- 1ENDS Report, Haymarket Media Group, Twickenham, United Kingdom
- 2Department of Natural Science, Manchester Metropolitan University, Manchester, United Kingdom
- 3Chemistry Matters, Calgary, AB, Canada
Landfills are a known source of PFAS pollution. Many have environmental permits allowing the discharge of treated leachate to controlled waters. In this article we compared leachate data for 17 PFAS from 17 different landfill sites across the UK. The results show that the landfill leachate treatment process (designed to improve water quality) is generating the banned PFAS; PFOA and PFOS. Approximately 80% of locations tested showed an increase in PFOS, with an increase of 1,335% in one sample. The highest concentrations of PFOS and PFOA in treated leachate were 2,460 ng L−1 and 26,900 ng L−1, respectively. When compared against the environmental quality standard of 0.65 ng L−1 for PFOS this leachate could pose a significant concern. Landfill leachate treatment has proven effective for a wide range of different pollutants, but this research shows in several instances that it is not appropriate for PFAS remediation.
1 Introduction
Per- and polyfluoroalkyl substances (PFAS) are persistent environmental pollutants of global concern. Perfluorooctanoic acid (PFOA) and perfluorooctanesulfonic acid (PFOS) were the first two PFAS to be listed under the Stockholm convention (Secretariat of the Stockholm Convention (UNEP), 2008). There is currently widespread contamination of PFOS and PFOA which is impacting the marine environment, freshwater environments and drinking water (Kurwadkar et al., 2022). PFOS and PFOA are not readily removed by conventional drinking or waste-water treatment processes, and growing evidence has shown that PFOA and PFOS can be generated from precursor compounds in chemical and biological processes. Xiao et al. (2012) identified a significant increase (up to 1,200%) in the wastewater concentrations of perfluorohexanoic acid (PFHxA) and PFOA at 22 out of 37 wastewater treatment plants. Similar increases have also been observed in other biological wastewater treatment plants (Guo et al., 2010; Schultz et al., 2006; Takagi et al., 2011; Xiao et al., 2012), and during the drinking-water disinfection processes (Appleman et al., 2014; Boiteux et al., 2017; Dauchy et al., 2012). Increases of 18–77% of the mass of perfluoroalkyl carboxylic acids PFCAs, including PFOA, have been reported after disinfection of ozone or chlorine. The authors concluded that this was caused by the transformation of unidentified precursors in surface water and other legacy precursor compounds (Boiteux et al., 2017).
These increases in PFAAs have been identified in a wide number of different treatment processes all over the globe. A survey of 15 U.S. water treatment plants, identified that the concentration of PFOA and PFOS in water was consistently higher after chemical disinfection treatments (Appleman et al., 2014). Similarly, increases in PFOA and PFOS concentrations in drinking-water processes have been reported in Japan, which was attributed to the transformation of precursor compounds (Takagi et al., 2011). The extremely strong carbon-fluorine bond makes PFAS inherently persistent to the biological treatment, with studies showing that the biological landfill leachate treatment process also resulted in elevated total PFAS concentrations in leachate after aeration (Fuertes et al., 2017; Solo-Gabriele et al., 2020). This indicates biotransformation of precursor compounds [such as fluorotelomer alcohols (FTOHs), fluorotelomer sulfonates (FTSs) and polyfluoroalkyl phosphate esters (PAPs)] in leachate to produce perfluoroalkyl acids (PFAAs) (Liu et al., 2021).
While this process has been studied in some global regions, to the best of our knowledge it has never been investigated or reported in the UK. The UK has a long industrial history where a large proportion of chemical waste was disposed of in landfill sites. Many of these are historic landfills, where, according to the Environment Agency definition, there is no pollution prevention and control permit or waste management license currently in force (Gov.UK, 2024). Climate change is resulting in more severe weather events and as a result the UK is experiencing increased frequency and severity of rainfall and coastal erosion. This is already putting pressure on these landfill sites, with nearly one in ten historic coastal landfill sites in England already at risk of erosion (Brand et al., 2018). Many of these historic landfill sites are also located within drinking water protection zones (Brand and Spencer, 2023) and so it is imperative that we understand the potential risk of PFAS from our industrial legacy.
Once a landfill operator in the UK has treated the leachate, they are legally allowed to discharge their waste directly into controlled waters via a Discharge to Water permit, this includes hazardous landfills (Gov.UK, 2024). Given that the treatment process has the potential to generate banned PFAS it is imperative we understand these risks further.
2 Methodology
In this study we evaluated leachate data from 17 landfill locations across the UK to identify if they are likely to be a significant source of PFAS to the environment. All data obtained for this report was obtained by an ENDS Report Freedom of Information Request. The data was collected by third-party contractors and formed part of a UK government analysis into landfill leachate for PFAS as part of a wider DEFRA-funded research project on persistent organic pollutants (Environment Agency, 2021). The data collected came from a range of operational, closed and historical landfills in England, they are predominantly classified as non-hazardous, accepting municipal solid wastes. All samples were collected between 2021–2022. The locations of the landfill sites and sample collection dates were not provided, we were informed this was because the contractors provided the Agency with an anonymized dataset. Where on-site leachate treatment facilities were available, raw and treated leachate (final effluent) samples were collected. The treatment facilities used biological, chemical, and physical technologies, the most commonly used in the UK landfill industry is biological sequence batch reactors (SBR) with alkaline-dosing. As the data set was anonymized we were unable to identify which technology was used at each location.
In total we obtained results for 48 samples. This included 32 raw samples and 16 treated samples from 17 different landfill sites, plus three blank samples. Duplicate samples were obtained from 4 locations (Landfills 1, 2, 6, & 11). Samples were prepared using solid phase extraction and quantified using isotope labeled internal standards, with analysis by HPLC-MS/MS (high pressure liquid chromatography coupled to a triple quadrupole mass spectrometer). Results were reported for 17 PFAS (perfluorooctyl sulphonate (PFOS), perfluorooctanoic acid (PFOA), 5: 3 fluorotelomer carboxylic acid (5, 3 FTCA), 6: 2 fluorotelomer sulphonate (6, 2 FTSA), 8: 2 fluorotelomer alcohol (8, 2 FTOH), perfluoropentyl sulphonate (PFPeS), perfluoroheptyl sulphonate (PFHpS), perfluoropentanoic acid (PFPA), perfluoroheptanoic acid (PFHpA), hexafluoropropylene oxide-dimer acid (HFPO-DA) (Gen-X), F53B (two components), perfluorobutyl sulphonate (PFBS), perfluorobutanoic acid (PFBA), perfluorononanoic acid (PFNA), perfluorohexanoic acid (PFHxA), perfluorohexyl sulphonate (PFHxS), perfluoroethylcyclohexane sulphonate (PFECHS)) with limits of detection of 0.065 ng L−1 for PFOS and PFOA. Data is presented in the Supplementary information.
As the data was obtained from a variety of different landfill sites, we urge caution comparing data between different locations. However, in this study we are focused on identifying overall trends and identifying relative trends in raw and treated leachate. These paired raw and treated samples were analyzed at the same time which facilitates this type of data analysis.
3 Results and discussion
Concentrations of all 17 PFAS in the raw leachate ranged from 1 ng L−1 to 87,100 ng L−1 (Table 1). The highest concentration for any PFAS in a treated sample was for perfluorobutyl sulphonate (PFBS), which was detected at a concentration of 44,800 ng L−1. PFOS concentrations in the raw leachate samples ranged from 16–320 ng L−1 and 15.3–2,460 ng L−1 in the treated samples. These concentrations greatly exceed the current environmental quality standards (EQSs) for PFOS. The annual average EQS for inland surface waters is 0.65 ng L−1 and the annual average EQS for other surface waters is 0.13 ng L−1 (Environment Agency, 2019). The EU Water Framework Directive applies an EQS of 0.65 ng L−1 for PFOS in (inland) surface waters (European Union, 2013), with a threshold of 4.4 ng L−1 proposed for a group of 24 PFAS in surface and groundwater (European Environmental Bureau, 2023). In England, the Drinking Water Inspectorate’s current guidance states that in England the acceptable levels of the two most common types of PFAS - PFOS and PFOA - is 100 ng L−1. In the U.S. the proposed legal limit is 0.004 ng L−1 for PFOA and 0.0 2 ng L−1 for PFOS. There are currently no guidelines for other PFAS chemicals. There is currently no guidance in England on PFBS, although animal studies following oral exposure to PFBS have shown health effects on the thyroid, reproductive organs and tissues, developing fetus, and kidney (U.S. Environmental Protection Agency, 2024).
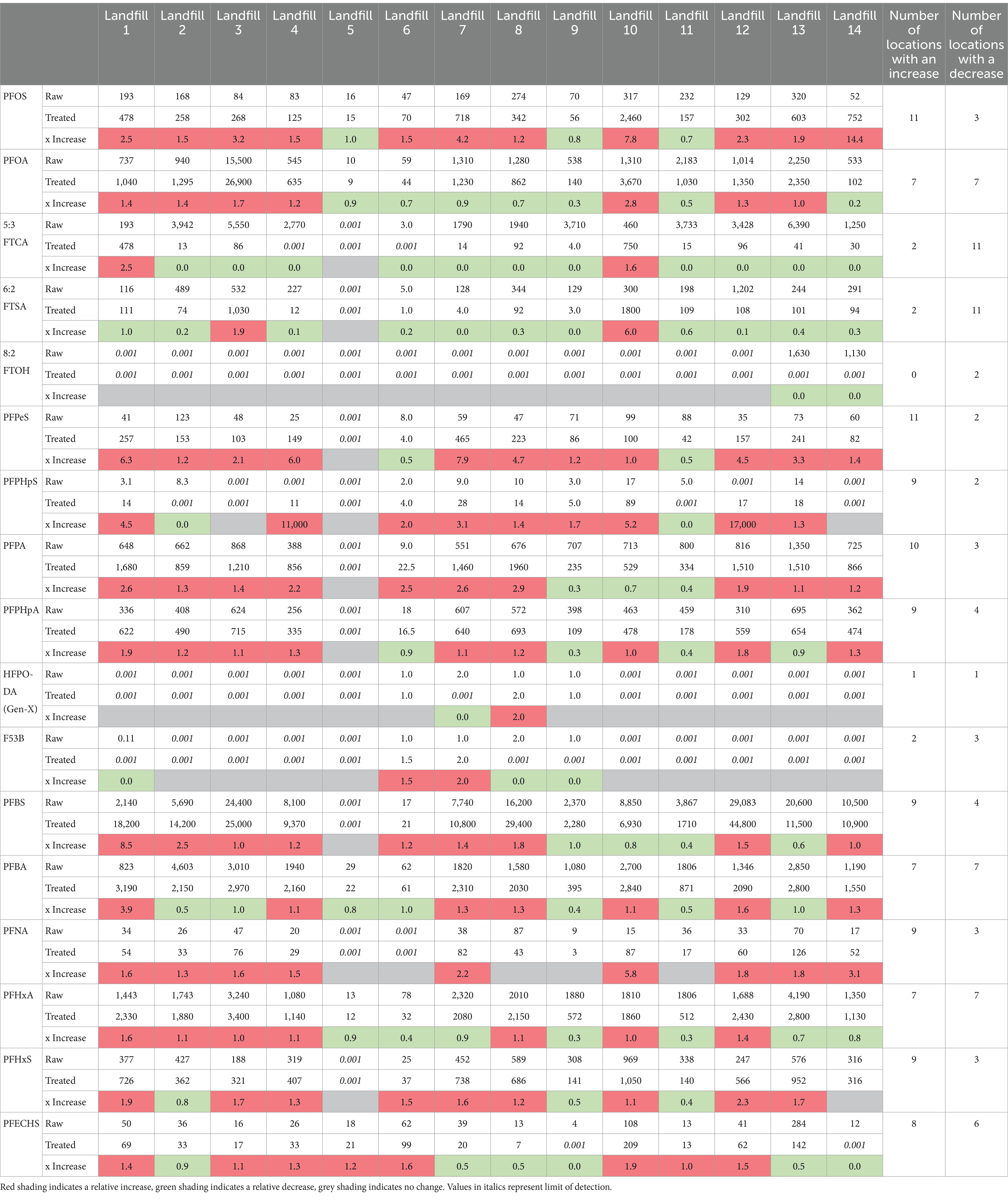
Table 1. Average concentrations and relative difference of the 17 PFAS in raw and treated leachate samples across the 14 paired locations.
Data was reported for 17 Landfill sites, but only 14 locations had data from both raw and treated leachate. This paired data was used to provide a broad assessment of the effectiveness of 14 sites to reduce PFAS concentrations. Only one location (landfill 11) displayed lower concentrations of every PFAS in the treated samples relative to the raw samples. The majority of locations displayed a relative increase in straight chain PFAAs, and a relative decrease in branched PFAS. There were more instances where higher concentrations of PFAS were detected in treated leachate than raw leachate for PFOS, PFPeS, PFPHpS, PFPA, PFPHpA, PFBS, PFNA, PFHxS, and PFECHS. The branched PFAS 5: 3FTCA, 6: 2FTCA and 8: 2FTOH were the only PFAS with more locations displaying a relative decrease in concentrations (Table 1). These results indicate that relative increases in straight chain PFAAs is likely due to precursor transformation during the treatment process (Fuertes et al., 2017; Solo-Gabriele et al., 2020; Liu et al., 2021). This transformation can occur slowly in the environment but appears to be drastically accelerated during treatment at some locations.
When the data is investigated it reveals many instances where the treatment process appears to be increasing the concentrations of the banned PFAS (PFOA and PFOS) (Table 1). Eleven (11) of the 14 paired samples displayed a higher concentration of PFOS in the treated leachate than the raw leachate (Figure 1). In 9 of these samples the PFOS concentrations increased by over 50% and in 6 of the samples the PFOS concentrations increased by over 100%. A paired T-test revealed concentrations of PFOS were significantly higher in the paired treated samples. The results reveal some of the highest ever reported relative potential increases in PFOS concentrations. The concentration in Landfill 14 increased by 1,335%, from 52.4 ng L−1 to 752 ng L−1, and in Landfill 10 by 676%, from 317 ng L−1 to 2,460 ng L−1 (Figure 1).
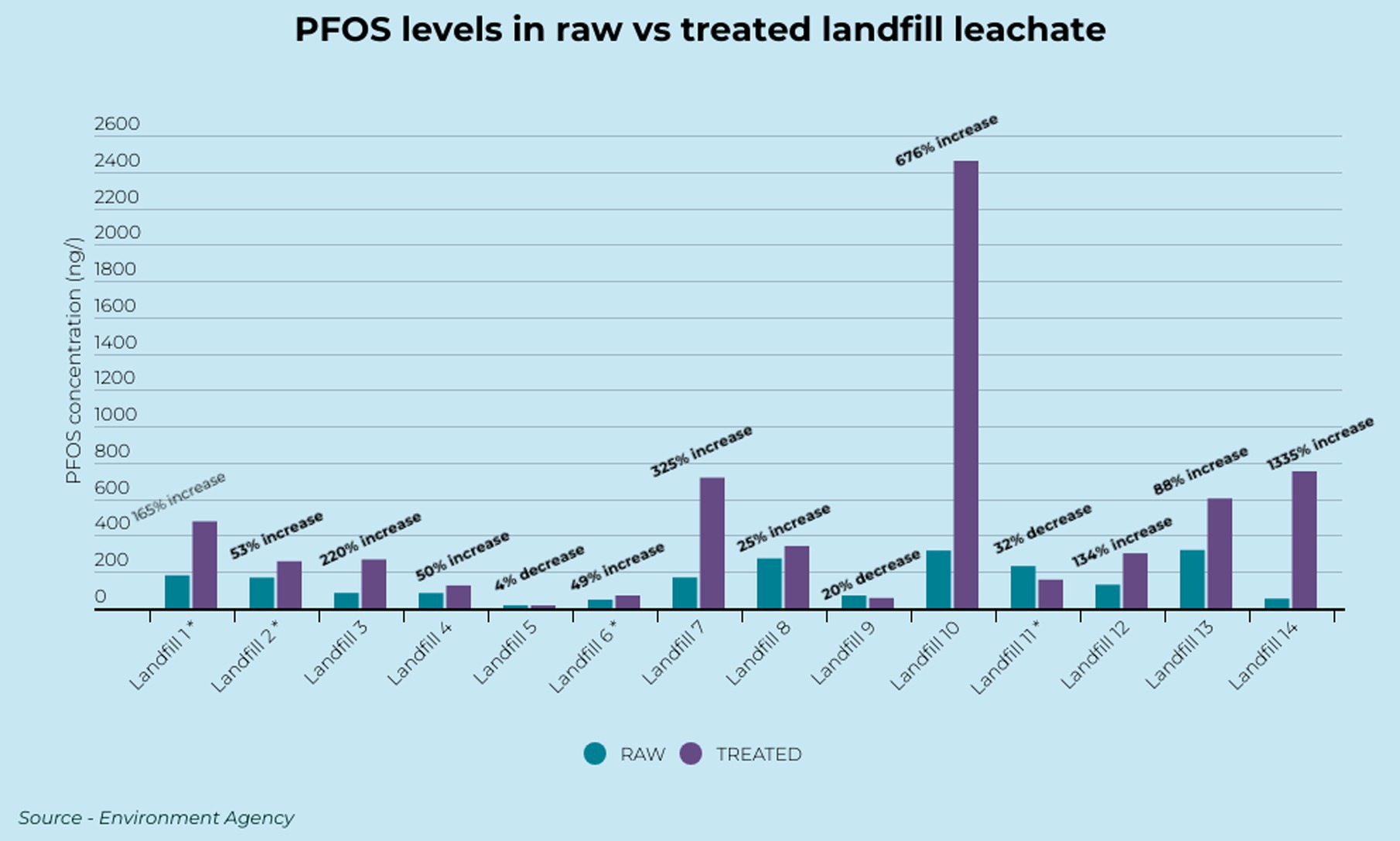
Figure 1. PFOS concentrations in raw and treated landfill leachate. *Represents average value for landfills with multiple treated or raw samples.
Seven (7) of the 14 paired samples displayed a higher concentration of PFOA in the treated leachate than the raw leachate (Figure 2). In six (6) of these samples the PFOA concentrations increased by over 50% and in two (2) of the samples the PFOA concentrations increased by over 100%. The highest relative increases were observed in Landfill 10 which displayed a potential increase of 180% from 1,310 ng L−1 in the raw sample to 3,670 ng L−1 in the treated sample (Figure 2). Despite these cases concentrations of PFOA in treated samples were not statistically significantly higher in the paired samples.
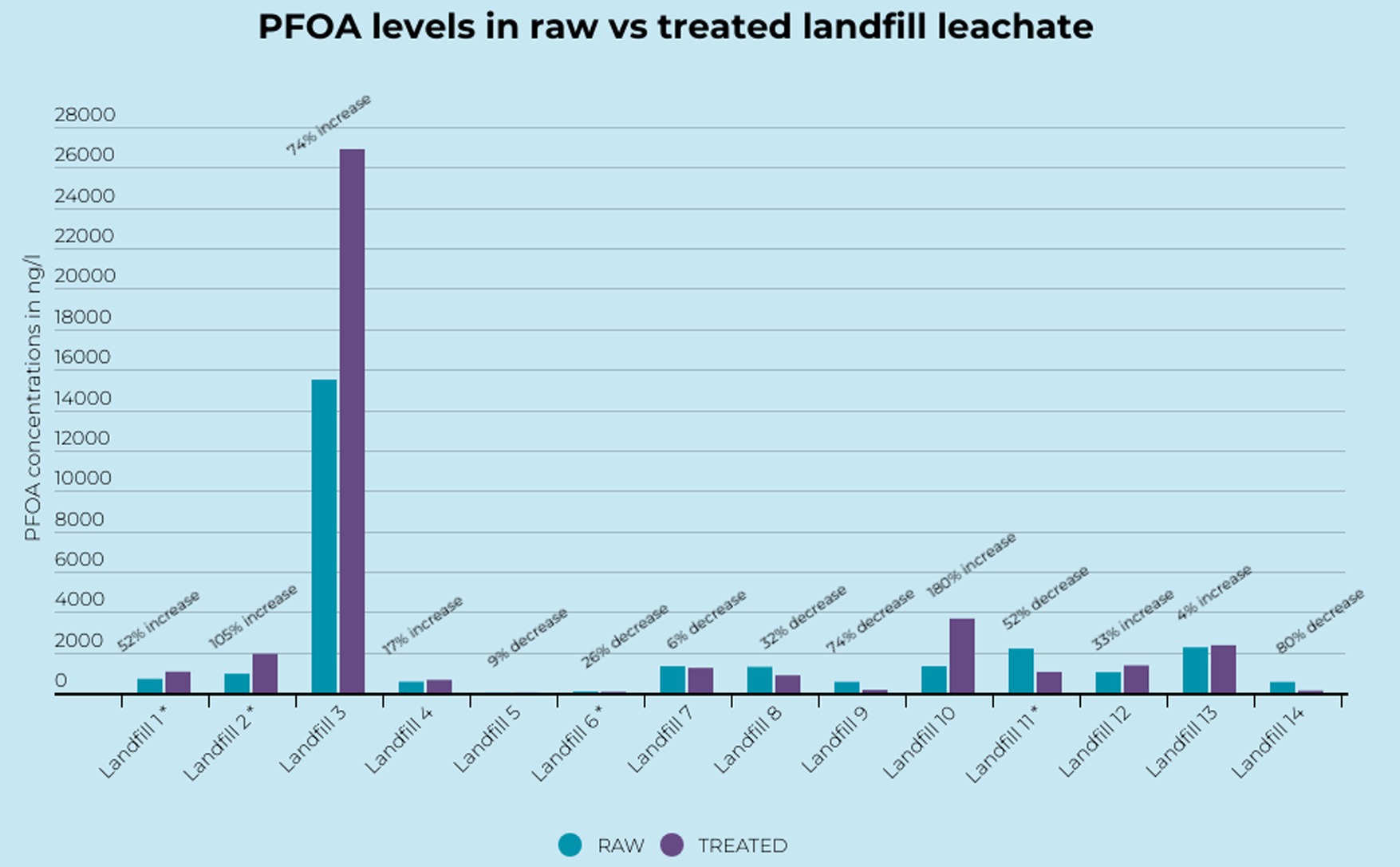
Figure 2. PFOA concentrations in raw and treated landfill leachate. *Represents average value for landfills with multiple treated or raw samples.
Relative increases in the straight chain PFAAs could not be explained by relative decreased in the 3 branched PFAS alone. As only targeted analysis was performed on the data it was not possible to ascertain what other PFAS were present in the raw leachate, or what degradation products are being generated from the treatment process. These results indicate that there are likely to be many more PFAS in these samples and so further analysis using total PFAS methods and non-targeted analysis would be recommended. Wastewater treatment plants can be effective at improving water quality for a wide variety of pollutants. However, our snapshot of data from UK treatment plants indicates that they are not effective at removing PFAS, and in many instances are generating the banned PFAS (PFOA and PFOS) through precursor transformation. This data was gathered from a targeted monitoring program however, future monitoring efforts would benefit from complimentary non-targeted and total PFAS methods to gain a more complete picture of what is entering our waterways.
4 Conclusion
The results of our study paint a concerning picture for the effectiveness of current efforts to treat PFAS in landfill leachate. As the locations of the landfill sites were not disclosed it is not possible to comment on the direct impact to the environment. However, the highest concentrations of PFOS and PFOA in treated leachate were 2,460 ng L−1 and 26,900 ng L−1, respectively. When comparing these against the environmental quality standard of 0.65 ng L−1 for PFOS in inland waters, it would indicate that if this treated leachate was to be discharged directly to the environment it would pose a significant concern. It was disappointing that spatial and temporal data was not collected by subcontractors (or was removed and anonymized) as this limits what could be interpreted from the dataset. Further monitoring would be strongly recommended to compare the performance of different treatment types, and assess seasonal variation. We hope this manuscript will prompt further research of this nature. This will enable the development and use of the most appropriate technologies for leachate treatment. This information will help support the development of more appropriate monitoring programs and policies, both in the UK and globally.
PFOS and PFOA are listed under the Stockholm convention under Annex B (Restriction) Annex A (elimination) respectively. They pose a significant risk to the environment and PFOA is a class one carcinogen and PFOS is possibly carcinogenic to humans (Group 2B). This study shows that there is an urgent need for further investigation and to upgrade inappropriate leachate treatment works in the UK. In several cases the technology that is designed to protect humans and the environment appears to be doing the opposite and is actively creating considerable amounts of PFOS and PFOA.
Data availability statement
The original contributions presented in the study are included in the article/Supplementary material, further inquiries can be directed to the corresponding author.
Author contributions
PN: Conceptualization, Data curation, Formal analysis, Investigation, Visualization, Writing – original draft, Writing – review & editing. DM: Formal analysis, Writing – original draft, Writing – review & editing.
Funding
The author(s) declare that no financial support was received for the research, authorship, and/or publication of this article.
Conflict of interest
PN was employed by Haymarket Media Group. DM was employed by Chemistry Matters.
Publisher’s note
All claims expressed in this article are solely those of the authors and do not necessarily represent those of their affiliated organizations, or those of the publisher, the editors and the reviewers. Any product that may be evaluated in this article, or claim that may be made by its manufacturer, is not guaranteed or endorsed by the publisher.
Supplementary material
The Supplementary material for this article can be found online at: https://www.frontiersin.org/articles/10.3389/frwa.2024.1480241/full#supplementary-material
References
Appleman, T. D., Higgins, C. P., Quiñones, O., Vanderford, B. J., Kolstad, C., Zeigler-Holady, J. C., et al. (2014). Treatment of poly- and perfluoroalkyl substances in U.S. full-scale water treatment systems. Water Res. 51, 246–255. doi: 10.1016/j.watres.2013.10.067
Boiteux, V., Dauchy, X., Bach, C., Colin, A., Hemard, J., Sagres, V., et al. (2017). Concentrations and patterns of perfluoroalkyl and polyfluoroalkyl substances in a river and three drinking water treatment plants near and far from a major production source. Sci. Total Environ. 583, 393–400. doi: 10.1016/j.scitotenv.2017.01.079
Brand, J. H., and Spencer, K. L. (2023). Potential pollution risks of historic landfills in England: further analysis of climate change impacts. Wires Water 11:e1706. doi: 10.1002/wat2.1706
Brand, J. H., Spencer, K. L., O'shea, F. T., and Lindsay, J. E. (2018). Potential pollution risks of historic landfills on low-lying coasts and estuaries. Wires Water 5:e1264. doi: 10.1002/wat2.1264
Dauchy, X., Boiteux, V., Rosin, C., and Munoz, J.-F. (2012). Relationship between industrial discharges and contamination of raw water resources by Perfluorinated compounds: part ii: case study of a Fluorotelomer polymer manufacturing plant. Bull. Environ. Contam. Toxicol. 89, 531–536. doi: 10.1007/s00128-012-0705-9
Environment Agency (2019). Perfluorooctane sulfonate (Pfos) and related substances: Sources, pathways and environmental data. Environment Agency.
Environment Agency (2021). Poly- and perfluoroalkyl substances (Pfas): sources, pathways and environmental data. Chief Scientist’s Group Report 2021:5. doi: 10.1016/j.fos.2021.03.013
European Environmental Bureau (2023). Policy briefing: Toxic tide rising: Time to tackle Pfas. National approaches to address Pfas in drinking water across Europe. Environment Agency.
European Union. (2013). Directive 2013/39/Eu of the European Parliament and of the council of 12 august 2013. European Environmental Bureau.
Fuertes, I., Gómez-Lavín, S., Elizalde, M. P., and Urtiaga, A. (2017). Perfluorinated alkyl substances (Pfass) in northern Spain municipal solid waste landfill leachates. Chemosphere 168, 399–407. doi: 10.1016/j.chemosphere.2016.10.072
Gov.UK. (2024). Landfill operators: environmental permits. Avilable at: https://www.gov.uk/guidance/landfill-operators-environmental-permits/manage-leachate (Accessed 11 April, 2024).
Guo, R., Sim, W.-J., Lee, E.-S., Lee, J.-H., and Oh, J.-E. (2010). Evaluation of the fate of perfluoroalkyl compounds in wastewater treatment plants. Water Res. 44, 3476–3486. doi: 10.1016/j.watres.2010.03.028
Kurwadkar, S., Dane, J., Kanel, S. R., Nadagouda, M. N., Cawdrey, R. W., Ambade, B., et al. (2022). Per-and polyfluoroalkyl substances in water and wastewater: a critical review of their global occurrence and distribution. Sci. Total Environ. 809:151003. doi: 10.1016/j.scitotenv.2021.151003
Liu, Y., Robey, N. M., Bowden, J. A., Tolaymat, T. M., Da Silva, B. F., Solo-Gabriele, H. M., et al. (2021). From waste collection vehicles to landfills: indication of per-and Polyfluoroalkyl substance (Pfas) transformation. Environ. Sci. Technol. Lett. 8, 66–72. doi: 10.1021/acs.estlett.0c00819
Schultz, M. M., Higgins, C. P., Huset, C. A., Luthy, R. G., Barofsky, D. F., and Field, J. A. (2006). Fluorochemical Mass Flows in a Municipal Wastewater Treatment Facility. Environ Sci Technol. 40. doi: 10.1021/es061025m
Secretariat of the Stockholm Convention (UNEP). (2008). Listing of pops in the Stockholm convention [online]. Châtelaine, Switzerland. Available online at: http://chm.pops.int/Convention/Thepops/Listingofpops/tabid/2509/Default.aspx (Accessed 11 April, 2024)
Solo-Gabriele, H. M., Jones, A. S., Lindstrom, A. B., and Lang, J. R. (2020). Waste type, incineration, and aeration are associated with per -and polyfluoroalkyl levels in landfill leachates. Waste Manag. 107, 191–200. doi: 10.1016/j.wasman.2020.03.034
Takagi, S., Adachi, F., Miyano, K., Koizumi, Y., Tanaka, H., Watanabe, I., et al. (2011). Fate of Perfluorooctanesulfonate and perfluorooctanoate in drinking water treatment processes. Water Res. 45, 3925–3932. doi: 10.1016/j.watres.2011.04.052
U.S. Environmental Protection Agency. (2024). Questions and answers: Drinking water health advisories for Pfoa, Pfos, GenX chemicals and Pfbs. Available online at: https://www.epa.gov/sdwa/questions-and-answers-drinking-water-health-advisories-pfoa-pfos-genx-chemicals-and-pfbs#:~:text=Animal%20studies%20following%20oral%20exposure,(decreased%20serum%20total%20thyroxine) (Accessed April 11, 2024)
Keywords: per- and polyfluoroalkyl substances, PFAS, PFOS, PFOA, landfill leachate, waste water treatment, effluent
Citation: Neill P and Megson D (2024) Landfill leachate treatment process is transforming and releasing banned per- and polyfluoroalkyl substances to UK water. Front. Water. 6:1480241. doi: 10.3389/frwa.2024.1480241
Edited by:
Paolo Fabbri, University of Padua, ItalyReviewed by:
Mariachiara Caschetto, University of Milano-Bicocca, ItalyZhengyu Jin, Minzu University of China, China
Copyright © 2024 Neill and Megson. This is an open-access article distributed under the terms of the Creative Commons Attribution License (CC BY). The use, distribution or reproduction in other forums is permitted, provided the original author(s) and the copyright owner(s) are credited and that the original publication in this journal is cited, in accordance with accepted academic practice. No use, distribution or reproduction is permitted which does not comply with these terms.
*Correspondence: David Megson, d.megson@mmu.ac.uk Pippa Neill, pippa.neill@haymarket.com