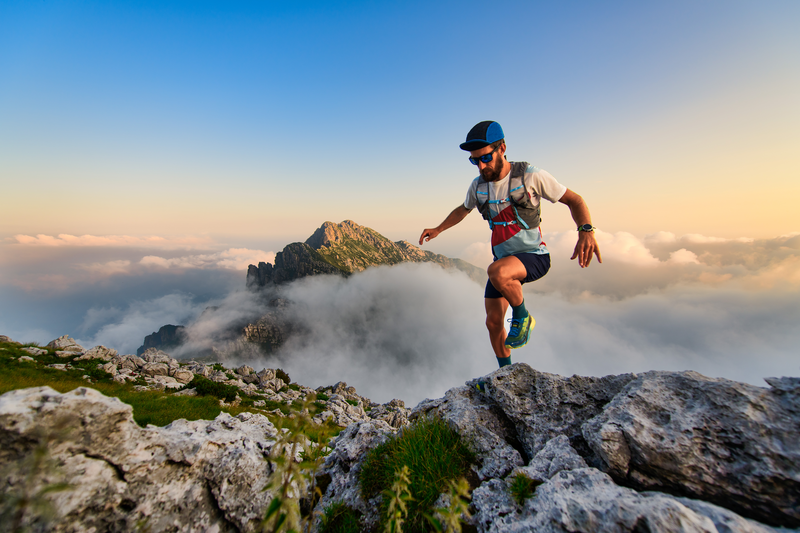
94% of researchers rate our articles as excellent or good
Learn more about the work of our research integrity team to safeguard the quality of each article we publish.
Find out more
ORIGINAL RESEARCH article
Front. Water
Sec. Water Resource Management
Volume 7 - 2025 | doi: 10.3389/frwa.2025.1266089
This article is part of the Research Topic Water Governance Across Management Scales View all 6 articles
The final, formatted version of the article will be published soon.
You have multiple emails registered with Frontiers:
Please enter your email address:
If you already have an account, please login
You don't have a Frontiers account ? You can register here
In the low-elevation plain above sea level, the south of Khuzestan province, Iran, productive activities and life of humans cause the water resource suffering from salinity issues, which can neither be recycled for agricultural production nor discharged into the Great Karun River. To protect the fresh surface water resource from salinization, a lagoon, also known as an evaporation pond, was formed to store and dispose of the drainage water. An investigation was carried out to assess the scale of water governance based on evaluating the effectiveness and efficiency of drainage water management, particularly in the discharge and salinity control of the lagoon. The result revealed that saline groundwater stays at a shallow level above the ground in the agricultural production area and is brought to the lagoon by the inappropriate layout of the drainage system, resulting in the phenomenon of overflooding in the study area. The overall discharge flows at a rate of 84.63 m 3 /s compared to the inflow threshold of 21.17 m 3 /s, and the disequilibrium is mainly caused by unexcepted saline groundwater and frequent exchanges of pond water in fish farms. The intensified level of salinity consecutively decreases the evaporation rate of the lagoon, potentially affecting the hydrological balance and altering the inflow threshold. The scale of water governance turns inefficient because of the reduced inflow threshold of the lagoon. In summary, cutting down the discharge and controlling the salinity of the lagoon should be jointly implemented to maintain the long-term sustainable development of water governance.
Keywords: Salinity control, discharge, inflow threshold, Evaporation rate, irrigation & drainage
Received: 24 Jul 2023; Accepted: 07 Mar 2025.
Copyright: © 2025 Mao, Riesbeck, Khodabakhshi and Mahjoobi. This is an open-access article distributed under the terms of the Creative Commons Attribution License (CC BY). The use, distribution or reproduction in other forums is permitted, provided the original author(s) or licensor are credited and that the original publication in this journal is cited, in accordance with accepted academic practice. No use, distribution or reproduction is permitted which does not comply with these terms.
* Correspondence:
Di Mao, Humboldt University of Berlin, Berlin, Germany
Disclaimer: All claims expressed in this article are solely those of the authors and do not necessarily represent those of their affiliated organizations, or those of the publisher, the editors and the reviewers. Any product that may be evaluated in this article or claim that may be made by its manufacturer is not guaranteed or endorsed by the publisher.
Research integrity at Frontiers
Learn more about the work of our research integrity team to safeguard the quality of each article we publish.