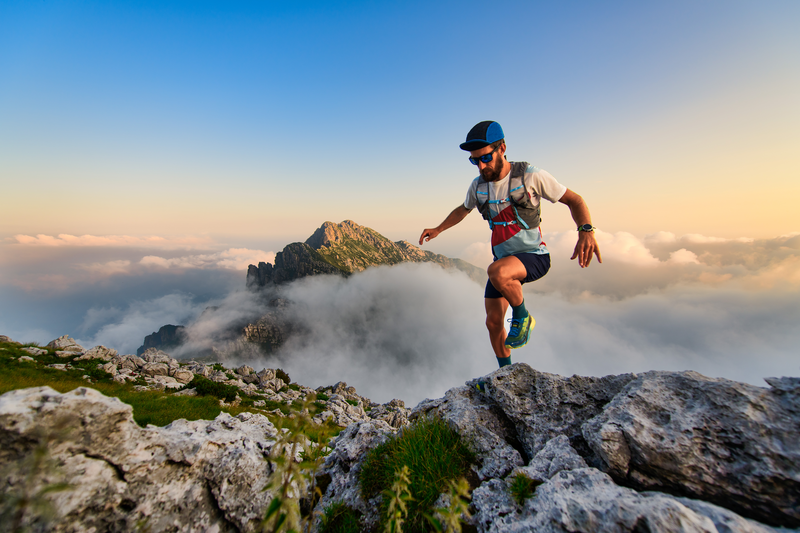
95% of researchers rate our articles as excellent or good
Learn more about the work of our research integrity team to safeguard the quality of each article we publish.
Find out more
ORIGINAL RESEARCH article
Front. Water , 20 November 2024
Sec. Water and Human Systems
Volume 6 - 2024 | https://doi.org/10.3389/frwa.2024.1492336
Introduction: In recent years, conflicts surrounding the use, distribution, and governance of surface water and groundwater in Germany have gained prominence in the media, on the political agenda, and in research. Increasing effects of climate change, such as heatwaves and drought but also extreme rain events and flooding, are considered to become more prominent and pressing in the future by different societal actors. However, it remains highly uncertain if and what type of conflicts related to water quantity Germany might actually face in the future (and how they will be framed). This paper addresses one dimension of this uncertainty—namely the future context uncertainty of possible resource and water governance conflicts. Our research contributes to an improved understanding of the uncertainty concerning future climatic, natural, land use related, political, economic, and other societal contexts that could impact water conflicts.
Method: We ask: What are possible coherent context scenarios for Germany in the year 2050, and how are they expected to influence future water conflicts? In an expert-based process, we apply a qualitative and systematic method of systems analysis, cross-impact balances (CIB). With CIB, we build internally consistent scenarios of possible futures and map the future scenario space.
Results and discussion: Diversity mapping with a new CIB web application of the ScenarioWizard reveals that the scenario space is rather large and diverse. The identified scenario space of n = 355 internally consistent scenarios spans four most diverse scenarios “Polycrisis,” “Economy and agriculture in crisis,” “Growth through adaptation to climate change,” and “Sustainable transformation.” Depending on the development of future contexts, the risk for future water resource and governance conflicts may unfold in various ways. We conclude that our scenario analysis provides a useful base for research and practice to address the context uncertainty of water conflicts in Germany. Our results can be used for risk assessment, to define societal framework assumptions for societal-hydrological modeling, and to develop robust and adaptive strategies and policies.
While Germany has historically been a country with abundant water resources, the last years have shown that water can become increasingly scarce—at least regionally and seasonally. The main reasons for this scarcity are attributed to climate change, which may lead to warmer and drier summers, changed precipitation patterns throughout the year (such as dry summers and heavy rainfalls in winter) (e.g., DWD, 2023; DVGW, 2022, 2023a), as well as poor infiltration of dry soils and high outflow rates, sometimes resulting in floods. These climate change effects exert pressure on freshwater reserves, groundwater renewal, and soil moisture (e.g., LAWA, 2017; DVGW, 2022; Wunsch et al., 2022). Such developments are expected to change the water balance in Germany (LAWA, 2022). At the same time, warm and dry springs and summers lead to increased water demand by private households, industry, agriculture, and water-related ecosystems, affecting both water quantity and quality. In the future, water extremes such as droughts and floods, associated with climate change, as well as conflicts between different water users are expected to increase (e.g., Stein et al., 2024; Tröltzsch et al., 2021; LAWA, 2022).
Conflict is defined as “a relationship between two or more parties (individuals or groups) who have, or think they have, incompatible goals” (Fisher et al., 2000, p. 4). Future water conflicts can be understood as (anticipated) conflicts related to the future use, distribution, and protection of surface and groundwater. They also comprise conflicts over protection against water and conflicts over the risk of water (e.g., assessment conflicts), conflicts over responsibilities and competencies to act, and, finally, the framing of a conflict. Framing conflicts or meta-conflicts (Feindt and Saretzki, 2010) may arise from contradicting perceptions and expectations of whether a conflict exists today, or is anticipated for the future, as well as the conflict type. To illustrate: If a problem is framed as scarcity, it necessitates more or alternative resources or a more economical use of available resources. If, on the other hand, a problem is framed as a distribution problem, new prioritizations and distribution keys must be developed. Future water conflicts broadly encompass both resource conflicts and governance conflicts (Dombrowsky, 2007). Governance is understood as the horizontal and vertical coordination and cooperation among various actors (Pahl-Wostl et al., 2020). Water conflicts also encompass conflicts regarding the appropriate goals and instruments (policies) related to resources and to governance.
Water scarcity and associated (future) conflicts in Germany are increasingly addressed by the media, research, and the political system. For instance, Joeres et al. (2022) regularly update reports from the media research group CORRECTIV on the website: https://correctiv.org/themen/kampf-um-wasser/. A scientific analysis documenting the rise of water conflicts as a media issue over the last years has been carried out by the WADKlim project (Stein et al., 2024) Future water conflicts in Germany have hardly been the subject of extensive research in social science or public policy (with the exception of the UBA WADKlim project, recently published in Stein et al., 2024).
Historically, Germany has experienced water conflicts before and in response, water issues have been subject to political regulation for decades (see, e.g., the Federal Water Act (WHG) since 1960, following state water acts from the 19th century, and the European Water Framework Directive since 2000). For instance, during the industrialization that led to the formation of the German Kaiserreich (empire), conflicts arose among different user groups: industrialists seeking to exploit water for production, municipalities in need of clean drinking water, and agricultural sectors dependent on clean irrigation sources. This situation prompted some of the earliest environmental regulations aimed at reducing water pollution, such as the Prussian Water Law of 1913, which regulated waste discharge into rivers (Cioc, 2002). In the aftermath of World War II, water pollution and resource depletion worsened due to rapid economic recovery and industrial growth. By the 1960s, the environmental degradation of German rivers became a significant public concern. Grassroots movements, scientific advocacy, and political pressures led to stronger regulatory frameworks to harmonize water use with environmental protection, setting standards for water quality and pollution control. Currently, in Germany, we observe a shift from water resource conflicts linked to industrial pollution to tensions related to climate change effects as drought events. As for governance conflicts, the German federal multi-level system can lead to tensions between federal and regional legislation. Furthermore, tensions between local interests (e.g., agriculture) and European Union (EU) legislation (especially under the European Water Framework Directive since 2000) do occur (Newig and Moss, 2010).
Currently, (potential) conflicts around water in the context of climate change in Germany are to a large extent an emerging problem. This means they are a new - or rather renewed - topic of public and political debate in Germany. Particularly at the federal and national levels, water conflicts are in the process of becoming visible public policy problems. Still, potential scarcity and water conflicts have already made their way to political agendas and documents, as indicated by a report by the German Working Group on water issues of the Federal States and the Federal Government (LAWA, 2022). Most prominently, the issue has been addressed by the national water strategy (BMUV, 2023), approved by the German Federal Parliament in 2023, which aims to guide water-related policy and regulation until the year 2050.
At the same time, uncertainty can be observed among various stakeholders in the water sector, broadly defined as all actors and structures involved in the use, distribution, protection, management and governance of surface and groundwater. This uncertainty refers to five different types (cf. Dewulf and Biesbroek, 2018; Walker et al., 2003): (a) data uncertainty regarding the current1 state of water usage (how much water do different user groups actually use?); (b) future or scenario uncertainty regarding the future development of the water situation (how might groundwater renewal rates develop in the future?); (c) framing uncertainty regarding the nature and severity of the problem (do we already have or will we have conflicts and if yes, what type of conflict?); (d) strategic uncertainty regarding (future) actors’ decisions and their interplay (if and how will—other—actors adapt to the new challenge and how will their actions impact mine?); and, finally, (e) context uncertainty (what natural and societal drivers influence future conflicts and how do they develop?). This paper primarily addresses this last dimension of uncertainty related to future water conflicts. These uncertainties are in addition linked to complexity (Kirschke et al., 2019). Climate change effects are not the only drivers influencing water futures and, more specifically, water conflicts. Instead, various factors as, e.g., land use, social, cultural and economic trends, as well as governance structures interact in the emergence of conflicts (Sneddon et al., 2002). These interactions are complex and their dynamics are at times non-linear. Future water conflicts in Germany are not only an emerging problem but can also be classified as a “wicked problem” (e.g., Head, 2022).
Preparing for a potentially conflictive and still uncertain future presents a current challenge for the water sector in Germany. The federation of German water providers (DVGW) asserts that “…to address the challenges of climate change, federal states, municipalities and actors of the water economy need to develop local and regional pictures of the future 2030–2050-2100” (URL: https://www.dvgw.de/themen/wasser/wasser-impuls/zukunftsbilder-2030-2100; own translation). Addressing future water conflicts pertains to the fields of adaptive governance (e.g., Folke et al., 2005), and even more so to anticipatory governance and its methods of futures-thinking (Alexandra et al., 2023). We focus particularly on the tool of scenario analysis (e.g., ElSawah et al., 2020) to address and manage future uncertainty and complexity (Kosow et al., 2022a)2. A scenario is understood as “…an internally consistent view of what the future might turn out to be—not a forecast, but one possible future outcome” (Porter, 1985, emphasis by the authors). Alternative scenarios (in plural!) describe the “scenario space,” i.e., the space of possible futures. Scenarios are used to transform future uncertainty—or openness—and complexity into a set of distinct, internally coherent alternative outlines of what might be (cf. also Grunwald, 2002). In contrast to forecast and prediction, which claim to provide information on alternative future presents (“zukünftige Gegenwarten,” Grunwald, 2011), scenarios are tools to reflect the knowledge, ideas, and expectations we have today with regard to the future (“gegenwärtige Zukünfte” ibid.). Scenarios on water futures have been developed for decades, with an early and prominent example being the World Water Visions (Gallopin and Rijsberman, 2000). There are water-centered scenario studies, primarily focusing on the effects of climate change—though less considering other drivers—on different scales: Regarding the water sector worldwide (e.g., OECD, 2012; UNESCO WWAP, 2022), in Europe (e.g., Ertug and Hoekstra, 2014; Mack et al., 2019) and also regarding Germany. Still, most of these latter scenario studies are sector - and region-specific (e.g., Shaochun et al., 2010; Wunsch et al., 2022) and/or were not (yet) publicly available (DVGW, 2023b). To our knowledge, there are currently no comprehensive water-related context scenarios for Germany—particularly none that: (a) consider the interplay of different context factors and (b) specifically address the potential drivers and contexts of water conflicts. This paper aims to fill this gap.
In summary, context uncertainty is a relevant challenge for water research and practice. Anticipating and addressing future water conflicts today requires an explicit understanding of the possible future situations in which resource conflicts and/or governance conflicts might unfold. Additionally, governance approaches (Pahl-Wostl et al., 2020) and policy instruments (Capano, 2024) exhibit different degrees of coherence and effectiveness under different contexts—and when future development is uncertain, robust and/or adaptive planning is necessary. Mapping the scenario space provides orientation knowledge that can be used by research, e.g., in the form of framework assumptions in socio-hydrological modeling, as well as in policy design. For administration and politics on different levels, it is highly relevant to identify strategies and policies that are robust under different possible futures: This concerns those designing and voting on regulations, as well as for administrations at regional and local levels that are in charge of issuing water usage rights. Context uncertainty also challenges water providers (public or private companies), who are facing very important investment decisions regarding future water infrastructures with effects and path-dependencies for several decades.
In this paper, we answer the following questions:
1. What societal (economic, political, etc.) and environmental (climatic, natural, etc.) contexts could impact future water conflicts in Germany?
2. What range of alternative developments of these contexts can be assumed as possible and plausible until the year 2050?
3. How can we condense this context uncertainty into coherent pictures of possible futures?
4. What context scenarios represent the scenario space in a comprehensive and diverse manner?
5. How might the risk of water conflicts in Germany in the year 2050 develop under different scenarios?
As Sivakumar (2011) suggests, we contribute an interdisciplinary study and methodology to better understand the future uncertainty of societal and environmental contexts for Germany through the year 2050. We focus on contexts that could impact both the hydrological situation and the water sector itself, as well as the potential future risk, type and intensity of conflicts related to water more specifically. Following Razavi et al. (2024), we apply an exploratory approach focusing on systems complexity “to navigate complexity and manage the uncertainty associated with understanding, diagnosing, predicting, and governing human-water systems” (Razavi et al., 2024, p. 2). We contribute a set of plausible, internally consistent and diverse qualitative scenarios of future contexts and assess their potential risk for water resource and governance conflicts. Second, this paper provides the qualitative systems model underlying the scenario space. In sum, this work supports the German water sector in developing future scenarios by providing a set of scenarios that encompass relevant developments outside the water sector itself. The future of the water sector, including central infrastructural options, could then be explored in a subsequent step, possibly using the methodological approach demonstrated in this paper.
In the following sections, we justify and explain the use of the semi-qualitative systems analysis CIB (cross-impact balances, Weimer-Jehle, 2006, 2023) to build and analyze exploratory context scenarios in an expert-based process (section 2). As results, we present the central drivers influencing future water-related conflicts, their plausible alternative future developments, and the interactions between these. We then provide a “map” of the future scenario space, bounded by a set of the four most diverse future scenarios, indicating their water conflict risk (section 3). We then discuss the limitations of our scenario analysis, explore the implications of our results for robust and anticipatory governance of potential future water conflicts in Germany, and outline potential use cases of these scenarios for water research and practice (section 4).
We justify and introduce the method CIB for exploratory context scenario analysis (section 2.1) and detail our specific expert-based and software-supported process of scenario construction and interpretation (section 2.2).
Scenario analysis is employed for various goals (e.g., Alcamo, 2008)—for instance, decision support and learning (Kosow and Gaßner, 2008). One of the most prominent goals is exploring possibilities and future spaces through exploratory scenarios (“What if” scenarios). Other goals include decision support and learning. Traditionally, exploratory scenarios are constructed using three different approaches: The first approach comprises qualitative, intuitive, and creative methods such as Intuitive Logics (IL) (Huss and Honton, 1987; Wilson, 1998). This is sometimes referred to as the scenario-axes approach if it maps future uncertainty spaces along two central axes, resulting in four scenario quadrants. The second approach, particularly used in the economic and engineering sciences, is numerical modeling to develop quantitative scenarios (e.g., Forrester, 1958, 1971). Finally, integrated modeling often relies on hybrid scenarios, combining qualitative storylines with numerical modeling, as in the Story And Simulation (SAS) approach (e.g., Alcamo, 2008).
Among the first type of approaches, Weimer-Jehle (2006) proposed CIB as a semi-qualitative scenario approach that allows for a more formalized construction of qualitative scenarios. CIB has been assessed as a promising alternative to IL (Girod et al., 2009; Kemp-Benedict, 2012; Schweizer and Kriegler, 2012; Schweizer and O’Neill, 2014; Lloyd and Schweizer, 2014; Kosow, 2015; Guivarch et al., 2017; Carlsen et al., 2016a; Carlsen et al., 2016b; Schweizer, 2020). CIB enables a “more systematic, consistent, complete, transparent and objective scenario construction process” (Prehofer et al., 2021). The method has been applied in various fields, such as energy research (e.g., Mier et al., 2023; Garcia-Teruel et al., 2022; Vögele et al., 2022), climate change (e.g., Schweizer and Kriegler, 2012; Schweizer, 2020; Drakes et al., 2020; Lazurko et al., 2022; Schuch et al., 2024), and sustainability studies (e.g., Renn et al., 2009; Kemp-Benedict et al., 2014; Carlsen et al., 2024), and increasingly in water research (e.g., Schütze et al., 2019; Kosow et al., 2022b; Lazurko et al., 2023). However, it has not yet been applied in the context of German water futures. Weimer-Jehle et al. (2016, 2020) have also demonstrated the use of CIB in hybrid scenarios processes, where CIB provides internally consistent qualitative scenarios on societal developments (framework assumptions) for numerical (economic, technological, or natural science) modeling. CIB-based qualitative scenarios informing hybrid scenario construction are called “context scenarios” (Weimer-Jehle et al., 2016, emphasis by the authors). Examples of CIB applications in hybrid scenario exercises are Ruth et al. (2015), Vögele et al. (2017), Schütze et al. (2019), and Pregger et al. (2020). A comparison of different hybrid methodological designs with CIB and their effects on interdisciplinary knowledge integration can be found in Prehofer et al. (2021). Given these advantages and experiences with the method, we chose CIB to qualitatively and systemically (instead of intuitively) explore the socio-natural contexts of future water conflicts.
CIB conceptualizes systems as qualitative impact networks (Weimer-Jehle, 2006, 2023). The main drivers of future developments (descriptors) are set as the nodes of the network. A small set of qualitatively and/or quantitatively defined alternative futures of the drivers (variants) are assigned to the nodes as discrete states. In terms of descriptors, consider a factor that can have multiple states (variants), each of which has the potential to influence the variants of another factor. Qualitative information about the promoting and hindering influences between the nodes is collected by literature review, expert elicitation, and/or stakeholder participation (cf. Prehofer et al., 2021). Table 1 provides an overview on the scale typically used to assess impacts between variants in a CIB analysis. Considering all direct mutual impacts pairwise results in a cross-impact matrix.
Table 1. CIB impact scale (Weimer-Jehle, 2006).
The core of the CIB method consists of a balance algorithm that scans all theoretically possible combinations of variant states and identifies the internally consistent configurations (consistent scenarios) within the impact network. These correspond to Nash equilibria (Nash, 1951), i.e., they are solutions where all descriptors are in a mutually reinforcing state, taking into account the hindering and fostering impacts they receive from other descriptors.3
A CIB process typically consists of four steps (Weimer-Jehle, 2006, 2023):
• Step 1: Identify, select, and define context factors (i.e., descriptors)
• Step 2: Identify, select, and define alternative future developments of each context factor (i.e., variants)
• Step 3: Assess cross-impacts between variants (pairwise).
• Step 4: Prepare and analyze the CIB matrix using the CIB algorithm to identify internally consistent constellations (i.e., scenarios).
However, we would like to add another step, that has sometimes remained implicit in prior CIB studies but is important for sense-making:
• Step 5: Interpret the scenario space and, potentially, select a (smaller) set of scenarios (i.e., sampling), elaborate these into storylines (Tori et al., 2023).
In addition to the consistency of descriptor constellations, CIB also provides information on the synergy of each constellation by calculating the total impact score (TIS), i.e., the sum of all negative and positive impacts active in this constellation. The TIS can be understood as a measure of the overall synergy of a scenario, i.e., the sum of mutually hindering and promoting impacts. The TIS provides relative (not absolute) values, as it is sensitive to the number of descriptors, variants, and impacts. The use of CIB is supported by the software ScenarioWizard, available for free download at: www.cross-impact.org (accessed on June 18, 2024). We also utilized the beta version of an online version of the ScenarioWizard that does not require any download and is now easily accessible on all operating systems.4 Both software versions offer further options for analysis, those that have been applied in this study will be specified below (section 2.2).
The scenario scope has been defined by the group of authors to identify the scenario space of contexts impacting future water conflicts in Germany, with a time horizon set for the year 2050. This aligns with the time horizon of the German national water strategy. Additionally, the year 2050 is distant enough to consider various alternative futures but not so far (as, for example, the year 2100 might be) that it introduces excessive uncertainty, making scenario analysis less applicable. The concrete methodology, following Hinkel (2008)—i.e., the constellation of people, techniques, and data used to construct and interpret these context scenarios—is detailed below.5 A full list of participating experts is provided in Supplementary A.
To identify relevant context factors for future water conflicts in Germany and their possible future developments until 2050, we organized an expert workshop with a total of n = 14 experts from hydrology, political science, economics, sociology, climatology, and practice actors from the water sector. In addition, we scanned existing studies on water futures for possible additional factors. As literature regarding German water futures is still scarce (for exceptions, see Shaochun et al., 2010; Wunsch et al., 2022), we also included studies related to water futures on a global (e.g., Gallopin and Rijsberman, 2000; Alcamo et al., 2003; OECD, 2012; UNESCO WWAP, 2022) and European scale (e.g., Leipprand et al., 2008; Ertug and Hoekstra, 2014; Mack et al., 2019; EEA, 2021). To select the most important factors, we conducted an internal workshop among the authors, assessing the uncertainty and impact of the different factors (cf. Amer et al., 2013). We included those factors that exhibited the highest degrees of both future uncertainty and impact on future water conflicts as these were considered relevant and most interesting to explore the uncertainty space.
To refine descriptors and variants, the team of authors drafted selections and definitions of descriptors and variants in the form of comprehensive descriptor essays, supported by additional literature research (see Supplementary B). A draft of each essay was then reviewed and validated by one to two external experts, mainly but not exclusively from academia (see Supplementary A). The validation review focused on the plausibility of the different future developments (i.e., the variants): Variants were defined in a way that their occurrence was considered possible—but not necessarily very probable until the year 2050. We thus agreed on a middle ground between choosing highly probable developments that mostly lead to “surprise-free” scenarios vs. choosing controversially assessed contingencies which can lead to striking but speculative scenarios (Weimer-Jehle, 2023). Further input and validation were collected through a workshop with n = 14 practice experts, comprising stakeholders from agriculture, water providers, nature and environmental protection, and city administration. This workshop led to the inclusion of the context factor “state of ecosystems” into the final short list (see section 3.1).
To assess if and how the alternative developments (variants) of the context factors (descriptors) influence each other, we conducted a two-phase process of impact assessment. First, the team of authors split into pairs, and these pairs jointly discussed and then assessed those descriptor-interactions they had the most expertise (splitting the matrix by rows). These initial assessments were then integrated into a first joint CIB matrix. This internal matrix served as a preparation and background knowledge for the second phase. In the second phase, we conducted online tandem interviews with two external issue experts simultaneously. We asked experts about the impacts they saw on “their” descriptor from the other descriptor variants (splitting the matrix by columns). In total, we interviewed a group of n = 15 experts from various disciplinary backgrounds, some of whom were willing to assess more than one descriptor. The tandem interview combined the advantage of expert interviews to collect individual experts’ knowledge with the necessity to discuss and justify assessment with a peer, thereby reaching a consensual understanding to reduce subjective and mono-disciplinary expert bias (cf. Kosow et al., 2022c). We noted verbal justifications of the impact assessments and recorded the interviews.
We combined the results from the tandem interviews and constructed one joint CIB matrix containing the final definitions of descriptors and variants, all impact assessments, and their verbal justifications. We then analyzed the matrix using the CIB balance algorithm supported by the CIB software ScenarioWizard and searched for internally consistent scenarios, i.e., scenarios without contradictions. This means we searched for combinations of variants that are stable in themselves.
We utilized the CIB software and web-application to interpret the scenario spaces represented by our CIB matrix. First, an analysis of active and passive sums (Vester, 2002) helped in understanding the direct effects of highly impactful and more dependent context factors. Next, we applied: (a) diversity sampling to identify four maximally diverse scenarios, and (b) the scenario axis (from the online version) to map the entire scenario space according to diversity in a scenario-axes style. This diversity mapping—which is more systematic than the one proposed by IL, and inductive rather than deductive—follows the min-max approach by Tietje (2005). The x-axis is formed by the two scenarios with the maximum difference in variants, while the next two most distinct scenarios define the y-axis. All other scenarios are sorted in relation to their similarity with these four most diverse (and most extreme) scenarios, i.e., their distance from both axes. We then interpreted the themes of the axes and quadrants content-wise.
The most diverse scenarios were elaborated into storylines (short versions in 4.3, long versions in Supplementary D). Finally, to demonstrate a possible use case application, we assessed the impacts of all scenarios on water conflict risk among the authors. We added the two passive descriptors “risk of water resource conflict” and “risk of water governance conflict” into the matrix and used these as indicators. We defined water resource conflicts as conflicts regarding the use, distribution and protection of water resources, and water governance conflicts as conflicts regarding the management, competencies, coordination, cooperation, and framing of water (problems) in the water sector comprising public and private actors. Risk was understood as a combination of hazard, vulnerability, and exposure (cf. IPCC, 2018). We assessed the impacts of all context variants on both conflict risks using the −3 to +3 CIB scale calculating and interpreting individual risk values for both dimensions, as well as an (equally weighted sum) water conflict risk index for all scenarios.
Identifying, selecting, and defining context factors resulted in a list of 11 factors (i.e., descriptors) with three to five alternative developments each (i.e., variants) until the year 2050. These developments cover the domains of climate, land use, politics, economy, society and water culture, and nature. Technological development has not been included in the context factors, as infrastructure and technology are rather part of the water system/sector itself—and thus not depicted by the context scenarios. Table 2 provides an overview of the contexts (descriptors) and their respective alternative developments (variants) that have been considered possible (but not necessarily highly probable) in Germany in the year 2050 by the participating experts. Detailed definitions can be found in Supplementary B, with referenced literature in Supplementary E.
Table 2. Uncertain context factors until the year 2050, influencing future water conflicts and their governance in Germany (water related instruments and strategies excluded; geographical reference: Germany, unless otherwise specified; detailed definitions see Supplementary B).
Step 3 of the CIB process, assessing the interrelations between the alternative developments, results in a conceptual model of factor interrelations stored in the form of a CIB matrix (Weimer-Jehle, 2006). Two special features of this particular CIB model should be noted. First, the factors H “Structural development of the water-intensive industry (energy transition)”6 and A “Change in temperature” are defined as autonomous factors, as they are primarily dependent on influences outside the scope of the considered system (i.e., exogenous factors from the “rest of the world,” such as global greenhouse gas emissions, global peace and war developments, and access to raw materials). The assumed influences of the other factors on H and A are documented in the text accompanying the matrix but do not play a numerical role, as these impacts are considered too weak (value 0 in the matrix). Conversely, the impacts of the energy transition (H) and temperature change (A) on other factors are considered. Second, initially, demographic development was considered a relevant context factor for water conflicts in Germany by the participating experts. However, the impact assessment process revealed that: (a) on a national average, the changes (the plausible variance) in demographic development until the year 2050 might be too small to be interesting to consider. This may differ significantly for regional or local developments though. It also revealed that: (b) the impacts of the selected factors of this analysis on the demographic development of Germany were considered as low and demography was recognized to be an inert development. The primary potential forces influencing demographics in Germany until 2050 are international migration fluxes, which are beyond the scope of this analysis. Consequently, demographic development (J) has been excluded from the remainder of the analysis, focusing solely on the impact network of 10 contextual factors.
The distribution of cross-impacts between variants assumed by the experts is rather typical (cf. Weimer-Jehle, 2023). Supplementary C provides the CIB matrix that indicates impact sectors of important interrelations through color coding, as well as a summary table on the distribution of impact assessments across all impact cells. The factors that exert the highest direct impact on the others (active factors) are, in decreasing order of their total impact, I “Water awareness and prioritization,” B “Changes in precipitation,” F “Political-regulatory framework” and A “Change in temperature.” The factors that receive the most direct impacts from other factors (passive factors) are K, “State of ecosystems” and to a lower degree also C the “Development of agriculture” in Germany, and interestingly, also E “Water governance,” which is seen as at least slightly influenced by most of the other developments. Still, this analysis does not consider the indirect impacts of the mutual influences between factors yet. These are considered in the following, i.e., in the analysis of the entire impact network with the CIB balance algorithm (section 3.3).
Analyzing the CIB matrix with the CIB algorithm results in 311,040 theoretically possible variant combinations. Not all of these combinations are sensible, as most of them contain internal contradictions. N = 74 scenarios are fully consistent (IC0) in the sense of CIB, and these scenarios include 26 out of the 36 variants. Allowing for small inconsistencies regarding individual descriptor states (IC1) results in a set of n = 355 scenarios, encompassing 34 out of 36 variants, i.e., almost the entire scenario space identified.7 The two variants absent from this latter set are C1 and E3. C1, i.e., “Hardly any changes in agriculture: Production and productivity as in 2020s,” has been considered less probable (but still plausible) than the other variants by experts in the agricultural sector, as agriculture is currently undergoing strong transformation pressure. Variant E3, “No effective cooperation, (cross-border) water conflicts,” has also been deemed rather less probable, at least if the general political conditions (democratic system and peace in the European Union—factors not explicitly considered in this analysis) remain intact until 2050. Thus, the lack of these two variants can be considered meaningful in our set of plausible scenarios.
To facilitate a better understanding and easier navigation of our scenario space by potential users of this study, we selected a small number of maximally diverse scenarios. For this purpose, we applied diversity sampling and diversity mapping with a scenario axis approach (see section 2.2). Figure 1 shows the overall results. Diversity sampling yields two maximally diverse scenarios out of n = 355 (IC1) that serve as extremes of the x-axis: “Polycrisis: Climate, politics, and environment in crisis” (scenario 1) vs. “Sustainable transformation” (scenario 4). These two scenarios differ regarding all descriptor variants. The next two scenarios, which differ maximally from each other and to the extremes of the x-axis, form the y-axis.8 We called these scenarios “Growth through adaptation to climate change” (scenario 2) and “Agriculture and economy in crisis” (scenario 3). The four maximally diverse scenarios diverge with regard to at least six out of 10 descriptor variants. They are marked by red dots in Figure 1. Table 3 presents the structure of the scenarios, while Table 4 provides short storyline descriptions. Longer versions, including descriptions of key interactions, can be found in Supplementary D. These four scenarios represent the extremes of the scenario space that has been deemed plausible in our analysis. They cover a maximum of future diversity by considering a small and cognitively discernible number of scenarios.
Figure 1. ZuWaKo context scenarios (n = 355) mapped by diversity [n = 355 scenarios at consistency level 1 (IC1), i.e., very slight inconsistencies allowed; red dots and titles mark the selected set of four maximally different scenarios].
Table 3. Overview of four selected, most diverse scenarios: Germany 2050–possible contexts for water conflicts (to be read column-wise).
The four extreme scenarios are neither equally consistent nor equally synergistic (see Table 3). Scenario 2 is particularly consistent and demonstrates a highly positive balance of mutual impacts between developments, as indicated by the highest total impact score (TIS). In contrast, scenario 4 shows slight inconsistencies in five out of 10 descriptors and has the lowest TIS among these four scenarios. Thus, the sustainable transformation scenario (scenario 4), while considered plausible in this analysis, can be considered as rather unstable. This implies that it is either easily disturbed by contingencies impacting the slightly inconsistent descriptor states (B2, E1, G3, K2) or requires particular attention in the form of additional policies and strategies to stabilize these developments. Interestingly, strong EU regulations (F2 “EU regulations implemented and complemented by a systems perspective”) can be found in three of the four scenarios (2, 3, and 4). Such a political framework seems consistent with different agricultural futures (C3 “High-Tech,” C5 “Loss of importance” and C4 “Organic farming”), which depend on constellation of the other context developments. K2 “Recovery” of ecosystems is plausible in two worlds: through a pathway to sustainable transformation in scenario 4, or through strongly reduced agricultural and economic activities —combined with strong regulations—in scenario 3. Scenario 1 “Polycrisis” has “twins,” meaning scenarios that are identical, equally consistent, and differ only in terms of precipitation (all variants of precipitation change are consistent). Scenario 2 “Growth through adaptation” has twins regarding all temperature variants. Thus, this scenario is rather stable and considered plausible under all different temperature developments.9 The other two selected diverse scenarios are not fully consistent in the case of differing climatic situations.
Numerous further consistent alternatives between these extremes can be found. Considering the entire space of n = 355 scenarios mapped in relation to the four most diverse scenarios, allows for a better understanding of the thematic axes and the typical characteristics of the four quadrants. Figures 2a–h indicate how different variants are distributed across the scenario space. Figure 3 provides an overview on the characteristics of the scenarios found in the four quadrants (A–D).
Figure 2. (a–h) Distribution of selected scenario characteristics across the ZuWaKo scenario space (grey/light dots indicate scenarios with the respective characteristic, blue/dark dots indicate all other scenarios).
Figure 3 illustrates that the x-axis divides the scenario space into scenarios characterized as sustainable vs. unsustainable (regarding environmental and socio-economic conditions); the y-axis distinguishes between scenarios oriented towards growth (and a development closer to the status quo) vs. those characterized by either economic decline or a degrowth logic (and overall greater changes compared to today). The overwhelming majority of consistent and synergetic scenarios are located in quadrant A, between the scenario of “Polycrisis” (scenario 1) and “Growth through adaptation to climate change” (scenario 2). The scenario with the highest synergy score (TIS 58)—presumably the most stable scenario—lies within this quadrant. This scenario depicts a growth scenario with a failing energy transition and the deterioration of ecosystems. Figure 3 also highlights that scenario 3 (“Agriculture and economy in crisis”) is a singular outlier. Additionally, at the intersection of both axes, there is an additional cluster (E) of scenarios that show their own characteristics10. Scenarios in cluster E combine distinct features from both quadrants B and D (C3 or C4, D1, E2, F2, G3, H1, I1, K2) under multiple challenging climate constellations.
Regarding individual variants, scenarios with a failing energy transition (H3) (Figure 2a) are located away from “Growth through adaptation” (scenario 2) toward “Polycrisis” (scenario 1). If economic recession (G4) is added to the picture (Figure 2b), scenarios are found in quadrant C. On the other side, organic farming (Figure 2c) characterizes most scenarios on the sustainability side of the scenario space. “Future stagnation of temperature increase” (A4) (Figure 2d), while present across the entire scenario space, is a typical feature for all scenarios in quadrant B. As to governance and regulation, “Rules bypassed” regulations (F4) (Figure 2e) are typical for scenarios in quadrant C, whereas “All you can take” (F3) (Figure 2f) regulations are present on the entire unsustainable side of the scenario space. Interestingly, “Strong cooperation and coordination” (E1) (Figure 2g) is—in individual cases—present at the most sustainable end of the scenario space but more often in challenging economic situations at the crossroad between more or less sustainable worlds. In contrast, highly ambitious EU regulations comprising a WEFE Nexus approach (F2) (Figure 2h) are present across the entire scenario space, albeit less to the unsustainable end of the spectrum and considerably less in times of recession (quadrant C).
Mapping the results of the water conflict risk assessment across the scenario space (Figure 4a, index summing resource conflict and governance conflict risk) reveals that water conflict risk is distributed very unequally across the scenario space and, not surprisingly, is considerably higher at the “unsustainable” end of the spectrum (see Figure 4). Still, when comparing conflict risk and synergy (total impact score) of all scenarios (Figures 4a,b), the overall stable scenarios (high TIS) show a rather important water conflict risk, whereas scenarios with low conflict risk on the ‘sustainability side’ of the scenario space tend to be less solid. The total impact score is positively correlated to both individual types of conflict and has a strong positive correlation with overall conflict risk (r = 0.63, p < 0.0001).
Figure 4. Distribution of (a) conflict risk, (b) total impact score (TIS), (c) resource conflict risk, and (d) governance conflict risk across the ZuWaKo scenario space. Please note that the scales of the four figures have not been normalized.
Differentiating between the risk for resource conflict and the risk for governance conflict across the scenario space indicates that these conflict types might not always occur at the same time. While scenarios close to scenario 2 (growth through adaptation to climate change) and in the upper left quadrant show both, high risk for resource and governance conflicts, scenarios close to scenario 1 (“Polycrisis”) and especially in the lower left quadrant C are marked by resource conflicts. Overall, resource conflicts and governance conflicts show a highly significant (p < 0.001), weak negative correlation (r = −0.20) in our sample. Scenarios with a higher risk for resource conflicts tend to have a lower risk for governance conflicts and vice versa.
We discuss the limitations and strengths of our scenario analysis (section 4.1), the implications of our results for future water conflicts and their governance in Germany (section 4.2), and the usability of these context scenarios for different potential users (section 4.3).
First, CIB is primarily a qualitative approach and does not claim to generate predictions. Instead, it provides multiple, internally consistent, and comprehensive pictures of possible futures. It does so by analyzing a conceptual model reflecting the mental models of those who provided knowledge and input. Its strength is to consider the complex interplay of aspects that are difficult to quantify and model in numerical ways (Weimer-Jehle, 2023). Future research could and should combine this analysis with quantitative data and numerical models whenever available.
The selection of descriptors and variants—i.e., the inclusion and exclusion of system elements in our conceptual model, can be viewed as a second general limitation. The selection criteria for our context factors were a high degree of uncertainty and a strong impact on water conflicts in Germany. To depict other potential futures of the water sector, the list of descriptors might need to be reconsidered. For instance, it could be beneficial to include technological trends such as grey vs. blue vs. blue-green infrastructure; the development of a hydrogen economy; and digitalization in the water sector. Alternatively, depending on the study’s focus, the CIB model might not only encompass external contexts but also depict the entire water sector and the hydrological system itself in form of internal factors. In addition, we did not consider the development of context factors such as war vs. peace or democracy vs. autocracy, or strong raise of populism, which could also become relevant by 2050. Furthermore, we excluded external international factors (beyond national borders), such as world market stability, virtual water (i.e., for crop or industrial production in other countries),11 effects of climate change and climate change agreements on other regions that might induce changes within Germany as adaptation or compensation measures (i.e., unprecedented climate migration). Thus, the selection of variants that are considered implausible (possibly too narrow or too broad) has to be adjusted depending on the study’s objective and the “conservative” vs. “creative” character of the scenarios.
The third limitation—which is a constraint of every expert-based CIB analysis—relates to the selection of experts and their assessments, which may exhibit bias; the impact assessments might have differed with a different selection of experts. To counter this issue, including many experts from diverse backgrounds and integrating multiple assessments would be best practice. This has been partially achieved in this study through tandem interviews with experts from various fields who discussed their judgments with each other. Therefore, we believe that our scenarios are useful. Centrally, they do not make predictions or statements regarding the probabilities of individual scenarios but rather can be considered as the scenario space of consistent factor relations, depicting the range of diverse potential future developments, which we can anticipate as plausible today. Scenarios are always open to critical discussion and rather an occasion for dialogue rather than making truth claims: If you, as a reader do not find them plausible (too extreme or too conservative, or containing implausible assumptions on interrelations?), you are invited to ask yourself: “Why? What are my ‘present futures’?” This questioning allows making pictures of the explicit, shareable and discussable.
Fourth, the demonstrated water risk assessment should be interpreted with caution, as it relies solely on estimates by the team of authors. Then, most importantly, conflict risk does not only depend on contexts but also heavily on the actions and behaviors of stakeholders in water politics, administration, economy, environmental protection, and agriculture. In addition, a more regional or even local perspective, as well as an explicit multi-level governance perspective, could provide additional useful insight on water conflict risks beyond our rather general assumptions referring to Germany as a whole. Future research should combine our approach with more detailed risk assessment techniques.
Nonetheless, our results allow deducing several implications regarding future water conflicts and their governance in Germany. The future context uncertainty and the mapped scenario space we found are rather broad. Society and in particular the water sector—including public administration on different levels need to navigate this uncertainty. This also means to develop and implement robust and adaptable policies and strategies to anticipate and address these future conflicts.
We have assumed from the outset that climate change-related factors (changes in temperature and precipitation) would play an important role for future water conflicts in Germany. Yet, our analysis paints a more differentiated picture by showing the important impacts of other, especially governance related, context factors as “political-regulatory frameworks” (F) or societal “water awareness and prioritization” (I). This is plausible, as Germany still enjoys a relatively comfortable situation from an international perspective, characterized by an initial abundance of water and successful buffering through ground water stocks in many regions. Regional and seasonal issues may be issues of distribution and access (technical access, i.e., through infrastructure, or legal access, i.e., through water rights) than of absolute scarcity.
Mapping the scenario space revealed a dominance of unsustainable economic growth or recession scenarios (across climate change variants) vs. relatively few and less synergetic scenarios of sustainable transformation, in which the variant “green liberation,” depicting a degrowth scenario of the German economy, dominates (see quadrant D in Figure 3). Additionally, the latter seem rather dependent on not too dramatic developments of climate change (see quadrant B in Figure 3). We also demonstrated that the energy transition plays a crucial role, not only due to its direct impact on the water use of the water-intensive (energy) industry but also through indirect impacts of a (failed or successful) energy transition on other context factors, which, in turn, add to the risk of water conflicts.
Our conflict risk assessment (section 3.3.3) revealed that: (i) overall stable scenarios (high TIS) correlate with higher water conflict risk, whereas scenarios with low conflict risk tend to be less solid, i.e., more prone to transitory situations and potentially less sustainable conditions. Although this result should not be over-interpreted in terms of probabilities or pathways, it does suggest that more sustainable context factor constellations with low water conflict risk appear to face greater trade-offs and internal instability compared to less sustainable constellations with high water risks, which may tend to stabilize and persist. Social science conflict theory indicates that latent, escalated, and collaborative conflicts (Fienitz and Siebert, 2023) play different roles for system stability vs. change in land use governance. Future research could apply this theoretical angle to water conflicts, too. Then the conflict analysis revealed (ii) that resource and water governance conflict risks do not appear to occur in parallel across all scenarios. Instead, some scenarios with reduced water resource conflict risks exhibit relatively high governance conflict risks. These scenarios often show ambitious regulation as WEFE Nexus approaches, but only medium to weak levels of cooperation and coordination in the water sector (see Figures 2g,h). This constellation seems to either make latent, previously unaddressed governance conflicts visible or to generate new governance conflicts. For illustration: strict protection of water-related ecosystems might, in the long term reduce resource conflict risk, as water quantity and quality improve—but at the same time, the risk of conflicts around the procedures and processes of intensified coordination, cooperation, and complex prioritization schemes increases, especially if this coordination and cooperation are only weakly institutionalized. Our results thus indicate that investing in more ambitious policy frameworks alone, without enhancing coordination and cooperation in the water sector, might shift but not solve water related conflicts. Social sciences conflict theory (for an overview, see Bonacker, 2008) confirms that conflicts are often not permanently resolved but rather shifted or transformed from one level to another (e.g., from national to local) or from one type to another (from resource conflicts to governance conflicts in our case).
Finally, “[u]ncertainties are not experienced in the same way by different people. Knowledges about the present and perspectives on the future are all constructed in particular contexts.” (Scoones and Stirling, 2020, p. 4). The uncertainty about future contexts identified among participating experts could also be related to framing uncertainties—for example, if and what type of conflicts (resource conflicts, governance conflicts, combinations, or others) might occur, and how severe these conflict risks might be in the future. Put differently, today’s framing conflicts might be linked to diverging representations of future contexts. This means that depending on what future context scenario(s) different actors expect, their anticipation and assessment of future conflict risk—and their assessment of the need to act to deal with these from now on—might also vary.
We believe that this study provides a useful foundation for research at the interface of water and human systems and for the water sector to deal with the context uncertainty linked to future water conflicts. We discuss potential use cases for (a) the scenarios, (b) the matrix, and (c) the CIB method itself.
The set of plausible, internally consistent and diverse qualitative scenarios on future contexts (and the corresponding map) can be utilized for various purposes: First, they can be used to formulate a comprehensive set of context assumptions for quantitative modeling, for instance, regarding the future water need agriculture or of urban green. These context assumptions then need to be quantified to serve as input parameters. Then, these scenarios can serve as systematic context scenarios in a hybrid scenario process, linking storylines and models (e.g., Weimer-Jehle et al., 2016; Prehofer et al., 2021). Second, the set of scenarios can support policy research and policy-design, especially on the national level to further develop the national water strategy (BMUV, 2023). For instance, policy-design can use these context scenarios to test the robustness of different strategies and measures under different possible futures, as well as their adaptability and flexibility in navigating different pathways across the scenario map. Third, this work can assist the German water sector in developing explorative and normative pictures of the future by providing a set of diverse scenarios covering relevant developments outside the water sector itself, which will impact the water sector and the conflicts it might be facing. This could inform risk assessment and strategy development. As Scoones and Stirling (2020) point out (21): “uncertainties can be generative of diverse, imagined alternatives. By opening up spaces to re-imagine futures, to dream and to construct alternatives, uncertainties can be confronted in positive ways: not as threats or sources of fear, but as sources of hope and possibility.”
This paper also provides the qualitative systems model underlying the scenario space, stored in the form of the CIB matrix. This conceptual model can be utilized by research, policy advice, and administrations for the case of Germany as it stands. Alternatively, it can be used as a starting point to be adapted and adjusted by research groups and/or consultants building their own water scenarios. Adaptations might be necessary if the plausibility of future developments is assessed differently, if the definition of variants needs to be changed, or if experts assess interactions differently. Also, if the model is adjusted to different research foci, including further descriptors, as well as to further geographic and temporal scales (as local, regional, global or, especially relevant, multi-level scenarios—or other time horizons, closer or further into the future) adaptations are possible and potentially necessary. For instance, it is possible to add external global developments (as, e.g., international climate agreements) as active factors to the CI matrix to explore their impact on the possible situation in Germany.
CIB, as a scenario method demonstrated by this paper, can be used by research, water management professionals, and administrations to build scenarios not only of the contexts of future water conflicts but also of the water system and its potential conflicts. Such a project could include descriptors to depict the water sector and different types of conflicts in terms of trends and drivers and build system scenarios (Gausemeier et al., 1998). With our application, we have shown, and this is a methodological innovation—that it is possible to analyze a scenario space identified CIB by using a scenario axis approach. Compared to IL, the CIB scenario axis analysis is systematic-inductive: it is based on systematic scenario construction with CIB and systematic diversity measures—and it requires to inductively interpret the two central axes. This approach results in a more complex, less straightforward mapping of the scenario space than with IL. In contrast, IL is more intuitive in defining the two main axes spanning the scenario space and then deductive to define the character of the quadrants.
Future applications of CIB to build water (conflict) scenarios could also involve stakeholders (potential conflict parties) of the water sector and their policy options (goals and alternative instruments) in the form of policy-mix scenarios (Kosow et al., 2022b; Kosow et al., 2022c; Kosow et al., 2023). These policy-mix scenarios would allow for the exploration of the strategic uncertainty of future water conflicts by examining the effects of measures and strategies from different stakeholders and their combinations (policy-mixes) under different scenarios. We recommend to follow this research avenue to address future water conflicts in Germany through robust policy-design.
The original contributions presented in the study are included in the article/Supplementary material, further inquiries can be directed to the corresponding author.
HK: Conceptualization, Data curation, Formal analysis, Funding acquisition, Investigation, Methodology, Project administration, Resources, Supervision, Validation, Visualization, Writing – original draft, Writing – review & editing. SB: Data curation, Investigation, Methodology, Writing – original draft, Writing – review & editing. AB: Data curation, Investigation, Methodology, Writing – original draft, Writing – review & editing. WH: Conceptualization, Formal analysis, Writing – original draft, Writing – review & editing. FH: Data curation, Investigation, Methodology, Writing – original draft, Writing – review & editing. JM: Data curation, Investigation, Methodology, Writing – original draft, Writing – review & editing. DR: Funding acquisition, Supervision, Writing – original draft. SV: Conceptualization, Funding acquisition, Methodology, Supervision, Validation, Writing – original draft, Writing – review & editing. WW-J: Conceptualization, Formal analysis, Funding acquisition, Project administration, Software, Supervision, Validation, Writing – original draft, Writing – review & editing.
The author(s) declare that financial support was received for the research, authorship, and/or publication of this article. This study was supported by the Daimler and Benz Foundation (Grant 41-02/22) for the project ZuWaKo-Future water conflicts in Germany.
The authors would like to acknowledge all experts for their input. Thanks also go to our colleagues Julia Wohlfarth and Sigrid Prehofer for critically commenting on earlier versions of the manuscript, as well as to Vanessa Smith for English proofreading. Special thanks to the Daimler and Benz Foundation for making this research possible.
The authors declare that the research was conducted in the absence of any commercial or financial relationships that could be construed as a potential conflict of interest.
All claims expressed in this article are solely those of the authors and do not necessarily represent those of their affiliated organizations, or those of the publisher, the editors and the reviewers. Any product that may be evaluated in this article, or claim that may be made by its manufacturer, is not guaranteed or endorsed by the publisher.
The Supplementary material for this article can be found online at: https://www.frontiersin.org/articles/10.3389/frwa.2024.1492336/full#supplementary-material
1. ^Notably, the actual water withdrawal in Germany, in particular by agriculture, partly remains a black box.
2. ^Please note, the title of our paper refers to Peter Wack (1985): "Scenarios: Uncharted waters ahead". Harvard Business Review, Sep.
3. ^A more detailed explanation of CIB including video tutorials can be found at: https://www.cross-impact.org/english/CIB_e_Alg.htm.
4. ^https://scenariowizard.org
5. ^The scenario building process started in September 2022 and interpretation was finalized in December 2023.
6. ^The structural development of the water-intensive industry is used in our model as a proxy for the impact of the energy transition because currently fossil energy generation is responsible for a significant share of industrial water use. Therefore, the phase-out of fossil power plants will reduce the industrial demand especially for cooling water.
7. ^Allowing even more inconsistencies (IC2) results in n = 1,099 consistent scenarios, including the same 34 out of 36 variants (94.4%) and thus does not yield a better representation of the uncertainty space. As the significance threshold for inconsistencies in case of 10 descriptors is 1.5 (ICs = ½ √ (n-1)) (Weimer-Jehle, 2023, p. 50), we proceed with the resulting scenarios when allowing slight inconsistencies only (i.e., IC1).
8. ^These axes are interpreted content-wise in the next sub-section 3.3.2.
9. ^Another “twin” of this scenario, namely its version including ecosystem degradation, is the scenario with the highest attractor weight within the scenario space (for the concept of attractors in CIB cf. Weimer-Jehle, 2006).
10. ^The scenarios of cluster E group around the 5th most diverse scenario, which differs most from the extreme scenarios 1–4.
11. ^Germany currently is a net virtual water importer (Brindha, 2020). This situation might change or at least be questioned and challenged in the future.
Alcamo, J. (2008). “The SAS approach: combining qualitative and quantitative knowledge in environmental scenarios” in Environmental futures: The practice of environmental scenario analysis. Developments in integrated environmental assessment. ed. J. Alcamo (Amsterdam: Elsevier), 123–150.
Alcamo, J., Henrichs, T., Kaspar, F., Lehner, B., Rösch, T., and Siebert, S. (2003). Global estimates of water withdrawals and availability under current and future "business-as-usual" conditions. Hydrol. Sci. J. 48, 339–348. doi: 10.1623/hysj.48.3.339.45278
Alexandra, C., Wyborn, C., Roldan, C. M., and van Kerkhoff, L. (2023). “Futures-thinking: concepts, methods and capacities for adaptive governance” in Handbook on adaptive governance. ed. S. Juhola (Cheltenham: Edward Elgar Publishing), 76–98.
Amer, M., Daim, T. U., and Jetter, A. (2013). A review of scenario planning. Futures 46, 23–40. doi: 10.1016/j.futures.2012.10.003
BMUV (2023). Nationale Wasserstrategie Kabinettsbeschluss. Available at: https://www.bmuv.de/fileadmin/Daten_BMU/Download_PDF/Binnengewaesser/nationale_wasserstrategie_2023_bf.pdf (Accessed May 18, 2023).
Bonacker, T. (2008). Sozialwissenschaftliche Konflikttheorie. Wiesbaden: VS Verlag für Sozialwissenschaften.
Brindha, K. (2020). Virtual water flows, water footprint and water savings from the trade of crop and livestock products of Germany. Water Environ. J. 34, 656–668. doi: 10.1111/wej.12601
Capano, G. (2024). Policy instruments. Encycl. Pub. Policy 12, 1–6. doi: 10.1007/978-3-030-90434-0_30-1
Carlsen, H., Eriksson, E. A., Dreborg, K. H., Johansson, B., and Bodin, Ö. (2016a). Systematic exploration of scenario spaces. Foresight 18, 59–75. doi: 10.1108/FS-02-2015-0011
Carlsen, H., Lempert, R., Wikman-Svahn, P., and Schweizer, V. (2016b). Choosing small sets of policy-relevant scenarios by combining vulnerability and diversity approaches. Environ. Model. Softw. 84, 155–164. doi: 10.1016/j.envsoft.2016.06.011
Carlsen, H., Weitz, N., Petrova, K., and Kemp-Benedict, E. (2024). A sustainable trajectory for the 2030 agenda: targeting historical lock-ins to accelerate progress on all SDGs. Sustain. Dev. 32:4738, –4747. doi: 10.1002/sd.2929
Cioc, M. (2002). The Rhine: An eco-biography, 1815–2000. Seattle, WA: University of Washington Press.
Dewulf, A., and Biesbroek, R. (2018). Nine lives of uncertainty in decision-making: strategies for dealing with uncertainty in environmental governance. Polic. Soc. 37, 441–458. doi: 10.1080/14494035.2018.1504484
Dombrowsky, I. (2007). Conflict, cooperation and institutions in international water management: An economic analysis. Cheltenham: Edward Elgar.
Drakes, C., Cashman, A., Kemp-Benedict, E., and Laing, T. (2020). Global to small island: a cross-scale foresight scenario exercise. Foresight 22, 579–598. doi: 10.1108/FS-02-2020-0012
DVGW (2022). Auswirkungen des Klimawandels auf das Wasserdargebot Deutschlands. Bonn: DVGW Deutscher Verein des Gas-und Wasserfaches e. V.
DVGW (2023a). Klimafolgenstudie für das DVGW-Innovationsprogramm Zukunftsstrategie Wasser. Bonn: DVGW Deutscher Verein des Gas-und Wasserfaches e. V.
DVGW (2023b). Roadmap 2030 und vision 2100. Available at: https://www.dvgw.de/themen/forschung-und-innovation/forschungsprojekte/dvgw-forschungsprojekt-roadmap-2030
DWD (2023). Available at: https://www.dwd.de/DE/klimaumwelt/klimaatlas/klimaatlas_node.html (Accessed October 2, 2024).
EEA European Environmnet Agency (2021). Water resources across Europe —confronting water stress: an updated assessment. EEA COpenhagen.
ElSawah, S., Hamilton, S., Jakeman, A., Rothman, D., Schweizer, V., Trutnevyte, E., et al. (2020). Scenario processes for socio-environmental analysis: a review of recent efforts and a salient research agenda to support decision making. Sci. Total Environ. 729:138393. doi: 10.1016/j.scitotenv.2020.138393
Ertug, E., and Hoekstra, A. J. (2014). Water footprint scenarios for 2050: a global analysis. Environ. Int. 64, 71–82. doi: 10.1016/j.envint.2013.11.019
Feindt, P. H., and Saretzki, T. (2010). Umwelt-und Technikkonflikte. Wiesbaden: Springer Fachmedien.
Fienitz, M., and Siebert, R. (2023). Latent, collaborative, or escalated conflict? Determining causal pathways for land use conflicts. Land Use Policy 134:106918. doi: 10.1016/j.landusepol.2023.106918
Fisher, S. P., Abdi, D. I., Ludin, J., Smith, R., and Williams, S. (2000). Working with Conflict: Skills and Strategies for Action..
Folke, C., Hahn, T., Olsson, P., and Norberg, J. (2005). Adaptive governance of social-ecological systems. Annu. Rev. Environ. Resour. 30, 441–473. doi: 10.1146/annurev.energy.30.050504.144511
Forrester, J. (1958). Industrial dynamics: a major breakthrough for decision makers. Brighton, MA: Havard Business Review. (Accessed October 2, 2024).
Forrester, J. (1971). World dynamics. Available at: https://archive.org/details/worlddynamics00forr_3du
Gallopin, G. C., and Rijsberman, F. R. (2000). Three global water scenarios. Int. J. Water 1, 16–40. doi: 10.1504/IJW.2000.002055
Garcia-Teruel, A., Scholz, Y., Weimer-Jehle, W., Prehofer, S., Cao, K. K., and Borggrefe, F. (2022). Teaching power-sector models social and political awareness. Energies 15, 1–24. doi: 10.3390/en15093275
Gausemeier, J., Fink, A., and Schlake, O. (1998). Scenario management: an approach to develop future potentials. Technol. Forecast. Soc. Chang. 59:111. doi: 10.1016/S0040-1625(97)00166-2
Girod, B., Wiek, A., Mieg, H., and Hulme, M. (2009). The evolution of the IPCC’s emissions scenarios. Environ. Sci. Pol. 12, 103–118. doi: 10.1016/j.envsci.2008.12.006
Grunwald, A. (2011). Energy futures: diversity and the need for assessment. Futures 43, 820–830. doi: 10.1016/j.futures.2011.05.024
Guivarch, C., Lempert, R., and Trutnevyte, E. (2017). Scenario techniques for energy and environmental research: an overview of recent developments to broaden the capacity to deal with complexity and uncertainty. Environ. Model Softw. 97, 201–210. doi: 10.1016/j.envsoft.2017.07.017
Head, B. W. (2022). “The rise of ‘wicked problems’—uncertainty, complexity and divergence” in Wicked problems in public policy. ed. B. W. Head (Cham: Palgrave Macmillan), 21–36.
Hinkel, J. (2008). Transdisciplinary knowledge integration: Cases from integrated assessment and vulnerability assessment. (dissertation). Available at: https://www.researchgate.net/publication/40099835_Transdisciplinary_knowledge_integration_cases_from_integrated_assessment_and_vulnerability_assessment (Accessed October 2, 2024).
Huss, W., and Honton, E. (1987). Alternative methods for developing business scenarios. Technol. Forecast Soc. Change 31, 219–238. doi: 10.1016/0040-1625(87)90012-6
IPCC. (2018). Special report global warming of 1.5°C. Available at: https://www.ipcc.ch/sr15/download/ (Accessed October 2, 2024).
Joeres, A., Steeger, G., Huth, K., Jacobsen, M., and Donheiser, M. (2022). Ausgetrocknet, Deutschland kämpft um Wasser. Correctiv.org. Online available under. Available at: https://correctiv.org/aktuelles/klimawandel/2022/06/14/klimawandel-konflikt-um-wasser-in-deutschland/?lang=de, (accessed August 14, 2024).
Kemp-Benedict, E. (2012). Telling better stories: strengthening the story in story and simulation. Environ. Res. Lett. 7:1004. doi: 10.1088/1748-9326/7/4/041004
Kemp-Benedict, E., de Jong, W., and Pacheco, P. (2014). “Forest futures: linking global paths to local conditions” in Forest under pressure - local responses to global issues. Part IV-possible future pathways. eds. G. Katila, P. Jong, and G. Mery (Stockholm: IUFRO World Series).
Kirschke, S., Franke, C., Newig, J., and Borchardt, D. (2019). Clusters of water governance problems and their effects on policy delivery. Polic. Soc. 38, 255–277. doi: 10.1080/14494035.2019.1586081
Kosow, H. (2015). New outlooks in traceability and consistency of integrated scenarios. Eur. J. Futures Res. 3, 1–12. doi: 10.1007/s40309-015-0077-6
Kosow, H., Brauner, S., Hölzlberger, F., Moschner, J., Vögele, S., and Weimer-Jehle, W. (2023). “Future water conflicts in Germany: serious gaming for policy design under future uncertainty” in 6th International Conference on Public Policy ICPP6, Toronto, Canada.
Kosow, H., and Gaßner, R. (2008). Methods of future and scenario analysis. Overview, assessment, and selection criteria. Bonn: Deutsches Institut für Entwicklungspolitik gGmbH. Available at: https://www.ssoar.info/ssoar/handle/document/19366
Kosow, H., Kirschke, S., Borchard, D., Cullmann, J., Guillaume, J. H. A., Hannah, D. M., et al. (2022a). Scenarios of water extremes - framing ways forward for wicked problems. Hydrol. Process. 36:14492. doi: 10.1002/hyp.14492
Kosow, H., Wassermann, S., Bartke, S., Goede, P., Imbert, I., Grimski, D., et al. (2022c). Addressing goal conflicts: new policy mixes for commercial land use management. Land 11:795. doi: 10.3390/land11060795
Kosow, H., Weimer-Jehle, W., Leon, C., and Minn, F. (2022b). Designing synergetic and sustainable policy mixes - a methodology to address conflictive environmental issues. Environ. Sci. Pol. 130, 36–46. doi: 10.1016/j.envsci.2022.01.007
LAWA (2017). Auswirkungen des Klimawandels auf die Wasserwirtschaft–Bestandsaufnahme, Handlungsoptionen und strategische Handlungsfelder. Bonn: Bund/Länder-Arbeitsgemeinschaft Wasser (LAWA).
LAWA (2022). Positionspapier zum Umgang mit Zielkonflikten bei der Anpassung der Wasserwirtschaft an den Klimawandel. Bonn: Bund/Länder-Arbeitsgemeinschaft Wasser (LAWA).
Lazurko, A., Kearney, N., Siddhantakar, N., Kurniawan, J. H., and Schweizer, V. (2022). “Weaknesses in corporate commitments to climate change adaptation and how to fix them: a systemic scenario assessment approach” in Business and policy solutions to climate change. Cham. eds. T. Walker, S. Wendt, S. Goubran, and T. Schwartz (Cham: Springer International Publishing), 115–137.
Lazurko, A., Schweizer, V., and Armitage, D. (2023). Exploring “big picture” scenarios for resilience in social–ecological systems - transdisciplinary cross-impact balances modeling in the Red River Basin. Sustain. Sci. 18, 1773–1794. doi: 10.1007/s11625-023-01308-1
Leipprand, A., Dworak, T., Benzle, M., Berglund, M., Kadner, S., Hattermann, F., et al. (2008). Impacts of climate change on water resources - adaption strategies for Europe. Dessau-Roßlau: Umweltbundesamt.
Lloyd, E. A., and Schweizer, V. J. (2014). Objectivity and a comparison of methodological scenario approaches for climate change research. Synthese 191, 2049–2088. doi: 10.1007/s11229-013-0353-6
Mack, L., Andersen, H. E., Beklioğlu, M., Bucak, T., Couture, R., Cremona, F., et al. (2019). The future depends on what we do today - projecting Europe's surface water quality into three different future scenarios. Sci. Total Environ. 668, 470–484. doi: 10.1016/j.scitotenv.2019.02.251
Mier, M., Siala, K., Govorukha, K., and Mayer, P. (2023). Collaboration, decarbonization, and distributional effects. Appl. Energy 341:121050. doi: 10.1016/j.apenergy.2023.121050
Newig, J., and Moss, T. (2010). Multi-level water governance and problems of scale: setting the stage for a broader debate. Environ. Manag. 46, 1–6. doi: 10.1007/s00267-010-9531-1
OECD (2012). “Chapter 5: water” in OECD environmental outlook to 2050- the consequences of inaction. eds. M. Leflaive, M. Witmer, B. Roberto, K. Marloes, and B. Tom (Paris: OECD), 207–273.
Pahl-Wostl, C., Knieper, C., Lukat, E., Meergans, F., Schoderer, M., Schütze, N., et al. (2020). Enhancing the capacity of water governance to deal with complex management challenges: a framework of analysis. Environ. Sci. Pol. 107, 23–35. doi: 10.1016/j.envsci.2020.02.011
Pregger, T., Naegler, T., Weimer-Jehle, W., Prehofer, S., and Hauser, W. (2020). Moving towards socio-technical scenarios of the German energy transition - lessons learned from integrated energy scenario building. Clim. Chang. 162, 1743–1762. doi: 10.1007/s10584-019-02598-0
Prehofer, S., Kosow, H., Naegler, T., Pregger, T., Vögele, S., and Weimer-Jehle, W. (2021). Linking qualitative scenarios with quantitative energy models. Knowledge integration in different methodological designs. Energy Sustain. Soc. 11, 1–20. doi: 10.1186/s13705-021-00298-1
Razavi, S., Duffy, A., and Eamen, L. (2024). Convergent and transdisciplinary integration: on the future of integrated modeling of human-water systems. ESS Open Archive. doi: 10.22541/essoar.171829793.30234109/v1
Renn, O., Jager, A., Deuschle, J., and Weimer-Jehle, W. (2009). A normative-functional concept of sustainability and its indicators. Int. J. Glob. Environ. Issues 9, 291–317. doi: 10.1504/IJGENVI.2009.027260
Ruth, M., Özgüna, O., Wachsmuth, J., and Gößling-Reisemann, S. (2015). Dynamics of energy transitions under changing socioeconomic, technological and climate conditions in Northwest Germany. Ecol. Econ. 111, 29–47. doi: 10.1016/j.ecolecon.2014.12.025
Schuch, E., Apergi, M., Kuen, C. Y., Eicke, L., Goldthau, A., Kurniawan, J. H., et al. (2024). Breaking the carbon lock-in: identifying pathways for Malaysia towards a low-carbon future. Technol. Forecasting Soc. Change 202:123331. doi: 10.1016/j.techfore.2024.123331
Schütze, M., Seidel, J., Chamorro, A., and León, C. (2019). Integrated modelling of a megacity water system – the application of a transdisciplinary approach to the Lima metropolitan area. J. Hydrol. 573, 983–993. doi: 10.1016/j.jhydrol.2018.03.045
Schweizer, V. J. (2020). Reflections on cross-impact balances, a systematic method constructing global socio-technical scenarios for climate change research. Clim. Chang. 162, 1705–1722. doi: 10.1007/s10584-019-02615-2
Schweizer, V. J., and Kriegler, E. (2012). Improving environmental change research with systematic techniques for qualitative scenarios. Environ. Res. Lett. 7:044011. doi: 10.1088/1748-9326/7/4/044011
Schweizer, V. J., and O’Neill, B. C. (2014). Systematic construction of global socioeconomic pathways using internally consistent element combinations. Clim. Chang. 122, 431–445. doi: 10.1007/s10584-013-0908-z
Scoones, I., and Stirling, A. (2020). The politics of uncertainty: Challenges of transformation. New York, NY: Taylor and Francis.
Shaochun, H., Krysanova, V., Österle, H., and Hattermann, F. (2010). Simulation of spatiotemporal dynamics of water fluxes in Germany under climate change. Hydrol. Process. 24, 3289–3306. doi: 10.1002/hyp.7753
Sivakumar, B. (2011). Water crisis: from conflict to cooperation—an overview. Hydrol. Sci. J. 56, 531–552. doi: 10.1080/02626667.2011.580747
Sneddon, C., Harris, L., Dimitrov, R., and Özesmi, U. (2002). Contested waters: conflict, scale, and sustainability in aquatic socioecological systems. Soc. Nat. Resour. 15, 663–675. doi: 10.1080/08941920290069272
Stein, U., Tröltzsch, J., Vidaurre, R., Schritt, H., Bueb, B., Reineke, J., et al. (2024). Auswirkung des Klimawandels auf die Wasserverfügbarkeit − Anpassung an Trockenheit und Dürre in Deutschland (WADKlim). Abschlussbericht. Umweltbundesamt: Dessau-Roßlau. UBA TEXTE 143/2024. Available at: https://www.umweltbundesamt.de/sites/default/files/medien/11850/publikationen/143_2024_texte_wadklim.pdf (Accessed November 11, 2024).
Tietje, O. (2005). Identification of a small reliable and efficient set of consistent scenarios. Eur. J. Oper. Res. 162, 418–432. doi: 10.1016/j.ejor.2003.08.054
Tori, S., te Boveldt, G., and Keseru, I. (2023). Building scenarios for urban mobility in 2030: the combination of cross-impact balance analysis with participatory stakeholder workshops. Futures 150:103160. doi: 10.1016/j.futures.2023.103160
Tröltzsch, J., Stein, U., Vidaurre, R., Bueb, B., Schritt, H., Flörke, M., et al. (2021). Auswirkung des Klimawandels auf die Wasserverfügbarkeit-Anpassung an Trockenheit und Dürre in Deutschland. WasserWirtschaft 111, 44–48. doi: 10.1007/s35147-021-0911-7
United Nations, The United Nations World Water Development Report 2022 (UN WWDR). (2022). Groundwater. UNESCO, Paris: Making the invisible visible.
Vester, F. (2002). Die Kunst, Vernetzt zu Denken. Available at: https://archive.org/details/diekunstvernetzt0000vest (Accessed October 2, 2024).
Vögele, S., Hansen, P., Poganietz, W.-R., Prehofer, S., and Weimer-Jehle, W. (2017). Scenarios for energy consumption of private households in Germany using a multi-level cross-impact balance approach. Energy 120, 937–946. doi: 10.1016/j.energy.2016.12.001
Vögele, S., Poganietz, W.-R., Kleinebrahm, M., Weimer-Jehle, W., Bernhard, J., Kuckshinrichs, W., et al. (2022). Dissemination of PV-battery Systems in the German Residential Sector up to 2050: technological diffusion from multidisciplinary perspectives. Energy 248:123477. doi: 10.1016/j.energy.2022.123477
Walker, W. E., Harremoës, P., Rotmans, J., van der Sluijs, J. P., van Asselt, M. B. A., Janssen, P., et al. (2003). Defining uncertainty: a conceptual basis for uncertainty Management in Model-Based Decision Support. Integr. Assess. 4, 5–17. doi: 10.1076/iaij.4.1.5.16466
Weimer-Jehle, W. (2006). Cross-impact balances: a system-theoretical approach to cross-impact analysis. Technol. Forecast. Soc. Chang. 73, 334–361. doi: 10.1016/j.techfore.2005.06.005
Weimer-Jehle, W. (2023). Cross-impact balances (CIB) for scenario analysis-fundamentals and implementation. Cham: Springer. doi: 10.1007/978-3-031-27230-1
Weimer-Jehle, W., Buchgeister, J., Hauser, W., Kosow, H., Naegler, T., Poganietz, W., et al. (2016). Context scenarios and their usage for the construction of socio-technical energy scenarios. Energy 111, 956–970. doi: 10.1016/j.energy.2016.05.073
Weimer-Jehle, W., Vögele, S., Hauser, W., Kosow, H., Poganietz, W.-P., and Prehofer, S. (2020). Socio-technical energy scenarios: state of the art and CIB-based approaches. Climatic Change Int. Scenario Building Energ. Transit. Res. 162, 1723–1741. doi: 10.1007/s10584-020-02680-y
Wilson, I. (1998). “Mental maps of the future. An intuitive logics approach to scenario planning” in Learning from the future: Competitive foresight scenarios. eds. L. Fahey and R. M. Randall (Hoboken, NJ: John Wiley and Sons), 81–108.
Keywords: water, scenarios, uncertainty, cross-impact balances (CIB), conflict assessment, complexity, year 2050, Germany
Citation: Kosow H, Brauner S, Brumme A, Hauser W, Hölzlberger F, Moschner J, Rübbelke D, Vögele S and Weimer-Jehle W (2024) Uncharted water conflicts ahead: mapping the scenario space for Germany in the year 2050. Front. Water. 6:1492336. doi: 10.3389/frwa.2024.1492336
Received: 06 September 2024; Accepted: 04 November 2024;
Published: 20 November 2024.
Edited by:
Shinichiro Nakamura, Nagoya University, JapanReviewed by:
Aviram Sharma, University of Vigo, SpainCopyright © 2024 Kosow, Brauner, Brumme, Hauser, Hölzlberger, Moschner, Rübbelke, Vögele and Weimer-Jehle. This is an open-access article distributed under the terms of the Creative Commons Attribution License (CC BY). The use, distribution or reproduction in other forums is permitted, provided the original author(s) and the copyright owner(s) are credited and that the original publication in this journal is cited, in accordance with accepted academic practice. No use, distribution or reproduction is permitted which does not comply with these terms.
*Correspondence: Hannah Kosow, aGFubmFoLmtvc293QHppcml1cy51bmktc3R1dHRnYXJ0LmRl
Disclaimer: All claims expressed in this article are solely those of the authors and do not necessarily represent those of their affiliated organizations, or those of the publisher, the editors and the reviewers. Any product that may be evaluated in this article or claim that may be made by its manufacturer is not guaranteed or endorsed by the publisher.
Research integrity at Frontiers
Learn more about the work of our research integrity team to safeguard the quality of each article we publish.