- 1Department of Environmental Management, Faculty of Earth and Environmental Sciences, Bayero University, Kano, Nigeria
- 2Department of Biology, Faculty of Life Sciences, Ahmadu Bello University, Zaria, Nigeria
- 3Department of Microbiology, Faculty of Life Sciences, Federal University, Dutsin-Ma, Nigeria
This study assessed the impact of seepage from pit latrines on groundwater quality and the prevalence of waterborne diseases in primary health care in Funtua town, Katsina State, Nigeria. Well water samples were collected from 15 different areas in 8 out of the 11 wards within Funtua Local Government, while three wards were excluded due to no record of the chlorella outbreak. Water samples were collected twice for each season (dry and wet) from the 15 sampling locations throughout the study area and transported immediately to the laboratory for analysis. The samples were analyzed according to the standard methods and procedures of APHA (2005). Most of the physicochemical parameter results fell within the limits established by WHO (2011) and NSDWQ (2015), except for dissolved oxygen (DO), chloride, total dissolved solids (TDS), and electrical conductivity (EC). The maximum mean concentrations of pH, phosphate, and sulphate (7.64 ± 0.54, 19.23 ± 4.63 mg/L, and 1.12 ± 0.64 mg/L) were recorded in Danduste ward, while a low turbidity mean value of 2.96 ± 0.31 NTU was observed in Goya ward. The high total coliform and faecal coliform counts were recorded from well water samples in all the wards, while Escherichia coli, Shigella dysenteriae, and Salmonella typhi were the bacterial isolates identified during this study. Diarrhoea, typhoid, and dysentery were the most commonly reported waterborne diseases in most primary health centers within this study area. The study revealed that well water sources were located close to pit latrines, averaging 11.4 m, which is below the WHO-recommended safe distance of 30 m. The quality of well water in the Funtua Local Government Area has been compromised due to the proximity of some water sources to pit latrines, rendering the water unsuitable for use without prior treatment.
1 Introduction
Access to safe and clean drinking water is fundamental for sustaining human life and promoting good health. It is vital for digestion, nutrient absorption, waste elimination, and overall well-being (WHO and UNICEF, 2019). Water covers approximately 70% of the Earth’s surface, and its distribution is unequal across the globe, varying in quality and quantity (Falkenmark and Rockstrom, 2006). The disparity in water resource availability has prompted international attention to address water-related challenges in achieving equitable public health and economic development (United Nations General Assembly, 2010). It is estimated that approximately 9% (1.1 billion) of the world population lacks access to safe water supplies, while 2.4 billion cannot adequately access a good sanitation environment, despite this being one of the global Millennium Development Goals (MDGs) to achieve (WHO and UNICEF, 2019). Despite significant efforts made by governments in many developing countries to meet the Millennium Development Goal (MDG) target for drinking water, reports show that 663 million people still lack improved drinking water sources (WHO, 2011).
Various sources of water support human and animal population growth in tropical regions, such as rivers, lakes, reservoirs, rainwater, streams, springs, boreholes, hand-dug wells, and well water (Ajibade et al., 2013). The pollution of freshwater bodies globally, caused by anthropogenic activities and the discharge of waste materials from pre-industrial sources and residents, renders many of these resources unsuitable for human consumption. This situation has led to the increased reliance on groundwater as an alternative source of potable water for populations. Water from beneath the ground has been exploited for domestic use, livestock, and irrigation since ancient times. Groundwater is easily the most important component, constituting about two-thirds of the world’s freshwater resources. The dominant role of groundwater is evident, and its use and protection are, therefore, fundamentally important to human life and economic activity. This importance stems from its convenient availability near where it is required, its excellent natural quality requiring little or no treatment, and the relatively low capital cost of development.
In developing countries such as Nigeria, wells and boreholes serve as primary sources of water, especially in rural areas (National Ground Water Association, 2009). To prevent groundwater pollution, these sources must be carefully located and situated far from pit latrines or refuse dump sites (Texas Groundwater Production Committee, 2000). As a general guideline, it is recommended that the bottoms of pit latrines be at least 2 to 15 m above groundwater level, maintaining a minimum horizontal distance of 30 m from any water source to limit exposure to microbial contamination (United State Environmental Protection Agency, 2001; WEDC, 2012; NSDWQ, 2015). Poor construction of pit latrines by individuals unaware of their impact on groundwater in developing countries can lead to contamination with pathogenic bacteria, viruses, protozoa, and helminths that cause outbreaks of waterborne diseases (Bakobie et al., 2020).
The widespread use of pit latrines in rural and suburban areas contributes to the contamination of underground water, especially when safe distances between water points and pit latrines are not adequately maintained. The presence of pit latrines in close proximity has been identified as a key contributor to the deterioration of groundwater quality (Ishaku, 2011). Their design allows for the infiltration of wastewater into underground water and poses a potential source of contamination when not properly constructed (Keshavarzi and Moore, 2017; Ndoziya et al., 2019). Maintaining drinking water quality is critical for public health, covering factors such as physical, chemical, and microbiological aspects (WHO, 2010). Microbial contamination in groundwater presents a serious health threat for the inhabitants of Nigeria, particularly in the northern region, where infrastructure and hygienic practices are often inadequate. The presence of faecal coliform bacteria in groundwater indicates contamination with faecal matter. Therefore, the enumeration of faecal bacteria is essential in basic research, applied microbial ecology, and the development of parameter-based technologies for assessing drinking water quality. The quantity of water is important; ensuring its safe quality is crucial to mitigating waterborne illnesses, particularly in economically disadvantaged communities.
The evidence from numerous studies conducted over the years on groundwater contamination has revealed that the distance between pit latrines and well water plays a significant role in groundwater contamination. Other factors, such as seepage flow, soil particle size, soil pH, and temperature, also contribute. Islam et al. (2016) reported that groundwater in monitoring wells in Manda, Mohanpur, and Bagmara was found to be contaminated at lateral and vertical distances of 2 m and 31 m, respectively. High levels of microbiological contamination were detected in the groundwater; while most sampled wells demonstrated good bacteriological quality of water, the presence and extent of bacterial contamination varied across different hydrogeological conditions at both lateral and vertical distances. Bakari et al. (2023) reported elevated concentrations of nitrate and chloride with increasing well water depth and shorter distances from pit latrines, while faecal coliform counts indicated that for every unit increase in distance from the pit latrine to the shallow wells, the counts decreased by 42 cfu/100 mL.
There is a significant relationship between total coliform levels and the distance from the well. This study suggests that other factors may also contribute to the presence of total coliform bacteria in water wells located in squatter settlements in Zanzibar. Chauque et al. (2012) confirmed that wells are prone to high faecal contamination during the rainy season, with 84.4% showing levels of up to 139 cfu/100 mL, compared to the dry season, during which 42.4% of the samples showed levels of up to 12 cfu/100 mL. Furthermore, 56.9% registered pH values between 6.5 and 8.5, and 63.4% showed EC values ranging from 50 to 571 μS/cm due to seepage from latrines into shallow wells in peri-urban neighborhoods in Lichinga, Mozambique.
Usman and Aliyu (2020) reported that water samples from boreholes, hand pumps, and wells contained various forms of bacterial contaminants, exceeding the limits of 0 cfu/100 mL recommended by NSDWQ and WHO in the Hausari, Bulabulin, Tsohon Nguru, Sabon Gari, and Garbi communities of Nguru town in Yobe State. The presence of total coliform and E. coli suggests faecal contamination of the water sources due to their close proximity to pit latrines. Abdulkadir et al. (2015) reported that the water collected from the 12 sampling sites was contaminated with general and/or faecal coliforms and E. coli at varying levels, except for five sample sites that had no E. coli among the settlements of Dala Local Government Area in Kano State, Nigeria. It was generally observed that water samples near the pit latrines had relatively higher microbial loads.
The recent disease outbreaks in Nigeria recorded 816 deaths among 31,425 suspected cholera cases in August, 2021, according to the Nigeria Centre for Disease Control (NCDC). The outbreak affected many states, including Katsina. The death toll from the cholera outbreak in Katsina continues to rise as it spreads to more local governments, occurring in 25 out of the 34 local government areas of Katsina State. Approximately 1,534 people tested positive for the disease, while the death toll in Katsina State has increased to 75 cases. Funtua LGA reported the highest number of cases with 384, followed by Sabuwa with 232, Kafur with 215, Charanchi with 135, Kankara with 71, and Jibia with 69 cases. In terms of mortality, Funtua also leads with 18 deaths, followed by Charanchi with 14, Jibia with 11, and Kankara with five, while Rimi and Sabuwa each reported four. This situation resulted in the congestion of many hospitals and primary healthcare centers with patients suffering from various diseases. The incident highlighted the urgency of addressing water quality challenges (Adamawa State Ministry of Health Diary, 2021).
The rationale behind this research stems from a chlorella outbreak in the Funtua Metropolis that led to the deaths of many people. This outbreak may be attributed to excreta seepage contaminating groundwater due to the coexistence of pit latrines and wells used for domestic purposes. Bacteriological contamination of groundwater remains a major concern, particularly in areas where numerous dispersed, shallow-dug wells or boreholes provide protected but untreated domestic water supplies. The population density of microorganisms depends on nutrient supply and environmental factors, as most microbes can survive under a variety of conditions, which are greatly affected by the soil formation and hydrogeology of the area. To characterize the bacteriological safety of water, it is essential to conduct sanitary inspections and determine water quality (Some et al., 2021).
Considering these factors, this study focuses on assessing the impact of faecal contaminants from pit latrines on groundwater quality, as well as how nutrients and changes in physical–chemical properties affect microbial occurrence in groundwater. The aim is to elucidate the potential risks associated with their proximity to water sources. This research aims to raise public awareness of the dangers linked to non-compliance with the distance guidelines outlined by WEDC (2012), regarding the construction of pit latrines and well water.
2 Materials and methods
2.1 Study area and sampling stations
The study area is the Funtua Local Government Area of Katsina State, Nigeria. This Local Government Area (LGA) is located between latitudes 11°32’N and 11°53’N and longitudes 007°19′E and 007°32′E, as shown in Figure 1. It covers an area of 448 km2 with a population ranging from 225,571 to 570,110. As one of the premier local governments in Nigeria, it was established following the local government reforms of 1976. The headquarters of the Katsina South senatorial district comprises eleven local governments. Funtua lies at the southern end of Katsina State and is the second-largest city in the state after Katsina. It borders the Giwa LGA of Kaduna State to the south, Bakori LGA to the east, Danja to the southeast, Dandume LGA to the west, and Faskari LGA (Mai Ruwa village) to the northwest, all within Katsina State. The inhabitants of this Local Government Area are predominantly of the Hausa Fulani tribe, with their main occupations being trading, farming, and animal rearing (Karkarna and Dabo, 2021). The climate is classified as tropical wet and dry (Aw) according to the Köppen climate classification. The total annual rainfall averages about 1,000 mm, while the average minimum and maximum temperatures are 19°C and 32°C, respectively, with humidity at 44%. Overall, the climate varies significantly throughout the months and seasons: a cool, dry (harmattan) season from December to February; a hot, dry season from March to May; a warm, wet season from June to September; and a less marked season post-rains from October to November, characterized by decreasing rainfall and a gradual reduction in temperature (Abaje et al., 2014).
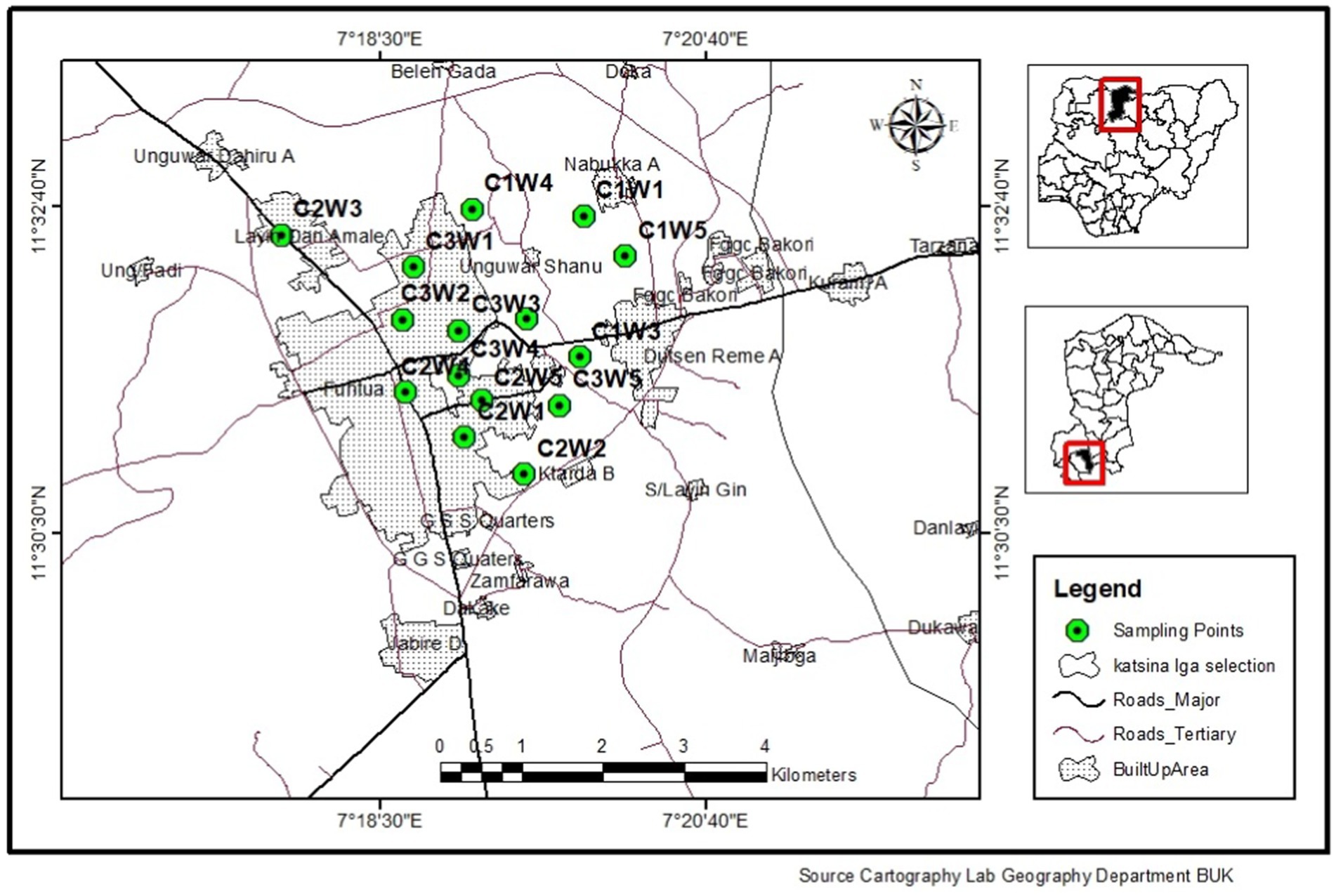
Figure 1. Map showing the sampling locations from wards in Funtua Local Government Area, Katsina State, Nigeria.
2.2 Water samples collection and analysis
Well-water samples were collected from fifteen different areas across eight wards with high cases of waterborne illnesses out of a total of 11 wards within the Funtua Local Government. Three wards were excluded as they had no record of chlorella outbreaks. Samples were collected twice each season, specifically early and late in both the dry and wet seasons, during the early hours between 7:00 and 10:00 am from the fifteen different sampling locations throughout the study area. The water samples were collected in 2-liter sterilized plastic bottles for physicochemical parameters and in sterilized 20-mL universal bottles for microbial analysis. The samples were placed in a cooler with ice packs and transported immediately to the laboratory for analysis to prevent changes in certain parameters or degradation of the samples. Due to their low stability, parameters such as temperature, conductivity, depth, and pH were determined immediately during the sampling period. The distance between pit latrines and well water sources was measured using a measuring tape, and the depth of the well was assessed with clean, calibrated rope and a measuring tape, which is essential for determining whether they comply with International Conventional Standards (WaterAid, 2011; WEDC, 2012; Jones, 2012; House et al., 2014).
2.3 Physicochemical analysis of the water samples
The physicochemical parameters analyzed include depth, water temperature, turbidity, pH, nitrate, phosphate, total dissolved solids (TDS), conductivity, chloride, chemical oxygen demand (COD), biochemical oxygen demand (BOD), dissolved oxygen (DO), sulphate and calcium (Adesakin et al., 2020). All samples were analyzed using the standard methods and procedures outlined by APHA (2005). Temperature, pH, conductivity, TDS, and DO were measured in situ with a multiparameter probe, while depth was determined using a clean rope and measuring tape. Turbidity was measured with a digital turbidity meter (HACH 2100 N). COD was determined using the reflux method, and calcium was analyzed using the ethylenediamine tetraacetic acid (EDTA) titrimetric method. Parameters such as nitrate, phosphate, chloride, bicarbonate, sulphate, and calcium were analyzed by adopting the standard methods and procedures established by Golterman et al. (1978), Ademoroti (1996), and APHA (2005).
2.4 Bacteriological analysis
A survey on the prevalence of waterborne diseases in primary healthcare centres and hospitals within the study area was conducted in collaboration with the Funta Local Government Area over a 1-year period.
2.4.1 Enumeration of coliform counts and faecal coliform counts
Serial dilutions of the water samples were conducted using tubes filled with 9 mL of sterile peptone water. Dilutions were prepared from 10−1 to 10−3. Nine tubes, each containing 9 mL of sterile lactose broth and inverted Durham tubes, were arranged. Exactly 1 mL of the 10−1 dilution was transferred into three sets of tubes, each containing 9 mL of sterile lactose broth and inverted Durham tubes labeled 10−1. This transfer was repeated with the 10−2 dilution into the second set of three tubes, which also contained 9 mL of sterile lactose broth and inverted Durham tubes, labeled 10−2, and repeated again with the 10−3 dilution into the third set of three tubes containing broth and inverted Durham tubes, labeled 10–3. The tubes were incubated for 24 h at 37°C for total coliform and at 40.5°C for faecal coliform, then checked for gas formation in the Durham tubes. After 24 h, the tubes showing gas production were scored as positive and compared with the Most Probable Number (MPN) table (Oyewalel et al., 2018).
2.4.2 Purification of bacterial isolates
Faecal coliforms were identified through cultural, morphological, and biochemical laboratory tests. The organisms were maintained on agar slants, specifically MacConkey agar (MA) and Eosin Methylene Blue (EMB) agar. Purification of E. coli was performed on EMB agar, resulting in a metallic green sheen, while Salmonella spp. and Shigella spp. were purified on XLD, yielding red colonies with black centres and pink/red colonies, respectively. The media were sterilised at 121°C and 1.05 kg/cm3 for 15 min in an autoclave. E. coli was enumerated using the membrane filtration method, filtering the water sample through a 0.45 μm membrane, with the colonies that grew on MacConkey agar identified as presumptive E. coli. These colonies were subsequently sub-cultured onto EMB agar for confirmation. The enumeration of Salmonella and Shigella species involved a two-step enrichment method. First, the water sample was incubated in sterile peptone water overnight. Then, 1 mL of the mixture was enriched in Rappaport-Vassiliadis enrichment broth for 24 h. The enriched sample was streaked onto xylose lysine deoxycholate (XLD) agar, with presumptive colonies confirmed using the Analytical Profile Index (API)-20E (Adesakin et al., 2020).
2.4.3 API 20E test
The organisms were confirmed using the Analytical Profile Index 20E, a standardized identification system for Enterobacteriaceae and Gram-negative rods. The API 20E strip consists of 20 microtubes containing dehydrated substrates to detect enzymatic activity or the fermentation of sugars by the inoculated organisms. The strips were inoculated with a 0.5 McFarland standard of the organisms, and sterile oil was added to the Arginine dihydrolase (ADH), Lysine decarboxylase (LDC), Ornithine decarboxylase (ODC), Hydrogen sulfide (H2S), and Urea (URE) compartments. The trays with the API test strips were incubated at 37°C for 18 to 24 h. After incubation, a drop of ferric chloride and Kovacs reagent was added to the tryptophan deaminase (TDA) and indole (IND) compartments, respectively, while a drop of 40% KOH (VP reagent 1) and a drop of α-Naphthol (VP Reagent 2) were added to the Voges Proskauer (VP) compartment. The color changes were compared against the API reading scale (color chart). The API 20E results were read in sets of three to generate a 7-digit profile, which was interpreted using the API 20E database to identify the organisms (Oyewalel et al., 2018).
2.5 Statistical analysis
The data obtained during this study was subjected to descriptive and inferential analysis; variance analysis (ANOVA) and Student’s t-test were used to compare the mean values of groundwater physicochemical parameters across different sampling stations and seasonal variations. Principal component analysis (PCA) was used to explore the interrelationships between water quality parameters and sampling locations. PCA also served to reduce the complexity of the data sets and to attribute concentration variations to significant processes that lead to groundwater source contamination. All statistics were analyzed using PAST (version 4), Microsoft Excel, and SPSS (version 25).
3 Results
3.1 Physico-chemical parameter of groundwater
The mean distance of wells from the pit latrine ranges from 7.00 ± 2.06 m to 15.01 ± 4.38 m, with the highest mean value recorded in the Tuduna Iya ward and the lowest observed in the Sabon Gari ward at 7.00 ± 2.06 m. There is a highly significant difference (p < 0.001) in the mean values of underground water distance to pit/soak-away latrines among the wards within Funtua Local Government, as presented in Table 1. The lowest mean well depth of 7.72 ± 0.15 m was observed in Makera ward, while the highest mean depth of 10.55 ± 0.38 m was recorded in Goya ward. The mean values of water temperature, EC, TDS, and chloride were significantly higher in the Goya ward compared to other sampling locations. The mean values of pH, phosphate, sulphate, and turbidity were relatively high in water samples collected from the Danduste ward. The minimum mean values of pH, phosphate, and sulphate (7.11 ± 0.13, 13.72 ± 2.47 mg/L, and 0.24 ± 0.07 mg/L, respectively) were observed in Unguwar Ibrahim ward, while a low turbidity mean value of 2.96 ± 0.31 NTU was recorded in Goya ward. The high mean concentrations of bicarbonate and calcium were recorded in Makera ward, whereas high mean concentrations of DO, nitrate, BOD, and COD were observed in Tudun Iya ward (Table 1).
The high mean concentrations of pH, bicarbonate, nitrate, COD, electrical conductivity, TDS, turbidity, and calcium (7.78 ± 0.37, 308.58 ± 142.56 mg/L, 11.49 ± 6.49 mg/L, 100.89 ± 16.38 mg/L, 2261.50 ± 430.35 μs/cm, 1231.65 ± 219.94 mg/L, 4.06 ± 0.36 NTU, and 89.00 ± 7.19 mg/L, respectively) were recorded in the Bagari sampling area of Makera ward (Supplementary Table 1). The low mean pH value of 6.98 ± 0.17 was observed in the Kasuwar Mata sampling area of Unguwar Ibrahim ward, while bicarbonate (73.96 ± 46.63 mg/L) was recorded in Unguwar Tukur of Unguwar Rabiu ward, and nitrate and COD (4.24 ± 0.77 mg/L and 26.22 ± 4.52 mg/L, respectively) were noted in Jabiri, Mai Gamji ward. Electrical conductivity (379.25 ± 182.75 μs/cm) was observed in Tudun Iya Mai of Tudun Iya ward, TDS (116.81 ± 13.34 mg/L) was measured in Unguwar Tukur of Unguwar Rabiu ward, turbidity (3.27 ± 0.26 NTU) was noted in Tudun Wada of Sabon Gari ward, and calcium (21.50 ± 4.35 mg/L) was found in Danlayi of Goya ward, as shown in Table 2. The high mean values of well depth and chloride (10.55 ± 0.38 m and 643.71 ± 123.05 mg/L, respectively) were observed in Danlayi of Goya ward, while water temperature (21.12 ± 1.95°C) was recorded in Filino Kwallo of Unguwar Ibrahim ward. High concentrations of phosphate and sulphate (19.23 ± 4.63 mg/L and 1.12 ± 0.64 mg/L) were recorded in Tsohuwan Kasuwar of Danduste ward, with DO (7.01 ± 1.67 mg/L) recorded in Tudun Iya Mai of Tudun Iya ward, and BOD (2.59 ± 0.49 mg/L) in Nasrawa of Unguwar Rabiu ward, as presented in Table 2. There is a highly significant difference (p < 0.001) in the mean values of depth, chloride, TDS, electrical conductivity, and calcium across all the sampling areas within the Funtua Local Government (Supplementary Table 1).
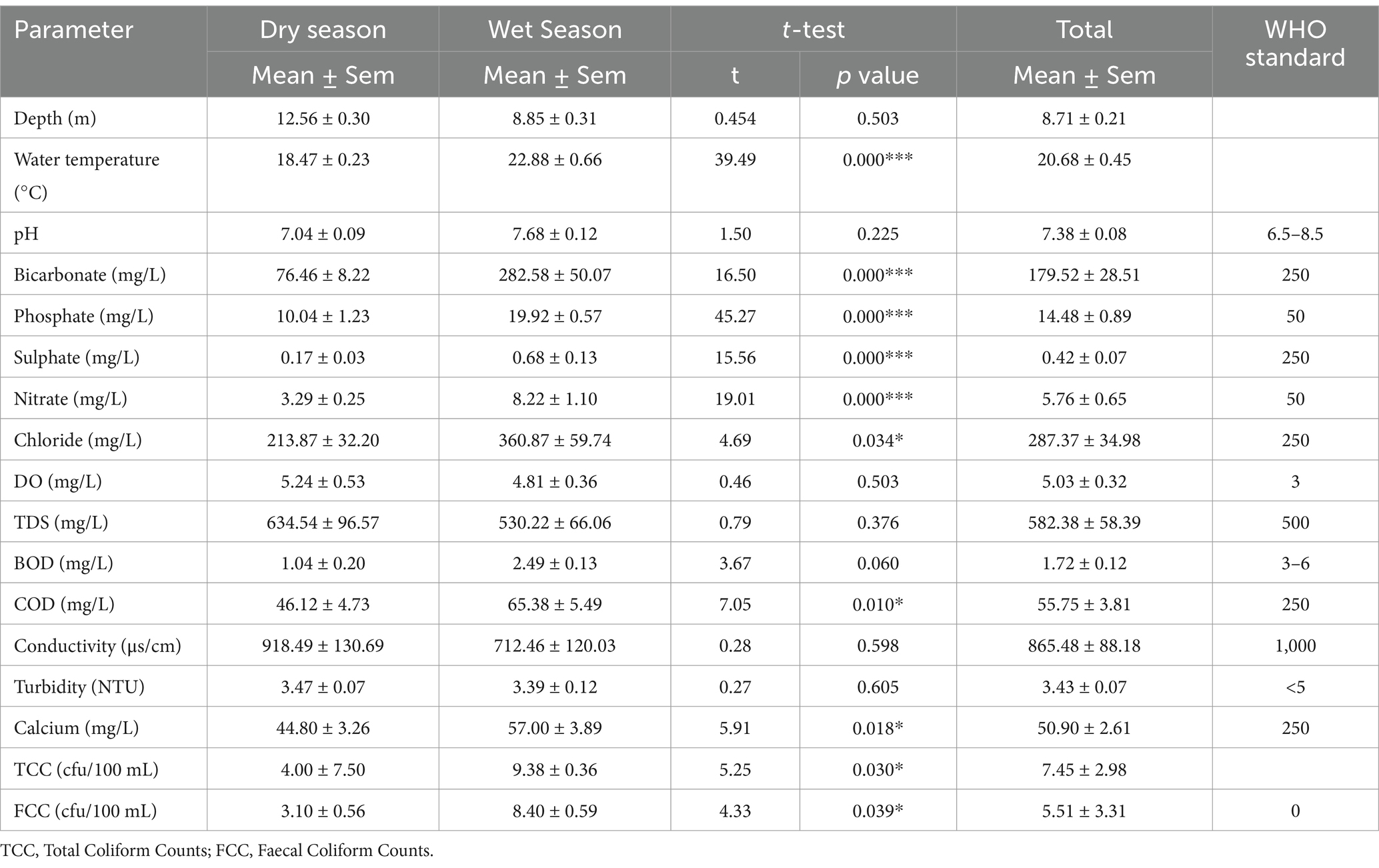
Table 2. Seasonal variation in the physicochemical parameters and bacteriological quality of groundwater in Funtua Local Government Area.
Seasonally, higher mean values of depth, DO, TDS, and electrical conductivity (12.56 ± 0.30 m, 5.24 ± 0.53 mg/L, 634.54 ± 96.57 mg/L, and 918.49 ± 130.69 μs/cm) were recorded during the dry season compared to the wet season, during which lower mean values were observed. In contrast, the highest mean values of water temperature, pH, bicarbonate, phosphate, sulphate, nitrate, chloride, BOD, COD, total coliform count, and faecal coliform count (7.68 ± 0.12°C, 282.58 ± 50.07 mg/L, 19.92 ± 0.57 mg/L, 0.68 ± 0.13 mg/L, 8.22 ± 1.10 mg/L, 360.87 ± 59.74 mg/L, 2.49 ± 0.13 mg/L, 65.38 ± 5.49 mg/L, 9.38 ± 0.36 cfu/mL, and 8.40 ± 0.59 cfu/mL) were recorded in the wet season compared to the dry season. There are significant differences (p < 0.05) in the mean values of water temperature, bicarbonate, phosphate, sulphate, nitrate, chloride, COD, calcium, and total coliform count between the seasons (Table 2). Figures 2, 3 illustrate the interrelationship between the physico-chemical parameters of groundwater and different wards within the Funtua Local Government Area.
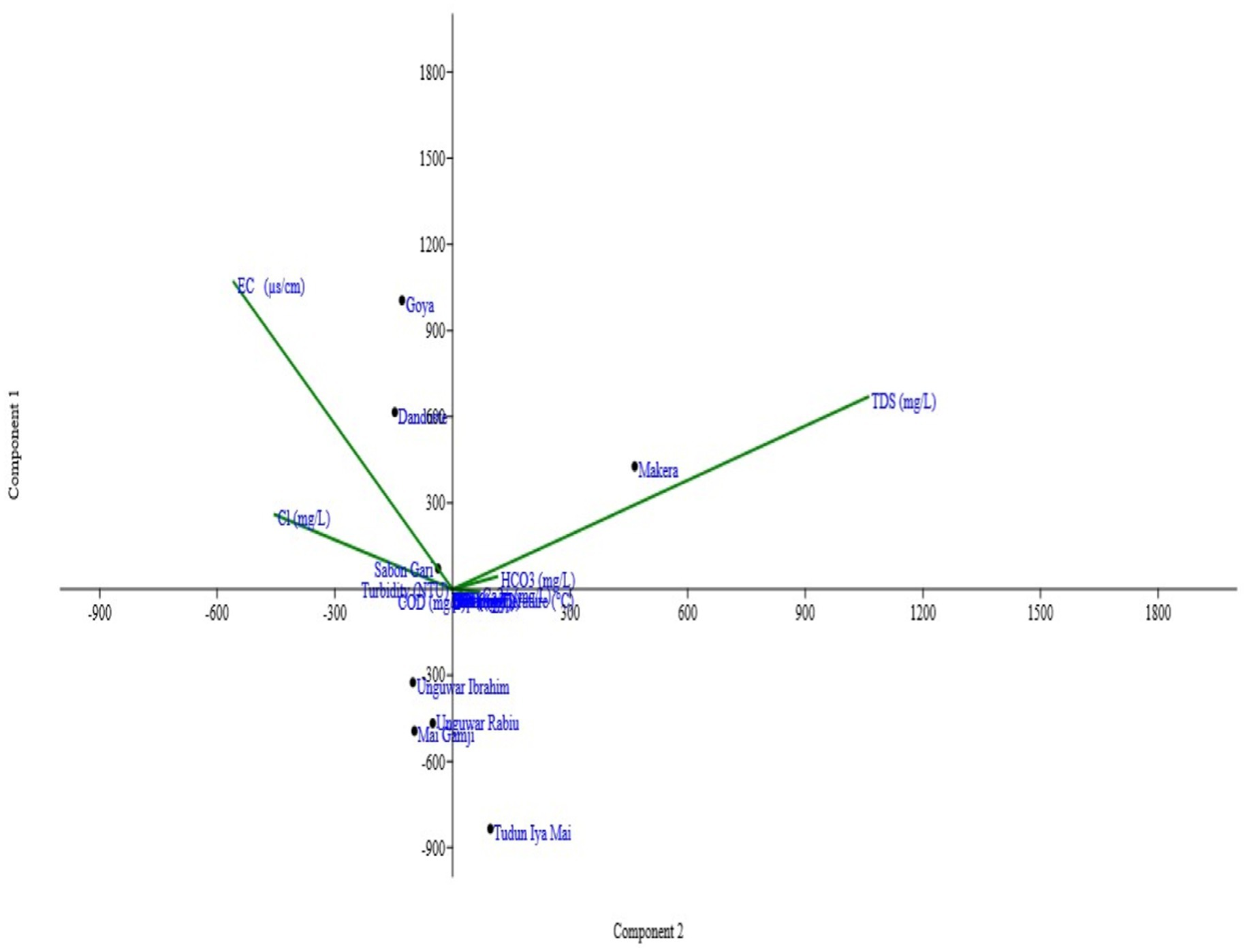
Figure 2. PCA showing the relationship between physicochemical parameters of groundwater and the wards of Funtua during the dry season.
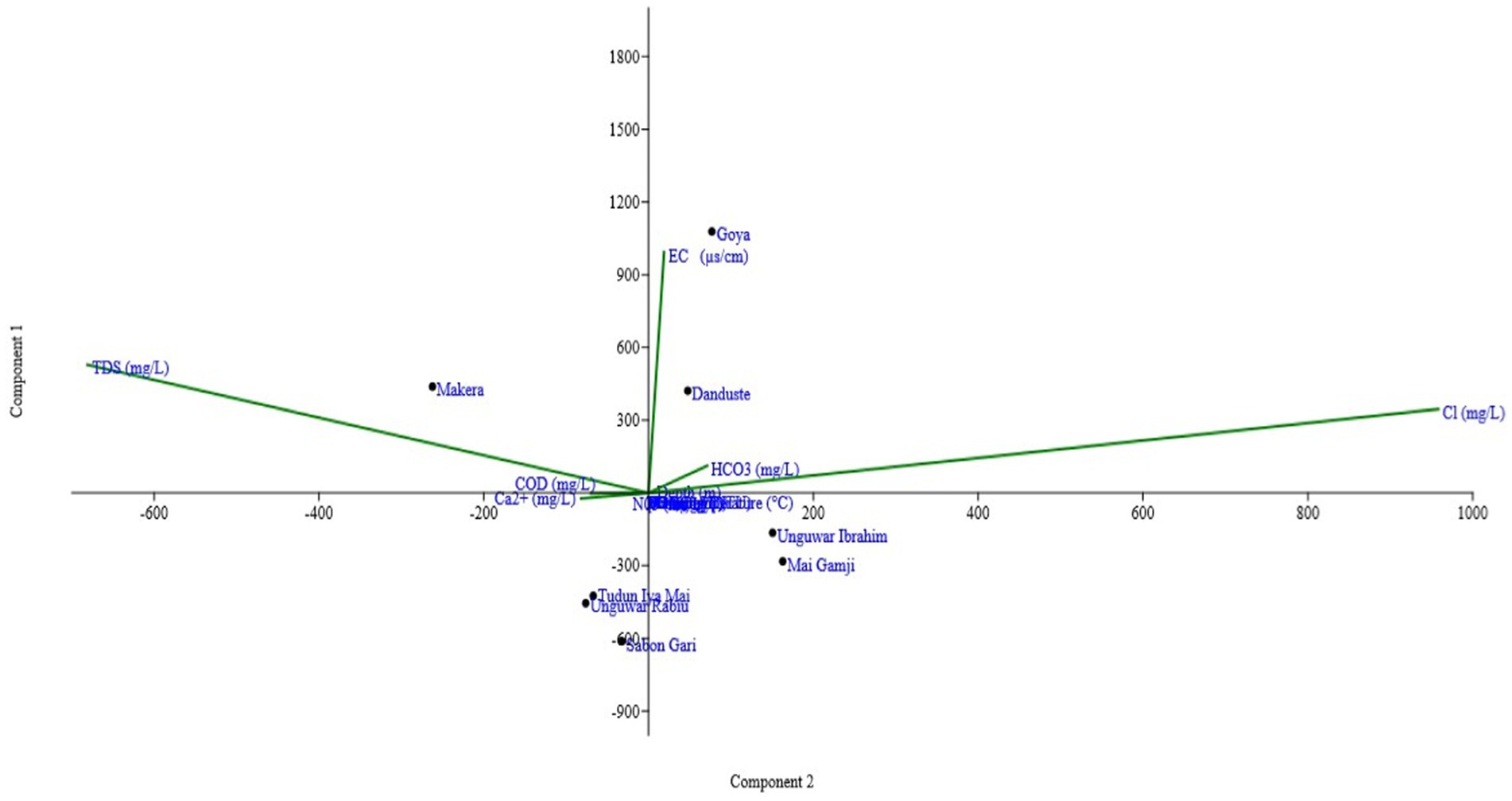
Figure 3. PCA illustrating the relationship between physicochemical parameters of groundwater and Funtua wards during the wet season.
3.2 Bacteriological quality
The highest total coliform counts were recorded in the Sabon Gari ward (93 cfu/mL), followed by the Unguwar Rabiu ward (75 cfu/mL), while the Tudun Iya ward had the lowest count of 3 cfu/mL as presented in Table 1. The highest faecal coliform count was also recorded in the Sabon Gari ward (17 cfu/mL), whereas the lowest was observed in the Tudun Iya ward (0 cfu/mL). The highest total coliform counts were recorded in the Tudun Wada area of the Sabon Gari ward, while faecal coliform counts were observed in the Unguwar Tukur area of the Unguwar Rabiu ward, as shown in Figure 4. High total coliform and faecal coliform counts were recorded during the wet season compared to the lower counts observed in the dry season (Table 2). There are significant differences (p < 0.05) in the mean values of total coliform and faecal coliform counts between the two seasons (Table 2).
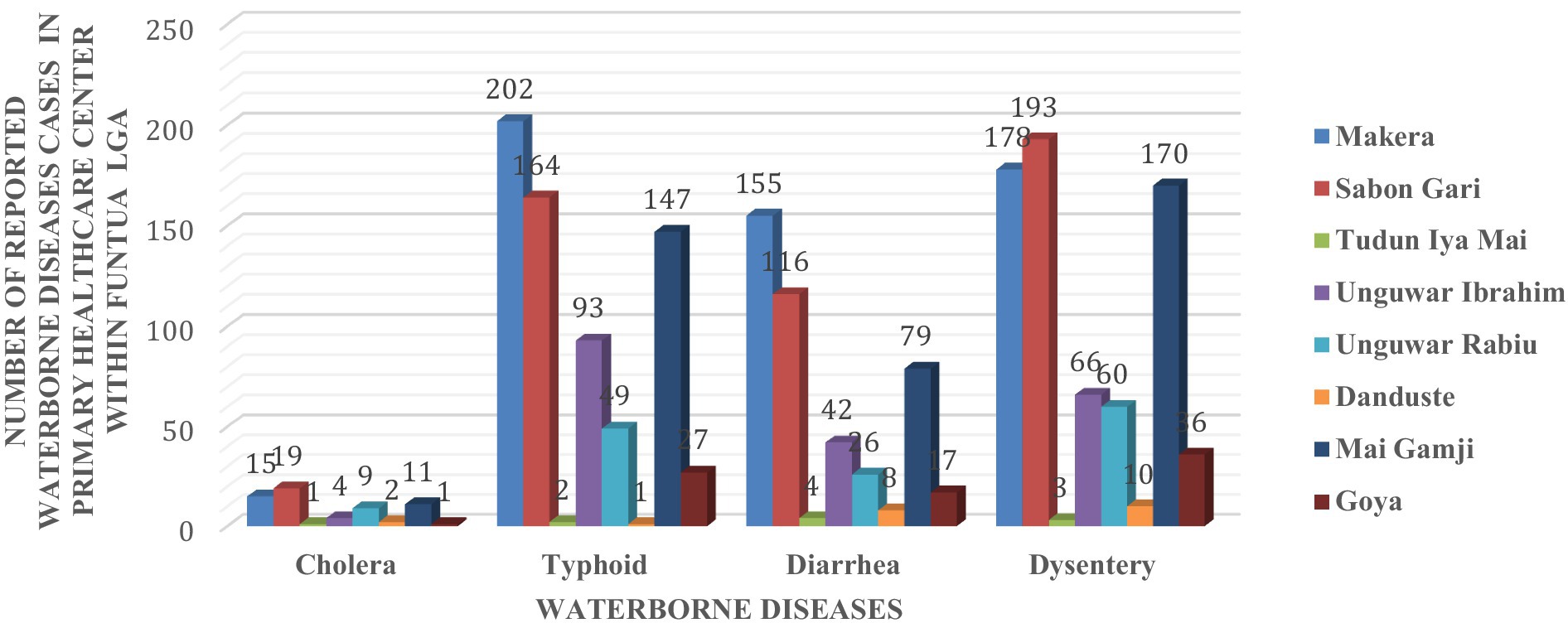
Figure 4. The prevalence of waterborne diseases reported in primary healthcare centers within the Funtua Local Government Area.
The prevalence of waterborne diseases was highest in the Danduste ward, followed by the Unguwar Rabiu ward and the Tudun Iya Mai ward, with the fewest occurrences recorded in the Goya ward (Figure 4). The highest number of cholera cases was found in the Sabon Gari ward, with a total of 19 cases, followed by the Makera ward, with 15 cases, while the least was reported in the Tudun Iya ward, with only one case, as shown in Figure 4. The highest incidence of typhoid was recorded in the Makera ward, with 202 cases, followed by the Sabon Gari ward, with 164 cases, and the fewest typhoid occurrences were in the Tudun Iya ward, with 2 cases. The Makera ward also reported the highest number of diarrhoea cases (155), followed by the Sabon Gari ward (116), while the Tudun Iya ward had significantly fewer diarrhoea cases (4). The largest number of dysentery cases was recorded in the Sabon Gari ward, with a total of 193 cases, followed by the Makera ward with 178 cases, and the Danduste ward had the least at 10 cases (Figure 4). The highest total coliform counts were observed in the Tudun Wada area, followed by the Filino Kwalli area, whereas faecal coliform counts were elevated in the Unguwar Tukur area, followed by the Unguwar Dahiru area in the Funta Local Government Area (Figure 5).
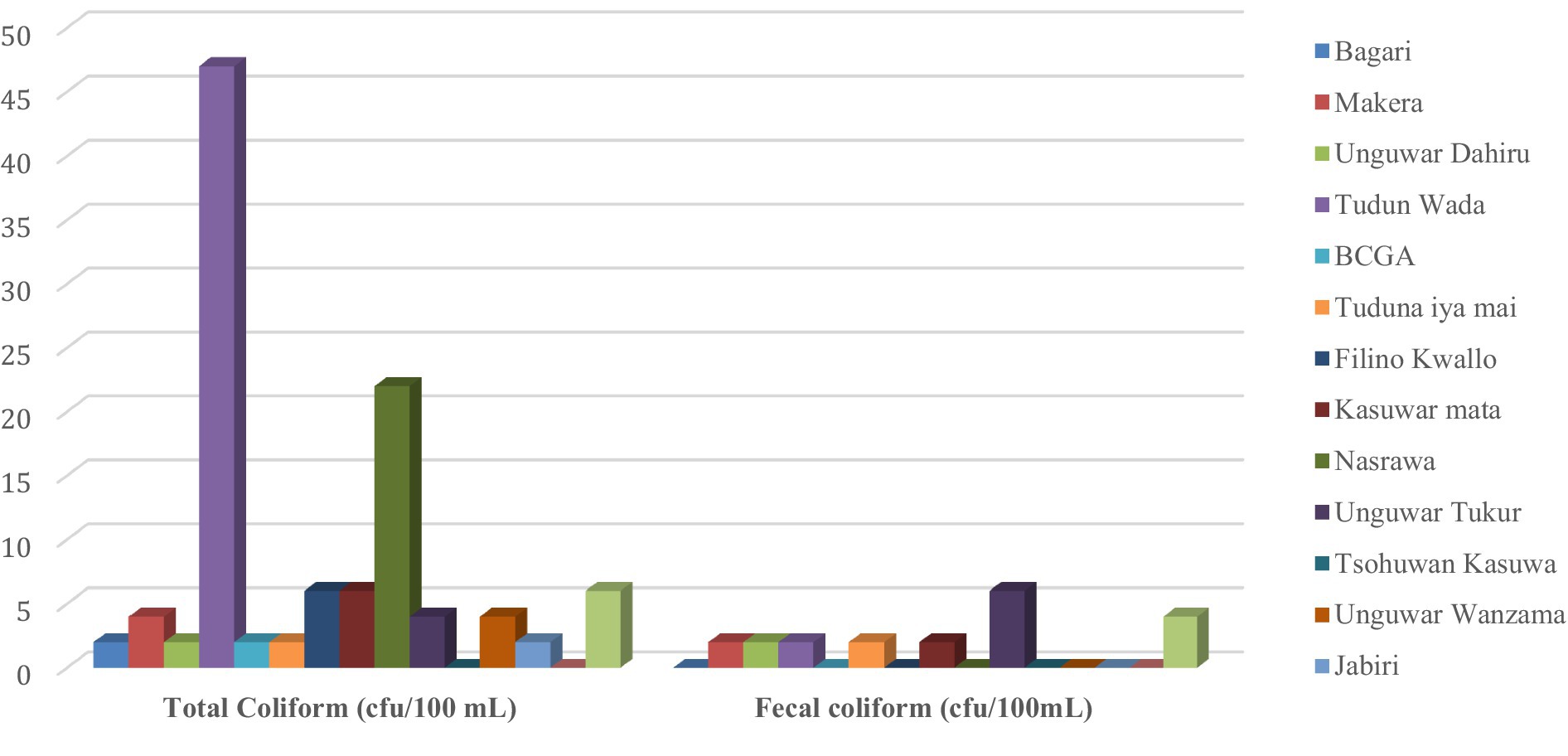
Figure 5. Bacteriological quality of groundwater recorded in different areas within the Funtua Local Government Area.
There is a correlation between total coliform counts, faecal coliform counts, well distance from the latrine, well depth, phosphate, chloride, TDS, conductivity, turbidity, and calcium in Makera Ward, while bicarbonate, sulfate, TDS, and COD correlate with total coliform counts and faecal coliform counts in Sabon Gari Ward (Table 1). In Unguwar Ibrahim, the well distance from the latrine, well depth, water temperature, bicarbonate, sulphate, chloride, DO, TDS, BOD, COD, EC, and turbidity are correlated with total coliform counts and faecal coliform counts.
Alternatively, in the groundwater at Unguwar Rabiu, the well distance from the latrine, bicarbonate, sulphate, chloride, TDS, BOD, COD, and conductivity correlate with total coliform counts and faecal coliform counts. In Danduste Ward, the well distance to the latrine, pH, phosphate, sulfate, nitrate, chloride, DO, TDS, BOD, COD, turbidity, and calcium correlate with total coliform counts and faecal coliform counts in well water, whereas in Mai Gamji Ward, the well distance from the latrine, well depth, bicarbonate, phosphate, sulphate, nitrate, chloride, DO, TDS, BOD, COD, turbidity, and calcium correlate with total coliform counts and faecal coliform counts recorded in groundwater. Additionally, bicarbonate, phosphate, sulphate, TDS, BOD, turbidity, and calcium also correlate with total coliform counts and faecal coliform counts observed in well waters from Goya Wards within Funta Local Government Area (Supplementary Table 2).
4 Discussion
4.1 Spatial variation
Based on the correlation between the distances of well water to pit latrines and the counts of total coliform and faecal coliform, this study indicates that most of the groundwater analyzed is polluted with faecal matter, likely due to the improper or inadequate positioning of the well water sources in relation to the latrines. This variation raises concerns about the potential contamination of water sources, as coliform bacteria can leach from pit latrines into nearby wells. The average distance from well water to pit latrines in this study is similar to that reported by Ahaneku and Adeoye (2014), who indicated a range of 6.1 to 17.9 meters for groundwater distances to pit latrines in Fokoslum, Ibadan, Nigeria, and by Bakari et al. (2023), who documented a range of 10 to 38 m in a squatter settlement in Zanzibar, Tanzania. However, the results of this study contradict the recommendations of Selendy and Janine (2011), who advised that the average distance between well water sources and pit latrines should be at least 30 m. Additionally, Vinger et al. (2012) noted that wells face contamination risks if located less than 12 m from pit latrines.
Wells located downstream from sources of contamination are more susceptible to leaching, allowing excreta from pit latrines to enter nearby wells more easily. The mean depth of the well water measured in this study ranged from 7.72 ± 0.15 to 10.55 m, possibly due to the area’s geology and the shallow nature of the wells. Shallow wells are more likely to be affected by activities such as agricultural runoff, septic systems, and industrial discharges. Pathogens from nearby pit latrines or septic systems can infiltrate shallow groundwater sources more easily, potentially compromising water quality.
The results obtained from this study were similar to the report by Abdulkadir et al. (2015), who recorded a range of 5.1 to 8.5 m in well water from the Dala Local Government Area, Kano State, and to Maju-Oyovwikowhe and Emofurieta (2021), whose reported depth range was 3.73 to 8.45 m from hand-dug wells in Ososo Town, Akoko-Edo, Edo State. The mean temperature, which ranged from 20.50 ± 1.07°C to 21.08 ± 1.97°C, can generally influence water quality and were within the recommended limits set by WHO (2011) and NSDWQ (2015). The mean turbidity value ranged from 2.96 ± 0.31 NTU to 3.87 ± 0.29 NTU recorded across all sampling areas, indicating that the well water in this area is relatively clear and free from suspended solids. This finding contrasts with the report by Adekola et al. (2015), who recorded turbidity values ranging from 6.12 to 60.00 NTU in groundwater from the Gassol area in Taraba State, Nigeria.
The pH values recorded from all sampling areas were within the acceptable limits for drinking water set by WHO (2011) and NSDWQ (2015), except for the pH value recorded from the Kasuwar Mata sampling area in Unguwar Ibrahim ward, which may be due to ion dissociation from soil composition into aquifer water. The results of this study are similar to those reported by Fadiji et al. (2019). The findings indicate that low electrical conductivity values were observed compared to the range of 300–2,230 μS/cm reported by Musa et al. (2023).
The high concentrations of TDS and electrical conductivity recorded from the Goya, Danduste, and Makera wards were compared with those from other wards. This may be due to the influence of pit latrine seepage water, which is rich in ions absorbed by the soil and could lead to increased ion concentration in underground water, as indicated by this study. Comparatively, the TDS results of this study were similar to those reported by Abdulkadir et al. (2015), who found TDS ranging from 360 to 6,000 mg/L in groundwater samples from the Dala Local Government Area of Kano State, Nigeria. However, these results were significantly higher than those of a similar study by Fadiji et al. (2019), who reported TDS values ranging from 2.77 ± 0.15 to 4.5 ± 0.07 mg/L from wells in Ijero-Ekiti, Ekiti State, Nigeria.
The observed range of mean DO concentrations was between 4.33 ± 0.63 and 7.01 ± 1.67 mg/L, likely due to the shallow depth of most wells, which allows oxygen from the air to dissolve in the groundwater. This finding aligns with a report by Ekute (2021) on groundwater in the vicinity of an industrial area in the Mopin Community, Ota, South-Western Nigeria. The BOD values obtained in this study were below the 3.66 mg/L reported by Nwidu et al. (2008). COD concentrations ranged from 35.94 ± 5.90 mg/L to 99.99 ± 16.27 mg/L, with the highest value observed in the Tudun Iya Mai wards. This contamination could arise from the proximity of pit latrines to the water sources. High COD levels can foster the growth of microorganisms that cause waterborne diseases such as cholera, typhoid, and hepatitis (World Health Organization, 2008). This study contradicts the findings of Raji et al. (2019), who reported COD values of 1.0–1.1 mg/L in Okada Town, Edo State, Nigeria.
The highest mean concentrations of bicarbonate and calcium were recorded in Makera ward, while sulphate was observed in Danduste ward, which could be attributed to the addition of ions in the groundwater, potentially originating from pit latrines, soak pits, and other sources. The sulphate values obtained in this study remained below the maximum limit of 250 mg/L set by the WHO (2011) and NSDWQ (2015). According to NSDWQ (2015), sulphate does not have a direct health impact on drinking water; rather, it can cause a bitter taste and odors. Abubakar and Saidu (2022) reported sulphate values ranging from 0.29 mg/L to 1.33 mg/L, which are similar to the values obtained in this study. In comparison, the results of this study showed higher bicarbonate concentrations than the values of 27.7–90.0 mg/L reported by Maju-Oyovwikowhe and Emofurita (2021).
The mean concentration of phosphate ranged from 13.72 mg/L to 19.23 mg/L, with the highest concentration (19.23 mg/L) observed in the Danduste ward. This result significantly differed from the report by Iliyasu et al. (2018), who recorded a range of 0.25 to 0.80 mg/L in wells within Kano Metropolis, Nigeria. The mean nitrate concentration varied from 4.40 mg/L to 8.22 mg/L during this study, remaining within the WHO (2011) permissible limits for drinking water. High chloride levels were recorded in the Danduste, Mai Gamji, and Goya wards, indicating that chloride levels in most water samples exceeded the acceptable limit of 250 mg/L recommended by WHO (2011) and NSDWQ (2015). However, the findings of this study were similar to the report by Fadiji et al. (2019), which noted a chloride concentration of 645 mg/L in wells; elevated chloride levels (> 250 mg/L) indicate a risk of pollution in the water.
4.2 Seasonal variation
The high mean depth recorded during the dry season may result from a reduction in underground water volume during this period, while the highest water temperature recorded in the wet season can be attributed to the rainfall pattern in this study area. Similar findings by Gongden and Lohdip (2015) in Plateau State reported a comparable temperature value of 25.67 ± 3.03°C. The mean turbidity value recorded during the dry season was slightly higher than that in the wet season, but it remained below the standards set by NSDWQ (2015) and WHO (2011). The soil particles are very small and light, making them easily dislodged from the well walls by water movement during water fetching.
The leakage of pit latrine waste materials into aquifer water and the subsequent loosening of the soil matrix could be the cause of these high turbidity values. The mean pH value recorded during the wet season was slightly higher than that in the dry season, which may be due to increased runoff into the groundwater during the wet season caused by heavy downpours, potentially leading to elevated mineral concentrations and, consequently, a higher pH level. The high TDS and electrical conductivity (EC) values observed during the dry season, in comparison to the wet season, may result from reduced water levels during this period, as well as seepage from pit latrines into the groundwater.
The mean DO recorded during the dry season was higher than during the wet season, possibly due to the shallow depth of most of these wells, which allows atmospheric oxygen to dissolve into the underground water. The high BOD concentration observed during the wet season, compared to the dry season, could be due to the increased organic matter content from agricultural waste entering these wells through runoff during this period.
High concentrations of calcium and bicarbonate were recorded in the wet season rather than the dry season, while a high sulphate value was observed during the dry season. This trend could be attributed to human activities such as agricultural runoff and industrial waste, along with rainwater infiltrating the ground, which causes an increase in ions. The mean phosphate value during the wet season was notably higher than that during the dry season. The phosphate value in this study suggests contamination of groundwater (well) with potentially human waste from sources such as soak pits and pit latrines.
Such contamination can introduce pathogens into the water, posing health risks. A high mean nitrate value was recorded in the wet season compared to the dry season. This may be attributed to poor well construction, as evidenced by cracks in the casing of some wells in the study area. Vulnerabilities in wells could lead to contamination, particularly during the wet season when nitrate-rich water from nearby sources like pit latrines may infiltrate the well. It is important to regulate nitrate levels in drinking water due to the significant health risks they pose.
Excessive nitrates in drinking water can have significant health implications, such as hypertension and methemoglobinemia (Mkadmi et al., 2018). The higher chloride concentration in well water during the wet season could be due to the addition of chlorine to purified well water, which leads to high runoff associated with this season. The mean COD during the wet season was slightly higher than during the dry season, possibly due to organic matter from runoff and seepage from pit latrines contaminating the well water.
The presence of bacterial species such as Escherichia coli, Shigella dysenteriae, and Salmonella typhi in the examined well water samples may stem from pathogens and bacteria from pit latrines infiltrating the soil and contaminating the groundwater. The detection of Shigella and E. coli in most water samples suggests that the well water sources likely came into contact with human or animal faeces. These findings corroborate the work of Some et al. (2021), Belina et al. (2023), and Wamyil et al. (2023), who reported Escherichia coli, Shigella spp., and Salmonella spp. as the most commonly isolated bacterial species in water sources. Similarly, it aligns with the research of Odonkor and Addo (2018), which reported a high prevalence of Shigella and Salmonella in drinking water sources.
Auta et al. (2021) reported high occurrences of Salmonella and E. coli in hand-dug well water in selected areas of the Rigachikun community, Kaduna. The total coliform bacterial counts ranged from 3 cfu/100 mL to 93 cfu/100 mL, with the highest counts observed in the Sabon-Gari and Unguwar Rabiu wards. The results of this study could be attributed to the close proximity of the wells to pit latrines, similar to the findings of Opara et al. (2011), who noted microbial contamination in water sources located within 27 m of a soak pit in Southeastern Nigeria. This finding aligns with Akinbile and Yusoff (2011), who reported total coliform counts exceeding the WHO (2011) recommended limit of 0 cfu/100 mL.
Total coliform bacterial counts and total faecal coliform counts were higher during the wet season compared to the dry season. This increase may be due to high runoff containing faecal droppings from infected cows grazing, as herdsmen access these wells during this season. The faecal coliform counts observed in this study ranged from 0 cfu/100 mL to 17 cfu/100 mL. The highest faecal coliform counts recorded at Sabon Gari and Goya wards were also associated with the highest number of disease cases [cholera (19), typhoid (164), diarrhoea (116), and dysentery (193)] reported in their primary health care centers in Funtua Local Government Area. It is likely that the elevated faecal coliform counts in well-water samples result from contamination by nearby pit latrines. Pit latrines are known to be significant sources of harmful microorganisms or pathogens that contaminate underground water due to the presence of faecal matter. The level of faecal contamination in this study indicates that most of the sampled well water is not suitable for drinking and exceeds the WHO (2011) and NSDWQ (2015) recommended standard of 0 cfu/100 mL.
Faecal coliform contaminants in water can lead to waterborne diseases such as cholera, typhoid, dysentery, and diarrhoea, among other illnesses, particularly gastrointestinal infections (Joseph and David, 2011). These infections can manifest as diarrhoea, abdominal pain, vomiting, nausea, and, in severe cases, even life-threatening conditions (Ohwo and Omidiji, 2021). Total coliform bacteria and total faecal coliform levels were found to be higher in the wet season, possibly due to the area’s soil texture, where sand forms the top layer and lacks the ability to absorb water, resulting in a runoff.
Open defecation is prevalent in this region, and disease-ridden cows contribute to contamination as herdsmen and their livestock move or graze openly due to the abundance of grasses. Runoff can then wash contaminants into many wells. These results align with those reported by Fadiji et al. (2019), indicating a high prevalence of water-related diseases in the Ijero-Ekiti community of Ekiti State, Nigeria. Similarly, Iren et al. (2019) noted a high incidence of diarrhoea, dysentery, and typhoid in the Santa Sub-Division of the North West Region of Cameroon. Water-related infectious diseases are a significant cause of illness and death, primarily due to unsafe water, inadequate sanitation, and poor hygiene practices (Water Aid, 2009; Hynds et al., 2012).
The Principal Component Analysis revealed that the majority of physicochemical parameters and bacteriological quality clustered during the dry season while they were scattered in the wet season. This might be due to increased water concentration in the dry season and dilution in the wet season. The physicochemical parameters of groundwater collected from various wards within the Funtua Local Government Area fluctuate, indicating that the geology or soil type of an area plays a crucial role in determining groundwater quality.
Based on the phosphate levels correlated with Total Coliform Counts (TCC) and Faecal Coliform Counts (FCC) across all sampling sites, phosphate is identified as a major contributor to microbial occurrence in well waters in this study when compared to nitrate concentration. This finding aligns with the study by Douterelo et al. (2020), which reports that phosphate increased the relative abundance of bacteria such as Pseudomonas, Paenibacillus, Massilia, Acinetobacter, and the fungi Cadophora, Rhizophagus, and Eupenicillium in water. Furthermore, limiting nutrients is considered beneficial for microbial growth in drinking water systems. DO also correlated with microbial presence at all sampling sites, implying that it contributed to microbial distribution in well waters.
5 Conclusion
Based on this study’s findings, the analyzed physicochemical parameters were mostly within the permissible limits set by WHO and NSDWQ in certain wards of the Funtua Local Government Area. However, the observed concentrations of DO, chloride, TDS, EC, total coliform counts, and faecal coliform counts during this study exceeded the recommended limits by WHO.
Phosphate and DO are considered major elements contributing to microbial growth in the well waters from this study, as they showed a positive correlation with Total Coliform Counts (TCC) and Faecal Coliform Counts (FCC) at all sampling stations, more so than nitrate. This indicates that the groundwater is contaminated with faecal matter, making the water unfit for human consumption without prior treatment. This poses a significant health risk, as it suggests the presence of pathogenic bacteria and potential waterborne diseases. The high occurrence of faecal coliform counts observed during this study indicates that much of the underground water in this area is contaminated, which could result in a high number of reported infection cases at their primary health centre since waterborne diseases are caused by drinking contaminated water or consuming contaminated foods. Based on the results of this study, many people in this area are still drinking contaminated water, which contradicts the MDGs goal of achieving access to sustainable, safe drinking water, basic sanitation, and the eradication of related waterborne diseases globally.
5.1 Recommendations
Authority concerns should develop a practical action plan, such as improving sanitation infrastructure in these sampling areas, providing public health education on water treatment methods, and implementing policies for safe well and pit latrine construction in this Local Government Area.
Data availability statement
The raw data supporting the conclusions of this article will be made available by the authors, without undue reservation.
Author contributions
LO: Conceptualization, Data curation, Formal analysis, Methodology, Software, Validation, Visualization, Writing – original draft, Writing – review & editing, Investigation, Project administration, Resources, Supervision. AI: Conceptualization, Data curation, Formal analysis, Investigation, Methodology, Project administration, Resources, Software, Supervision, Validation, Visualization, Writing – original draft. TA: Conceptualization, Data curation, Formal analysis, Methodology, Software, Validation, Visualization, Writing – original draft, Writing – review & editing. JO: Conceptualization, Data curation, Methodology, Validation, Visualization, Writing – review & editing.
Funding
The author(s) declare that no financial support was received for the research and/or publication of this article.
Conflict of interest
The authors declare that the research was conducted in the absence of any commercial or financial relationships that could be construed as a potential conflict of interest.
Generative AI statement
The authors declare that no Gen AI was used in the creation of this manuscript.
Publisher’s note
All claims expressed in this article are solely those of the authors and do not necessarily represent those of their affiliated organizations, or those of the publisher, the editors and the reviewers. Any product that may be evaluated in this article, or claim that may be made by its manufacturer, is not guaranteed or endorsed by the publisher.
Supplementary material
The Supplementary material for this article can be found online at: https://www.frontiersin.org/articles/10.3389/frwa.2025.1561777/full#supplementary-material
References
Abaje, I. B., Sawa, B. A., and Ati, O. F. (2014). Climate variability and change, impacts and adaptation strategies in Dutsin-ma local government area of Katsina state, Nigeria. J. Geo. Geol 6, 103–112. doi: 10.5539/jgg.v6n2p103
Abdulkadir, R. S., Mahmoud, A. M., Adnan, A., Shamsuddeen, U., Adamu, R. T., and Yunusa, I. (2015). Effect of pit latrine leaks on shallow well water effect of pit latrine leaks on shallow well water. Int. J. Microbiol. Appl. 1, 46–51.
Abubakar, A. U., and Saidu, M. D. (2022). Assessment of Some physicochemical parameters in borehole water samples drilled near public conveniences in Kano Metropolis, Nigeria. Chem Sear. J. 13, 94–105.
Adamawa State Ministry of Health Diary (2021). Adamawa State Ministry of Health Diary : Adamawa State Government. Available at: https://en.wikipedia.org/wiki/Adamawa_State
Adekola, O., Abubakar, B., and Abdul-Munin, K. (2015). Physico-chemical characteristics of borehole quality in Gassol Taraba state, Nigeria. Afri. J. Environ. Sci. Technol. 9, 143–154. doi: 10.5897/AJEST2014.1794
Adesakin, T. A., Oyewale, A. T., Bayero, U., Mohammed, A. N., Aduwo, I. A., Ahmed, P. Z., et al. (2020). Assessment of bacteriological quality and physico-chemical parameters of domestic water sources in Samaru community, Zaria, Northwest Nigeria. Heliyon 6:e04773. doi: 10.1016/j.heliyon.2020.e04773
Ahaneku, I. E., and Adeoye, P. A. (2014, 2014). Impact of pit latrines on groundwater quality of Fokoslum, Ibadan, southwestern Nigeria. Br. J. Appl. Sci. Technol. 4, 440–449. doi: 10.9734/BJAST/2014/5079
Ajibade, O., Oke, I. A., Adeyemi, S., and Adesina, A. (2013). Water supply in selected villages in Katsina state, Nigeria. Igdr Univ. J. Instit. Sci. Technol. 3, 19–30.
Akinbile, C. O., and Yusoff, M. S. (2011). Environmental impact of leachate pollution on groundwater supplies in Akure, Nigeria. Int. J. Environ. Sci. Develop. 2, 81–86. doi: 10.7763/IJESD.2011.V2.101
APHA (2005). Standard methods for the examination of water and wastewater. 21st Edn. American public health association American water works association : Water Environment Federation (WEF).
Auta, K. I., Lawal, A., Basira, I., Mohammed, S. A., and Isaac, I. A.. (2021). Bacteriological and parasitological analysis of hand-dugged well water in selected areas of Rigachikun community, Kaduna. Sci. Wor. J. 16, 428–432.
Bakari, S. S., Suleiman, Z. N., Ali, H. R., and Kai, K. H. (2023). Impact of pit latrines on groundwater quality in squatter settlements in Zanzibar. Earth Environ. Sci. Res. Rev., 1–10. doi: 10.33140/EESRR.06.03.03
Bakobie, N., Ibrahim, A. R., and Duwiejuah, A. B. (2020). Sanitation practices and microbial quality of drinking water in open Defaecation free and open Defaecation communities in the Savelugu municipality, Ghana. Ghana J. Sci. 61, 1–12. doi: 10.4314/gjs.v61i2.1
Belina, D., Gobena, T., Kebede, A., Chimdessa, M., Hailu, Y., and Hald, T. (2023). Occurrence of Diarrheagenic pathogens and their coinfection profiles in diarrheic under five children and tracked human contacts in urban and rural settings of eastern Ethiopia, sage. Micro. Insight 16, 1–11. doi: 10.1177/11786361231196527
Chauque, B. J. M., Chicumbe, C. M., Cossa, V. C., and Rott, M. B. (2012). Spatial management of well and latrine and their influence on water quality in clayey soil- a study in low-income peri-urban neighborhoods in Lichinga, Mozambique. J. Water Sanit. Hygiens Dev, 1–14. doi: 10.2166/washdev.2021.137
Douterelo, I., Dutilh, B. E., Calero, C., Rosales, E., Martin, K., Husband, S., et al. (2020). Impact of phosphate dosing on the microbial ecology of drinking water distribution systems: Fieldwork studies in chlorinated networks. Water Research. 187:e116416.
Ekute (2021). Physicochemical analysis of groundwater in the vicinity of an industrial area of Mopin community, Ota, South-Western Nigeria. Sci. Wor. J. 16, 23–67.
Fadiji, A. E., Omomowo, I. O., Adebayo, P. A., Omomowo, I. O., and Awogbami, S. (2019). Effect of pit latrines on hand Dugwell water quality - a case study of ijero-Ekiti community in Ekiti state, Nigeria. Int. J. Technic. Res. Sci. 4, 1–12. doi: 10.30780/IJTRS.V04.I06.001
Falkenmark, M., and Rockstrom, J. (2006). The new blue and green water paradigm: breaking new ground for water resources planning and management. J. Wat. Resour. Plann. Manag. 132, 129–132. doi: 10.1061/(ASCE)0733-9496(2006)132:3(129)
Golterman, H. L., Clymo, R. S., and Ohnstand, M. A. M. (1978). Methods for physical and chemical analysis of freshwater. second Edn. Oxford: Blackwell Scientific Publication.
Gongden, J. J., and Lohdip, Y. N. (2015). Seasonal variation of the surface water quality of two dams in plateau state, north Central Nigeria. Internat. J. Environ. Impt. 6, 291–298.
House, S., Suzanne, F., Marni, S., and Sue, C. (2014). Violence, gender & WASH: A Practitioner's toolkit – Making water, sanitation and hygiene safer through improved programming and services. London, UK: WaterAid/SHARE.
Hynds, P. D., Misstear, B. D., and Gill, L. W. (2012). Development of a microbial contamination susceptibility model for private domestic groundwater sources. Wat. Resour. Resear. 48, 4812–12504. doi: 10.1029/2012WR012492
Iliyasu, H., Abdullahi, B. A., and Kano, A. H. (2018). An assessment of the physicochemical quality of Some bottle water sold in Kano Metropolis, Nigeria. Bayer J. Pur. Appl. Sci. 11, 40–44. doi: 10.4314/bajopas.v11i1.6S
Iren, K. N., Kengni, L., Tamen, J., Awah, T. M., Tamungang, N. E. B, et al. (2019). Spring quality assessment and effects on the health of inhabitants of Santa sub-division, north west region. Cameroon. Int. J. Biol. Chem. Sci. 13, 2894–2913. doi: 10.4314/ijbcs.v13i6.37
Ishaku, H. (2011). Hydrochemical evolution of groundwater in Jimeta Yola area, northeastern Nigeria. Glob. J. Geol. Sci. 9, 99–121.
Islam, M. S., Mahmud, Z. H., Islam, M. S., Saha, G. C., Zahid, A., Ali, Z. A. H. M., et al. (2016). Safe distances between groundwater-base water wells and pit latrines at different hydrogeological conditions in the Ganges Atrai floodplains of Bangladesh. J. Health Popul. Nutr., 35–26. doi: 10.1186/s41043-016-0063-z
Joseph, A. O., and David, A. O. (2011). Pollution effects of pit latrines on shallow wells at Isale Igbehin community, Nigeria. J. Geol. Min. Res. 3, 211–218.
Karkarna, M., and Dabo, Z. M. (2021). Assessment of selected heavy metals concentrations in Mairua reservoir Funta, Katsina state, Nigeria for fishing purpose. Bayer. J. Pur. Appl. Sci. 14, 207–213. doi: 10.4314/bajopas.v14i1.24
Keshavarzi, B., and Moore, F. (2017). Groundwater pollution assessment of industrial effluents in Kerman, Iran. Environ. Ear. Sci. 76:262.
Maju-Oyovwikowhe, E. G., and Emofurieta, O. (2021). Groundwater quality determination from hand dug Wells in Ososo town Akoko-Edo, Edo state. Niger. J. of Techn. 40.
Mkadmi, Y., Benabbi, O., Fekhaoui, M., Benakkam, R., Bjijou, W., Elazzouzi, M., et al. (2018). Study of the impact of heavy metals and physico-chemical parameters on the quality of the wells and waters of the Holcim area (oriental region of Morocco). J. Mater. Environ. Sci. 9, 672–679.
Musa, A., Mala, B. M., Bukar, L. K., Mala, B. M., Bukar, L. K., and Wakil, N. A. (2023). Quality assessment of borehole water samples in terms of selected physicochemical parameters in Maiduguri urban areas Borno state, Nigeria. J. Appl. Sci. Environ. Manage. 27, 5–8. doi: 10.4314/jasem.v27i1.1
National Ground Water Association (2009). Annual report on water potential and quality : National Ground Water Association.
Ndoziya, A. I., Hoko, Z., and Gumindoge, W. (2019). Assessment of the impact of pit latrines on groundwater contamination in Hopely settlement, Harane, Zimbabwe. J. Water Sanitat. Hygiene Dev., 1–13. doi: 10.2166/washdev.2019.179
NSDWQ (2015). Nigerian industrial standard, approved by standard Organization of Nigeria (SON). Available at: https://africacheck.org/wp-content/uploads/2018/06/Nigerian-Standard-for-Drinking-Water-Quality-NIS-554-2015.pdf
Nwidu, L. L., Oveh, B., Okoriye, T., and Vaikosen, N. A. (2008). Assessment of the water quality and prevalence of water borne diseases in Amassoma, Niger Delta, Nigeria. Afri. J. Biotechnol. 7, 2993–2997.
Odonkor, S. T., and Addo, K. K. (2018). Prevalence of multidrug-resistant Escherichia coli isolated from drinking water sources. Int. J. Microbiol. 2018, 1–7. doi: 10.1155/2018/7204013
Ohwo, O., and Omidiji, A. O. (2021). Pattern of Waterborn diseases in Yenagoa. J. Appl. Sci. Environ. Mgt. 25, 1015–1023.
Opara, A. U., Nnodim, J., Okorochi, E., and Nwanjo, H. U. (2011). Microbial and physicochemical qualities of water sources in a pig-rearing rural community, Southeastern Nigeria. Int. Sci. Res. J. 3, 25–28. doi: 10.5897/AJEST2015.2042
Oyewalel, A. T., Adesakin, T. A., Oyedeji, O., Aduwo, I. A., and Bakare, M. K. (2018). Impact assessment of poultry discharge on the Physico-chemical and microbiological water quality of Olosuru stream in Ikire, southwestern Nigeria. J. Water Resour. Protect. 2018, 1061–1082. doi: 10.4236/jwarp.2018.1011062
Raji, W. A., Amih, C. E., and Obeta, P. O. (2019). Assessment of physicochemical properties and heavy metals in borehole water used for drinking in Okada town, Edo state, Nigeria. J. Appl. Sci. Environ. Managt. 23, 2205–2209.
Selendy, M., and Janine, M. H. (2011). Water and sanitation-related diseases and the environment challenges, interventions, and preventive measures. Hoboken, N.J: Wiley-Blackwell.
Some, S., Mondal, R., Mitra, D., Jain, D., Verma, D., and Das, S.. (2021). Microbial pollution of water with special reference to coliform bacteria and their nexus with environment. Energy Nexus 1, 1–9.
Texas Groundwater Production Committee, (2000). Joint groundwater monitoring and contamination report. Texas Commission on Environmental Quality Austin TX 78711-3087 TCEQ SFR-56/. Available at: https://www.tceq.texas.gov/publications. (Accessed May 22, 2023).
United Nations General Assembly (2010). Resolution 64/292: The human right to water and sanitation : United Nations General Assembly.
United State Environmental Protection Agency (2001). Design manual: Municipal waste water disinfection. Washington, DC: United State Environmental Protection Agency.
Usman, U. F., and Aliyu, B. (2020). Impact of pit latrine on groundwater quality in some communities of Ngune town, Nguru local government area, Yobe state, Nigeria. East Afr. Schol. Multidiscip. Bull. 3, 218–225. doi: 10.36349/easmb.2020.v03i05.003
Vinger, B., Hlophe, M., and Selvaratnam, T. (2012). Relationship between nitrogenous pollution of borehole waters and distances separating them from pit latrines and fertilized fields. Life Sci. J. 9, 402–407.
Wamyil, J. F., Nkemakonam, O. C., Adewale, O. S., Nabona, J., Ntulume, I., Wamyil, F. B., et al. (2023). Microbiological quality of water samples obtained from water sources in Ishaka, Uganda, Sage. Medicine 11, 1–8. doi: 10.1177/20503121231194239
Water Aid (2009). Fatal neglect - how health systems are failing to comprehensively address child mortality. London, UK. Available at: http://www.wateraid.org/documents/plugin_documents/wateraid:fatal_neglect.pdf
WaterAid, (2011). Off-track, off-target: Why investment in water, sanitation and hygiene is not reaching those who need it most. Available online at: www.wateraid.org (Accessed February 03, 2013).
WEDC, (2012). Latrine slabs: An engineer’s guide, water, engineering and development centre school of civil and building engineering Loughborough University. Leicestershire LE11 3TU UK: WEDC, Loughborough University.
WHO (2010). Guidelines for drinking-water quality: Fourth edition incorporating the first addendum. Geneva: WHO.
WHO and UNICEF (2019). Progress report on household drinking water, sanitation and hygiene 2000–2017: Special focus on inequalities. Geneva: WHO.
World Health Organization, (2008). Guidelines for drinking water quality: incorporating first addendum 4th, Geneva: WHO.
Keywords: groundwater quality, outbreak, bacteriological quality and waterborne diseases, faecal matter, seepage contaminat
Citation: Odewade LO, Imam AA, Adesakin TA and Odewade JO (2025) Assessment of human faecal contamination on groundwater quality and reporting consequent waterborne diseases in Funtua Metropolis, Katsina State, Nigeria. Front. Water. 7:1561777. doi: 10.3389/frwa.2025.1561777
Edited by:
Pamela Monaco, University of Molise, ItalyReviewed by:
Alina Deshpande, Los Alamos National Laboratory (DOE), United StatesChiemeka Ezechinyere, Imperial College London, United Kingdom
Copyright © 2025 Odewade, Imam, Adesakin and Odewade. This is an open-access article distributed under the terms of the Creative Commons Attribution License (CC BY). The use, distribution or reproduction in other forums is permitted, provided the original author(s) and the copyright owner(s) are credited and that the original publication in this journal is cited, in accordance with accepted academic practice. No use, distribution or reproduction is permitted which does not comply with these terms.
*Correspondence: T. A. Adesakin, YXRhaXdvbmVsc29uQGdtYWlsLmNvbQ==