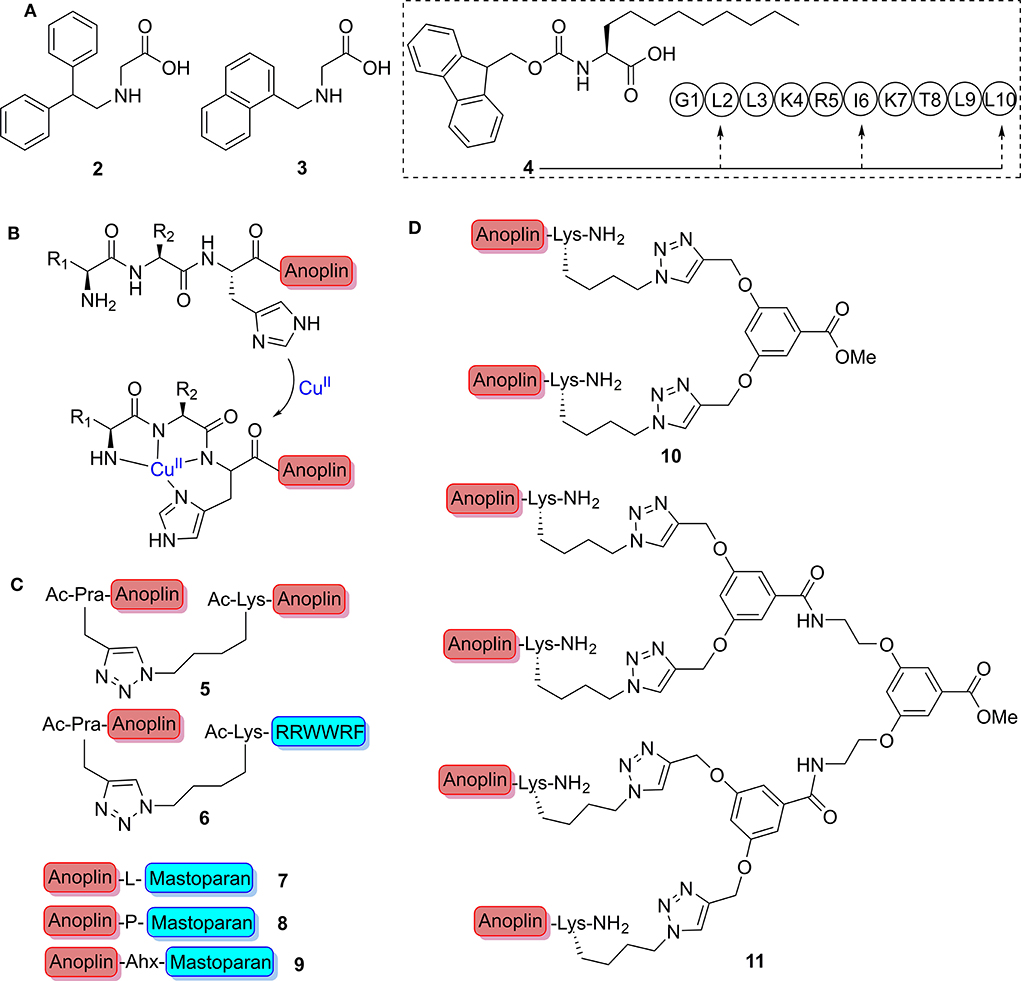
Figure 2. (A) Point mutation of nonnatural amino acid. (B) Schematic representation of the ATCUN-anoplin bound to a Cu2+ ion. (C) Structure of dimeric peptides. (D) The conjugation of anoplin to divalent and tetravalent dendrimers.
To further explore suggested activity-improving discoveries, the Hansen group (Munk et al., 2013) presented 19 new anoplin analogs that substituted in amino acid positions 2, 3, 5, 6, 8, 9, and 10 with Lys, Trp, Phe, β-2-naphthylalanine (2Nal), and β-cyclohexylalanine (Cha). Some discrete substitutions using Lys showed some promise, but Trp and Phe did not provide anoplin analogs with improved microbial specificity or lessened hemolytic activity (Braun and Heijne, 1999; Chen et al., 2005; Schmitt et al., 2007; Wiradharma et al., 2011). This was probably because Trp and Phe in those sites hindered the interaction between membrane and peptides owing to the structural distortions. It was gratifying that the use of 2Nal6 and Cha3 exhibited promising TI against all strains tested. Afterwards, they successfully developed analysis of variance models that describe the relationship between peptide properties (high-performance liquid chromatography retention time, hemolytic property, and MIC) and the structural characteristics of the anoplin analogs by point mutation, and they verified the properties predicted by the mathematical models, which were in reasonable agreement with the measurements (Munk et al., 2014).
Cabrera et al. (2008) synthesized deamidated analog of anoplin to explore the effect of deamidation at the C-terminus, and they found that although anoplin-OH still retained the same α-helical content, it lost the characteristic antimicrobial and mast cell degranulating activities of anoplin. A reasonable explanation was that carboxylation correlated with electrostatic repulsion and amphipathic character. Subsequently, Pripotnev et al. confirmed that C-terminal amide group was important for the structural stabilization, extra charge and function of anoplin, and the physicochemical properties of peptides defined its AMP activity (Pripotnev et al., 2010). However, the amidation of anoplin was not essential for its antifungal and plant defense stimulating activities (Jindrichova et al., 2014).
Won et al. (2011b) applied several biophysical techniques (UV resonance Raman spectroscopy in combination with Langmuir–Blodgett monolayer technique vesicle leakage assay) to study the relationship between physicochemical properties (secondary structure and surface activity) with biological activities of anoplin and its two derivatives-anoplin-8K (GLLKRIKKLL-NH2) and anoplin-1K5V8K (KLLKVIKKLL-NH2). These results indicated that higher helical tendency, stronger amphipathicity, and extra positive charges could be beneficial for higher antimicrobial activity with weak membrane lytic activity.
As a membrane anchor, the length of lipophilic alkyl chain of lipopeptides is considered highly vital for the bioactivity of peptides (Taft and Selitrennikoff, 1990). To increase the membrane affinity of anoplin without sacrificing important positively charged side chains, Slootweg et al. incorporated the lipophilic amino acid, (S)-2-aminoundecanoic acid (4, Figure 2A), which they synthesized into the peptide sequence (Leu-2, Ile-6, and Leu-10) of anoplin (Slootweg et al., 2013). As expected, all the derivatives exhibited enhanced activities and selectivity toward microbial membranes, while the effect of hemolysis showed considerably increase.
To better comprehend the structure–activity relationships of short cationic α-helical AMPs, and to achieve a generally applicable set of guidelines on how to increase AMP activity without concomitant introduction of strong hemolytic activity, Wimmer and the Hansen group (Uggerhoj et al., 2015) presented the NMR structure of anoplin in a micellar environment and systematically set up a vast library of substitutions for antimicrobial activity, hemolytic activity, and changes in structure and lipid interactions. They successfully proposed a do's and don'ts list with the core concept of subtle hydrophobicity increase in the hydrophobic face and the polarity increase in the hydrophilic face, including the following: (1) do not rely on the helical wheel model to design AMP derivatives, especially for nonconservative point mutations; (2) do identify whether each residue interacts with the membrane interior, with lipid head groups, or with the bulk solvent; (3) do not substitute those hydrophobic residues that interact with the lipid head; (4) do increase the polarity of side chains that interact with bulk solvent; and (5) do increase the hydrophobicity of the hydrophobic face in small steps and a few positions. Based on these findings, it is not hard to see that the stronger amphipathicity plays an important role for the activity of anoplin.
N-Terminal Auxiliary
Amino-terminal copper and nickel (ATCUN) binding motifs, H2N-AA1-AA2-His, are known to actively form reactive oxygen species (ROS) upon metal ions binding, and ROS is primarily a result of Cu(II) binding (Harford and Sarkar, 1997; Donaldson et al., 2001; Du et al., 2013) (Figure 2B). The formed ROS can render the bacteria more susceptibility to antimicrobial agents (Fang, 2004). The Angeles-Boza group (Libardo et al., 2014) prepared three ATCUN binding motifs containing derivatives of anoplin (Asp-Ala-His, Gly-Gly-His, and Val-Ile-His), which were found to be more active than the parent anoplin against a panel of clinically relevant bacteria with non-hemolysis through ROS-induced membrane damage. Whereafter, they found two additional ATCUN sequences (Arg-Thr-His and Leu-Lys-His), which produce ·OH and other ROS at faster rates than did other ATCUN complexes. When evaluated by the degree of oxidative stress brought induced by new ATCUN-anoplin conjugates, the Arg-Thr-His-anoplin peptide was up to four times more potent than is anoplin alone against standard test bacteria (Libardo et al., 2015). The metal binding tripeptide motifs provided a simple approach to increase potency of anoplin by conferring a secondary action.
Similar with lipophilic alkyl chain of lipopeptides, acylation of the N-terminus with fatty acid moiety can also increase the membrane affinity and the activity of AMPs (Avrahami and Shai, 2002). So Chionis et al. (2016) incorporated the lipophilic octanoic, decanoic, and dodecanoic acid residues into the N-terminus of anoplin. Those results indicated that fatty acid moiety was good for the membrane affinity, antimicrobial potency, and the higher helical content of the peptides, and these peptides were nontoxic to erythrocytes and stable to proteolysis. In addition, the increase of the length of the lipophilic moiety did not further affect the above properties. Salas et al. (2018) thought that the loss of activity upon truncation of anoplin in the literature (Ifrah et al., 2005) was correlated to lowering of hydrophobicity, so they synthesized and investigated the effects of truncated palmitoylated anoplin analogs, and their results demonstrated that palmitoylation of truncated anoplin analogs can increase antimicrobial activity, and even helicity, in water.
d-Amino Acid Conversion
D-Peptide and D-protein composed of D-amino acids are potential therapeutic agents with longer half-lives or oral possibility in vivo (He et al., 1996; Weinstock et al., 2014). Therefore, D-amino acid substitution is a useful method to improve the enzymatic stability and activity of AMPs. Won et al. (2011a) found that the replacement of all amino acids with their D-stereoisomers did not change bactericidal activity of anoplin, so the antibacterial mechanism is through nonspecific interaction.
Inspired by the experience of Ifrah et al. (2005), Wang et al. (2014) designed and synthesized a group of anoplin analogs by multiple residue substitutions with D-amino acids. Owing to the increased charge, hydrophobicity, and amphiphilicity, anoplin-4 (kllkwwkkll-NH2) composed of D-amino acids displayed the characteristics of the highest antimicrobial activity in vivo, higher proteolytic stability, and lower toxicity to normal cells, which make anoplin-4 a great candidate for future optimization and treatment of infection.
Dimerization and Multivalent Presentation
Hybrid peptide analogs can effectively combine the therapeutic advantages of different peptides and achieve collaborative treatments (Liu et al., 2013; Kamysz et al., 2015; Le et al., 2015). Hexapeptide, RRWWRF (named RW), is a cationic peptide derived from the screening of a hexapeptide combinatorial library; it is beneficial to interact with the negatively charged components of the bacterial membrane (Haug et al., 2008). Liu et al. (2017) successfully synthesized the homodimeric peptide 5 and the heterodimeric peptide 6 by copper(I) catalyzed azide-alkyne cycloaddition (CuAAC “click chemistry”) (Figure 2C); the dimer peptides not only had significantly enhanced antimicrobial activity against multidrug-resistant (MDR) bacteria in vitro and in vivo but also showed synergy and additivity effects when used in combination with conventional antibiotics rifampin or penicillin.
To break α-helix and obtain greater flexibility of heterodimeric AMPs, Kai et al. (2018) used different amino acids (7–9, Figure 2C), including Leu, Pro, and Ahx (aminocaproic acid), as linker to connect anoplin and mastoparan, another α-helical AMP from Vespula lewisii venom. Findings manifested that Pro and Ahx contributed to the design of ideal dimer AMPs with high selectivity and potency while reducing lytic activity with respect to red blood cells. However, Leu cannot confer increased flexibility to heterodimeric AMPs. It is flexible heterodimeric structure induced by Pro and Ahx that improved the potency of heterodimeric AMPs for negatively charged membranes while reducing interaction with zwitterionic membranes. As can be seen, dimerization offers an effective strategy to screen for the development of novel antimicrobial agents.
Given the toxicity, the short half-life in vivo, and stimulation of an immune response of AMPs, multivalent AMPs formed by attaching several peptide monomers to reactive polymer scaffolds via naturally occurring intermolecular disulfide bridges or unnatural scaffold linkers have been used to overcome the problems mentioned above (Liu et al., 2010). Chamorro et al. showed that anoplin was successfully conjugated via click chemistry to divalent and tetravalent dendrimers (10 and 11, Figure 2D) (Chamorro et al., 2012). Compounds 10 and 11 clearly resulted in enhanced pore formation of anoplin.
Chitosan is a natural polymer comprised of β-(1-4)-linked glucosamine and some degree of N-acetyl glucosamine with low antimicrobial activity and has been widely applied in the field of biomedicine. Sahariah et al. (2015) reported that grafting of anoplin to chitosan polymers was a rational design for abolishing the hemolytic propensity and increasing the activity of the parent peptide; at the same time, the anoplin–chitosan conjugates had a high degree of control over the resulting peptide grafting density (12, Figure 3).
Combined Modification
Combination of multiple modification methods may represent more effective strategies with their respective advantages. Based on the substitutive anoplin sequence Ano-D4, 7 (13, Figure 4A), GLLkRIkTLL-NH2 (lowercase letters indicate the D-enantiomer), further modifications were carried out to systematically screen excellent AMPs with optimal therapy potential. Zhong et al. (2020b) conjugated various lengths of fatty acid chains ranging from 4 to 16 carbons onto the side chain of the position 4 or 7 D-amino acid of Ano-D4, 7 (Figure 4B). The antimicrobial activity of those new peptides was highly correlated with the lengths of the fatty acid chain. When the length of the fatty acid chain was ≤12 carbons, there was a remarkable increase in the MIC values of the new peptides after the hydrophobicity went beyond preceding threshold. Interestingly, the peptides conjugated with the same fatty acid chain (≤12 carbons) at position 7 of Ano-D4, 7, exhibited better activity than the corresponding peptide with the modification at position 4. This is probably because side chains of Arg-5 and Thr-8 shielded the fatty acid on the position 4 D-Lys side chain. These peptides also significantly reduced the bacterial load in mice without obvious adverse reactions or any death at the effective dose.
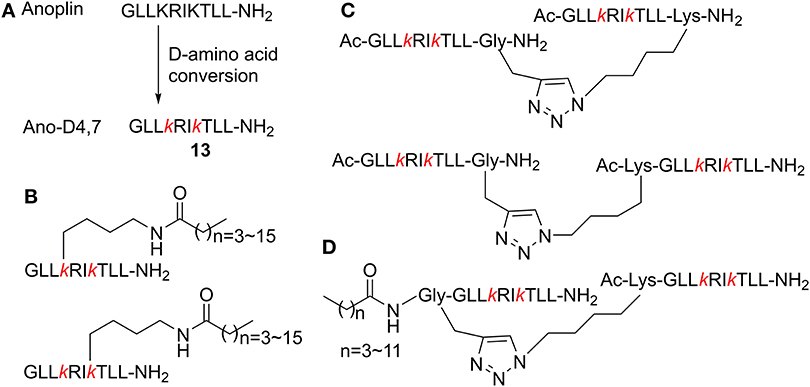
Figure 4. (A) D-Amino acid conversion of anoplin to Ano-D4, 7. (B) Antimicrobial peptides conjugated with fatty acids on the side chain of Ano-D4, 7. (C) Different dimerization positions based on Ano-D4, 7. (D) N-terminal fatty acid modified-dimer Ano-D4, 7.
According to previous studies on dimeric modification, Zhong et al. (2020a) further inquired the effects of different dimerization positions on biological activity. They found that the anti-biofilm activity of the C- and N-terminal dimer peptides was better than C–C terminal ones (Figure 4C). Meanwhile, new peptides combining D-amino acid conversion and dimerization displayed higher TI without hemolytic activity. In addition, on the basis of the previous two reports, they designed dimer peptide conjugated fatty acids at the N-terminus of Ano-D4, 7 and dimerization (Figure 4D) (Zhong et al., 2019). The multi-modified analogs were more inclined to present β-strand structure; this pre-assembled state of the dimer peptides led to fast interaction without the demand for extra aggregation process, which caused more efficient permeabilization. Furthermore, the previous peptides also exerted high stability toward protease, serum, salts, and different pH environments, which proved that combined modification techniques provided more flexible approaches to develop novel AMPs.
Potential Development Direction of Anoplin
Conformational Constraint
Despite the above notable advances, there are still many disadvantages in the previous modifications. For example, residue substitutions cannot solve the low stability toward protease, and there also may be metabolic problems for D-peptide in vivo compared with natural amino acid. The synthesis of dimerization and multivalent presentation are difficult and tedious. A promising alternative approach would constrain α-helical conformation by stapling chemistry (Schafmeister et al., 2000; Walensky et al., 2004; Moellering et al., 2009; Walensky and Bird, 2014) (Figure 5A). The first stapled peptide, ALRN-6924, is currently in phase II trials to treat advanced solid tumors or lymphomas in connection with MDM2 and MDMX (Carvajal et al., 2018; Ng et al., 2018). We have previously reported the design and synthesis of a series of hydrocarbon stapled melittin peptides of which, some analogs showed remarkable enhancement not merely in antihepatoma activity but also in α-helicity and protease resistance (Wu et al., 2017a). Subsequently, we developed a new series of stapling amino acids, which contained the native amino acid side chains to expand the scope of the all-hydrocarbon stapled peptide strategy (Wu et al., 2017b).
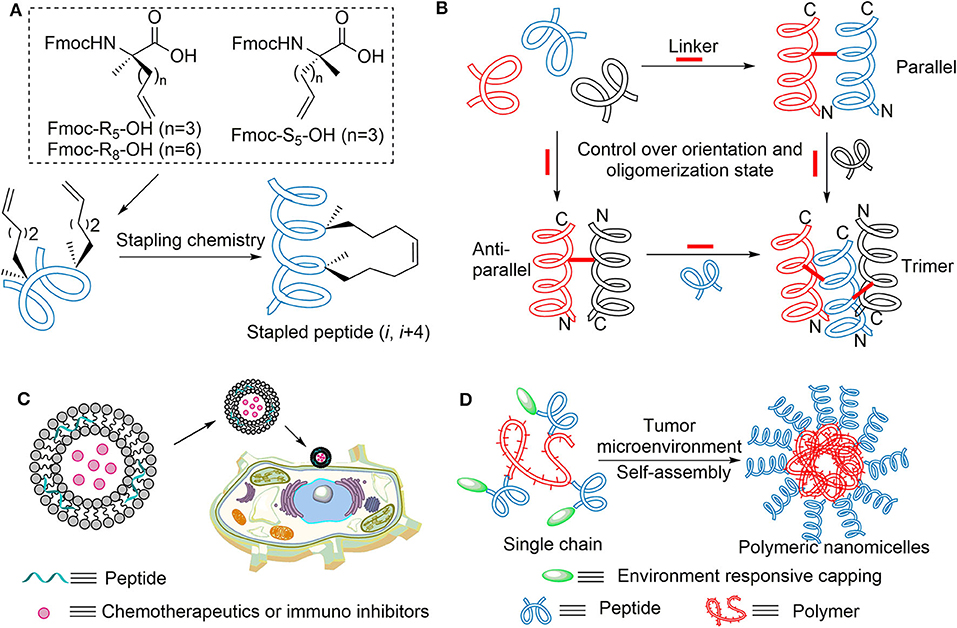
Figure 5. (A) All-hydrocarbon stapled peptide strategy. (B) Strategies to induce helix dimer and trimer formation of designed peptides. N and C designations indicate the amino and carboxy termini in the helix models, respectively. (C) Targeted delivery system based on bioactive peptides. (D) Self-assembly of polymer–peptide conjugates in tumor microenvironment.
In addition to α-helical constraint of single short peptide, nature actually exhibits its own approach to maintain the structural integrity of proteins and protect natural peptides from enzymolysis. A noteworthy example was the self-assembly of β-sheet peptides into amyloid-β protein by inter-strand hydrophobic interactions, which exhibited extreme resistance toward intracellular proteases (Barz et al., 2018). Inspired by this, the Wu group reported that crosslinked helix dimers can be constructed by two disulfide bonds for ultrahigh proteolytic stability (Chen et al., 2015). To overcome the instability of disulfide linkage under the intracellular reducing condition, Wuo et al. developed more judicious covalent bonds like bis-triazole linkers (Wuo et al., 2015) and hexafluoroisopropanol-based bisthioether crosslinkers (Wuo et al., 2018; Chen et al., 2019) (Figure 5B). We expect these effective strategies be used to modify anoplin with preferable biological function.
Targeted Delivery System
Bio-functional nanosystems are also regarded as novel strategies to address some drawbacks of peptides (Aronson et al., 2018). Through the widely accepted enhanced permeability and retention (ERP) effect (He J. Y. et al., 2019), drug delivery platforms based on cytotoxic peptides possessed prolonged circulation time and targeting capability, which improve its therapeutic utility and reduce side effects. More importantly, this technology can achieve co-delivery of multiple drugs for the purpose of combination therapy in precision medicine (Jin et al., 2018) (Figure 5C). To obtain deeper solid-tumor penetration and perfuse more homogeneously within tumor tissue, one ingenious design is tumor microenvironment (TME)-induced in situ self-assembly of polymer–peptide conjugates (Cong et al., 2019) (Figure 5D); this transformation-enhanced accumulation and retention (TEAR) effect showed higher anticancer activity than the ERP effect of traditional nanoparticles (He P. P. et al., 2019).
Conclusion and Outlook
The emergence of MDR bacteria and the markedly declining supply of safe and efficacious antibiotics have made it urgent to develop new antibiotics. On the other hand, MDR malignant tumor is the major threat to public health. In this context, natural AMPs have been considered as promising strategy for these two disease threats because of the lower potency of resistance for bacteria and tumor toward AMPs (Felício et al., 2017; Mishra et al., 2017). Owing to typical non-receptor-mediated membrane mechanisms (Sveinbjornsson et al., 2017), anoplin possesses more particular superiority than do conventional antibiotics and chemotherapeutics. In addition, anoplin offers great advantages with simple structure and broad-spectrum activity, especially nonhemolytic toxicity. With the anoplin in hand, it is more convenient to obtain lead compounds for potential translational research. We hope that anoplin and its derivatives will inspire the advancement of AMPs as a novel class of drug candidates.
Author Contributions
YW and RH wrote the manuscript. J-MJ and L-JZ contributed to the creation of most figures in this manuscript. HZ and H-ZC contributed to the editing of this manuscript. L-LC and XL revised and edited the final manuscript. All authors contributed to reference collection, selection, and final proof.
Funding
This work was supported by funds from the National Natural Science Foundation of China (No. 81903654), Program for Professor of Special Appointment (Young Eastern Scholar) at Shanghai Institutions of Higher Learning (QD2018035), Shanghai Chenguang Program of Education Commission of Shanghai Municipality (No. 18CG46), and Shanghai Sailing Program (No. 19YF1449400) of Science and Technology Commission of Shanghai Municipality, National Key Subject of Drug Innovation (2019ZX09201005-007), National Key R&D program for key research project of modernization of traditional Chinese medicine (2019YFC1711602), and Graduate Innovation Training research project of Shanghai University of Traditional Chinese Medicine (No. Y2019064).
Conflict of Interest
The authors declare that the research was conducted in the absence of any commercial or financial relationships that could be construed as a potential conflict of interest.
References
Argiolas, A., and Pisano, J. J. (1985). Bombolitins, a new class of mast cell degranulating peptides from the venom of the bumblebee Megabombus pennsylvanicus. J. Biol. Chem. 260, 1437–1444. doi: 10.1016/0196-9781(85)90410-3
Arifuzzaman, M., Mobley, Y. R., Choi, H. W., Bist, P., Salinas, C. A., Brown, Z. D., et al. (2019). MRGPR-mediated activation of local mast cells clears cutaneous bacterial infection and protects against reinfection. Sci. Adv. 5:eaav0216. doi: 10.1126/sciadv.aav0216
Aronson, M. R., Simonson, A. W., Orchard, L. M., Llinas, M., and Medina, S. H. (2018). Lipopeptisomes: anticancer peptide-assembled particles for fusolytic oncotherapy. Acta. Biomater. 80, 269–277. doi: 10.1016/j.actbio.2018.09.025
Avrahami, D., and Shai, Y. (2002). Conjugation of a magainin analogue with lipophilic acids controls hydrophobicity, solution assembly, and cell selectivity. Biochemistry 41, 2254–2263. doi: 10.1021/bi011549t
Barz, B., Liao, Q. H., and Strodel, B. (2018). Pathways of amyloid-β aggregation depend on oligomer shape. J. Am. Chem. Soc. 140, 319–327. doi: 10.1021/jacs.7b10343
Braun, P., and Heijne, G. V. (1999). The aromatic residues Trp and Phe have different effects on the positioning of a transmembrane helix in the microsomal membrane. Biochemistry 38, 9778–9782. doi: 10.1021/bi990923a
Cabrera, M. P. D. S., Arcisio-Miranda, M., Costa, L. C. D., Souza, B. M. D., Costa, S. T. B., Palma, M. S., et al. (2009). Interactions of mast cell degranulating peptides with model membranes: a comparative biophysical study. Arch. Biochem. Biophys. 486, 1–11. doi: 10.1016/j.abb.2009.03.009
Cabrera, M. P. D. S., Arcisio-Miranda, M., Costa, S. T. B., Konno, K., Ruggiero, J. R., Procopio, J., et al. (2008). Study of the mechanism of action of anoplin, a helical antimicrobial decapeptide with ion channel-like activity, and the role of the amidated C-terminus. J. Pept. Sci. 14, 661–669. doi: 10.1002/psc.960
Carter, V., Underhill, A., Baber, I., Sylla, L., Baby, M., Larget-Thiery, I., et al. (2013). Killer bee molecules: antimicrobial peptides as effector molecules to target sporogonic stages of Plasmodium. PLoS Pathog. 9:e1003790. doi: 10.1371/journal.ppat.1003790
Carvajal, L. A., Neriah, D. B., Senecal, A., Benard, L., Thiruthuvanathan, V., Yatsenko, T., et al. (2018). Dual inhibition of MDMX and MDM2 as a therapeutic strategy in leukemia. Sci. Transl. Med. 10:eaao3003. doi: 10.1126/scitranslmed.aao3003
Chamorro, C., Boerman, M. A., Arnusch, C. J., Breukink, E., and Pieters, R. J. (2012). Enhancing membrane disruption by targeting and multivalent presentation of antimicrobial peptides. Biochim. Biophys. Acta 1818, 2171–2174. doi: 10.1016/j.bbamem.2012.04.004
Chen, Y. Q., Liang, J. J., Li, T., Lin, P., Zhao, Y. B., and Wu, C. L. (2019). Interchain doubly-bridged α-helical peptides for the development of protein binders. Chin. Chem. Lett. 30, 924–928. doi: 10.1016/j.cclet.2019.02.013
Chen, Y. Q., Yang, C. Q., Li, T., Zhang, M., Liu, Y., Gauthier, M. A., et al. (2015). The interplay of disulfide bonds, α-helicity, and hydrophobic interactions leads to ultrahigh proteolytic stability of peptides. Biomacromolecules 16, 2347–2355. doi: 10.1021/acs.biomac.5b00567
Chen, Y. X., Mant, C. T., Farmer, S. W., Hancock, R. E. W., Vasil, M. L., and Hodges, R. S. (2005). Rational design of α-helical antimicrobial peptides with enhanced activities and specificity/therapeutic index. J. Biol. Chem. 280, 12316–12329. doi: 10.1074/jbc.M413406200
Chionis, K., Krikorian, D., Koukkou, A. I., Sakarellos-Daitsiotis, M., and Panou-Pomonis, E. (2016). Synthesis and biological activity of lipophilic analogs of the cationic antimicrobial active peptide anoplin. J. Pept. Sci. 22, 731–736. doi: 10.1002/psc.2939
Cong, Y., Ji, L., Gao, Y. J., Liu, F. H., Cheng, D. B., Hu, Z. Y., et al. (2019). Microenvironment-induced in situ self-assembly of polymer-peptide conjugates that attack solid tumors deeply. Angew. Chem. Int. Ed. 58, 4632–4637. doi: 10.1002/anie.201900135
Da Silva, A. M. B., Silva-Gonçalves, L. C., Oliveira, F. A., and Arcisio-Miranda, M. (2018). Pro-necrotic activity of cationic mastoparan peptides in human glioblastoma multiforme cells via membranolytic action. Mol. Neurobiol. 55, 5490–5504. doi: 10.1007/s12035-017-0782-1
Donaldson, L. W., Skrynnikov, N. R., Choy, W. Y., Muhandiram, D. R., Sarkar, B., Forman-Kay, J. D., et al. (2001). Structural characterization of proteins with an attached ATCUN motif by paramagnetic relaxation enhancement NMR spectroscopy. J. Am. Chem. Soc. 123, 9843–9847. doi: 10.1021/ja011241p
Du, X. B., Li, H. Y., Wang, X. H., Liu, Q., Ni, J. Z., and Sun, H. Z. (2013). Kinetics and thermodynamics of metal binding to the N-terminus of a human copper transporter, hCTR1. Chem. Commun. 49, 9134–9136. doi: 10.1039/c3cc45360j
Fang, F. C. (2004). Antimicrobial reactive oxygen and nitrogen species: concepts and controversies. Nat. Rev. Microbiol. 2, 820–832. doi: 10.1038/nrmicro1004
Felício, M. R., Silva, O. N., Gonçalves, S., Santos, N. C., and Franco, O. L. (2017). Peptides with dual antimicrobial and anticancer activities. Front. Chem. 5:5. doi: 10.3389/fchem.2017.00005
Harford, C., and Sarkar, B. (1997). Amino terminal Cu(II)- and Ni(II)-binding (ATCUN) motif of proteins and peptides: metal binding, DNA cleavage, and other properties. Acc. Chem. Res. 30, 123–130. doi: 10.1021/ar9501535
Haug, B. E., Stensen, W. S., Kalaaji, M., Rekdal, Ø., and Svendsen, J. S. (2008). Synthetic antimicrobial peptidomimetics with therapeutic potential. J. Med. Chem. 51, 4306–4314. doi: 10.1021/jm701600a
He, J. Y., Li, C. C., Ding, L., Huang, Y., Yin, X. L., Zhang, J. F., et al. (2019). Tumor targeting strategies of smart fluorescent nanoparticles and their applications in cancer diagnosis and treatment. Adv. Mater. 31:e1902409. doi: 10.1002/adma.201902409
He, P. P., Li, X. D., Wang, L., and Wang, H. (2019). Bispyrene-based self-assembled nanomaterials: in vivo self-assembly, transformation, and biomedical effects. Acc. Chem. Res. 52, 367–378. doi: 10.1021/acs.accounts.8b00398
He, Y. L., Murby, S., Gifford, L., Collett, A., Warhurst, G., Douglas, K. T., et al. (1996). Oral absorption of D-oligopeptides in rats via the paracellular route. Pharm. Res. 13, 1673–1678. doi: 10.1023/A:1016440707092
Hisada, M., Konno, K., Itagaki, Y., Naoki, H., and Nakajima, T. (2000). Advantages of using nested collision induced dissociation/post-source decay with matrix-assisted laser desorption/ionization time-of-flight mass spectrometry: sequencing of novel peptides from wasp venom. Rapid Commun. Mass. Spectrom. 14, 1828–1834. doi: 10.1002/1097-0231(20001015)14:19<1828::AID-RCM101>3.0.CO;2-G
Hou, C. S., Guo, L. Q., You, L. F., Wang, J. R., Lin, J. F., Wu, W. H., et al. (2014). Molecular cloning and characterization of a venom phospholipase A2 from Apis mellifera spp. J. Entomol. Res. Soc. 16, 55–66.
Ifrah, D., Doisy, X., Ryge, T. S., and Hansen, P. R. (2005). Structure-activity relationship study of anoplin. J. Pept. Sci. 11, 113–121. doi: 10.1002/psc.598
Jin, H. L., Wan, C., Zou, Z. W., Zhao, G. F., Zhang, L. L., Geng, Y. Y., et al. (2018). Tumor ablation and therapeutic immunity induction by an injectable peptide hydrogel. ACS Nano 12, 3295–3310. doi: 10.1021/acsnano.7b08148
Jindrichova, B., Burketova, L., and Novotna, Z. (2014). Novel properties of antimicrobial peptide anoplin. Biochem. Biophys. Res. Commun. 444, 520–524. doi: 10.1016/j.bbrc.2014.01.097
Jittikoon, J. (2015). Short native α-helical cationic antimicrobial peptides: promising alternative antibiotics. Thai. J. Pharm. Sci. 39, 1–9.
Kai, M., Zhang, W., Xie, H., Liu, L. W., Huang, S. J., Li, X., et al. (2018). Effects of linker amino acids on the potency and selectivity of dimeric antimicrobial peptides. Chin. Chem. Lett. 29:1163–1166. doi: 10.1016/j.cclet.2018.04.011
Kamysz, E., Sikorska, E., Dawgul, M., Tyszkowski, R., and Kamysz, W. (2015). Influence of dimerization of lipopeptide Laur-Orn-Orn-Cys–NH2 and an N-terminal peptide of human lactoferricin on biological activity. Int. J. Pept. Res. Ther. 21, 39–46. doi: 10.1007/s10989-014-9423-y
Konno, K., Hisada, M., Fontana, R., Lorenzi, C. C., Naoki, H., Itagaki, Y., et al. (2001). Anoplin, a novel antimicrobial peptide from the venom of the solitary wasp Anoplius samariensis. Biochim. Biophys. Acta 1550, 70–80. doi: 10.1016/S0167-4838(01)00271-0
Le, C. F., Yusof, M. Y. M., Hassan, H., and Sekaran, S. D. (2015). In vitro properties of designed antimicrobial peptides that exhibit potent antipneumococcal activity and produces synergism in combination with penicillin. Sci. Rep. 5:9761. doi: 10.1038/srep09761
Leung, B. O., Hitchcock, A. P., Won, A., Ianoul, A., and Scholl, A. (2011). Imaging interactions of cationic antimicrobial peptides with model lipid monolayers using X-ray spectromicroscopy. Eur. Biophys. J. 40, 805–810. doi: 10.1007/s00249-011-0690-7
Li, G. Z., Lei, Q. F., Wang, F., Deng, D. S., Wang, S. P., Tian, L. L., et al. (2019). Fluorinated polymer mediated transmucosal peptide delivery for intravesical instillation therapy of bladder cancer. Small 15:e1900936. doi: 10.1002/smll.201900936
Libardo, M. D., Cervantes, J. L., Salazar, J. C., and Angeles-Boza, A. M. (2014). Improved bioactivity of antimicrobial peptides by addition of amino-terminal copper and nickel (ATCUN) binding motifs. ChemMedChem 9, 1892–1901. doi: 10.1002/cmdc.201402033
Libardo, M. D., Nagella, S., Lugo, A., Pierce, S., and Angeles-Boza, A. M. (2015). Copper-binding tripeptide motif increases potency of the antimicrobial peptide Anoplin via Reactive Oxygen Species generation. Biochem. Biophys. Res. Commun. 456, 446–451. doi: 10.1016/j.bbrc.2014.11.104
Liu, B. J., Huang, H. F., Yang, Z. B., Liu, B. Y., Gou, S. H., Zhong, C., et al. (2017). Design of novel antimicrobial peptide dimer analogues with enhanced antimicrobial activity in vitro and in vivo by intermolecular triazole bridge strategy. Peptides 88, 115–125. doi: 10.1016/j.peptides.2016.12.016
Liu, S. P., Zhou, L., Lakshminarayanan, R., and Beuerman, R. W. (2010). Multivalent antimicrobial peptides as therapeutics: design principles and structural diversities. Int. J. Pept. Res. Ther. 16, 199–213. doi: 10.1007/s10989-010-9230-z
Liu, Y. F., Xia, X., Xu, L., and Wang, Y. Z. (2013). Design of hybrid β-hairpin peptides with enhanced cell specificity and potent anti-inflammatory activity. Biomaterials 34, 237–250. doi: 10.1016/j.biomaterials.2012.09.032
Meinike, K., and Hansen, P. R. (2009). Peptoid analogues of anoplin show antibacterial activity. Protein Pept. Lett. 16, 1006–1011. doi: 10.2174/092986609789055421
Mishra, B., Reiling, S., Zarena, D., and Wang, G. (2017). Host defense antimicrobial peptides as antibiotics: design and application strategies. Curr. Opin. Chem. Biol. 38, 87–96. doi: 10.1016/j.cbpa.2017.03.014
Moellering, R. E., Cornejo, M., Davis, T. N., Del, B. C., Aster, J. C., Blacklow, S. C., et al. (2009). Direct inhibition of the NOTCH transcription factor complex. Nature 462, 182–188. doi: 10.1038/nature08543
Monincová, L., Buděšínský, M., Slaninová, J., Hovorka, O., Cvačka, J., Voburka, Z., et al. (2010). Novel antimicrobial peptides from the venom of the eusocial bee Halictus sexcinctus (Hymenoptera: Halictidae) and their analogs. Amino Acids 39, 763–775. doi: 10.1007/s00726-010-0519-1
Monteiro, M. C., Romão, P. R., and Soares, A. M. (2009). Pharmacological perspectives of wasp venom. Protein Pept. Lett. 16, 944–952. doi: 10.2174/092986609788923275
Munk, J. K., Ritz, C., Fliedner, F. P., Frimodt-Moller, N., and Hansen, P. R. (2014). Novel method to identify the optimal antimicrobial peptide in a combination matrix, using anoplin as an example. Antimicrob. Agents Chemother. 58, 1063–1070. doi: 10.1128/AAC.02369-13
Munk, J. K., Uggerhoj, L. E., Poulsen, T. J., Frimodt-Moller, N., Wimmer, R., Nyberg, N. T., et al. (2013). Synthetic analogs of anoplin show improved antimicrobial activities. J. Pept. Sci. 19, 669–675. doi: 10.1002/psc.2548
Ng, S. Y., Yoshida, N., Christie, A. L., Ghandi, M., Dharia, N. V., Dempster, J., et al. (2018). Targetable vulnerabilities in T- and NK-cell lymphomas identified through preclinical models. Nat. Commun. 9:2024. doi: 10.1038/s41467-018-04356-9
Pripotnev, S., Won, A., and Ianoul, A. (2010). The effects of L- to D-isomerization and C-terminus deamidation on the secondary structure of antimicrobial peptide Anoplin in aqueous and membrane mimicking environment. J. Raman Spectrosc. 41, 1355–1359. doi: 10.1002/jrs.2608
Sahariah, P., Sorensen, K. K., Hjalmarsdottir, M. A., Sigurjonsson, O. E., Jensen, K. J., Masson, M., et al. (2015). Antimicrobial peptide shows enhanced activity and reduced toxicity upon grafting to chitosan polymers. Chem. Commun. 51, 11611–11614. doi: 10.1039/C5CC04010H
Salas, R. L., Garcia, J., Miranda, A., Rivera, W. L., Nellas, R. B., and Sabido, P. (2018). Effects of truncation of the peptide chain on the secondary structure and bioactivities of palmitoylated anoplin. Peptides 104, 7–14. doi: 10.1016/j.peptides.2018.03.019
Schafmeister, C. E., Julia Po, A., and Verdine, G. L. (2000). An all-hydrocarbon cross-linking system for enhancing the helicity and metabolic stability of peptides. J. Am. Chem. Soc. 122, 5891–5892. doi: 10.1021/ja000563a
Schmidt, J. O. (1982). Biochemistry of insect venoms. Ann. Rev. Entomol. 27, 339–368. doi: 10.1146/annurev.en.27.010182.002011
Schmitt, M. A., Weisblum, B., and Gellman, S. H. (2007). Interplay among folding, sequence, and lipophilicity in the antibacterial and hemolytic activities of alpha/beta-peptides. J. Am. Chem. Soc. 129, 417–428. doi: 10.1021/ja0666553
Slootweg, J. C., van Schaik, T. B., Quarles, V. U. H., Breukink, E., Liskamp, R. M., and Rijkers, D. T. (2013). Improving the biological activity of the antimicrobial peptide anoplin by membrane anchoring through a lipophilic amino acid derivative. Bioorg. Med. Chem. Lett. 23, 3749–3752. doi: 10.1016/j.bmcl.2013.05.002
Sveinbjornsson, B., Camilio, K. A., Haug, B. E., and Rekdal, Ø. (2017). LTX-315: a first-in-class oncolytic peptide that reprograms the tumor microenvironment. Future Med. Chem. 9, 1339–1344. doi: 10.4155/fmc-2017-0088
Syed, H., Tauseef, M., and Ahmad, Z. (2018). A connection between antimicrobial properties of venom peptides and microbial ATP synthase. Int. J. Biol. Macromol. 119, 23–31. doi: 10.1016/j.ijbiomac.2018.07.146
Taft, C. S., and Selitrennikoff, C. P. (1990). Cilofungin inhibition of (1-3)-β-glucan synthase: the lipophilic side chain is essential for inhibition of enzyme activity. J. Antibiot. 43, 433–437. doi: 10.7164/antibiotics.43.433
Tsai, I. H., Wang, Y. M., Cheng, A. C., Starkov, V., Osipov, A., Nikitin, I., et al. (2011). cDNA cloning, structural, and functional analyses of venom phospholipases A2 and a Kunitz-type protease inhibitor from steppe viper Vipera ursinii renardi. Toxicon 57, 332–341. doi: 10.1016/j.toxicon.2010.12.012
Uggerhoj, L. E., Poulsen, T. J., Munk, J. K., Fredborg, M., Sondergaard, T. E., Frimodt-Moller, N., et al. (2015). Rational design of alpha-helical antimicrobial peptides: do's and don'ts. Chembiochem 16, 242–253. doi: 10.1002/cbic.201402581
Walensky, L. D., and Bird, G. H. (2014). Hydrocarbon-stapled peptides:principles, practice,and progress: miniperspective. J. Med. Chem. 57, 6275–6288. doi: 10.1021/jm4011675
Walensky, L. D., Kung, A. L., Escher, I., Malia, T. J., Barbuto, S., Wright, R. D., et al. (2004). Activation of apoptosis in vivo by a hydrocarbon-stapled BH3 helix. Science 305, 1466–1470. doi: 10.1126/science.1099191
Wang, Y., Chen, J. B., Zheng, X., Yang, X. L., Ma, P. P., Cai, Y., et al. (2014). Design of novel analogues of short antimicrobial peptide anoplin with improved antimicrobial activity. J. Pept. Sci. 20, 945–951. doi: 10.1002/psc.2705
Weinstock, M. T., Jacobsen, M. T., and Kay, M. S. (2014). Synthesis and folding of a mirror-image enzyme reveals ambidextrous chaperone activity. Proc. Natl. Acad. Sci. U. S. A. 111, 11679–11684. doi: 10.1073/pnas.1410900111
Wiradharma, N., Khoe, U., Hauser, C. A., Seow, S. V., Zhang, S., and Yang, Y. Y. (2011). Synthetic cationic amphiphilic α-helical peptides as antimicrobial agents. Biomaterials 32, 2204–2212. doi: 10.1016/j.biomaterials.2010.11.054
Won, A., Khan, M., Gustin, S., Akpawu, A., Seebun, D., Avis, T. J., et al. (2011a). Investigating the effects of L- to D-amino acid substitution and deamidation on the activity and membrane interactions of antimicrobial peptide anoplin. Biochim. Biophys. Acta 1808, 1592–1600. doi: 10.1016/j.bbamem.2010.11.010
Won, A., Pripotnev, S., Ruscito, A., and Ianoul, A. (2011b). Effect of point mutations on the secondary structure and membrane interaction of antimicrobial peptide anoplin. J. Phys. Chem. B 115, 2371–2379. doi: 10.1021/jp108343g
Wu, Y., Han, M. F., Liu, C., Liu, T. Y., Feng, Y. F., Zou, Y., et al. (2017a). Design, synthesis, and antiproliferative activities of stapled melittin peptides. RSC Adv. 7, 17514–17518. doi: 10.1039/C6RA26427A
Wu, Y., Li, Y. H., Li, X., Zou, Y., Liao, H. L., Liu, L., et al. (2017b). A novel peptide stapling strategy enables the retention of ring-closing amino acid side chains for the Wnt/β-catenin signalling pathway. Chem. Sci. 8, 7368–7373. doi: 10.1039/C7SC02420G
Wuo, M. G., Hong, S. H., Singh, A., and Arora, P. S. (2018). Synthetic control of tertiary helical structures in short peptides. J. Am. Chem. Soc. 140, 16284–16290. doi: 10.1021/jacs.8b10082
Wuo, M. G., Mahon, A. B., and Arora, P. S. (2015). An effective strategy for stabilizing minimal coiled coil mimetics. J. Am. Chem. Soc. 137, 11618–11621. doi: 10.1021/jacs.5b05525
Yan, H. L., Chen, W. L., Chen, L. L., and Ren, L. (2011). A novel bioactive peptide with myotropic activity from wasp venoms. Chin. J. Nat. Med. 9, 317–320. doi: 10.1016/S1875-5364(11)60069-1
Zhong, C., Gou, S. H., Liu, T. Q., Zhu, Y. W., Zhu, N. Y., Liu, H., et al. (2020a). Study on the effects of different dimerization positions on biological activity of partial d-Amino acid substitution analogues of Anoplin. Microb. Pathog. 139:103871. doi: 10.1016/j.micpath.2019.103871
Zhong, C., Liu, T. Q., Gou, S. H., He, Y. T., Zhu, N. Y., Zhu, Y. W., et al. (2019). Design and synthesis of new N-terminal fatty acid modified-antimicrobial peptide analogues with potent in vitro biological activity. Eur. J. Med. Chem. 182:111636. doi: 10.1016/j.ejmech.2019.111636
Zhong, C., Zhu, N. Y., Zhu, Y. W., Liu, T. Q., Gou, S. H., Xie, J. Q., et al. (2020b). Antimicrobial peptides conjugated with fatty acids on the side chain of D-amino acid promises antimicrobial potency against multidrug-resistant bacteria. Eur. J. Pharm. Sci. 141:105123. doi: 10.1016/j.ejps.2019.105123
Keywords: anoplin, structural modification, structure–activity relationship, antimicrobial activity, antitumor activity
Citation: Wu Y, Huang R, Jin J-M, Zhang L-J, Zhang H, Chen H-Z, Chen L-L and Luan X (2020) Advances in the Study of Structural Modification and Biological Activities of Anoplin. Front. Chem. 8:519. doi: 10.3389/fchem.2020.00519
Received: 20 March 2020; Accepted: 19 May 2020;
Published: 07 July 2020.
Edited by:
Laurent G. Désaubry, Centre National de la Recherche Scientifique (CNRS), FranceReviewed by:
Katsuhiro Konno, University of Toyama, JapanAlfredo M. Angeles-Boza, University of Connecticut, United States
Copyright © 2020 Wu, Huang, Jin, Zhang, Zhang, Chen, Chen and Luan. This is an open-access article distributed under the terms of the Creative Commons Attribution License (CC BY). The use, distribution or reproduction in other forums is permitted, provided the original author(s) and the copyright owner(s) are credited and that the original publication in this journal is cited, in accordance with accepted academic practice. No use, distribution or reproduction is permitted which does not comply with these terms.
*Correspondence: Li-Li Chen, bGxjaGVuJiN4MDAwNDA7c2h1dGNtLmVkdS5jbg==; Xin Luan, bHVhbnhpbiYjeDAwMDQwO3NodXRjbS5lZHUuY24=