Published in
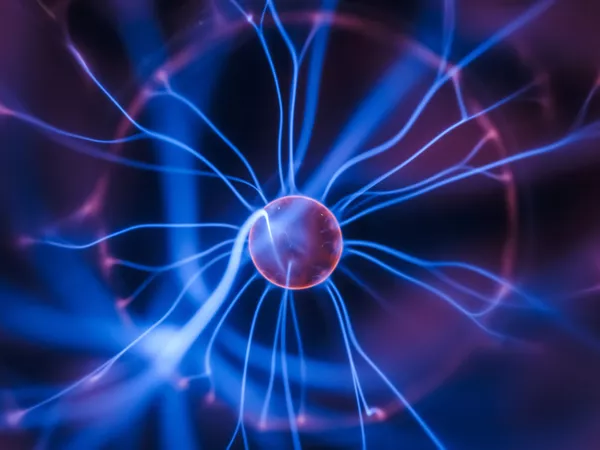
Frontiers in Physics
- Medical Physics and Imaging
- Radiation Detectors and Imaging
- Biophysics
5,215
Total downloads
29k
Total views and downloads
Select the journal/section where you want your idea to be submitted:
Frontiers in Molecular Biosciences
Frontiers in Oncology
Frontiers in Physiology