- 1The Key Laboratory for Green Processing of Chemical Engineering of Xinjiang Bingtuan, School of Chemistry and Chemical Engineering, Shihezi University, Shihezi, China
- 2Key Laboratory of Material Chemistry for Energy Conversion and Storage, Ministry of Education, Hubei Key Laboratory of Material Chemistry and Service Failure, School of Chemistry and Chemical Engineering, Huazhong University of Science and Technology, Wuhan, China
- 3Laboratory of Green S.O.C., Dipartimento di Chimica, Biologia e Biotecnologie, Università degli Studi di Perugia, Perugia, Italy
An iodine/DMSO catalyzed selective cyclization of N-tosylhydrazones with sulfur without adding external oxidant was developed for the synthesis of 4-aryl-1,2,3-thiadiazoles. In this reaction, oxidation of HI by using DMSO as dual oxidant and solvent is the key, which allowed the regeneration of I2, ensuring thus the success of the synthesis. This protocol features by simple operation, high step-economy (one-pot fashion), broad substrate scope as well as scale-up ability.
Introduction
1,2,3-Thiadiazole, as an important 2N1S-heterocyclic structural unit, is ubiquitous in natural products and drug molecules (Figure 1; Bakulev and Dehaen, 2004; Shafran et al., 2018). Because of their unique biological activity and intrinsic reactivity, 1,2,3-thiadiazoles were widely used in medicine (Mloston and Huisgen, 1985, 1989; Thomas et al., 1985; Huisgen and Mloston, 1999; Wu et al., 2007; Cikotiene et al., 2009; Atta et al., 2010; Dong et al., 2010; Amirhamzeh et al., 2013), pesticides (Jalilian et al., 2003; Li et al., 2005; Fan et al., 2009; Wang et al., 2009; Zheng et al., 2010) and organic synthesis (Förster et al., 1997; Takimiya et al., 1997; Androsov and Neckers, 2007; Androsov, 2008; Teplyakov et al., 2013). In the past two decades, many efforts have been made to construct the 1,2,3-thiadiazole skeleton. The reported methods can be cataloged as the followings: (a) the 1,3-dipolar cycloaddition of diazoalkanes to thiocarbonyl compounds (Pechmann and Nold, 1896; Sheehan and Izzo, 1949; Martin and Mucke, 1965; Capuano et al., 1983; Aoyama et al., 1986); (b) Hurd-Mori synthesis and the analogous processes (Hurd and Mori, 1955; Kumar et al., 2012; Mo et al., 2019; Zhang et al., 2019); (c) the cyclization of Lawesson reagent with diazotized α-aminoketone (Caron, 1986); and (d) the [3 + 2] cycloaddition of α-enolic dithioester with tosyl azide (Singh et al., 2013). Although these methods provided some promising routes to access 1,2,3-thiadiazoles, the reported protocols also plagued by some drawbacks, such as the use of highly reactive reagents or pre-functionalized substrates, harsh reaction conditions, and a limited scope of substrate. Therefore, an effective route to construct 1,2,3-thiadiazole skeleton by using readily available chemicals is appealingly needed.
Recently, N-tosylhydrazones, which are readily accessible and inexpensive chemicals, have attracted much attention in the construction of heterocyclic compounds (Xia and Wang, 2017). In particular, iodine-catalyzed cyclization of N-tosylhydrazone with elemental sulfur has become one of the most efficient methods to synthesize 4-aryl-1,2,3-thiadiazoles (Chen et al., 2015; Ishikawa et al., 2017; Liu et al., 2018; Li et al., 2019). This transformation was triggered by α-iodation of acetophenone tosylhydrazone, which was generated in situ from the corresponding precursors (Scheme 1). One molecule of hydrogen iodide (HI) was also formed at the same time. To avoid the detrimental effect of acidic HI, and also to facilitate progress of a catalytic reaction, in the previous reports, an oxidizing reagent was adopted to convert HI to I2. With this strategy, some effective systems, such as K2S2O8/TBAI (Scheme 1, method a; Chen et al., 2015), TBHP/NH4I (method b; Li et al., 2019), and flavin-catalyzed O2 oxidation/NH4I (method c; Liu et al., 2018), have been developed successfully. Electrochemical oxidation in the combination of using NH4I as additive was also proved to be effective (method d; Ishikawa et al., 2017). Although the reported synthesis of 4-aryl-1,2,3-thiadiazoles through cyclization of N-tosylhydrazones is promising, owing to the addition of a large amount of oxidizing reagent or the use of special equipment, the effectiveness and the greenness of the synthesis were negatively affected by a time-consuming product separation procedure and the generation of waste.
Very recently, Wu et al. reported an efficient I2/CuCl2-promoted one-pot three-component strategy for the construction of 1,2,3-thiadiazoles from aliphatic- or aromatic-substituted methyl ketones, p-toluenesulfonyl hydrazide, and potassium thiocyanate in the presence of DMSO as solvent (Wang et al., 2019). However, excess stoichiometric amounts of I2 and CuCl2 are required. On the other hand, the combination of I2 and DMSO emerged recently as an effective and eco-friendly oxidative system for organic synthesis (Saba et al., 2015, 2016; Rafique et al., 2016; Silva et al., 2017; Monga et al., 2018). The past decade already witnessed the powerful productivity of this unique system to strengthen atom- and step-economic organic synthesis. Particularly, under appropriate conditions, regeneration of I2 from HI with the aid of DMSO proven to be practically feasible (Kalmode et al., 2014; Wu et al., 2014; Deshidi et al., 2015; Mohammed et al., 2015; Huang et al., 2019; Li et al., 2019). This not only minimized the dosage of I2 but also enabled us to establish some new reactions without adding external auxiliary reagent, simplifying thus the reaction system. Based on this observation, we envisaged that the I2/DMSO system may be applicable in the cyclization of N-tosylhydrazone with elemental sulfur. The dual role of DMSO as both solvent and oxidant, if it works, will allow us to synthesize 1,2,3-thiadiazoles in a simple system. Our preliminary results show that our speculation is indeed reasonable. Herein, we wish to report a facile synthesis of 4-aryl-1,2,3-thiadiazoles via an I2-catalyzed reaction between N-tosylhydrazones and element sulfur in DMSO solvent (Scheme 1, method e).
Results and Discussion
Our study commenced from a reaction of N-tosylhydrazone 1a and sulfur (S8). Initially, 0.30 mmol of 1a was mixed with 0.90 mmol of S8. The reaction was performed in DMSO, and the obtained results are listed in Table 1. No reaction occurred after 5 h of heating under air at 100°C (entry 1). Addition of 20 mol% of KI or tetrabutylammonium iodide (TBAI) cannot initiate the reaction either (entries 2 and 3). This is quite reasonable because, under non-acidic conditions, it is difficult to oxidize the iodide anion to elemental iodine by DMSO. Ammonium iodide (NH4I) has stronger acidity compared with that of TBAI. By using NH4I as a catalyst, the reaction proceeded slowly. And after 5 h, the desired product, 4-phenyl-1,2,3-thiadiazole 3a, was obtained in 11% of yield (entry 4). Intriguingly, I2 can catalyze the cyclization reaction effectively in conjunction with using DMSO as solvent, and the reaction yield reached 79% (entry 5). The choice of solvent is crucial. When DMSO was replaced by the other organic solvents, such as toluene, DMF, and 1,4-dioxane, the reaction proceeded hardly (entries 6–8). In an alcoholic solvent, isopropanol (IPA), the reaction can be initiated, but it proceeded very slowly. As a result, the yield stopped only at 15% (entry 9). To further improve the reaction yield, the effect of the dosage of I2 was scrutinized. Interestingly, it was found that the reaction yield could be improved to 86% by decreasing the amount of I2 to 10 mol% (entry 10). However, a further decrease of the I2 loading resulted in a drastic loss of the reaction yield (entry 11). With 2.5 mol% of I2, 3a can be isolated only in 10% yield (entry 12). We also tested the model reaction under argon. In this case, the reaction proceeded smoothly, and 3a can be isolated in 90% yield (entry 13). The yield can be slightly improved by decreasing the amount of S8 to 2.0 equivalents (92%, entry 14). From the viewpoint of green chemistry, the best system should allow also the use of an equal amount of precursors. Unfortunately, when 1a and 2a were charged equally, the reaction proceeded sluggishly (entry 15). Also, adjustment of the reaction temperature, reaction time, and the amount of DMSO did not significantly promote the reaction (entries 16–21). And finally, to reach a compromise of all of the reaction parameters, the optimal conditions were confirmed to be I2 catalyst (10 mol%), DMSO solvent, the ratio of 1a/2a is 1/2. The performance of NBS or NIS, which has also been used as an oxidizing reagent (Huang et al., 2017; Gu et al., 2018a,b; Xu et al., 2019), was also examined under the optimal conditions. However, only a trace amount of 3a can be detected (entries 22 and 23). This result demonstrated that to take the oxidizing ability of DMSO as a means to implement the synthesis, the use of I2 is mandatory.
With the optimized reaction conditions in hand, we then explored the effect of the arylsulfonyl group in the sulfonylhydrazone component on the reaction. As shown in Scheme 2, all the examined N-arylsulfonylhydrazones reacted with sulfur readily. Electron-rich N-arylsulfonylhydrazones seemingly like favorable for producing 3a. For example, while 81% of yield was obtained with an N-phenylsulfonylhydrazone formed from PhSO2NHNH2, the electron-deficient congener from 4-F-C6H4SO2NHNH2 gave 3a in 73% yield. Similarly, the reactions with electron-rich N-arylsulfonylhydrazones, like N-tosylhydrazone or its analogous with a methoxy group formed from 4-OMe-C6H4SO2NHNH2, provided a slightly higher yield of 3a than the former two (92 and 84%).
The effect of the imine part in the N-tosylhydrazone component on the reaction was also investigated, and the results are shown in Scheme 3. N-tosylhydrazones with different functional groups on the arene ring of the imine part all worked well under the standard conditions, efficiently providing the corresponding 4-aryl-1,2,3-thiadiazoles 3b−3i with yields ranging from 82 to 92%. The electronic nature of the substituents on the arene ring of the imine part, involving electron-donating (4-Me, 4-nBu, and 4-MeO) and electron-withdrawing (4-F, 4-Cl, 4-Br, 4-I, and 4-CF3) groups, had no obvious effect on the yields. Further, substituents in the meta position of the arene ring also showed good compatibility, giving cyclization products 3j and 3k in 91 and 92% yield, respectively. The N-tosylhydrazones with an ortho-substituted arene in their imine parts were also applicable in this reaction. For example, 3l and 3m can be synthesized in 90 and 72% yield, respectively. More sterically demanding N-tosylhydrazones derived from substituted acetylnaphthalenes participated also successfully in the reaction, furnishing the desired products 3n−3q in 83–98% yields. Similarly, biaryl substituted N-tosylhydrazones could also be used in this transformation, delivering the desired products 3r−3t in 83–97% yields. The N-tosylhydrazone synthesized from trans-4-phenyl-3-buten-2-one participated readily into this reaction as well. And the double bond in the substrate 1 was delivered into the structure of the expected product 3u, without any damage. While good yield was obtained with acetophenone-derived N-tosylhydrazones, the introduction of a functional group on the carbon of the imine group significantly decreased the reactivity of substrate 1. As a result, the N-tosylhydrazones came from 2-phenylacetophenone, 1H-indene-1,3(2H)-dione, and propiophenone, reluctantly engaged in the reaction, giving 3v−3x in <50% yield. The usefulness of this method was demonstrated by a very efficient synthesis of the neuroprotective reagent 3y. Through a reaction of 1-(3,4,5-trimethoxyphenyl)ethan-1-one N-tosylhydrazone with sulfur, 3y was obtained in 85% yield. It should be noted that, although the reported method for synthesizing 3y started also from the same N-tosylhydrazone derivative (Thomas et al., 1985), owing to the use of highly toxic reagent, thionyl chloride, our method can be considered as a green protocol for implementing the synthesis of 3y. Furthermore, the transformations of aliphatic and heterocyclic N-tosylhydrazones with sulfur were also investigated, but the desired products 3z and 4a were not obtained. We also tried to use selenium or tellurium instead of sulfur to perform this reaction, but unfortunately, none of them succeeded.
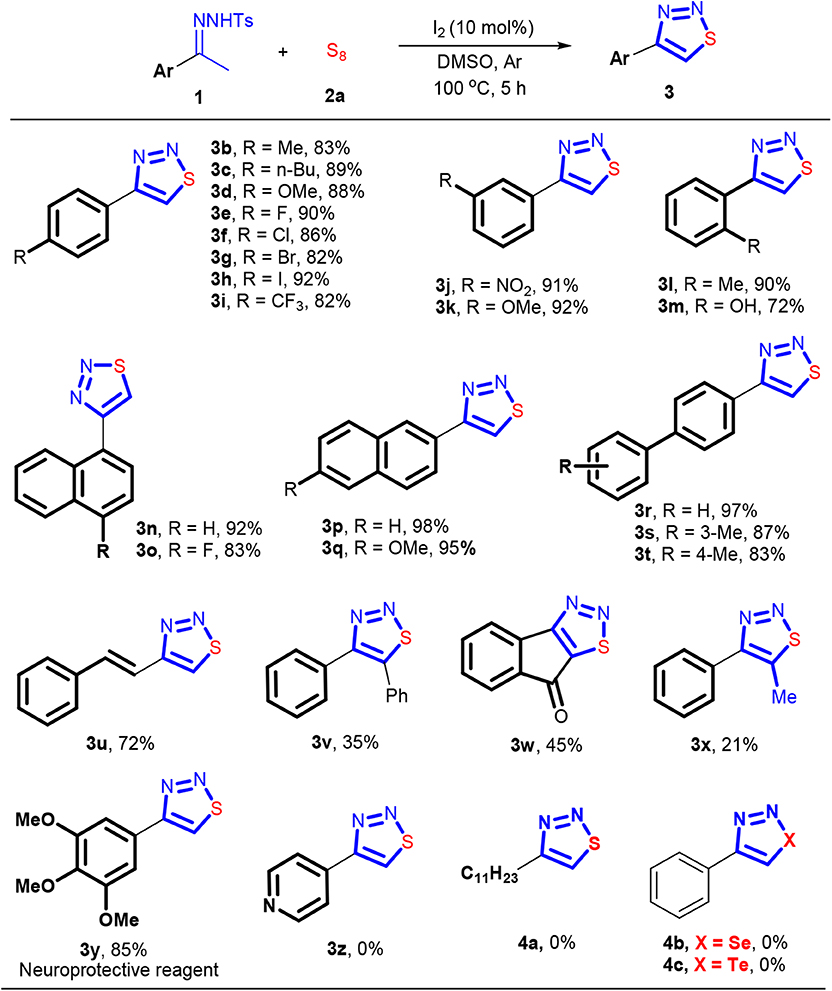
Scheme 3. The substrate scope of N-tosylhydrazones. Reaction conditions: 0.3 mmol 1, 0.6 mmol S8, I2 (10 mol%), DMSO (3 mL), 5 h, under argon. Isolated yield.
Since N-tosylhydrazone 1 can be easily formed from p-toluenesulfonhydrazide and a ketone (Sun et al., 2018; El-Harairy et al., 2019a,b; Li et al., 2019; Liu et al., 2019), we then investigated whether or not the cyclization reaction could be carried out in one-pot fashion directly using a ketone and the hydrazide as the precursors. If it was established, the isolation and purification of the N-tosylhydrazone component can be avoided, thus significantly strengthening the synthetic efficiency. The results showed that this idea was indeed feasible, and the cyclization products were obtained with 70–97% yields (Scheme 4). To ensure a good yield of the reaction, all the three-component reactions were performed under argon atmosphere. It should be noted that 2-hydroxyacetophone that contains a reactive arene ring toward electrophilic iodination can tolerate the I2-based conditions. The corresponding product 3m can be formed in 70% yield.
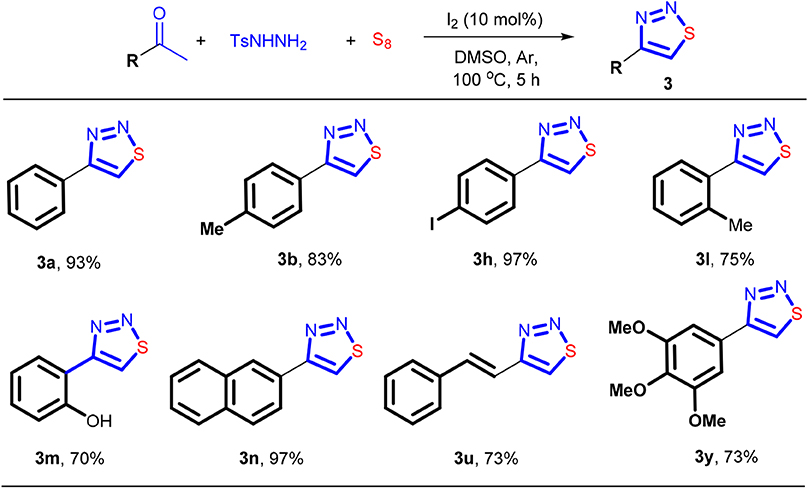
Scheme 4. The synthesis of 4-aryl-1,2,3-thiadiazoles via one-pot fashion. Reaction conditions: 0.3 mmol of aryl ketone, 0.33 mmol of TsNHNH2, 0.6 mmol of S8, I2 (10 mol%), DMSO (3 mL), 5 h, under argon. Isolated yield.
The neuroprotective reagent 3y can also be synthesized in this way, but the yield obtained is slightly inferior compared with the method in Scheme 3. Despite this fact, this three-component protocol is quite promising as it saved one step while minimized the generation of waste. The reaction can also be carried out on a gram-scale synthesis. For instance, the reaction performed well using 7.5 mmol of 4-bromoacetophenone, 8.25 mmol of TsNHNH2, and 15 mmol of sulfur, leading to isolation of 1.57 g of the product 3a with 87% of yield (Scheme 5).
To shed light on the mechanism, some control experiments were conducted. As shown in Scheme 6, although 1a was completely consumed, 3a can be hardly detected when 2.0 equivalents of 2,2,6,6-tetramethylpiperidinooxy (TEMPO) were added. The addition of benzoquinone (BQ) was found to be detrimental for the reaction either. But in this case, the reaction was not quenched, and it proceeded slowly, and 3a can be isolated in 32% yield under the identical conditions. The effects of 9,10-dihydroanthracene and 1,1-diphenylethylene, which are acid-compatible radical scavengers, on the model reaction were also investigated. And 3a can be isolated in 93 and 90% yields, respectively. The reaction proceeded uneventfully in the presence of 2,6-di-tert-butyl-4-methylphenol (BHT). In the absence of sulfur, decomposition of 1a occurred, providing II′ as the main product (Pramanik et al., 2019). In this case, the isolated II′ is not pure, and the 1H and 13C NMR spectra led us to have speculation on the formation of II. HRMS also supported our speculation as a peak at 287.0575 (M + H+) can be observed. The treatment of the mixture of 2-iodo-1-phenylethan-1-one and TsNHNH2 with sulfur resulted in the formation of 3a. It should be noted that, when DMSO was used as solvent, the transformation was always successful either in the presence or in the absence of I2. However, replacing DMSO with the other solvents, such as DMF and toluene, resulted in a dramatic loss of the reaction yield. These results indicated that the choice of solvent is the key to ensure a good yield of this cyclization.
Based on all these observations, a plausible mechanism was proposed. We conjectured that the reaction followed a polar reaction mechanism rather than a free-radical mechanism. As shown in Scheme 7, the initial event should be α-iodation of 1a, which gives I as an intermediate. Then, an elimination of one molecule of HI of I occurred, providing an intermediate II. Because of the presence of an electron-rich vinyl group, this species behaves like a nucleophile, can thus react with sulfur to form an intermediate III (Chen et al., 2015; Ishikawa et al., 2017; Liu et al., 2018; Li et al., 2019). Finally, 3a was formed through an intramolecular cyclization and the following elimination of TsH and S7. In the first two steps of the reaction, two molecules of HI were generated. To establish a catalytic cycle, HI must be oxidized to I2. The unique oxidizing ability of the solvent DMSO played the key role in regenerating I2 (Steuer et al., 2011; Deshidi et al., 2014, 2015; Kalmode et al., 2014, 2015; Wu et al., 2014; Mohammed et al., 2015; Mupparapu et al., 2015). The combination of I2 and DMSO ensured the success of this synthetic reaction.
Conclusion
In summary, we have developed an iodine-catalyzed cyclization of N-tosylhydrazones with sulfur using DMSO a dual solvent and oxidant. This reaction provided an efficient approach to produce diversified 4-aryl-1,2,3-thiadiazoles in good yields. This method can be used in a gram scale synthesis. Furthermore, a one-pot synthesis was also established, which allowed the direct use of ketone as substrate, without isolating the N-tosylhydrazone intermediate. This approach was proven also to be applicable in the synthesis of a neuroprotective reagent. An eminent advantage of this strategy is that it avoided the use of external oxidants. Considering expensive photochemical catalyst or electrochemical instruments are required in the previously reported methods, the present method can be considered as a practically applicable and environmentally benign approach for the synthesis of 1,2,3-thiadiazoles.
Materials and Methods
Chemicals were obtained commercially and used as received. NMR spectra were recorded on a Bruker DPX−400 spectrometer using TMS as the internal standard. DMSO as solvent was used directly without any treatment. All products were isolated by short chromatography on a silica gel (200–300 mesh) column using petroleum ether (60–90°C), unless otherwise noted. All of reagents were of analytical grade quality, purchased from Adamas-beta Pharmaceuticals, Inc.
General Procedure for I2/DMSO-Catalyzed Transformation From N-tosylhydrazones and Sulfur to 4-aryl 1,2,3-thiadiazoles
A mixture of substituted N-tosylhydrazones (0.3 mmol), sulfur (0.6 mmol), I2 (10 mol%) were loaded into a Schlenk tube (25 mL). Then, the tube was degassed for 30 s and filled with Argon. This process was repeated for a total of three times. Afterward, DMSO (3 mL) was added under an argon atmosphere. The resulting reaction mixture was stirred and heated to 100°C for 5 h. After reaction completion, the solution was quenched the saturated solution of sodium thiosulfate (5 mL) and extracted with EtOAc (3 × 10 mL). The combined EtOAc extracts were dried over anhydrous Na2SO4, filtered, and concentrated under reduced pressure. The crude residue was purified by flash column chromatography on silica gel using PE/EtOAc as the eluent.
General Procedure for the Synthesis of 4-aryl-1,2,3-thiadiazoles via One-Pot Fashion
A Schlenk tube (25 mL) equipped with a stir bar was charged with TsNHNH2 (0.33 mmol), sulfur (0.6 mmol), I2 (10 mol%). Then, the tube was degassed for 30 s and filled with Argon. This process was repeated for a total of three times. Afterward, aryl ketone (0.3 mmol) and DMSO (3 mL) was added under an argon atmosphere. The resulting reaction mixture was stirred and heated to 100°C for 5 h. After reaction completion, the solution was quenched the saturated solution of sodium thiosulfate (5 mL) and extracted with EtOAc (3 × 10 mL). The combined EtOAc extracts were dried over anhydrous Na2SO4, filtered, and concentrated under reduced pressure. The crude residue was purified by flash column chromatography on silica gel using PE/EtOAc as the eluent.
Data Availability Statement
The raw data supporting the conclusions of this article will be made available by the authors, without undue reservation, to any qualified researcher.
Author Contributions
PL, YG, and LV constructed the workflow. WL, JZ, and JH synthesized and purified the compounds. LX performed the NMR spectrometric analysis. PL and YG completed the paper.
Funding
This work was supported by the National Natural Science Foundation of China (Nos. 21563025, 2171101076, and 21872060) and the Shihezi University (No. CXRC201602). MIUR was acknowledged for the support via the project AMIS - Dipartimenti di Eccellenza 2018-2022.
Conflict of Interest
The authors declare that the research was conducted in the absence of any commercial or financial relationships that could be construed as a potential conflict of interest.
Supplementary Material
The Supplementary Material for this article can be found online at: https://www.frontiersin.org/articles/10.3389/fchem.2020.00466/full#supplementary-material
References
Amirhamzeh, A., Vosoughi, M., Shafiee, A., and Amini, M. (2013). Synthesis and docking study of diaryl-isothiazole and 1,2,3-thiadiazole derivatives as potential neuroprotective agents. Med. Chem. Res. 22, 1212–1223. doi: 10.1007/s00044-012-0124-9
Androsov, D. A. (2008). Synthesis of 1, 1-dialkylindolium-2-thiolates via base-induced transformation of 4-(2-chloro-5-nitrophenyl)-1,2,3-thiadiazole in the presence of secondary amines. J. Org. Chem. 73, 8612–8614. doi: 10.1021/jo801801y
Androsov, D. A., and Neckers, D. C. (2007). Synthesis and reactivity of 4-(2-chloro-5-nitrophenyl)-1, 2, 3-thiadiazole. A novel one-pot synthesis of N-substituted indole-2-thiols. J. Org. Chem. 72, 5368–5373. doi: 10.1021/jo0707784
Aoyama, T., Iwamoto, Y., and Shioiri, T. (1986). New methods and reagents in organic synthesis. 59. Lithium trimethylsilydiazomethane: a new synthon for the preparation of 5-substituted 1,2,3-thiadiazoles. Heterocycles 24, 589–592. doi: 10.3987/R-1986-03-0589
Atta, S. M. S., Farrag, D. S., Sweed, A. M., and Abdel-Rahman, A. H. (2010). Preparation of new polycyclic compounds derived from benzofurans and furochromones. An approach to novel 1,2,3-thia-, and selena-diazolofurochromones of anticipated antitumor activities. Eur. J. Med. Chem. 45, 4920–4927. doi: 10.1016/j.ejmech.2010.07.065
Bakulev, V. A., and Dehaen, W. (2004). The Chemistry of 1,2,3-Thiadiazoles. New York, NY: John Wiley and Sons Press.
Capuano, L., Boschat, P., Müller, I., Zander, R., Schramm, V., and Hädicke, E. (1983). Synthesen mit 2,4-dimethylen-1,3-dithietanen. mercaptopyridine; thiazole; dithiole; mesoionische und nichtmesoionische 1,2,3-thiadiazole. Chem. Ber. 116, 2058–2067. doi: 10.1002/cber.19831160603
Caron, M. (1986). Convenient preparation of 5-alkyl-4-carbalkoxy-1,2,3-thiadiazoles. J. Org. Chem. 51, 4075–4077. doi: 10.1021/jo00371a033
Chen, J., Jiang, Y., Yu, J. T., and Cheng, J. (2015). TBAI-catalyzed reaction between N-tosylhydrazones and sulfur: a procedure toward 1,2,3-thiadiazole. J. Org. Chem. 81, 271–275. doi: 10.1021/acs.joc.5b02280
Cikotiene, I., Kazlauskas, E., Matuliene, J., Michailoviene, V., Torresan, J., Jachno, J., et al. (2009). 5-Aryl-4-(5-substituted-2,4-dihydroxyphenyl)-1,2,3-thiadiazoles as inhibitors of Hsp90 chaperone. Bioorg. Med. Chem. Lett. 19, 1089–1092. doi: 10.1016/j.bmcl.2009.01.003
Deshidi, R., Devari, S., and Shah, B. A. (2015). Iodine-promoted oxidative amidation of terminal alkenes–synthesis of α-ketoamides, benzothiazoles, and quinazolines. Eur. J. Org. Chem. 1428–1432. doi: 10.1002/ejoc.201403547
Deshidi, R., Kumar, M., Devari, S., and Shah, B. A. (2014). A general metal free approach to α-ketoamides via oxidative amidation-diketonization of terminal alkynes. Chem. Commun. 50, 9533–9535. doi: 10.1039/C4CC03783A
Dong, W. L., Liu, Z. X., Liu, X. H., Li, Z. M., and Zhao, W. G. (2010). Synthesis and antiviral activity of new acrylamide derivatives containing 1,2,3-thiadiazole as inhibitors of hepatitis B virus replication. Eur. J. Med. Chem. 45, 1919–1926. doi: 10.1016/j.ejmech.2010.01.032
El-Harairy, A., Lai, B., Vaccaro, L., Li, M., and Gu, Y. (2019a). A sulfone-containing imidazolium-based brønsted acid ionic liquid catalyst enables replacing dipolar aprotic solvents with butyl acetate. Adv. Synth. Catal. 361, 3342–3350. doi: 10.1002/adsc.201900246
El-Harairy, A., Yue, M., Fan, W., Popowycz, F., Queneau, Y., Li, M., et al. (2019b). Novel non-toxic and non-hazardous solvent systems for the chemistry of indoles: use of a sulfone-containing brønsted acid ionic liquid catalyst in butyl acetate. ChemCatChem. 11, 4403–4410. doi: 10.1002/cctc.201900784
Fan, Z., Shi, Z., Zhang, H., Liu, X., Bao, L., Ma, L., et al. (2009). Synthesis and biological activity evaluation of 1,2,3-thiadiazole derivatives as potential elicitors with highly systemic acquired resistance. J. Agric. Food Chem. 57, 4279–4286. doi: 10.1021/jf8031364
Förster, W. R., Isecke, R., Spanka, C., and Schaumann, E. (1997). Functionalized β-thiolactams by lewis acid catalyzed addition of alkynyl silyl sulfides to azomethines. Synthesis 1997, 942–948. doi: 10.1055/s-1997-1275
Gu, Y., Huang, W., Chen, S., and Wang, X. (2018a). Bismuth (III) triflate catalyzed three-component reactions of indoles, ketones, and α-bromoacetaldehyde acetals enable indole-to-carbazole transformation. Org. Lett. 20, 4285–4289. doi: 10.1021/acs.orglett.8b01707
Gu, Y., Wu, F., and Yang, J. (2018b). Oxidative [3+3] annulation of atropaldehyde acetals with 1,3-bisnucleophiles: an efficient method of constructing six-membered aromatic rings, including salicylates and carbazoles. Adv. Synth. Catal. 360, 2727–2741. doi: 10.1002/adsc.201800462
Huang, W., Liu, C., and Gu, Y. (2017). Auto-tandem catalysis-induced synthesis of trisubstituted furans through domino acid-acid-catalyzed reaction of aliphatic aldehydes and 1,3-dicarbonyl compounds by using N-bromosuccinimide as oxidant. Adv. Synth. Catal. 359, 1811–1818. doi: 10.1002/adsc.201700074
Huang, W., Xu, J., Liu, C., Chen, Z., and Gu, Y. (2019). Lewis acid-catalyzed synthesis of benzofurans and 4,5,6,7-tetrahydrobenzofurans from acrolein dimer and 1,3-dicarbonyl compounds. J. Org. Chem. 84, 2941–2950. doi: 10.1021/acs.joc.9b00270
Huisgen, R., and Mloston, G. (1999). Adamantanethione and diazomethane: dual regiochemistry of cycloadditions [1]. Pol. J. Chem. 73, 635–644. doi: 10.1002/chin.199928044
Hurd, C. D., and Mori, R. I. (1955). On acylhydrazones and 1,2,3-thiadiazoles. J. Am. Chem. Soc. 77, 5359–5364. doi: 10.1021/ja01625a047
Ishikawa, T., Kimura, M., Kumoi, T., and Iida, H. (2017). Coupled flavin-iodine redox organocatalysts: aerobic oxidative transformation from N-tosylhydrazones to 1,2,3-thiadiazoles. ACS Catal. 7, 4986–4989. doi: 10.1021/acscatal.7b01535
Jalilian, A. R., Sattari, S., Bineshmarvasti, M., Daneshtalab, M., and Shafiee, A. (2003). Synthesis and in vitro antifungal and cytotoxicity evaluation of substituted 4,5-dihydronaphtho [1,2-d] [1,2,3] thia (or selena) diazoles. Farmaco 58, 63–68. doi: 10.1016/S0014-827X(02)00029-0
Kalmode, H. P., Vadagaonkar, K. S., and Chaskar, A. C. (2014). Metal-free in situ sp3, sp2, and sp C–H functionalization and oxidative cross coupling with benzamidines hydrochloride: a promising approach for the synthesis of α-ketoimides. RSC Adv. 4, 60316–60326. doi: 10.1039/C4RA07556K
Kalmode, H. P., Vadagaonkar, K. S., and Chaskar, A. C. (2015). The oxidative cross-coupling of benzonitriles with multiform substrates: a domino strategy inspired easy access to α-ketoimides. Synthesis 47, 429–438. doi: 10.1055/s-0034-1379955
Kumar, A., Muthyala, M. K., Choudhary, S., Tiwari, R. K., and Parang, K. (2012). Ionic liquid as soluble support for synthesis of 1,2,3-thiadiazoles and 1,2,3-selenadiazoles. J. Org. Chem. 77, 9391–9396. doi: 10.1021/jo301607a
Li, W., He, J., Liu, P., Zhang, J., and Dai, B. (2019). Synthesis of 4-aryl-1,2,3-thiadiazoles via NH4I-catalyzed cyclization of N-tosylhydrazones with sulfur. Chem. Select 4, 10587–10590. doi: 10.1002/slct.201902684
Li, Z., Wu, Z., and Luo, F. (2005). Synthesis and antifungal activities of alkyl N-(1,2,3-thiadiazole-4-carbonyl) carbamates and S-alkyl N-(1,2,3-thiadiazole-4-carbonyl) carbamothioates. J. Agric. Food Chem. 53, 3872–3876. doi: 10.1021/jf0501746
Liu, B. B., Bai, H. W., Liu, H., Wang, S. Y., and Ji, S. J. (2018). Cascade trisulfur radical anion (S3∙−) addition/electron detosylation process for the synthesis of 1,2,3-thiadiazoles and isothiazoles. J. Org. Chem. 83, 10281–10288. doi: 10.1021/acs.joc.8b01450
Liu, Y., Chen, L., Wang, Z., Liu, P., Liu, Y., and Dai, B. (2019). Cascade reaction of arylboronic acids and 2′-cyano-biaryl-2-aldehyde N-Tosylhydrazones: access to functionalized 9-amino-10-arylphenanthrenes. J. Org. Chem. 84, 204–215. doi: 10.1021/acs.joc.8b02605
Martin, D., and Mucke, W. (1965). Cycloaddition von diazoalkanen an isothiocyanate. Justus Liebigs Ann. Chem. 682, 90–98. doi: 10.1002/jlac.19656820109
Mloston, G., and Huisgen, R. (1985). Acid-base reactions of 1,3,4-thiadiazolines and thiocarbonyl ylides; 1,3,4-thiadiazoline-2-spiro-2′-adamantane. Tetrahedron Lett. 26, 1053–1056. doi: 10.1016/S0040-4039(00)98510-1
Mloston, G., and Huisgen, R. (1989). Open-chain aliphatic thiones and diazomethane; reactions of 1,3,4-thiadiazolines and thiocarbonyl ylides. Tetrahedron Lett. 30, 7045–7048. doi: 10.1016/S0040-4039(01)93419-7
Mo, S. K., Teng, Q. H., Pan, Y. M., and Tang, H. T. (2019). Metal- and oxidant- free electrosynthesis of 1,2,3-thiadiazoles from element sulfur and N-tosyl Hydrazones. Adv. Synth. Catal. 361, 1756–1760. doi: 10.1002/adsc.201801700
Mohammed, S., Vishwakarma, R. A., and Bharate, S. B. (2015). Iodine catalyzed oxidative synthesis of quinazolin-4 (3 H)-ones and pyrazolo [4,3-d] pyrimidin-7(6H)-ones via amination of sp3 C–H bond. J. Org. Chem. 80, 6915–6921. doi: 10.1021/acs.joc.5b00989
Monga, A., Bagchi, S., and Sharma, A. (2018). Iodine/DMSO oxidations: a contemporary paradigm in C–N bond chemistry. New J. Chem. 42, 1551–1576. doi: 10.1039/C7NJ04513A
Mupparapu, N., Vishwakarma, R. A., and Ahmed, Q. N. (2015). Iodine-DMSO promoted C–H (SP3) functionalization approach to α-ketoamides. Tetrahedron 71, 3417–3421. doi: 10.1016/j.tet.2015.03.088
Pechmann, H. V., and Nold, A. (1896). Ueber die einwirkung von diazomethan auf phenylsenföl. Ber. Dtsch. Chem. Ges. 29, 2588–2593. doi: 10.1002/cber.18960290336
Pramanik, M., Choudhuri, K., and Mal, P. (2019). N-Iodosuccinimide as bifunctional reagent in (E)-selective C(sp2)–H sulfonylation of styrenes. Asian J. Org. Chem. 8, 144–150. doi: 10.1002/ajoc.201800644
Rafique, J., Saba, S., Rosario, A. R., and Braga, A. L. (2016). Regioselective, solvent- and metal-free chalcogenation of imidazo [1,2-a] pyridines by employing I2/DMSO as the catalytic oxidation system. Chem. Eur. J. 22, 11854–11862. doi: 10.1002/chem.201600800
Saba, S., Rafique, J., and Braga, A. L. (2015). Synthesis of unsymmetrical diorganyl chalcogenides under greener conditions: use of an Iodine/DMSO system, solvent- and metal-free approach. Adv. Synth. Catal. 357, 1446–1452. doi: 10.1002/adsc.201500024
Saba, S., Rafique, J., and Braga, A. L. (2016). DMSO/iodine-catalyzed oxidative C–Se/C–S bond formation: a regioselective synthesis of unsymmetrical chalcogenides with nitrogen-or oxygen-containing arenes. Catal. Sci. Technol. 6, 3087–3098. doi: 10.1039/C5CY01503K
Shafran, Y., Glukhareva, T., Dehaen, W., and Bakulev, V. (2018). Recent developments in the chemistry of 1,2,3-thiadiazoles. Adv. Heterocycl. Chem. 126, 109–172. doi: 10.1016/bs.aihch.2017.12.001
Sheehan, J. C., and Izzo, P. T. (1949). The reaction of diazomethane with isocyanates and isothiocyanates. J. Am. Chem. Soc. 71, 4059–4062. doi: 10.1021/ja01180a054
Silva, L. T., Azeredo, J. B., Saba, S., Rafique, J., Bortoluzzi, A. J., and Braga, A. L. (2017). Solvent- and metal-free chalcogenation of bicyclic arenes using I2/DMSO as non-metallic catalytic system. Eur. J. Org. Chem. 2017, 4740–4748. doi: 10.1002/ejoc.201700744
Singh, M. S., Nagaraju, A., Verma, G. K., Shukla, G., Verma, R. K., and Srivastava, A. (2013). Eco-efficient, regioselective and rapid access to 4,5-disubstituted 1,2,3-thiadiazoles via [3 + 2] cycloaddition of α-enolicdithioesters with tosyl azide under solvent-free conditions. Green Chem. 15, 954–962. doi: 10.1039/C3GC37047J
Steuer, C., Gege, C., Fischl, W., Heinonen, K. H., Bartenschlager, R., and Klein, C. D. (2011). Synthesis and biological evaluation of α-ketoamides as inhibitors of the dengue virus protease with antiviral activity in cell-culture. Bioorg. Med. Chem. 19, 4067–4074. doi: 10.1016/j.bmc.2011.05.015
Sun, Z., Du, C., Liu, P., Wei, Y., Xu, L., and Dai, B. (2018). Highly selective β-hydride elimination in the Pd-catalyzed cross-coupling of N-Tosylhydrazones with benzyl bromides. Chem. Select 3, 900–903. doi: 10.1002/slct.201702521
Takimiya, K., Morikami, A., and Otsubo, T. (1997). A convenient preparation of 1,3-dithiole-2-thione and 1,3-diselenole-2-selone derivatives. Synlett 1997, 319–321. doi: 10.1055/s-1997-787
Teplyakov, F. S., Vasileva, T. G., Petrov, M. L., and Androsov, D. A. (2013). A new synthesis of benzo [b] thiophene-2-thiolates and their derivatives via base-promoted transformation of 4-(2-mercaptophenyl)-1,2,3-thiadiazoles. Org. Lett. 15, 4038–4041. doi: 10.1021/ol401981w
Thomas, E. W., Nishizawa, E. E., Zimmermann, D. C., and Williams, D. J. (1985). Synthesis and platelet aggregation inhibitory activity of 4,5-bis (substituted)-1,2,3-thiadiazoles. J. Med. Chem. 28, 442–446. doi: 10.1021/jm00382a009
Wang, C., Geng, X., Zhao, P., Zhou, Y., Wu, Y. D., and Cui, Y. F. (2019). I2/CuCl2-promoted one-pot three-component synthesis of aliphatic or aromatic substituted 1,2,3-thiadiazoles. Chem. Commun. 55, 8134–8137. doi: 10.1039/C9CC04254G
Wang, Z. H., Guo, Y. Z., Zhang, J., Ma, L., Song, H. B., and Fan, Z. J. (2009). Synthesis and biological activity of organotin 4-methyl-1,2,3-thiadiazole-5-carboxylates and Benzo [1,2,3] thiadiazole-7-carboxylates. J. Agric. Food Chem. 58, 2715–2719. doi: 10.1021/jf902168d
Wu, M., Sun, Q., Yang, C., Chen, D., Ding, J., Chen, Y., et al. (2007). Synthesis and activity of combretastatin A-4 analogues: 1,2,3-thiadiazoles as potent antitumor agents. Bioorg. Med. Chem. Lett. 17, 869–873. doi: 10.1016/j.bmcl.2006.11.060
Wu, X., Gao, Q., Liu, S., and Wu, A. (2014). I2-catalyzed oxidative cross-coupling of methyl ketones and benzamidines hydrochloride: a facile access to α-ketoimides. Org. Lett. 16, 2888–2891. doi: 10.1021/ol501029w
Xia, Y., and Wang, J. (2017). N-Tosylhydrazones: versatile synthons in the construction of cyclic compounds. Chem. Soc. Rev. 46, 2306–2362. doi: 10.1039/C6CS00737F
Xu, J., Huang, W., Bai, R., Queneau, Y., Jérôme, F., and Gu, Y. (2019). Utilization of bio-based glycolaldehyde aqueous solution in organic synthesis: application to the synthesis of 2,3-dihydrofurans. Green Chem. 21, 2061–2069. doi: 10.1039/C8GC04000A
Zhang, Y., Cao, Y., Lu, L., Zhang, S., Bao, W., Huang, S., et al. (2019). Perylenequinonoid-catalyzed [4+1]-and [4 + 2]-annulations of azoalkenes: photocatalytic access to 1,2,3-thiadiazole/1,4,5,6-tetrahydropyridazine derivatives. J. Org. Chem. 84, 7711–7721. doi: 10.1021/acs.joc.9b00545
Keywords: N-tosylhydrazone, 1,2,3-thiadiazoles, iodine, DMSO, sulfur
Citation: Li W, Zhang J, He J, Xu L, Vaccaro L, Liu P and Gu Y (2020) I2/DMSO-Catalyzed Transformation of N-tosylhydrazones to 1,2,3-thiadiazoles. Front. Chem. 8:466. doi: 10.3389/fchem.2020.00466
Received: 16 January 2020; Accepted: 04 May 2020;
Published: 12 June 2020.
Edited by:
Jian-Wei Han, East China University of Science and Technology, ChinaReviewed by:
Jamal Rafique, Federal University of Mato Grosso do Sul, BrazilFeng-Cheng Jia, Wuhan Institute of Technology, China
Copyright © 2020 Li, Zhang, He, Xu, Vaccaro, Liu and Gu. This is an open-access article distributed under the terms of the Creative Commons Attribution License (CC BY). The use, distribution or reproduction in other forums is permitted, provided the original author(s) and the copyright owner(s) are credited and that the original publication in this journal is cited, in accordance with accepted academic practice. No use, distribution or reproduction is permitted which does not comply with these terms.
*Correspondence: Ping Liu, bGl1cGluZzE5NzkxMTJAYWxpeXVuLmNvbQ==; Yanlong Gu, a2xneWxAaHVzdC5lZHUuY24=