MINI REVIEW
Published on 13 Apr 2023
Plasmonics for microwave photonics in the THz range
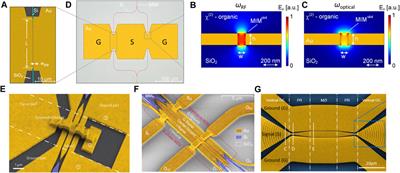
doi 10.3389/fphot.2023.1067916
- 1,932 views
- 4 citations
1,409
Total Downloads
6,546
Total Views and Downloads
Select the journal/section where you want your idea to be submitted:
MINI REVIEW
Published on 13 Apr 2023
ORIGINAL RESEARCH
Published on 05 Apr 2023
ORIGINAL RESEARCH
Published on 06 Sep 2022