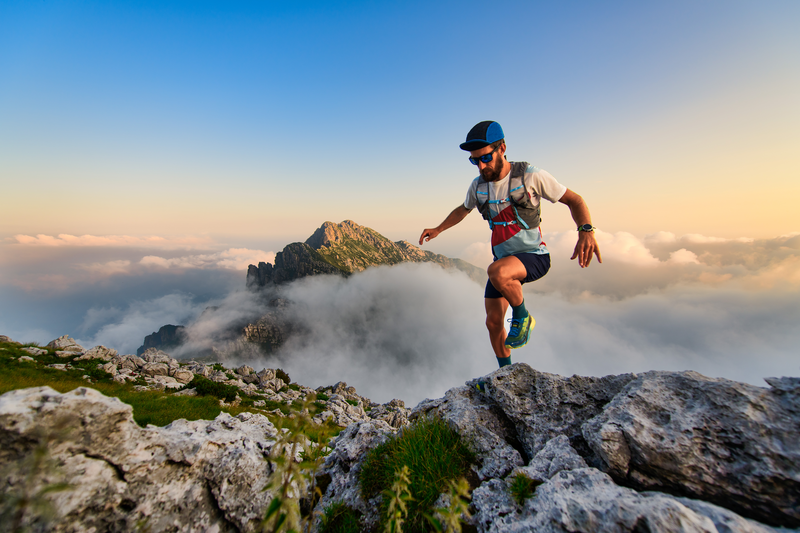
95% of researchers rate our articles as excellent or good
Learn more about the work of our research integrity team to safeguard the quality of each article we publish.
Find out more
ORIGINAL RESEARCH article
Front. Water , 04 February 2025
Sec. Water Resource Management
Volume 7 - 2025 | https://doi.org/10.3389/frwa.2025.1521812
Introduction: Analyzing the hydrological dynamics and assessing the impact of Soil and Water Conservation (SWC) techniques provides crucial insights for developing region-specific conservation strategies and advancing effective watershed management.
Methods: A multi-objective calibration concept was applied to the Soil and Water Assessment Tool (SWAT) model, where simultaneous calibration across the watershed andits sub-watersheds was performed using multiple objective criteria. This study investigates the impact of SWC measures on the hydrological dynamics of the Merguellil watershed, Central Tunisia. The research includes a sensitivity analysis, as well as the calibration and validation of the SWAT model, revealing seven sensitive parameters.
Results and discussion: During calibration (2000-2012), NSE was 0.82 and R2 was 0.9, RSR was 0.19 and PBIAS was 11.62%. In validation (2013–2020), NSE was 0.81 and R2 remained 0.9, RSR was 0.22 and PBIAS was 10.96%, indicating a strong correlation. Results of multi-watershed calibration were analyzed in two representative sub-watersheds (SW 8 and SW 16) and present good agreement between simulated and observed values. Simulating the SWAT model with and without SWC techniques reveals a consistent reduction in surface runoff, notably in central subbasins with values exceeding 15%. The observed decrease is attributed to vegetation cover, indicating the effectiveness of SWC practices. In contrast, subbasins lacking SWC interventions exhibit minimal runoff changes. The study further assesses the impact of SWC techniques on soil erosion, revealing negative percentage differences that indicate a reduction in erosion of over 30% following the implementation of these techniques. The central subbasins, marked by olive trees and strategic conservation, demonstrate substantial decreases, emphasizing successful erosion control efforts. Groundwater recharge analysis shows that SWC practices, along with favorable conditions, significantly enhance percolation and groundwater recharge, highlighting their beneficial impact. Variations in recharge percentages across subbasins reflect the nuanced responses influenced by anthropogenic and natural factors. Erosion hotspots were identified using sediment yield (SY) data. Six sub-watersheds were categorized from moderate to severe sediment severity classes and pinpointed as soil erosion hotspots, requiring immediate intervention. Finally, the study underscores the vital role of SWC techniques in mitigating surface runoff, reducing soil erosion, and enhancing groundwater recharge in the semi-arid Merguellil watershed. The findings emphasize the need for tailored conservation strategies considering geographical variations for effective watershed management and sustainability.
The persistent challenge of water scarcity appears large over the dry and semi-arid regions that border the coast of the Mediterranean in Northern Africa (Barredo et al., 2019). These areas, particularly Tunisia’s semi-arid northeast, find themselves in an existing water resource crisis and the outlook for their water security seems increasingly terrible (Maddocks et al., 2015). In the particular context of Tunisia, the complex relationship between water and soil issues has been a long-standing concern (Jarray et al., 2023b). This intrinsic connection can be attributed to a multitude of environmental factors, each exacerbating the other’s impact. First, the rugged terrain of these regions poses a significant obstacle to the efficient distribution and retention of water (Hermassi et al., 2023). The uneven topography makes it challenging to create effective water management systems, leading to wastage and inefficient use of the limited water available (Cherif et al., 1995). Furthermore, the irregular and unpredictable rainfall patterns in these regions exacerbate the existing water challenges. Droughts and erratic precipitation make it increasingly difficult to rely on rainfall as a dependable water source (Vogel et al., 2021). This forces communities to draw even more heavily on already strained water resources.
According to the dual challenges of water scarcity and soil degradation in these regions, it becomes evident that a complete approach is necessary (Akhtar-Schuster et al., 2017). This approach should encompass not only water resource management but also sustainable land use practices, infrastructure development, and community engagement to mitigate the severe water stress these areas face (Roose, 1994). Soil erosion poses a challenge that is further intensified by the degradation of agricultural land (Gashaw et al., 2019). This deterioration substantially increases the average runoff when compared to undegraded land, thereby intensifying the issue. Recognizing the gravity of these concerns, and according to the Ministry of Agriculture (2017), Tunisian institutions embarked on a path of action by initiating SWC measures since 1990. These proactive steps aim to mitigate surface runoff, enhance groundwater recharge, and limit soil loss to protect both the environment and the agricultural sector (Abera et al., 2020). In general, SWC works are strategically constructed in upland areas to address erosion and water scarcity challenges (Lakshmi et al., 2015). Moreover, they play a central role in facilitating the infiltration of captured surface runoff, effectively reloading the local groundwater reserves (Brempong et al., 2023). Thus, this approach not only helps combat erosion but also contributes significantly to alleviating water scarcity issues in the region (Le Goulven et al., 2009). Indeed, the selection and design of SWC structures should take account the specific land morphology and planned land uses in the given area (Keesstra et al., 2016).
The incorporation of monitoring, remote sensing and modelling techniques in evaluating land degradation and hydrological processes plays a pivotal role in enhancing the successful implementation of conservation methodologies (Taguas et al., 2013). Over the last three decades, hydrological models have experienced widespread adoption on a global level (Sorooshian et al., 2018). To conduct a comprehensive analysis of rainfall-runoff models, a cooperative approach melding remote sensing and Geographic Information Systems (GIS) technologies has been adopted (Aghsaei et al., 2020). This harmonious integration yields a strong tool capable of accurately calculating critical hydrological parameters (Gautam et al., 2022a,b). It is worth noting that the increasing global adoption and enhancement of these models have been significantly forced by the advancements in new technologies (Subhadip and Sneha, 2017).
This synergistic integration of technology and hydrological modeling is instrumental in furthering our understanding and stewardship of water resources on a larger scale (Arturo et al., 2023). Moreover, hydrological models have not only proven to be invaluable instruments but have also undergone continuous refinement, significantly enriching our capacity to comprehend and mitigate the challenges associated with land degradation and water-related concerns (Sorooshian et al., 2018).
Advanced technologies serve a dual purpose: firstly, to identify the fundamental drivers shaping hydrological systems and intricately replicate the complex processes within them, encompassing sediment generation, runoff dynamics, and the critical facet of groundwater recharge (Meshram et al., 2023). Secondly, their principal mission is to enhance the knowledge base of decision-makers, equipping them with a robust understanding of hydrological details to inform and guide decisions related to effective hydrological management within catchment areas (Hermassi et al., 2023; Jarray et al., 2023b, 2024). Within the toolbox of available models, a variety of specialized options exists for simulating long-term hydrological patterns at the watershed level. This toolkit includes renowned models such as Soil and Water Assessment Tool (SWAT) (Arnold et al., 2012), MIKE SHE (Refsgaard, 1996), Hydrological Simulation Program Fortran (Johanson et al., 1980), MOHID Land (Braunschweig et al., 2004), Systeme Hydrologique Européen (Abbott et al., 1986) and Topographic Model (Ambroise et al., 1996), each bringing its unique capabilities to the forefront of hydrological research and management, thus advancing our ability to sustainably steward vital water resources.
The SWAT model is a semi-distributed hydrological model that simulates processes continuously over time at the catchment and watershed scales (Arnold et al., 1998; Gassman et al., 2007). This model was powered by the presence of the SWAT-Calibration and Uncertainty Programs (SWAT-CUP) stand out as crucial tools, which are conducting parameter sensitivity analyses (Kouchi et al., 2017).
The SWAT model has been successfully applied globally to evaluate the effectiveness of SWC practices in mitigating runoff, soil erosion, and enhancing groundwater recharge. Studies in semi-arid regions of China have shown that SWC practices can substantially decrease sediment yield on hillslopes, particularly during periods of intense rainfall (Chen et al., 2020; Jia et al., 2022). Furthermore, SWAT modeling has been utilized to evaluate the spatial and temporal patterns of groundwater recharge in the Thuthapuzha subbasin, revealing the impact of topographic and land-use factors (Prabhakar et al., 2022). Numerous studies conducted across Africa have highlighted the significant impact of SWC practices on hydrological processes. In Ethiopia, research has shown that SWC structures can reduce surface runoff by 28–40% and sediment yield by 43–68% in treated watersheds compared to untreated ones (Berihun et al., 2020). In East Africa, terrace cultivation, a widely adopted practice, has demonstrated remarkable benefits in reducing soil erosion, conserving water, and preserving nutrients at the watershed scale (Gachene et al., 2020). Furthermore, contour ridge practices in West Africa have proven effective in minimizing runoff and erosion while simultaneously enhancing infiltration and soil water storage (Nafi, 2020).
It was frequently used in the semi-arid context of Tunisia. Its applications encompass evaluating the influence of climate change on water resource management (Sellami et al., 2015), quantifying runoff (Jarray et al., 2023a; Bouraoui et al., 2005) and sediment transport (Mosbahi et al., 2012), and assessing the impact of SWC structures on watershed hydrological processes and soil erosion in North regions of Tunisia (Jarray et al., 2023b) and in South regions (Ouessar et al., 2009). In Merguellil watershed, SWAT modeling revealed that contour ridges decreased surface runoff by 32% and increased aquifer recharge by 50% (Abouabdillah et al., 2014).
On the other hand, the model is capable of pinpointing areas highly susceptible to erosion and assessing the efficiency of best management practices (BMPs) in mitigating sediment yields. Structural BMPs proved to be more effective than agricultural ones, with the best outcomes achieved through a combination of practices (Uniyal et al., 2020). Moreover, implementing BMPs can enhance aquifer recharge, baseflow, and percolation, while decreasing surface runoff (Uniyal et al., 2020). In northeast of Tunisia, SWAT model was used to evaluate the global impact of BMPs (Benrhouma et al., 2024). Indeed, the research has proven that BMPs were effective in reducing soil loss. This reduction was 1.5 t/ha/year for mulching and 2 t/ha/year for terracing. Finally, these studies demonstrate the effectiveness of SWAT model in assessing the impact of SWC techniques on surface runoff, soil erosion, and groundwater recharge in various semi-arid regions.
The primary objectives of this research are: to evaluate calibration and validation processes using streamflow, soil erosion and groundwater recharge data under multi-objective calibration methodology, to assess the impact of SWC measures on sediment yield, runoff, and groundwater recharge in the Merguellil watershed, and to identify soil erosion hotspot areas within the studied watershed. This specific watershed, due to its characteristic semi-arid climate and high susceptibility to various phenomena, is considered an ideal case study. The unique characteristics of the Merguellil catchment make it a valuable context in which to study and understand the effectiveness of SWC installations in meeting this important conservation challenge.
The study area encompasses the Merguellil watershed, a significant watershed in semi-arid Tunisia, located west of the Kairouan governorate in central Tunisia (Figure 1). The Merguellil Wadi emanates from the elevated regions of Kesra and Makthar, traversing in a northwestern to southeastern direction towards its natural terminus, the El Kalbia Sebkha, adjacent to the northwestern periphery of Kairouan city (Kingumbi, 2006). The studied watershed has two distinct regions: a mountainous upstream area and a flat downstream plain, connected by the El Houareb dam, built in 1989 (Lazard, 2013). The Merguellil watershed features a mix of hilly terrain, including Jebel Kesra, Barbrou, and Trozza, along with expansive plains like El Alaa in its upper reaches. In contrast, the lower section is a broad alluvial plain primarily used for agriculture (Saadi, 2018; Jerbi, 2018).
The Merguellil watershed experiences a semi-arid climate (Henia, 2003) characterized by substantial year-to-year variations in rainfall, notable temperature disparities, and pronounced summer dry spells (Lacombe et al., 2008; Ben Ammar et al., 2006). Between 1980 and 2020, the region’s average annual rainfall was approximately 300 mm, varying from 265 mm in the plains to 515 mm in the highlands. The average annual temperature was 19.5°C, with daily temperatures typically falling below 12°C in January and exceeding 28°C during July and August. The local weather patterns are predominantly influenced by the convergence of disparate air masses emanating from the temperate Northern Tellian mountains and the arid southern regions (Saadi, 2018). During the winter months, the prevailing winds emanate from the north and northwest (Bouzaiane and Lafforgue, 1986), whereas during the summer months, they predominantly originate from the south and southwest. In the study area, in the year of 2020, the annual soil erosion rate was 16 t/ha/year and the sediment yield was 8.95 t/ha/year (Hermassi et al., 2023).
The main input parameter for the SWAT model is the Digital Elevation Model (DEM). This DEM is essential for defining the watershed and generating Hydrologic Response Units (HRUs). It serves as the foundation for the catchment delineation process in the SWAT hydrological model. Mainly, the DEM is used to determine slope, stream network, and outlet site locations. For the current study, the DEM used had a spatial resolution of 30 × 30 m2 and was obtained from the global USGS and SRTM (Shuttle Radar Topography Mission) format.
Land use and land cover (LULC) information is essential for hydrologic modeling, as it significantly influences runoff and sediment flow within a watershed (Pande et al., 2021). Accurate mapping and thorough investigation of LULC are essential for precise hydrologic modelling. In this research, two LULC maps were developed utilizing Landsat images. These maps represent the conditions during two representative periods: the calibration period (2005) and the validation period (2015). These maps contributed significantly to a comprehensive understanding of the LULC dynamics within the study area. The classification process was carried out using the Support Vector Machine (SVM) method. For the multi-objective analysis, the maps were used as key inputs for the SWAT model, particularly in defining spatial variability of land use and its influence on hydrological processes. The integration of dynamic LULC data allowed for improved calibration by aligning land use practices with observed hydrological responses. For the calibration and validation periods, objective functions like NSE and R2 were evaluated across multiple sub-watersheds, with a particular focus on representing the influence of LULC changes over time. The multi-objective analysis revealed that incorporating temporal LULC dynamics significantly enhanced the model’s ability to simulate hydrological processes.
Soil data exert a significant impact on catchment hydrology and serve as a crucial component in modelling, particularly in the identification of areas most susceptible to erosion phenomena. In this research, diverse classes were delineated using a digitized Tunisian soil map at a scale of 1/50,000. To determine missing variables and soil characteristics in the SWAT database, the SPAW (SOIL–PLANT-ATMOSPHERE-WATER) model was used. It is a daily hydrological simulation model designed for agricultural and hydrological fields. SPAW has been developed through collaboration between the USDA and the Department of Biological Systems Engineering (DBSE) at Washington State University.
The SWAT software facilitates the incorporation of data from multiple weather stations, encompassing various climatic parameters such as precipitation (PCP), minimum and maximum temperature (TMP), wind speed (WND), solar radiation (SLR), and relative humidity (HMD). In this case, the daily data sources employed encompassed (i) information collected from 20 rain gauge stations from Directorate General of Water Resources, Tunisia and (ii) data obtained from the National Centers for Environmental Prediction (NCEP). Additionally, daily flow data were observed in the El Houareb dam for the period from 2000 to 2020. This variable will be utilized for the calibration and validation processes.
In the past two decades, most agricultural areas within the Merguellil watershed have received SWC interventions. These techniques are designed to capture and store runoff water, enhance infiltration and reduce soil erosion (Cherif et al., 1995). The goal has been to promote landscape restoration and support sustainable watershed development. The SWC works within the watershed consist of five small dams situated along the hydrographic network of Merguellil, which are simulated as reservoirs. These dams serve various purposes, including water storage and management. Furthermore, there are 55 hill lakes distributed across the watershed contributing to its hydrological complexity and potential for water retention. Additionally, some terraces cover a substantial area of the Merguellil watershed (29% in 2020) (Hermassi et al., 2023). These terraces are constructed parallel to contour lines to intercept runoff water and preventing its concentration. In this case study, the areas with SWC measures were identified using satellite imagery and field-based surveys. Following the same methodology used for LULC mapping, we created SWC maps for 2005 and 2015. These maps were then used to assess the impact of SWC evolution on watershed behavior.
Using SWAT model, the first step of simulation process was the division of the study area into sub-basins (Neitsch et al., 2009). Next step was the elaboration of HRUs, which were established using combinations of slope, LULC and soil type datasets, with a threshold value of 10% applied to each dataset for consistency (Arnold et al., 2012). Moreover, all the necessary climatic variables were precisely prepared in a appropriate format and supplied as inputs to the model (Winchell et al., 2007). For this case, a monthly simulation has taken place, from 2000 to 2020, with an initial one-year warm-up period incorporated into the analysis.
The Sequential Uncertainty Fitting-2 (SUFI-2) algorithm was employed for model calibration and validation (Abbaspour et al., 2007). Sensitivity analysis and calibration, which are closely related, are crucial steps in effectively configuring the SWAT model (Abbaspour, 2015; Kouchi et al., 2017). SWAT-CUP’s primary objective is to identify the most suitable combination of pertinent parameter values following the initial sensitivity analysis. This selection aims to accomplish a strong alignment between the observed data and the simulated values throughout both processes. In this study, model calibration was conducted for the period from 2000 to 2012, while validation covered 2013 to 2020. Sensitivity analysis, a crucial phase in identifying the SWAT input parameters that most affect model output variability (Baker and Miller, 2013), was performed using the SUFI-2 algorithm. This analysis evaluated each parameter using two key indicators: the t-stat and the p-value (Abbaspour, 2015). The parameter with the highest absolute t-stat and the smallest p-value was considered the most sensitive (Abbaspour et al., 2007). In this specific context, a total of 7 parameters were selected for sensitivity analysis. These parameters were explored by taking into account the physical properties defined by the guidelines provided by Arnold et al. (2012). After 1,000 iterations, parameter sensitivity analysis was completed. In fact, 1,000 iterations in SWAT model calibration provide a balance between thorough parameter space exploration, reliable model performance, and reasonable computational effort, making it a widely accepted default in hydrological modeling. The sensitivity analysis was carried out on a monthly basis, spanning the period from 2000 to 2020. The initial year was a warm-up period to initialize unknown variables.
The effectiveness of a hydrologic model is gauged by how well it aligns its simulated outputs with observed data. In fact, according to Abbaspour (2015), the assessment of hydrologic models is significantly contingent upon two crucial steps: sensitivity analysis and parameter calibration.
In this study, the SWAT Calibration and Uncertainty Procedures (SWAT-CUP) software was employed to facilitate automatic calibration process (Abbaspour et al., 2007). This process was supported by the SUFI-2 algorithm (Abbaspour, 2012), a widely recognized tool for calibrating watershed hydrology models (Yang et al., 2008). SUFI-2 is adept at accounting for numerous sources of uncertainty, encompassing parameter uncertainty, conceptual model uncertainty, and input data uncertainty (Gupta et al., 2009). Two key performance indicators, recommended by SUFI-2, were used to evaluate the model’s performance during calibration and validation. These indicators include Nash–Sutcliffe Efficiency (NSE), Coefficient of Determination (R2), the ratio of the root mean squared error to the standard deviation of measured data (RSR) and percent bias (PBIAS).
These metrics collectively assess the model’s ability to replicate observed hydrologic behavior, indicating its accuracy and reliability.
To develop an accurate watershed model, initiating a calibration process is essential. Given that model parameters represent various natural processes and that many parameters collectively affect watershed outcomes, conducting a sensitivity analysis before calibration is crucial. The sensitivity analysis determines which parameters most affect key variables like runoff and refines the calibration process by concentrating on the most influential parameters while excluding less sensitive ones. A global sensitivity analysis was conducted utilizing the SWAT-CUP model. According to the statistical ranking of the model outputs, six parameters were identified as sensitive. The assessment of parameter sensitivity primarily relied on two statistical criteria: the p-value and the t-stat. Among these seven parameters, six emerged as the most sensitive, meeting the criteria of possessing absolute t-stat values equal to or surpassing 2, coupled with p-values less than 0.05. The key parameters identified included the curve number (CN2), SOL_AWC and the ESCO factor. Parameters delineated in Table 1 play a key role in influencing the attitude of runoff within the watershed.
Calibration and validation processes were employed to assess the impact of changes in the SWC area on runoff, soil erosion and groundwater recharge. In this study, a concept of multi-objective calibration in SWAT was applied and revolved around the simultaneous calibration of SWAT model across the watershed and its sub-watersheds using multiple objectives criteria. To assure a multi-objective calibration study, we use multiple criteria such as streamflow, sediment yield and groundwater recharge. Moreover, an objective function which are the performance indicators (NSE and R2) are often used. On the other hand, a multi-watershed calibration has taken place where the SWAT model is calibrated for the entire watershed and some sub-watersheds (SW) simultaneously. In fact, this approach search to ensure that the model performs well across various metrics, not just one.
The calibration was carried out for the period spanning from 2000 to 2012, while the validation phase encompassed data from 2013 to 2020. The study assessed the reliability of parameters that had been calibrated and validated using two different approaches. To evaluate streamflow consistency, observed and simulated monthly flows were compared graphically (Moriasi et al., 2015) during calibration and validation (Figure 2). The findings demonstrated a strong agreement between the simulated and observed values during calibration and validation phases in the outlet of the studied watershed. In terms of evaluating the model’s performance, various statistical metrics (Moriasi et al., 2015) were employed for both calibration and validation stages, using flow data. These indicators included NSE and R2, RSR and PBIAS (Table 2). During the calibration phase, the performance indicators produced values of 0.82 for NSE 0.9 for R2, 0.19 for RSR and 11.62% for PBIAS. As for the validation process, there was a strong correlation, with NSE reaching 0.81 and R2 at 0.9. Moreover, for the validation process RSR was equal to 0.22 and PBIAS equal to 10.96%. Consequently, the statistical indices showed a very good agreement between the observed and simulated streamflow for the calibration and validation processes.
Figure 2. Graphical comparison of observed and simulated monthly streamflow during the calibration and validation processes.
Table 2. Statistics for calibration and validation processes with flow data in the Merguellil watershed.
In the next step, results of multi-watershed calibration were analyzed in two representative sub-watersheds (SW 8 and SW 16). Graphical comparison presents good agreement between simulated and observed values in SW 8 with a NSE equal to 0.75 and R2 equal to 0.85, and in SW 16 with a NSE of 0.53 and R2 of 0.57, for the period 2002–2008 (Figure 3). The strong R2 value in SW 8 (0.85) confirms a high correlation between observed and simulated streamflow, indicating that the model effectively captures the variability and trends in this sub-watershed. The combination of a high NSE (0.75) and R2 shows that both the magnitude and timing of peak flows, as well as baseflow conditions, are well simulated. This can likely be attributed to homogeneous watershed characteristics and fewer anthropogenic impacts in SW 8. In contrast, SW 16 exhibits moderate model performance, with a lower NSE (0.53) and R2 (0.57) (Figure 3). These values suggest that while the model captures some aspects of streamflow variability, it struggles to accurately represent the dynamics of peak flows and low-flow periods. The discrepancy may stem from complex watershed characteristics in SW 16, such as mixed land use, varying soil properties and concentration of SWC techniques. Despite the weaker performance in SW 16, the calibration across both sub-watersheds demonstrates the model’s ability to handle diverse conditions within the multi-watershed framework.
For the studied area, the outlet was the Houareb reservoir, where only one bathymetric survey was conducted in 2011, providing a sediment yield value of 9.39 t/ha/year. Subsequently, a multi-watershed analysis was performed for sediment yield across several representative sub-watersheds (SW) in the region. Due to limited data availability, this investigation was restricted to four small dams located in SW 3, SW 7, SW 8, and SW 24, for the year 2011. The simulated and observed sediment yield values for these sub-watersheds are as follows (Table 3).
A comparison of simulated and observed sediment yield values reveals acceptable model performance. In fact, the model accurately simulates sediment yield in SW 7 and SW 8 however, it shows slight overestimations in SW 3 and SW 24. The observed value of 9.39 t/ha/year from the Houareb reservoir bathymetry falls within the range of simulated values, confirming that the model captures the overall trend of sediment yield, although local adjustments may be needed for more accurate predictions.
The modeling of groundwater recharge is of paramount importance in understanding and managing water resources effectively. Indeed, SWAT model is a powerful tool that provides quantitative insights into the dynamics of water movement within the watershed.
This study reveals a significant correlation between the simulated values generated by the SWAT model and the observed groundwater recharge values. The strength of this correlation is exemplified by a coefficient of determination reaching (0.8) (Figure 4).
The surface runoff distribution in the Merguellil watershed exhibits a clear lack of uniformity, indicating a non-uniform distribution across its subbasins. To assess the effect of these techniques, the SWAT model was run with and without SWC managements. Figure 5 displays the percentage of difference in runoff values between the treated watershed and the untreated condition.
Figure 3 provides a comprehensive overview of the runoff percentage differences observed in a watershed that has undergone SWC techniques compared to an untreated condition, yielding valuable insights into the impact of these practices. Negative percentage values in all subbasins signify a consistent reduction in surface runoff within the treated watershed.
The variations in the magnitude of reduction across subbasins, ranging from 0% to (−23%), reveal a diverse response to SWC implementation. Significant reductions in values, particularly in the form of larger negative values, were found to be concentrated within the central subbasins of the watershed, which are number 4, 5, 11, 13, and 15. These reductions surpassed 15% when compared to the untreated condition, indicating a considerable impact on the environmental parameters under consideration (Figure 5). For instance, subbasin 3 stands out with a significant difference, showing a 15% decrease in runoff due to the implementation of SWC techniques.
The distinctive feature of these central areas lies in their new cultivation approach, marked by the implementation of olive trees, orchards and wheat crops. In fact, the vegetation cover provided by these crops serves as a natural barrier, reducing the speed of water flow across the soil surface. This helps to minimize the risk of soil erosion and surface water runoff. Furthermore, it’s noteworthy that these zones have undergone thorough treatment employing SWC techniques. This comprehensive approach to SWC signifies a proactive effort to manage soil and water resources effectively. Indeed, the implementation of SWC techniques is crucial for sustaining the health and productivity of the cultivated areas (Figure 5). Overall, the combination of agricultural areas and strategic conservation measures highlights a holistic approach to environmental protection.
On the other hand, the subbasins, namely numbers 1, 2, 8, and 27, exhibit a relatively lower change in comparison, with the recorded change being around 2%. Upon investigating the LULC maps and SWC maps, it becomes apparent that these areas are characterized by low vegetation cover, primarily consisting of rangelands and cereals. Additionally, there is an absence of any form of SWC techniques in these regions.
The combination of these factors provides a realistic explanation for the minimal runoff changes detected in these areas. The low vegetation cover suggests less effective protection against runoff and soil erosion. Without the presence of SWC techniques, there are limited mechanisms in place to mitigate the impact of water runoff. Moreover, the low percentage of runoff changes in these subbasins may also be attributed to a decrease in rainfall values over the years. The combination of low vegetation cover, lack of SWC techniques and reduced rainfall collectively contributes to the observed minimal change in these specific subbasins. Finally, the overall negative values underscore the general mitigating effect of SWC techniques on surface runoff.
The Merguellil watershed demonstrates negative percentage differences in soil erosion, and this favorable trend can be attributed to the effective implementation of SWC practices. These practices have contributed to mitigating soil erosion, leading to a notable improvement in the overall soil conservation status within the watershed.
The observed negative percentage differences reveal varying magnitudes of reduction, ranging from 0% to (−56%). This variability underscores the diverse responses of different subbasins within the Merguellil watershed to the implemented SWC measures. Each subbasin responds differently to conservation practices, shaped by factors like topography, land use, and specific SWC techniques (Figure 6).
The substantial reduction of up to (−56%) in soil erosion in some subbasins highlights the highly effective impact of SWC practices, particularly in areas with vulnerable soil conditions or high erosion risks. Conversely, subbasins showing a 0% reduction may indicate that existing SWC measures are effectively maintaining current soil erosion levels, reflecting a stable conservation state (Figure 6). Overall, the reduction in soil erosion rates, indicated by the negative percentage differences, demonstrates the effectiveness of SWC techniques in promoting sustainable land use practices and safeguarding the Merguellil watershed.
The presence of larger negative values, particularly in subbasins 4, 5, 11, 13, 14, and 15, indicates substantial decreases in soil erosion within these areas. These negative values reflect significant improvements in soil conservation, underscoring the effectiveness of the measures implemented to combat erosion (Figure 6).
The considerable reduction in soil erosion can be attributed to factors such as robust SWC practices, changes in land use patterns, and other conservation initiatives. These combined efforts have contributed to a more resilient and sustainable environment in the mentioned subbasins. This observation is key to understanding the positive impact of conservation measures on soil health and erosion control.
It implies that the implemented strategies in central subbasins have been successful in minimizing the adverse effects of erosion, promoting soil stability, and fostering a healthier ecological balance. Moreover, the relatively minor change in soil erosion is particularly evident in the northwest areas, encompassing subbasins number 1, 2, and 8, as well as in the southern zone represented by subbasin number 27 (Figure 6). Multiple factors contribute to this scenario, including the patterns of LULC, topographical characteristics, and the presence of SWC techniques in these subbasins. Notably, all these areas were classified as untreated zones, indicating the absence of specific interventions.
This outcome emphasizes the crucial role of implemented measures in these specific areas. The limited variation in soil erosion values suggests that SWC measures have been instrumental in maintaining soil stability and effectively controlling erosion.
To enhance the efficacy of future conservation strategies, it is imperative to elucidate the role of land use land cover, topography, and the application of SWC techniques in untreated zones on soil erosion processes. This recognition underscores the importance of continuous efforts to implement effective soil conservation measures to the specific conditions of each geographical region, ensuring sustained environmental health and resilience.
The impact of SWC techniques on groundwater recharge is a critical aspect of watershed management and hydrological sustainability. SWC practices has a central role in influencing the quantity and quality of water that infiltrates the soil and reloads groundwater aquifers. By minimizing soil erosion and controlling runoff, SWC techniques contribute to increased water absorption and percolation into aquifers. The effectiveness of SWC techniques in groundwater recharge can vary based on factors including topography, soil type, climate variables and the implemented SWC techniques.
The modelling of groundwater recharge is of paramount importance in understanding and managing water resources effectively. Indeed, the SWAT model is a powerful tool that provides quantitative insights into the dynamics of water movement within the watershed.
In the Merguellil watershed, the range of groundwater recharge displays noticeable variability, extending from below 10 mm to surpassing 50 mm. The variation observed is likely affected by a combination of meteorological, pedological and anthropogenic factors (Land use practices), as well as the differential effectiveness of SWC techniques. The interplay of these elements contributes to the observed fluctuations in groundwater recharge across the studied area.
Figure 7 explains the percentage of differences in groundwater recharge across various subbasins, revealing distinctive responses influenced by both anthropogenic interventions and natural conditions. The observed variations underscore the importance of water management strategies, considering the unique characteristics of each subbasin. In fact, the differential responses of subbasins to environmental and anthropogenic pressures are influenced by factors such as land use land cover change, altered precipitation regimes, and the implementation of various SWC strategies. Ranging from less than 5% to more than 20%, the differences in groundwater recharge percentages across various subbasins exhibit a diverse pattern. Notably, several subbasins, including subbasins 4, 5, 11, 13 and 15, exhibit an important percentage of groundwater recharge difference (Figure 7). The enhanced infiltration rates into shallow aquifers in central zones can be ascribed to the adoption of innovative SWC practices. Thus, the present study highlights the important role of these techniques in conserving and recharging shallow aquifer in a semi-arid watershed.
Finally, the detection of groundwater recharge in subbasins with established SWC practices highlights the efficacy of these techniques in enhancing water retention and infiltration capacities. This aligns with the main objective of mitigating surface runoff and increasing soil moisture, contributing to sustainable water resource management.
To pinpoint erosion hotspots, we used sediment yield (SY) data simulated by the SWAT model. This data spatially mapped sediment concentration, highlighting areas with the highest erosion rates. To assess the severity of erosion, the annual sediment yield was computed and categorized into different levels, from low to very severe. In fact, the majority of sediment yield originates from cropland areas, with soil erosion severity categorized as follows: (0–5) low, (5–10) moderate, (10–15) high, (15–20) very high, and > 20 t/ha/year as extremely severe. However, the distribution of severity varies across the different sub-watersheds. Through spatial analysis and categorization of watershed areas, we delineated erosion hotspots. These regions, characterized by high erosion susceptibility, require immediate attention for the application of erosion control strategies to mitigate sediment loss and maintain landscape stability.
The result shows that the average annual sediment yield of the study area ranges from <1 to >20 t/ha/year (Figure 8), showing the spatial variations of sediment yield. A comparative analysis of runoff values across sub-basins reveals substantial differences in runoff generation. On the other hand, it is important to notice that the spatial patterns of runoff, soil erosion, and groundwater recharge are significantly influenced by the spatial distribution of soil and water conservation management practices.
Among the 27 sub-watersheds, SW 1, SW 6, SW 7, SW 8, SW 23 and SW 25 presents a very high and extremely severe classes of sediment yield which is >15 t/ha/year (Figure 8). Finally, all sub-watersheds which presented a category from moderate to severe sediment severity classes, are identified as soil erosion hotspot areas. Therefore, the integration of SWC measures becomes crucial in ensuring sustainable water infiltration and groundwater recharge, even in areas naturally predisposed to favorable conditions. Finally, this approach does not only reduce overall sediment yield, but also preserves soil fertility and prevents further land degradation in the watershed. Within the watershed.
Global changes, encompassing changes in climate patterns and land use practices, alongside the adoption of SWC techniques, have considerable influence over water resources. These factors can lead to notable effects on surface runoff, soil erosion, and groundwater recharge dynamics. Indeed, investigating the interplay between biogeophysical processes and anthropogenic disturbances emphasizes the critical need for adopting sustainable land use practices and climate change adaptation measures.
Innovative technologies, when integrated with effective SWC strategies, can contribute to mitigating the negative consequences of climate change on ecological and hydrological systems. By enhancing ecosystem resilience and promoting sustainable development, they provide practical solutions to mitigate the impacts of environmental degradation and climate change.
This study underscores the positive influence of SWC techniques in qualifying surface runoff, reducing soil erosion, and enhancing groundwater recharge in the semi-arid Merguellil watershed. It emphasizes the importance of conservation strategies considering geographical variations for effective watershed management and sustainability.
The high values of NSE and R2, RSR and PBIAS obtained in this study indicate that the SWAT model accurately simulates hydrological processes. This research demonstrates a significant alignment between observed and simulated flows at a monthly timescale, showcasing the model’s proficiency. Moreover, these results are in agreement with the findings of similar studies conducted worldwide (Jarray et al., 2023b; Čerkasova et al., 2019; Cecílio et al., 2019).
An analysis of the runoff and soil erosion percentage differences observed in the watershed, both with and without SWC techniques, provides a comprehensive understanding of the impact of these practices. By comparing the treated and untreated conditions, valuable insights are gained into the impact of SWC techniques in mitigating runoff and soil erosion. This comparison offers a nuanced overview of the benefits brought about by implementing SWC measures, highlighting their significance in sustainable watershed management.
Globally, anthropogenic impacts, especially through the implementation of SWC measures, play a significant role in reducing runoff. These results have been reinforced by numerous research studies conducted under various climate conditions. The consistent findings across diverse environmental settings further underscore the effectiveness of SWC techniques in reducing runoff. The research conducted by Jarray et al. (2023a) reveals a notable linear relationship between SWC measures and runoff in the case of the Wadi Rmel watershed, localized in Northeast of Tunisia. Indeed, SWC techniques, along with topography, vegetation, and precipitation, reduced surface runoff by 44% from 2015 to 2020. This underscores the effectiveness of employing comprehensive strategies to manage water resources and mitigate runoff in a semi-arid region. Moreover, these findings are consistent with the results of Li et al. (2007), who demonstrated that soil conservation measures were responsible for an 87% reduction in runoff in China from 1972 to 1997. Consistent with the findings of Zhang et al. (2017), terracing has been shown to reduce runoff coefficients.
These results highlight the substantial contribution of soil conservation efforts in mitigating runoff and underscore their importance in sustainable water resource management. Furthermore, SWC measures demonstrate their efficacy in reducing soil erosion worldwide and across diverse climate conditions. This widespread success underscores the robustness and adaptability of SWC techniques in mitigating soil erosion, regardless of geographical location or climatic variability. This alignment between changes in landscape condition and hydrologic processes was pronounced in North Africa such as the results obtained by Jarray et al. (2023b) which proved the importance of SWC in reducing soil erosion in the Wadi Rmel watershed, in North Africa. In addition, Nafi (2020) has shown that contour ridge practices are effective in mitigating runoff and erosion in West Africa. Gachene et al. (2020) have shown that terrace cultivation is an effective practice in East Africa for mitigating soil erosion, conserving water, and preserving nutrients at the watershed scale. On the other hand, Chen et al. (2020) and Jia et al. (2022) have shown that SWC practices can significantly reduce sediment yield from hillslopes in semi-arid China, even during extreme rainfall events. This emphasizes the efficacy of SWC practices in controlling erosion and maintaining soil health, even under adverse environmental conditions.
Furthermore, this research demonstrates that significant groundwater recharge was observed in subbasins where SWC practices were implemented, highlighting the potential beneficial effects of these techniques on water retention and infiltration. Specifically, an increase in the volume of water infiltrating was observed in central zones characterized by the adoption of new SWC techniques for soil and water conservation. Indeed, Hermassi et al. (2023) demonstrate a notable expansion in treated areas from 1980 to 2020. Specifically, the treated surface area expanded from 2% of the total area in 1980 to 29% in 2020. Moreover, the cited study shows that SWC interventions primarily targeted croplands and rangelands (central zones), recognizing these areas as particularly vulnerable to erosion and anthropogenic pressures. On the other hand, there has been an approximate increase of 27% in treated areas for croplands and 30% for rangelands in the last few decades. This augmentation is concurrently and synchronously aligned with the evolution of groundwater recharge (Hermassi et al., 2023). Similar results were observed in West Africa, where Nafi (2020) demonstrated that contour ridge practices play an effective role in enhancing infiltration and soil water storage.
The SWAT model was performed to simulate sediment yield (SY) data and identify erosion hotspots. Sediment concentrations were spatially mapped, highlighting areas with the highest erosion rates. Annual sediment yield was then categorized into varying levels of severity, ranging from low to very severe, to assess erosion intensity. Among the 27 sub-watersheds, SW 1, SW 6, SW 7, SW 8, SW 23, and SW 25 exhibit very high to extremely severe sediment yield levels, exceeding 15 t/ha/year. Sub-watersheds classified with moderate to severe sediment severity are identified as soil erosion hotspots.
This underscores the necessity of integrating SWC measures to conserve natural resources. These findings were aligned with various studies which underscores the necessity of identifying hotspots areas which urgent intervention. In fact, achieving significant reductions in soil erosion hinges on accurately identifying erosion hotspots within the watershed. This process is most effectively accomplished through the integration of hydrological modeling and Geographic Information System (GIS) tools. Hydrological models provide a quantitative framework for simulating sediment dynamics and understanding watershed processes, while GIS tools enable the spatial mapping and analysis of sediment yield, land use patterns, and topographical features. This combined approach facilitates a detailed assessment of erosion-prone areas, allowing for targeted intervention strategies. The critical role of this methodology has been emphasized by Gashaw et al. (2020), Merriman et al. (2019), and López-Ballesteros et al. (2019), who demonstrated its utility in delineating erosion hotspots across diverse geographical settings. Their findings underscore that integrating these tools not only enhances the precision of erosion assessments but also aids in developing region-specific conservation measures.
This study demonstrates the utility of the SWAT model in assessing the impact of SWC practices on hydrological processes in arid environments.
The use of the SWAT model, while highly effective for simulating hydrological processes and assessing watershed management practices, comes with certain limitations. SWAT relies heavily on the availability and quality of input data. Incomplete or inaccurate data can introduce uncertainties into model outputs. In this study, the model’s performance in SW 16 is moderate, with lower NSE (0.53) and R2 (0.57). These values suggest that while the model can represent some aspects of streamflow variability, it has limitations in accurately simulating peak flow events and low-flow periods. In fact, this is explained by the model’s structure which is built on several assumptions and simplifications, such as lumped parameterization at the sub-watershed level, which may overlook localized variations in hydrological and erosion processes.
Finally, the SWAT hydrological model significantly enhances our understanding of water resource management dynamics in semi-arid environments by providing a detailed representation of the complex interactions between land use, soil, climate, and hydrological processes. Its ability to simulate the impacts of soil and water conservation (SWC) practices on runoff, erosion, and groundwater recharge makes it an invaluable tool for decision-makers and researchers. By integrating physical and empirical principles, the model helps identify critical areas requiring intervention, such as erosion hotspots and regions with high runoff or limited infiltration. Furthermore, SWAT’s adaptability to various scenarios, including climate change and land-use alterations, enables the assessment of long-term sustainability and resilience of water resources in semi-arid regions. This capability is particularly crucial in these vulnerable areas, where water scarcity and environmental degradation pose significant challenges.
Hydrological models serve as essential tools for simulating and understanding the complex interactions within water systems. Examining the hydrological dynamics and assessing the impact of SWC techniques provides essential insights for crafting region-specific conservation strategies and advancing sustainable watershed management practices. This study explores the impact of SWC measures on the hydrological dynamics of the Merguellil watershed, employing a comprehensive approach including sensitivity analysis, calibration and validation processes of the hydrological SWAT model. Results present six sensitive parameters, with strong performance indicators during both calibration with NSE of 0.82, R2 of 0.9, RSR of 0.19 and PBIAS of 11.62% and validation with NSE of 0.81, R2 of 0.9, RSR of 0.22 and PBIAS of 10.96%.
Furthermore, the investigation into runoff distribution reveals non-uniformity across subbasins, shaped by topography and SWC techniques. Running the SWAT model with and without SWC measures demonstrates a consistent decrease in surface runoff, particularly notable in central subbasins with reductions exceeding 15%. This decline is attributed to enhanced vegetation cover, indicating the efficacy of SWC practices. Conversely, subbasins lacking SWC interventions show minimal runoff alterations. Additionally, the study evaluates the impact of SWC techniques on soil erosion, showcasing negative percentage differences that signal a reduction in erosion. Central subbasins, characterized by olive trees and strategic conservation efforts, demonstrate substantial decreases, highlighting successful erosion control measures. Moreover, groundwater recharge analysis shows a significant correlation between simulated and observed values, with percolation conditions supporting SWC-treated areas. SWC practices, with specific conditions, strengthen percolation and groundwater recharge, underscoring their positive impact. Variations in recharge percentages across subbasins underscore nuanced responses influenced by anthropogenic and natural factors. Erosion hotspots areas were identified using the SWAT model. A spatial analysis was taken place using GIS tools and categorize SY in all studied sub-watersheds. In fact, SW 1, SW 6, SW 7, SW 8, SW 23 and SW 25 presents a very high and extremely severe classes of sediment yield which is >15 t/ha/year.
In conclusion, the study underscores the indispensable role of SWC techniques in mitigating surface runoff, reducing soil erosion, and enhancing groundwater recharge in the semi-arid Merguellil watershed. Finally, these findings emphasize the necessity for conservation strategies that account for geographical variations, facilitating effective watershed management and long-term sustainability. Thus, a thorough understanding of these concepts is imperative for informed decision-making and effective regional planning.
The datasets presented in this article are not readily available because the dataset include proprietary data/information collected under specific agreements with local authorities, limiting its use to authorized research purposes only. Requests to access the datasets should be directed to dGFvdWZpay5oZXJtYXNzaUBpbmdyZWYudWNhci50bg==.
TH: Conceptualization, Data curation, Formal analysis, Funding acquisition, Investigation, Methodology, Project administration, Resources, Software, Supervision, Validation, Visualization, Writing – original draft, Writing – review & editing. FJ: Conceptualization, Data curation, Formal analysis, Investigation, Methodology, Resources, Software, Visualization, Writing – original draft, Writing – review & editing. WT: Conceptualization, Investigation, Methodology, Project administration, Resources, Software, Validation, Writing – original draft. IA: Formal analysis, Investigation, Methodology, Software, Validation, Visualization, Writing – original draft. MM: Data curation, Investigation, Methodology, Resources, Software, Supervision, Validation, Writing – review & editing.
The author(s) declare that financial support was received for the research, authorship, and/or publication of this article. The authors were thankful to the VENUS project for funding this research. This project was part of the PRIMA program supported by the European Union. Thanks were also due to the National Research Institute for Rural Engineering, Water and Forestry (INRGREF) for the valuable support of this research.
The authors declare that the research was conducted in the absence of any commercial or financial relationships that could be construed as a potential conflict of interest.
The author(s) declare that no Gen AI was used in the creation of this manuscript.
All claims expressed in this article are solely those of the authors and do not necessarily represent those of their affiliated organizations, or those of the publisher, the editors and the reviewers. Any product that may be evaluated in this article, or claim that may be made by its manufacturer, is not guaranteed or endorsed by the publisher.
Abbaspour, K. C. (2012). SWAT-CUP-2012. SWAT Calibration and Uncertainty Program—A User Manual, Swiss Federal Institute of Aquatic Science and Technology: Dübendorf, Switzerland
Abbaspour, K. (2015). SWAT Calibration and Uncertainty Program (SWAT-CUP), User Manual, Eawag, Swiss Federal Institute of Aquatic Science and Technology: Dübendorf, Switzerland.
Abbaspour, K. C., Yang, J., Maximov, I., Siber, R., Bogner, K., Mieleitner, J., et al. (2007). Modelling hydrology and water quality in the pre-alpine/alpine Thur watershed using SWAT. J. Hydrol. 333, 413–430. doi: 10.1016/j.jhydrol.2006.09.014
Abbott, M., Bathurst, J., Cunge, J., Connell, P., and Rasmussen, J. (1986). An introduction to the European Hydrological System—Système Hydrologique Européen, SHE. J. Hydrol. 87, 45–59. doi: 10.1016/0022-1694(86)90114-9
Abera, W., Tamene, L., Tibebe, D., Adimassu, Z., Kassa, H., Hailu, H., et al. (2020). Characterizing and evaluating the impacts of national land restoration initiatives on ecosystem services in Ethiopia. Land Degrad. Dev. 31, 37–52. doi: 10.1002/ldr.3424
Abouabdillah, A., White, M. J., Arnold, G., De Girolamo, A. M., Oueslati, O., Maataoui, A., et al. (2014). Evaluation of soil and water conservation measures in a semi-arid river basin in Tunisia using SWAT. Soil Use Manag. 49:2014. doi: 10.1111/sum.12146
Aghsaei, H., Mobarghaee, D. N., Moridi, A., Asadolahi, Z., Delavar, M., Fohrer, N., et al. (2020). Effects of dynamic land use/land cover change on water resources and sediment yield in the Anzali wetland catchment, Gilan, Iran. Sci. Total Environ. 712:136449. doi: 10.1016/j.scitotenv.2019.136449
Akhtar-Schuster, M., Stringer, L. C., Erlewein, A., Metternicht, G., Minelli, S., Safriel, U., et al. (2017). Unpacking the Concept of Land Degradation Neutrality and Addressing Its Operation through the Rio Conventions. J. Environ. Manag. 195, 4–15. doi: 10.1016/j.jenvman.2016.09.044
Ambroise, B., Beven, K., and Freer, J. (1996). Toward a Generalization of the TOPMODEL Concepts: Topographic Indices of Hydrological Similarity. Water Resour. Res. 32, 2135–2145. doi: 10.1029/95WR03716
Arnold, J. G., Moriasi, D. N., Gassman, P. W., Abbaspour, K. C., White, M. J., Srinivasan, R., et al. (2012). SWAT: Model use, calibration, and validation. Am. Soc. Agric. Biol. Eng. 2012, 1491–1508. doi: 10.13031/2013.42256
Arnold, J. G., Srinivasan, R., Muttiah, R. S., and Williams, J. R. (1998). Large area hydrologic modeling and assessment part I: Model development. J. Am. Water Resour. Assoc. 34, 73–89. doi: 10.1111/j.1752-1688.1998.tb05961.x
Arturo, A. K., Kendra, G., Nalini, R., Eladio, K., and Jeffrey, T. (2023). Hydrological models for climate-based assessments at the watershed scale: A critical review of existing hydrologic and water quality models. Sci. Total Environ. 867:161209. doi: 10.1016/j.scitotenv.2022.161209
Baker, T. J., and Miller, S. N. (2013). Using the Soil and Water Assessment Tool (SWAT) to assess land use impact on water resources in an East African watershed. J. Hydrol. 486, 100–111. doi: 10.1016/j.jhydrol.2013.01.041
Barredo, J. I., Mauri, A., Caudullo, G., and Dosio, A. (2019). “Assessing shifts of Mediterranean and arid climates under RCP4.5 and RCP8.5 climate projections in Europe” in Meteorology and climatology of the Mediterranean and black seas (Cham, Switzerland: Birkhäuser), 235–251.
Ben Ammar, S., Zouari, K., Leduc, C., and M’barek, J. (2006). Isotopic characterization of the dam-aquifer water transfer in the Merguellil catchment (Kairouan Plain, central Tunisia). Hydrol. Sci. J. 51, 272–284. doi: 10.1623/hysj.51.2.272
Benrhouma, A., Jarray, F., Bouajila, K., Dridi, S., and Hermassi, T. (2024). Combined impacts of climate and land use scenarios on sediment loads in small agricultural watershed in Tunisia. GSC Adv. Res. Rev. 21:351361. doi: 10.30574/gscarr.2024.21.1.0390
Berihun, M. L., Tsunekawa, A., Haregeweyn, N., Taddele Dile, Y., Tsubo, M., Almaw Fenta, A., et al. (2020). Evaluating runoff and sediment responses to soil and water conservation practices by employing alternative modeling approaches. Sci. Total Environ. 747:141118. doi: 10.1016/j.scitotenv.2020.141118
Bouraoui, F., Benabdallah, S., Jrad, A., and Bidoglio, G. (2005). Application of the SWAT model on the Medjerda river basin (Tunisia). Phys. Chem. Earth Parts A/B/C 30, 497–507. doi: 10.1016/j.pce.2005.07.004
Bouzaiane, S., and Lafforgue, A. (1986). Monographie hydrologique des oueds Zéroud et Merguellil. DGRE–ORSTOM.
Braunschweig, F., Leitao, P., Fernandes, L., Pina, P., and Neves, R. (2004). The object-oriented design of the integrated water modelling system MOHID. Dev. Water Sci. 55, 1079–1090. doi: 10.1016/S0167-5648(04)80126-6
Brempong, M. B., Amankwaa-Yeboah, P., Yeboah, S., Owusu Danquah, E., Agyeman, K., Keteku, A. K., et al. (2023). Soil and water conservation measures to adapt cropping systems to climate change facilitated water stresses in Africa. Front. Sustain. Food Syst. 6:1091665. doi: 10.3389/fsufs.2022.1091665
Cecílio, R. A., Pimentel, S. M., and Zanetti, S. S. (2019). Modeling the influence of forest cover on streamflows by different approaches. Catena 178, 49–58. doi: 10.1016/j.catena.2019.03.006
Čerkasova, N., Ertürk, A., and Umgiesser, G. (2019). Assessing climate change impacts on streamflow, sediment, and nutrient loadings of the Minija River (Lithuania): A hillslope watershed discretization application with high-resolution spatial inputs. Water 11:676. doi: 10.3390/w11040676
Chen, X., Liang, Z., Zhang, Z., and Zhang, L. (2020). Effects of soil and water conservation measures on runoff and sediment yield in red soil slope farmland under natural rainfall. Sustain. For. 12:3417. doi: 10.3390/su12083417
Cherif, B., Mizouri, M., and Khaldi, R. (1995). Guide de Conservation des Eaux et du Sol, Projet PNUD/FAO, TUN/86/020. Tunis, Tunisia: PNUD/FAO, 274p.
Gachene, C. K. K., Nyawade, S. O., and Karanja, N. N. (2020). “soil and water conservation: an overview” in Encyclopedia of the UN sustainable development goals. eds. W. L. Filho, A. M. Azul, L. Brandli, P. G. Özuyar, and T. Wall (Cham, Switzerland: Springer Nature), 810–823.
Gashaw, T., Tulu, T., Argaw, M. W., and Worqlul, A. (2019). Modeling the impacts of land use–land cover changes on soil erosion and sediment yield in the Andassa watershed, upper Blue Nile basin, Ethiopia. Environ. Earth Sci. 78:679. doi: 10.1007/s12665-019-8726-x
Gashaw, T., Worqlul, A., Dile, Y., Addisu, S., Bantidar, A., and Zeleke, G. (2020). Evaluating potential impacts of land management practices on soil erosion in the Gilgel Abay watershed, upper Blue Nile Basin. Ethiopia. Heliyon. 6:e04777. doi: 10.1016/j.heliyon.2020.e04777
Gassman, P. W., Reyes, M. R., Green, C. H., and Arnold, J. G. (2007). The soil and water assessment tool: historical development, applications, and future research directions. Trans. ASABE 50, 1211–1250. doi: 10.13031/2013.23637
Gautam, V. K., Kothari, M., Singh, P. K., Bhakar, S. R., and Yadav, K. K. (2022a). Decadal groundwater level changes in Pratapgarh district of Southern Rajasthan. Ecol. Environ. Conserv. 28, 277–283. doi: 10.53550/EEC.2022.v28i01.040
Gautam, V. K., Kothari, M., Singh, P. K., Bhakar, S. R., and Yadav, K. K. (2022b). Analysis of groundwater level trending Jakham River Basin of Southern Rajasthan. J. Groundw. Sci. Eng. 23, 234–243. doi: 10.36953/ECJ.021936-2175
Gupta, H. V., Kling, H., Yilmaz, K. K., and Martinez, G. F. (2009). Decomposition of the mean squared error and NSE performance criteria: Implications for improving hydrological modelling. J. Hydrol. 377, 80–91. doi: 10.1016/j.jhydrol.2009.08.003
Henia, L. (2003). Les grandes sécheresses en Tunisie au cours de la dernière période séculaire, pp. 25–36, Université de Tunis, Faculté des sciences humaines et sociales, Tunisie © ENS Éditions. Available at: http://www.openedition.org/6540 (Accessed March 16, 2024).
Hermassi, T., Kotti, M. L., and Jarray, F. (2023). Soil Erosion in a Changing Environment over 40 Years in the Merguellil Catchment Area of Central Tunisia. Appl. Sci. 13:11641. doi: 10.3390/app132111641
Jarray, F., Hermassi, T., Kotti, M. L., and Mechergui, M. (2024). Soil erosion assessment risk using Modeling and spectral indices: a case study of Wadi Rmel Watershed, Northeastern Tunisia. J. Indian Soc. Remote Sens. 52, 1611–1621. doi: 10.1007/s12524-024-01878-2
Jarray, F., Hermassi, T., Mechergui, M., and Abdallah, M. A. (2023a). Assessment of runoff variability under various factors using SWAT model in a Tunisian semi-arid watershed. GSC Adv. Res. Rev. 17, 075–086. doi: 10.30574/gscarr.2023.17.2.0430
Jarray, F., Hermassi, T., Mechergui, M., Zucca, C., and Le, Q. B. (2023b). Long-term impact of soil and water conservation measures on soil erosion in a tunisian semi-arid watershed. Land. 12:1537. doi: 10.3390/land12081537
Jerbi, H. (2018). Anthropisation des processus hydrologiques autour de l’oued Merguellil Tunisie centrale: Caractérisation des formes d’évolution et quantification des flux. Thèse de doctorat en cotutelle: Universite de Montpellier/Universite de Tunis-Carthage. Available at: https://www.researchgate.net/publication/334281513 (Accessed November 22, 2023).
Jia, H., Wang, X., Sun, W., Mu, X., Gao, P., Zhao, G., et al. (2022). Estimation of soil erosion and evaluation of soil and water conservation benefit in terraces under extreme precipitation. Water 14:1675. doi: 10.3390/w14111675
Johanson, R. C., Imhoff, J. D., and Davis, H. H. (1980). User’s Manual for Hydrological Simulation Program-Fortran (HSPF), Environmental Research Laboratory, U.S. Environmental Protection Agency: Athens, GA, USA. EPA-600/9-80-015.
Keesstra, S. D., Bouma, J., Wallinga, J., Tittonell, P., Smith, P., Cerdà, A., et al. (2016). The significance of soils and soil science towards realization of the United Nations Sustainable Development Goals. Soil 2, 111–128. doi: 10.5194/soil-2-111-2016
Kingumbi. (2006). Modélisation hydrologique d’un bassin affecte par des changements d’occupation. Cas du Merguellil en Tunisie centrale. Thèse de doctorat. Université de Tunis El Manar Ecole nationale d’ingénieurs de Tunis.
Kouchi, D. H., Esmaili, K., Faridhosseini, A., Sanaeinejad, S. H., Khalili, D., and Abbaspour, K. C. (2017). Sensitivity of calibrated parameters and water resource estimates on different objective functions and optimization algorithms. Water 9:384. doi: 10.3390/w9060384
Lacombe, G., Cappelaere, B., and Leduc, C. (2008). Hydrological impact of water and soil conservation works in the Merguellil catchment of central Tunisia. J. Hydrol. 359, 210–224. doi: 10.1016/j.jhydrol.2008.07.001
Lakshmi, V., Alsdorf, D., Anderson, M., Nianmaria, S., Cosh, M., Entin, J., et al. (2015). Remote sensing of the terrestrial water cycle, Geophysical Monograph 206. Hoboken, NJ, USA: American Geophysical Union and John Wiley & Sons, 556.
Lazard, M. (2013). Etude des relations surface-souterrain système d’El Houareb (Tunisie Centrale) sous contraintes climatiques et anthropiques (p. P376). Thèse de doctorat: IRD, UMR G-Eau, Université de Montpellier.
Le Goulven, P., Leduc, C., Bachta, M. S., and Poissin, J. C. (2009). “Sharing scarce resources in a mediterranean river basin: Wadi Merguellil in Central Tunisia” in River basin trajectories: societies, environments and development. eds. F. Molle and P. Wester (Bodmin, UK: MPG Books Group), 147–170.
Li, L., Zhang, L., Wang, H., Wang, J., Yang, J., Jiang, D., et al. (2007). Assessing the impact of climate variability and human activities on streamflow from the Wuding River basin in China. Hydrol. Process. 21, 3485–3491. doi: 10.1002/hyp.6485
López-Ballesteros, A., Senent-Aparicio, J., Srinivasan, R., and Pérez-Sánchez, J. (2019). Assessing the impact of best management practices in a highly anthropogenic and ungauged watershed using the SWAT model: A case study in the el beal watershed (southeast Spain). Agronomy 9. doi: 10.3390/agronomy9100576
Maddocks, A., Young, R. S., and Reig, P. (2015). Ranking the World’s Most Water Stressed Countries in 2040. Washington, DC: World Resources Institute.
Merriman, K. R., Daggupati, P., Srinivasan, R., and Hayhurst, B. (2019). Assessment of site specific agricultural best management practices in the upper east river watershed, wisconsin, using a field-scale SWAT model. J. Gt. Lakes. Res. 45:619641. doi: 10.1016/j.jglr.2019.02.004
Meshram, S. G., Tirivarombo, S., Meshram, C., and Alvandi, E. (2023). Prioritization of soil erosion-prone sub-watersheds using fuzzy-based multi-criteria decision-making methods in Narmada basin watershed, India. Int. J. Environ. Sci. Technol. 20, 1741–1752. doi: 10.1007/s13762-022-04044-8
Ministry of Agriculture. (2017). Elaboration de la Stratégie de Conservation des Eaux et des Sols de la Tunisie, Technical Report, Ministry of Agriculure: Tunis, Tunisia, p. 202.
Moriasi, D. N., Gitau, M. W., Pai, N., and Daggupati, N. (2015). Hydrologic and water quality models: Performance measures and evaluation criteria. Am. Soc. Agric. Biol. Eng. 58, 1763–1785. doi: 10.13031/trans.58.10715
Mosbahi, M., Benabdallah, S., and Boussema, M. R. (2012). Assessment of soil erosion risk using SWAT model. Arab. J. Geosci. 6:40114019. doi: 10.1007/s12517-012-0658-7
Nafi, E. (2020). Interactive Tillage & Crop Residue Management Effects on Soil Properties, Crop Nutrient Uptake & Yield in Different Weathered Soils of West Africa. Bonn, Germany: Universität Bonn.
Neitsch, S. L., Arnold, J. G., Kiniry, J. R., and Williams, J. R. (2009). Soil and Water Assessment Tool Theoretical Documentation Version, Grassland, Soil and Water Research Laboratory, Agricultural Research Service and Blackland Research Center, Texas Agricultural Experiment Station, College Station, TX, USA
Ouessar, M., Bruggeman, A., Abdelli, F., Mohtar, R. H., Gabriels, D., and Cornelis, W. M. (2009). Modelling water-harvesting systems in the arid south of Tunisia using SWAT. Hydrol. Earth Syst. Sci. 13, 2003–2021. doi: 10.5194/hess-13-2003-2009
Pande, C. B., Moharir, K. N., and Khadri, S. (2021). “Watershed planning and development based on morphometric analysis and remote sensing and GIS techniques: A case study of semi-arid watershed in Maharashtra, India,” in Groundwater resources development and planning in the semi-arid region. eds. C. B. Pande and K. N. Moharir (Cham: Springer).
Prabhakar, M., Sasikala, D., and Varughese, A. (2022). Assessment of recharge in shallow groundwater aquifers using soil and water assessment tool: A case study of the thuthapuzha subbasin. Int. J. Environ. Clim. Chang. 12, 102–12. doi: 10.9734/ijecc/2022/v12i830729
Refsgaard, J. C. (1996). “Terminology, modeling protocol and classification of hydrological model codes” in Distributed hydrological modelling. eds. M. B. Abbott and J. C. Refsgaard (Dordrecht the Netherlands: Kluwer Academic), 17–39.
Roose, E. (1994). Introduction à la Gestion Conservatoire de L’eau, de la Biomasse et de la Fertilite des Sols (GCES), Bulletin Pedologique de la FAO 70. Rome, Italy: FAO.
Saadi, S. (2018). Spatial estimation of actual evapotranspiration and irrigation volumes using water and energy balance models forced by optical remote sensing data (VIS/NIR/TIR). PhD dissertation. University Toulouse 3: Paul Sabatier and INAT University of Carthage. Available at: https://www.researchgate.net/publication/323542990 (Accessed December 27, 2023).
Sellami, H., Benabdallah, S., La Jeunesse, I., and Vanclooster, M. (2015). Climate models and hydrological parameter uncertainties in climate change impacts on monthly runoff and daily flow duration curve of a Mediterranean catchment. Hydrol. Sci. J. 61, 1415–1429. doi: 10.1080/02626667.2015.1040801
Sorooshian, S., Hsu, K. L., Coppola, E., Tomasseti, B., Verdecchia, M., and Visconti, G. (2018). Hydrological Modeling and the Water Cycle: Coupling the Atmospheric and Hydrologic Models. Dordrecht, The Netherlands: Springer, 291.
Subhadip, K., and Sneha, M. (2017). Rainfall-runoff modelling of Ajay river catchment using SWAT model. 7th International Conference on Environment and Industrial InnovationVolume: IOP Conf. Series: Earth and Environmental Science 67:012033. doi: 10.1088/1755-1315/67/1/012033
Taguas, E., Ayuso, J., Pérez, R., Giráldez, J., and Gómez, J. (2013). Intra and inter-annual variability of runoff and sediment yield of an olive micro-catchment with soil protection by natural ground cover in Southern Spain. Geoderma 206, 49–62. doi: 10.1016/j.geoderma.2013.04.011
Uniyal, B., Jha, M. K., Verma, A. K., and Anebagilu, P. K. (2020). Identification of critical areas and evaluation of best management practices using SWAT for sustainable watershed management. Sci. Total Environ. 744:140737. doi: 10.1016/j.scitotenv.2020.140737
Vogel, J., Paton, E., Aich, V., and Bronstert, A. (2021). Increasing compound warm spells and droughts in the Mediterranean Basin. Weather Clim. Extrem. 32:100312. doi: 10.1016/j.wace.2021.100312
Winchell, M., Srinivasan, R., and Di Luzio, M. (2007). ArcSWAT Interface for SWAT2005—User’s Guide. College Station, TX, USA: Texas Water Resources Institute.
Yang, J., Abbaspour, K. C., Reichert, P., and Yang, H. (2008). Comparing uncertainty analysis techniques for a SWAT application to the Chaohe Basin in China. J. Hydrol. 358, 1–23. doi: 10.1016/j.jhydrol.2008.05.012
Keywords: SWC techniques, runoff, soil erosion, groundwater recharge, modelling, semi-arid watershed
Citation: Hermassi T, Jarray F, Tlili W, Achour I and Mechergui M (2025) Integrative hydrologic modelling of soil and water conservation strategies: a SWAT-based evaluation of environmental resilience in the Merguellil watershed, Tunisia. Front. Water. 7:1521812. doi: 10.3389/frwa.2025.1521812
Received: 02 November 2024; Accepted: 09 January 2025;
Published: 04 February 2025.
Edited by:
Hafzullah Aksoy, Istanbul Technical University, TürkiyeReviewed by:
Babak Vaheddoost, Bursa Technical University, TürkiyeCopyright © 2025 Hermassi, Jarray, Tlili, Achour and Mechergui. This is an open-access article distributed under the terms of the Creative Commons Attribution License (CC BY). The use, distribution or reproduction in other forums is permitted, provided the original author(s) and the copyright owner(s) are credited and that the original publication in this journal is cited, in accordance with accepted academic practice. No use, distribution or reproduction is permitted which does not comply with these terms.
*Correspondence: Taoufik Hermassi, dGFvdWZpay5oZXJtYXNzaUBpbmdyZWYudWNhci50bg==
Disclaimer: All claims expressed in this article are solely those of the authors and do not necessarily represent those of their affiliated organizations, or those of the publisher, the editors and the reviewers. Any product that may be evaluated in this article or claim that may be made by its manufacturer is not guaranteed or endorsed by the publisher.
Research integrity at Frontiers
Learn more about the work of our research integrity team to safeguard the quality of each article we publish.