EDITORIAL
Published on 20 Aug 2021
Editorial: Crystal Archives of Magmatic Processes
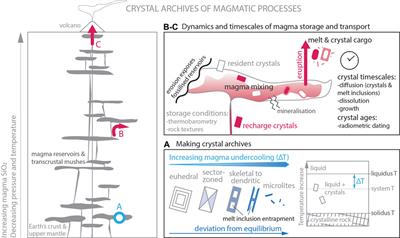
doi 10.3389/feart.2021.749100
- 3,032 views
- 10 citations
15k
Total downloads
81k
Total views and downloads
You will be redirected to our submission process.
EDITORIAL
Published on 20 Aug 2021
ORIGINAL RESEARCH
Published on 15 Jan 2021
ORIGINAL RESEARCH
Published on 29 Sep 2020
ORIGINAL RESEARCH
Published on 28 Aug 2020
ORIGINAL RESEARCH
Published on 31 Jul 2020
ORIGINAL RESEARCH
Published on 10 Jul 2020
ORIGINAL RESEARCH
Published on 19 Jun 2020
ORIGINAL RESEARCH
Published on 18 Jun 2020
ORIGINAL RESEARCH
Published on 10 Jun 2020
ORIGINAL RESEARCH
Published on 05 Jun 2020
ORIGINAL RESEARCH
Published on 22 May 2020
ORIGINAL RESEARCH
Published on 06 May 2020