- 1Programa de Engenharia Ambiental, Universidade Federal do Rio de Janeiro, Rio de Janeiro, Brazil
- 2Programa de Engenharia Civil, Universidade Federal do Rio de Janeiro, Rio de Janeiro, Brazil
- 3Programa de Engenharia Urbana, Universidade Federal do Rio de Janeiro, Rio de Janeiro, Brazil
- 4Escola Politécnica, Universidade Federal do Rio de Janeiro, Rio de Janeiro, Brazil
- 5Programa de Pós-Graduação em Arquitetura, Universidade Federal do Rio de Janeiro, Rio de Janeiro, Brazil
- 6Programa de Pós-Graduação em Urbanismo, Universidade Federal do Rio de Janeiro, Rio de Janeiro, Brazil
- 7Faculdade de Arquitetura e Urbanismo, Universidade Federal do Rio de Janeiro, Rio de Janeiro, Brazil
- 8Mestrado Profissional em Arquitetura Paisagística, Universidade Federal do Rio de Janeiro, Rio de Janeiro, Brazil
Cities are increasingly dealing with challenges regarding the negative impact of rapid and mismanaged urbanization. Therefore, city planning must cope with the natural environment limitations, seeking a balance between the human activities and the well-functioning of the hydrologic cycle. This work aims to present a conceptual framework able to properly integrate the stormwater dynamics into the open spaces system in a functional way, establishing a Hydrological Interest Area, HIA, to structure urban expansion integrated into and respecting watershed natural processes. The initial step is to define a HIA, primarily consisting of open spaces that can be used for supporting urban drainage functions and to order land use in the urban expansion process. This delimitation offers the background for interpreting the watershed in three functional arches, especially covering the upstream, mid-reach and downstream areas of the basin, guiding the design of a set of flood mitigation interventions focusing on the use of Blue-Green Infrastructure. To illustrate and validate the proposed methodological framework, the design is evaluated by a flood modeling tool, using a hydrological-hydrodynamic cell-model. A case study was driven in the Bambu Watershed, a rapidly developing area in the municipality of Maricá, Rio de Janeiro, Brazil. The proposed intervention includes an urban expansion scenario for a low impact development on flood behavior alongside with four parks: an upstream park with reservoirs, two multifunctional floodable urban parks, and a park dedicated to lagoon restoration. This plan complements riverbed modifications designed to enhance water discharge. The simulation showed significant reduction of water depths with a consequent decrease in exposure of buildings and roads, especially in the most critical region of the watershed. This framework highlights the importance of a multifunctional approach in land use and serves as a robust foundation for controlling urban expansion and proposing projects.
1 Introduction
Cities are increasingly dealing with challenges regarding the negative impact of rapid and mismanaged urbanization. It is common for the urbanization process to bring major land use changes, comprising the decrease of green and permeable spaces, increase of imperviousness rates, and densification of built-up areas (Barbaro et al., 2021; Kabisch et al., 2016; Veról et al., 2020). Consequently, the expansion of cities is directly related to hydrological disturbances (McGrane, 2016), reduced functionality of ecosystem services (Kabisch et al., 2016), and the increasing frequency of urban flooding. In particular, floods can disrupt critical infrastructure and lead to cascading effects that may reflect in different systems failures, such as housing, mobility and community facilities, while triggering financial damages to the more vulnerable and less prepared areas; thus, creating a spiral of unrecoverable losses (Barbaro et al., 2021; Majidi et al., 2019; de Oliveira et al., 2022). These aspects are related to urban degradation over time. In developing countries, urban drainage infrastructure is usually outdated and unable to adapt to future scenarios of urban expansion and climate change (Kim et al., 2016) when compared to developed countries, increasing the urban flood hazard and putting even more vulnerable people at risk, emphasizing the need for a paradigm shift regarding urban drainage measures.
Therefore, city planning must cope with the natural environment limitations, seeking a balance between the human activities and the well-functioning of the hydrologic cycle, aiming to reverse the social and economic damages caused by urban floods. In other words, especially in highly urbanized areas, storm waters require free space to play its natural dynamics (de Oliveira et al., 2022). In this way, environmentally relevant zones must remain unoccupied to preserve ecosystem functions/services, including temporary flooding storage. Therefore, the urban open space system, generally composed by parks, urban forests, or public spaces, can play a key role in flood mitigation, while also providing social and ecological benefits (Xia et al., 2024). Using green open spaces in multifunctional arrangements, including storage and infiltration measures, can be an effective approach to offer a flood control alternative integrated with urban functions. Accommodating water volumes in the open spaces can recover part of the stormwater retention and dampen peak flows (Jing et al., 2022; Kim et al., 2016; Lourenço et al., 2020). However, the urban sprawl phenomenon usually advances over open green spaces, which are frequently seen as potential buildable areas, since their functional roles are not always highlighted, or even not recognized in the urban planning process (Jing et al., 2022). Consequently, the lack of free available areas in consolidated cities is currently one of the main factors that restricts opportunities to face urban flood challenges (de Oliveira et al., 2022). The recognition of land as a valuable resource and the progressive use of the open spaces system as a multifunctional facility (to optimize the use of the remaining urban open spaces) can preserve environmental attributes while ordering the future urban occupation (Lourenço et al., 2020).
Considering the current challenges faced in the urban context, the adoption of more sustainable and resilient approaches intends to reduce the effect of urbanization on the worsening of flooding and to reduce its consequences on the diverse urban sub-systems. This strategy aims to restore flow patterns closer to the natural ones, in addition to mitigating the impact of urbanization on the water cycle. Therefore, dealing with storm water dynamics that should precede urban growth planning and support land-use zoning (de Oliveira et al., 2022). Then, adequate spaces must be allocated for water accommodation as well as for environmental preservation, properly regulating urban occupation.
In this way, this work aims to present a conceptual framework able to properly integrate the stormwater dynamics into the open spaces system in a functional way, establishing a Hydrological Interest Area, HIA, to structure urban expansion integrated into and respecting watershed natural processes. Additionally, design interventions should consider the use of multifunctional landscapes including flood management solutions, where the fluvial corridor acts as an integrating element across different spatial units of the watershed, from upstream to downstream, stablishing a true Blue-Green Infrastructure—BGI system. This approach is especially necessary when considering areas under pressure of rapid urbanization growth, to prevent the overload of the supporting capacity of these areas. Therefore, this study hypothesizes that rivers are the main connecting elements between the urban space and the natural environment and urban flood solutions should be considered along the fluvial space, from upstream to downstream, recognizing the importance of using BGI to recover natural functions that support more sustainable solutions. The rational definition of the Hydrological Interest Area, HIA, based on physical aspects of the territory, is a significant innovation presented in this paper. In order to illustrate the application of this framework and verify the proposed hypothesis, a case study was driven in the Bambu Watershed, in the municipality of Maricá, Rio de Janeiro, Brazil. It is a city in a rapidly developing process, within an environmentally relevant area that includes a large coastal lagoon system and preserved Atlantic Forest areas, facing several challenges regarding its urban quality of life and urban flooding problems.
The paper is organized as follows: Section 2 presents the conceptual basis supporting the proposed framework, discussing the relationship between water and cities, leading up to the development of the blue-green infrastructure approach; Section 3 describes the proposed framework in detail; Section 4 introduces the study area; Section 5 provides the results and further discussion is provided in Section 6; and Section 7 concludes the study and highlights the most important aspects of the discussion.
2 Conceptual basis
2.1 Water in the urban environment: from fragmented edges and degrading driver to an opportunity to foster urban quality of life and integrate natural and built environments
According to Waldheim in “Claiming Landscape as Urbanism” (2016), the traditional urban design is challenged in the frontier spaces of the city, where conventional categories that traditionally underpin the common understanding of cities fail - typically based on morphological elements such as streets, lots, buildings, squares, among others constituting the urban fabric (Lamas, 1993). These spaces encompass sparsely developed suburbs, large-scale commercial and sports facilities, highways and junctions, plantations, major reservoirs, treatment plants, landfills, quarries, interstitial vacant lots, among others (Folch, 2002).
Waldheim (2016) argues that landscape architecture, particularly infrastructural landscape, has proven to be a valuable tool for rethinking urban design in areas undergoing significant ecological, infrastructural, or economic changes, whether due to decline or appreciation—an albeit rarer scenario, but not uncommon. Landscape infrastructure often aids in city planning to address and mitigate the impacts of climate crisis, especially in areas where ecological systems and complex infrastructures intersect, precisely as examined in this study. These are scenarios where landscape design aligns with Blue-Green Infrastructure, what can be especially useful in less formalized or in-process formalization areas, also considered as frontier spaces. This perspective also addresses challenges related to risk, resilience, adaptation, and the diverse changes that cities face from internal and external forces, bringing opportunities to integrate flood management solutions into urban and landscape design, such as Low Impact Development—LID (Dabas et al., 2019; Wang et al., 2023), Sustainable Urban Drainage Systems—SUDS (Karami et al., 2022; Miguez et al., 2015; Rodríguez-Rojas et al., 2024), and Water-Sensitive Urban Design—WSUD (Avazpour et al., 2023; Meng, 2022).
From a conventional perspective based on city centralities, the open spaces in the city frontiers are predominantly described as peripheral, marginal, encircling the city - border or fringe areas—and they are not considered to be integrated into the conventional urban fabric. It is essential to note that these descriptions are always contextual and observer dependent. However, our argument is that these open spaces should be properly recognized and designed as integral components of the urban structure, not merely as peripheral leftovers, composing a functional open space system capable of ordering an adequate city growth, similarly to what was proposed by Tardin (2013).
It is pertinent to highlight some interdependencies among urban subsystems, despite the risk of using an oversimplified logic. For instance, the effects of increased mobility, notably through the hyper acceleration of goods and information, lead to shortening distances, thereby short-circuiting voids along the way. Consequently, these voids become “non-important,” fragmented spaces at the urban scale (Graham and Marvin, 2001; Illich, 2005). This aspect intertwines with persistent effects of economic uncertainties, climate crises, and flaws of traditional urban planning in face of contemporary city dynamics. In such situation, large-scale infrastructures are prioritized over a truly systemic and functional composition of open spaces.
Activities related to water management, however, should follow the biotic rhythm consistent to sustaining life. Although frequently neglected in the urban fabric production, the water dynamics should be crucially considered in contemporary urban practices, shaping urban spaces and infrastructures. The interaction among rainwaters, urban environment and fluvial corridors defines edges and interfaces that will shape the local environmental quality. If inadequately managed, this process can lead to urban flooding, population impoverishment, health problems, and river degradation. On the other side, if this interaction is understood and fostered, urban biodiversity can increase (Roggema et al., 2021), buildings in the waterfront and in areas where flood risk were mitigated can rise in value (Kozak et al., 2020), leisure options become available and ecosystem services can be provided (Brom et al., 2023; Lovell and Taylor, 2013). In this way, it would be useful to define a shared area where natural water dynamics could develop and support a qualified urban development, systematically meeting natural and urban needs. This area is named here as Hydrological Interest Areas (HIA), and it should be central to the urban fabric structuring due to its ability to promote natural and anthropic connections, in general.
Urban form, landscape and infrastructures are key elements in the spatial conception, especially at ground level (Argan, 1983). Urban rivers and water bodies can delineate privileged natural spaces in the urban tissue, emphasizing the importance of urban planning integrated with water management. The passage and presence of water in the territory precede the city itself, and the understanding of these processes enables urban growth to coexist harmoniously with these natural flows, as highlighted by White (1945) in his classic publication “Human adjustment to floods,” revisited by Macdonald et al. (2012).
This approach reveals an ability to integrate environmental and infrastructural systems, establishing what is structuring in the urban planning process, but also remaining flexible for future decisions based on unforeseen demands and needs, especially pertinent in cities undergoing significant changes.
Water management initiatives promoting the expansion of green areas can be considered the basis for actions enhancing community life, closely tied to Henri Lefebvre’s concept of the “right to the city.” Green spaces provide venues for leisure and social interaction crucial for climate adaptation, by moderating temperature, improving soil quality, and effectively managing floods, serving as water reservoirs during extreme events (Brom et al., 2023). These areas enrich biodiversity and contribute to sociocultural cohesion, reducing crime rates and enhancing both physical and mental health (Brom et al., 2023).
Lefebvre (2009) argues that urban planning, considered here as the joint urban, landscape-infrastructure planning, should prioritize public interest and ensure fair distribution of resources. Therefore, water management strategies, combined with the provision of open green spaces, are essential to reach this right. These initiatives not only enhance water quality and strengthen local community engagement with open space systems but also embody aspects of environmental justice (Mullenbach et al., 2019). To address these challenges, the BGI system emerges as a smart concept to integrate built environment and natural areas into a cohesive whole, enhancing benefits and resilience across the entire watershed, as discussed on next section.
2.2 Blue-green infrastructure toward a water-oriented urban planning
Natural blue and green elements are being increasingly recognized in the literature for their capacity to support biodiversity conservation (Kabisch et al., 2016) and perform ecological and societal functions in cities, including the accommodation of stormwaters. According to Cohen-Shacham et al. (2019), this conception comes from a paradigm shift in nature conservation, in which society turned from being passive beneficiaries of nature, to being proactive in efforts to protect, manage or restore ecosystems. Therefore, urban planning guidelines should harmonize the natural and built environments, “building the city” together with water dynamics (Moura Rezende et al., 2019). In this sense, the Blue-Green Infrastructure (BGI) concept is commonly defined as an interconnected network of natural and designed landscape elements, composed of water space and open green spaces, providing several benefits related to connectivity and multifunctionality (Battemarco et al., 2022; Ghofrani et al., 2017; Qiao et al., 2022). BGI can act effectively to reverse the ongoing degradation of natural resources, while also increasing the balance between the conservation and sustainable development objectives (Cohen-Shacham et al., 2019). This comprehension is strictly related to the ecological connotation of BGI, in which the natural processes play a fundamental role, especially considering the watershed as a territorial planning unit. Benedict and McMahon (2006), for instance, described the green infrastructure network through a system of hubs, links and sites. Therefore, the hubs are responsible for anchoring the network and providing space for native plants, animal communities, and ecological processes; links are the ecological corridors that serve as biological conduits for wildlife; and sites are smaller-scale elements that can contribute to the protection of wildlife and providing space for nature-based recreation inside cites, integrated in the urban fabric. When combined, these elements can support biodiversity through the provision of structurally complex vegetated systems capable of increasing the number and variety of niches and species (Molné et al., 2023), as well as creating space for water accommodation.
Increasing urban expansion, both planned and unplanned, has led to a significant decline in urban green spaces, exerting pressure on natural ecosystems (Brom et al., 2023). Aiming to avoid undesired urban sprawl, many cities have adopted protection areas, as greenbelts (Bueno-Suárez and Coq-Huelva, 2020). Considering that environmental protection areas are not isolated from the surrounding landscape, being usually situated in the edge of densely populated centers, the rapid urbanization pressure and the anthropogenic activities affect the availability of green open spaces, expose ecological functions and increase the risk of flood occurrence (Monteiro et al., 2020; Veselinović et al., 2023). Therefore, urban planning must be aligned to the design of sustainable and water-oriented cities, a strategy that can be achieved through a more harmonious interaction between the natural and built environment. To this end, multifunctionality and integration are key principles that considers the synergies between green and grey devices, while improves the efficient use of the available space, since open areas are usually a limited object of competition (Ahern, 2013; Monteiro et al., 2020). In an integrated perspective of urban planning, vegetated elements, expressed by greenways or ecological networks, play an essential role in the urban landscape, promoting the environment connectivity in the urban ecosystem (Ahern, 2013), while preserving the connectivity of urban water systems generates economic effects, such as the improvement of water quality, the increase of property values, and the provision of convenient spaces for the population use (Havránková et al., 2023). Thus, blue-green elements act as links between the nature and human society, playing an important role in living conditions (Qiao et al., 2022).
BGI is capable of dealing with flood risks and minimizing the urban impacts on the hydrological cycle (Hamel and Tan, 2022; O’Donnell et al., 2021). This performance is related to the ability of BGI to decrease the pressure on the existing drainage system, by storing, infiltrating, and decelerating runoff, thereby decreasing the flood depths and duration (Ambily et al., 2024; Richter et al., 2024). Therefore, in contrast to the conventional urban drainage infrastructure, which is commonly built underground, isolated from the perspective of urban planning and sustainable needs, BGI can support urban solutions and cope with natural demands (Qiao et al., 2022; Wang and Foley, 2023) (Xie and Bulkeley, 2020). This approach is directly related to several concepts of flood risk management, in which waterways are perceived as structuring elements in city planning, such as they appear in water-sensitive urban design (Sharma et al., 2016), sponge cities (Qi et al., 2021), and blue-green cities (O’Donnell et al., 2020). In particular, a sponge city refers to the development of a city with a water system similar to a sponge, capable of absorbing, storing, infiltrating and purifying rainwater before its release (Li et al., 2017). In China, sponge cities are recognized as an official planning strategy since 2013, especially due to rapid urbanization and pressure on urban water systems (Qi et al., 2021). Recently, considering the evolving approaches of stormwater management—from the flood control measures to a focus on flood risk reduction—the need of a more holistic perspective that encompasses the entire watershed territory has led to the reconceptualization of the Sponge City Approach as the Sponge Watershed Approach (Peng et al., 2022). Thus, considering flood management, green open spaces act as a key element toward more sustainable and integrated solutions. In Seoul, Kim et al. (2016) found that flooding occurrence could be reduced by over 50% in areas with greater amounts of green spaces. In Rio de Janeiro, de Oliveira et al. (2022) showed that the drainage network supported by BGI is capable to provide several gains for different urban systems, such as housing, sewage, mobility, community facilities, economy, and cultural heritage. On the other hand, in Portland, Thorne et al. (2015) highlighted the additional benefits provided by BGI, such as improved air quality, energy savings, reduced greenhouse gas emissions, improvements in amenities and aesthetic value, decrease in crimes, and better access to nature.
The application of BGI in the urban environment can vary in different scales, from local to regional use, which includes typologies such as rain gardens, green roofs, fluvial parks and urban reforestation (Zaręba et al., 2022). Therefore, the solutions can be applied distributed at the watershed scale, even in large interventions, like the restoration of rivers, the introduction of larger water retention or detention reservoirs, and the creation of interconnected blue-green corridors (Zaręba et al., 2022). Moreover, BGI is considered a well-suited strategy for dense urban areas since it reduces the need to both upgrade or expand traditional drainage systems, in spatially and financial terms (Ahmed et al., 2019). In general, the BGI effectiveness is related to understanding the urban context, and recognizing the physical characteristics and local biodiversity, in order to adequate the interventions to the local natural, social and economic conditions (Babí Almenar et al., 2021; Sowińska-Świerkosz and García, 2022). The availability of green spaces allows the design of a functional open space system that can offer environmental services as well as co-benefits related to human well-being (Kabisch et al., 2016; Xia et al., 2024). In-between these possibilities, flood retention is both an environmental service, since lost water cycle functions can be recovered, and an urban service, since cities can be healthier and continue to offer a myriad of urban services, without losing its functions during flooding.
3 Materials and methods
The method involves proposing and evaluating a framework to integrate urban drainage measures into the urban open space system, by defining a formal area to be used as the main stage for projected actions. Therefore, the initial step is to define a Hydrological Interest Area (HIA), primarily consisting of open spaces that can be used for supporting urban drainage functions and to order land use in the urban expansion process. This delimitation offers the background for interpreting the watershed in three functional arches, especially covering the upstream, mid-reach and downstream areas of the basin, allowing the proposition of different types of strategies that can be more suitable for each arch of the watershed. In this context, preserved vegetated upstream areas should be conserved, urban and natural needs should be integrated in the mild areas and outflowing discharges should be guaranteed in the lower downstream reaches, concerning water quantity and quality aspects.
Furthermore, the HIA serves as a valuable tool for guiding future urban expansion, considering water dynamics to structure the territory and guaranteeing an adequate land use planning. The HIA can identify in advance the areas naturally prone to flooding or those suitable for accommodating flood flows. Consequently, these flood-vulnerable zones can be prioritized during the initial stages of urban planning, not only to prevent population exposure increasing to floods but also to allow the allocation of functional areas where compensatory measures can effectively manage floodwaters. This strategic approach aims to deal with the modifications introduced in the urban water cycle, mitigating the negative impacts of urbanization. Finally, the effectiveness of this approach, including a set of proposed flood mitigation interventions focusing on the use of BGI, is evaluated by a flood modeling tool, using a hydrological-hydrodynamic cell-model to illustrate and validate the proposed methodological framework.
The general framework is illustrated in Figure 1. The method starts with the mapping of four criteria that are going to form the three categories of the HIA. This definition is followed by the functional interpretation of the watershed in three zones—or spatial arches, each suited for different types of intervention. This analysis identifies the most appropriate locations for intervention and their potential functions, leading to the subsequent step of designing a targeted intervention for the Watershed. The proposed project must be integrated with controlled land use, as ensured by the AIH, and its effectiveness can be evaluated using a hydrological-hydrodynamic simulation tool. If the intervention does not prove effective, it may require revision. These steps are discussed in detail in the sequence.
3.1 Definition of the hydrological interest area
The HIA is a new zoning category proposed and designed to ensure that areas in the urban watershed can be able to assume blue-green functions, providing space to accommodate stormwaters without disrupting urban services and quality of life and promoting a better environment, guaranteeing the natural connections across the whole territory. The establishment of a HIA integrates the natural watershed behavior into the urban planning process, aiming to preserve critical regions from an urban drainage perspective (Gomes et al., 2023). This delimitation is composed mainly by susceptible open spaces, which reinforces the need for greater control over the densification of the territory, to ensure that flood prone areas remain free from occupation—what is certainly the most economical way to reduce flood risks (and costs), by avoiding exposure.
Designing a system structured by green and blue components is essential for sustainable urban development and resilience to floods. The HIA facilitates the interaction between these natural elements, promoting ecological connectivity, and improving the relationship between the built and natural environments. Nonetheless, the HIA should not only consist of articulated blue and green components, but also supports urban activities and provides urban services. To achieve this aim, a robust management plan must be in place, ensuring the preservation of environmental quality and the capacity of delivering fundamental environmental services, including flood control. The HIA must serve as a backdrop for planning actions to order urban expansion, integrating urban and ecological functions. The goal of this integration is to achieve a truly interconnected built and natural environment where the city becomes part of the nature.
The HIA can encompass areas that are currently protected by law along with additional areas needing further control—especially due to flood susceptibility. In fact, protected areas are not a main worry, but they are important to be jointly considered to offer a functional systematic approach. Moreover, the HIA can incorporate multiple levels of control: from fully protected areas, where urbanization is prohibited, to areas allowing sustainable land use management. The HIA’s delimitation is based on the overlap of four criteria: (i) legally protected areas (that already exists), (ii) coastal lowlands, (iii) (almost) flat open spaces and (iv) riverine flood-prone regions. The resulting HIA can be divided into three categories: (1) Environmental Full Protected Areas (already existing and defined by the first criterion); (2) Environmental Areas subject to Sustainable Land Use Management (already existing and also defined by the first criterion) (3) Environmental Areas subject to Sustainable Land Use Management (to be created as identified by the other 3 criteria in the HIA construction). It should be noted that “Environmental Areas subject to Sustainable Land Use Management” refer to areas with environmental importance, but that do not require a full protection. Eventually, these areas can be partially occupied, if this occupation follows preventive rules and specific building standards to be defined in specific Environment Management Plans. Therefore, while the first two categories correspond to the already Legally Protected Areas, the third corresponds to the combination of the other criteria (coastal lowlands, flat open spaces and riverine flood-prone regions), which are currently not legally protected but need a stricter occupation control. The resulting network comprises vast upstream green conservation areas, downstream lowlands, flat open spaces throughout the watershed, connecting floodplains and fluvial corridors, embodying the concept of hubs (large conservation areas), sites (distributed opens spaces) and links (fluvial corridors and floodplains), which form the Blue-Green Infrastructure approach.
3.1.1 Legally protected areas
The delimitation of the HIA should begin by identifying legally protected areas, whether they are strictly protected or designated for controlled urban development, to prioritize environmental preservation. To achieve this, laws that include provisions for the creation and management of protected areas must be consulted. Some examples of environmental protection laws are: the National Park Service Organic Act (United States Congress, 1916), from the US, the European Union’s Habitat Directive (European Union, 1992), the Indian Wildlife Protection Act (Government of India, 1972), Australia’s Environmental Protection and Biodiversity Conservation Act (EPBC Act) (Government of Australia, 1999) AND South Africa’s Protected Areas Act (Government of South Africa, 2003).
In the development of this method, the test case refers to an application in Brazil, which specifically adheres to the Brazilian Forest Code (Government of Brazil, 2012) and National System of Conservation Units (SNUC) (Government of Brazil, 2000). The Forest Code defines Permanent Preservation Areas (PPA) as fully protected zones with the purpose of preserving landscapes, water resources, geological stability, and biodiversity. PPAs include Riparian Buffer Strips, whose width varies depending on the width of the river course, with a minimum value of 30 meters. They also cover slopes steeper than 45°, mountain tops with a minimum height of 100 meters, certain types of vegetation, among other specific criteria.
The CUs are environmentally relevant areas established to preserve biodiversity, ensure sustainable use of natural resources, protect water bodies, restore degraded ecosystems and promote contact with nature through research and recreational activities. CUs are categorized into Integral Protection and Sustainable Use: the first permits only indirect use of natural resources while the latter aims to balance conservation with sustainable use of part of their resources.
These laws ensure that critical natural spaces are preserved, managed, and utilized in a way that supports ecological balance and long-term environmental health. It is important to highlight that, while upper-level governments (such as national or regional authorities) provide the overall framework for planning, local authorities are often responsible for land use definitions, making decisions about how city will expand (Krawchenko and Schumann, 2023).
3.1.2 Coastal lowlands
Coastal lowlands are particularly susceptible to flooding due to their proximity to sea level or other adjacent water bodies (like coastal lagoon systems). This physical predisposition results in a faster water accumulation during heavy rainfall, while discharge into the water body is hampered by low slope and downstream level control, increasing flooding likelihood. Therefore, the HIA incorporates all the areas with low absolute elevation. The definition of the elevation ranges to be incorporated into the HIA must take into consideration the variations of the sea level in the study area, which may vary from one region to another.
3.1.3 Flat open spaces
Flat terrains pose significant drainage challenges and tend to easily be flooded. Open spaces hold the potential to allow redesigning urban landscape, contrasting with the limited planning opportunities of dense urbanized areas. Therefore, the HIA should prioritize the integration of open spaces that fall within flat or mild-slope areas, defined here as regions with terrain slopes up to 0.5%. This approach recognizes that the intersection between open spaces and mild-slope areas present a unique opportunity to implement effective drainage solutions and enhance flood management. Besides, these areas should be protected from future urban occupation. Due to drainage difficulties, these areas are ideal for storing rainwater, as their physical characteristics favor water accommodation. By including flat open spaces, the HIA can optimize the use of available land for environmental preservation and avoid urban densification in areas with poor drainage conditions.
3.1.4 Riverine flood-prone region
This criterion maps the potential extent of river overflows, considering the physical characteristics of the local terrain. It is calculated through the combination of two indicators: the Slope Indicator (IS) and the Proximity Indicator (IPROX), both originally developed to compose the Index of Physical Susceptibility to Flooding - PhySFI (Miranda et al., 2023). While IS measures the surface slopes, considering that flat areas are more difficult to drain, IPROX consists of the combination between the distance and the transverse slope relative to the nearest waterbody. The normalization of IS and IPROX are represented in Tables 1 and 2, respectively. The results of both indicators, ranging from 0 to 100, are displayed in 5 categories (0, 25, 50, 75 and 100), which can be, respectively, and qualitatively interpreted as: very low, low, medium, high and very high possibility of river overflow. Mapping the Riverine Flood-prone Region (RFPR) corresponds to the combined observation of areas where at least one of the indicators score very high and other score high. Differently from the definition of the previous criteria, which were directly related to one indicator, the representation of Riverine Flood-prone Regions is equated using the combination of two indicators. It can be calculated through a weighted product considering equal weights for both indicators, as shown in the following equations (Equations 1, 2).
The Floodplain overflow region covers areas which score at least 86.6 (which is the result of combining a high and very high scores for any of the indicators, that is, ).
3.2 Functional interpretation of the watershed areas: spatial arches
After the delimitation of the HIA, which will act as the background of urban interventions, the following step in this procedural approach is to interpret the functionality of the whole watershed, from upstream to downstream. This understanding can guide the selection of different types of interventions within the watershed, tailoring actions to each segment characteristics and needs. These actions should be settled in the open space system introducing BGI solutions, to create a network of blue and green elements that fulfill both environmental and social functions, including effective flood mitigation.
This step aims to present a spatial evaluation procedure for watersheds, based on recognizing three watershed general segments, addressed as “spatial arches,” each with different behaviors and relations with the constructed environment. The arches correspond to the upstream, middle and downstream stretches along the watershed. The main river and its tributaries act as an integrating driver, connecting these segments from springs to outfall. It is possible to suggest solutions that combine open spaces—located within the previously set HIA—with flood control measures fostering ecological and urban interactions, in an organized system.
The functional spatial arches are summarized by three concepts: Protect (upstream arch), Integrate (mid-watershed arch) and Respect (downstream arch). Figure 2 schematically shows this configuration. In the highest areas of the watersheds, where hillsides, rural areas, river springs and environmental conservation units are generally located, usually sheltering remanent forest areas, the planning strategy should combine the preservation of open green spaces with restoring the natural retention capacity that typically characterizes these areas. The upstream arch can receive temporary storage measures, such as reservoirs (if needed to mitigate downstream floods) and be regulated by guidelines ensuring the protection of ecologically significant natural areas and preventing dense urban occupation in its borders. These measures aim to balance the water cycle, attenuating the flow generation to protect the downstream areas from floods.
In the “mid-watershed arch,” marked by the transition between natural environment and denser urban occupations, located in mid-slope and flatter regions, the main concern is balancing these different dynamics. Green open spaces are often targeted for real estate development, which can foster environmental degradation and increase soil imperviousness. The issues in the mid-watershed area include: (1) maintaining permeable spaces within the built environment; (2) preventing occupation of flood-prone areas; and (3) reversing existing floodable urban areas, defining preferred and controlled flooded areas. To address these issues, urban parks incorporating retention or detention ponds can be established in near flood plains and along the riverine environment protected strips. These parks should temporarily store floodwaters and provide urban functions to justify their existence, being integrated in local community. Multifunctional parks offer recreational areas, protect residents from urban flooding, and ensure ecological connectivity and a well-functioning hydrological cycle.
In the “downstream arch,” typically representing the most urbanized area of the watershed near the river outfall (into another river, lagoon, bay or sea), the main concern is the system’s inertia. River discharges face greater challenges due to mild slopes and backwater effects, leading to water accumulation in urban spaces. Therefore, in the downstream arch, final discharges of the drainage system must be optimized to reduce backwater effects, but without transferring flooding downstream. Increasing discharge capacity through dredging and widening the river’s final stretch may be considered for guaranteeing the river discharge capacity, provided there are no negative downstream consequences. It is interesting to note that these river stretches are usually prone to siltation and dredging may be needed to sustain the discharging capacity. It is important to guarantee a good quality of water discharging to the next waterbody.
Urban pluvial and fluvial waters flow longitudinally through the watershed, passing through these three spatial arches. The river serves as the primary blue-green link from upstream to downstream, emphasizing the need for city planning and design to consider watersheds as the reference planning unit, with solutions addressing rivers from source to mouth.
This proposal integrates flood mitigation actions within a landscape design project, aiming not only to reduce hydraulic risks but also to enhance the quality of blue and green spaces for the population, ultimately contributing to a city with a better quality of life.
3.3 Urban expansion prediction
The methodological step relates to the urban expansion prediction and involves estimating an urban expansion scenario over a 10-year timeframe (which was taken as reference, in accordance with Brazilian legal provisions). This scenario should be aligned with controlled land use conditions in a way that a sustainable land use prevails and allows the implementation of HIA. In this situation, the macro drainage system behavior considering the urban growth, occurs under a favorable urban occupation in terms of ecological preservation. This future situation sets the foundations for implementing alternative flood control alternatives design within a blue-green infrastructure system, while emphasizing the crucial role of proper urban planning in flood mitigation. Inadequate planning leading to unregulated growth can undermine sustainable urban drainage solutions.
In this expansion scenario, new inhabitants are predominantly allocated in vacant urban lots already equipped with proper infrastructure, favoring compacity. This initiative tries to consolidate urban development, optimizing existing infrastructure and avoiding urban sprawl. By prioritizing development in already established urban areas, one can consume less land (which, in fact, is a primary natural resource) and prevent exacerbating social and environmental vulnerabilities while ensuring the protection of riparian buffer zones and preservation areas, by reducing social pressures toward these lands. Flood-prone regions, including natural wetlands, riverine areas, and floodplains, must remain unoccupied and preserve their ecological functions.
Finally, urban expansion within the HIA should be strictly controlled to preserve this ecologically vital zone. New developments inside this kind of land use zone must be sensitive to the natural presence of water in the area and its ecological importance. Consequently, any new housing projects within this region will be regulated under stringent conditions. Ensuring controlled development in the HIA is essential to maintain the balance of the watershed and support sustainable urban growth. It is proposed that urbanization within these areas considers the Water Sensitive Urban Design concept.
3.4 Framework assessment
A hydrological-hydrodynamic simulation model, the Urban Flow-Cell Model (MODCEL) (Miguez et al., 2017), was used to map floods in current and future urban conditions, allowing to assess flood control project results over the proposed urban development. MODCEL is particularly tailored to represent urban floods and illustrative applications can be found in the literature (Battemarco et al., 2022; de Oliveira et al., 2023; Pérez-Montiel et al., 2022; Scionti et al., 2018). The use of this specific model was defined by a practical, but nonobligatory, choice of the modelers - other hydrodynamic models could also be used.
MODCEL can represent a complex flow network in the urban watershed, joining rainfall-runoff transformation, superficial flows, storm drains flows and river flows in an integrated way. It works as a quasi-2D multilayer model, since it represents the two-dimensional characteristics of the watershed using only traditional 1D hydraulic equations, like the Saint-Venant equation, while it has also a pseudo-3d approach since it vertically combines surface flows interacting with storm drain networks (Miguez et al., 2019).
The watershed is divided into flow-cells, which act as homogeneous compartments and can assume a set of predefined types: channel, natural plains, urban plains, reservoir, storm drains, among others. Information such as surface runoff, terrain elevation and urbanization patterns must be assigned. The cells connect with each other through hydraulic links, which can be also configured according to their type—channel, surface flow, weirs, flap gates, gutters, inlets, pumps, storm drains, among others. The model results provide information regarding water depths in each cell, discharges and flow velocities in each hydraulic link between cells. Figure 3 represents the elements of the model and the functional processes of a flow-cell.
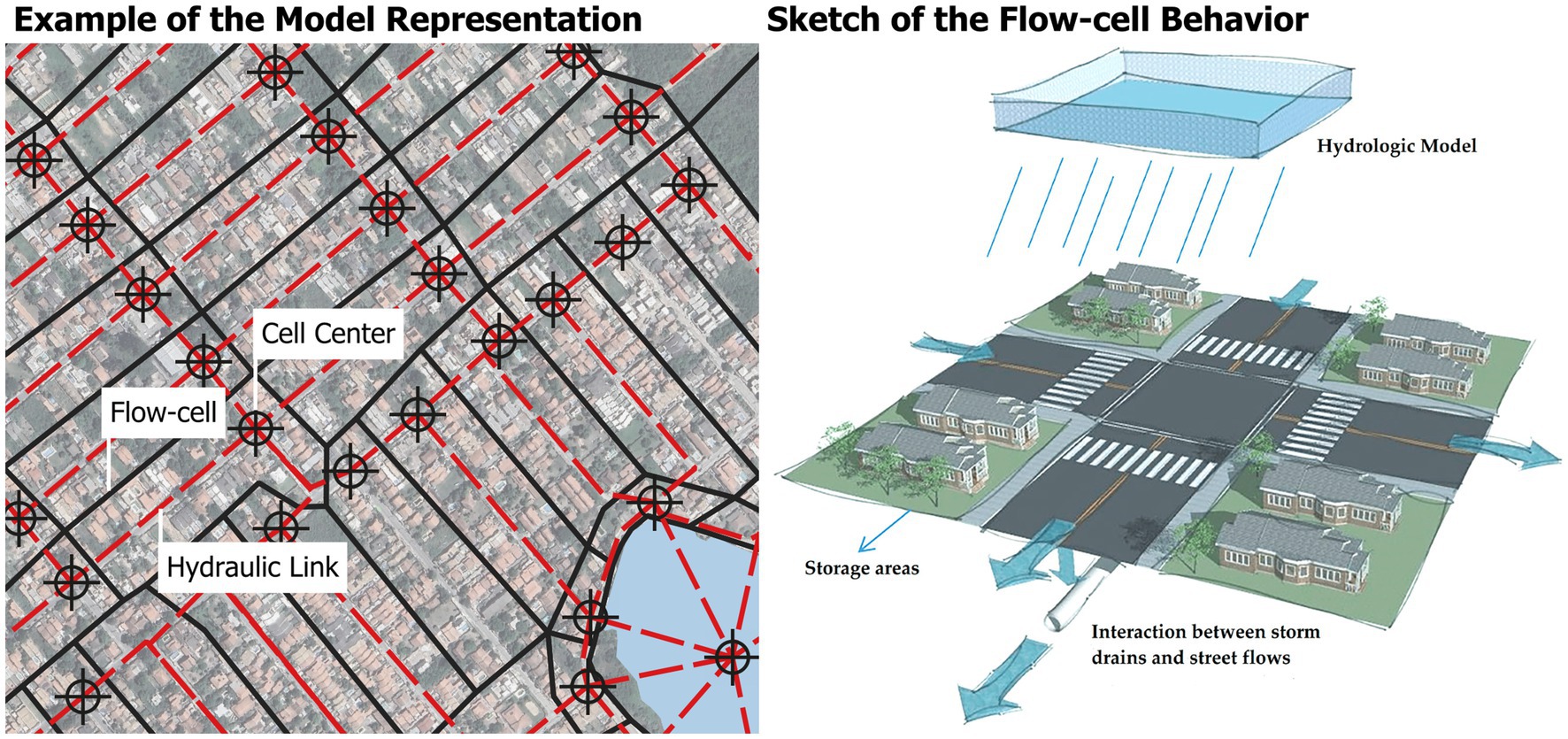
Figure 3. Representation of MODCEL. Adapted from Miguez et al. (2017).
4 Study area
To illustrate, discuss and validate the outcomes of the proposed framework, it was applied in a chosen study area. The criteria for that selection include environmental fragility, which indicates a natural propensity for water accumulation, and rapid urban growth, which could increase future flood exposure and accelerate environmental degradation. In face of these expected characteristics, the study focuses on the Bambu River watershed in an expanding region of the municipality of Maricá, which is part of the Metropolitan Region of Rio de Janeiro, Brazil (Figure 4). The municipality of Maricá covers an area of 361,572 km2. It has experienced a rapid urban expansion drive by social programs, greatly supported by an economic growth coming from oil royalties. The municipality features significant cultural, historical, and environmental assets, including the extensive Maricá-Guarapina lagoon complex.
It is also noticeable that the municipality naturally presents an amphitheater-like topography, in which the existing mountain range has a high slope in short stretches, leading to rapid water flows in the rivers and floodplain areas. The downstream effect of the sea on the lagoon system creates a restriction to fluvial discharges at the river outlets, increasing backwater effects and the occurrence of urban flooding. Currently, urban areas cover only 19.85% of Maricá territory, concentrated along highways and the coastline. Concerns over future urban expansion threatening flood-prone river plains highlight the importance of preserving green spaces to mitigate flood risks.
Specifically, the Bambu Watershed, defined as the interest area inside Maricá, occupies approximately 109 km2, stretching 18.7 km along its main river. Altitude ranges from sea level to 870 meters, with urbanization predominantly in coastal lowlands below 50 meters. Upstream areas are mountainous with steep slopes (50 to 75%), while downstream areas are flat with minimal slope. This topography accelerates rainwater flows into urban areas, exacerbating flooding issues, primarily flowing toward the Maricá Lagoon or to the underutilized and silted Channel of the Coast, which discharges into the sea after running 14 km along the coastal shoreline. Annual precipitation averages 1,150 mm, with a wet summer Land use classification shows that 37.80% of the municipality is urbanized for residential and commercial purposes, green spaces constitute 60.37%, blue spaces (wetlands and water bodies) account for 1.13, and 0.7% comprises other elements such as sand and rocks. The urban fabric is concentrated along the coast with scattered development toward the northern limit.
5 Results
5.1 Hydrological interest area designation
The outcome of the application of the proposed method for the delimitation of the Hydrological Interest Area is represented in Figure 5, along with each criterion. It is divided in the three categories previously mentioned: (1) Environmental Full Protected Areas (already existing); (2) Environmental Areas subject to Sustainable Land Use Management (already existing) (3) Environmental Areas subject to Sustainable Land Use Management (to be created as identified in the HIA construction). The third category is composed by coastal lowlands, flat open spaces and Riverine Flood-prone Region.
The mapping of the Legally Protected Areas considered both the Forest Code (Government of Brazil, 2012) and the Conservation Units (CUs) (Government of Brazil, 2000). In the Bambu Watershed, the Integral Protections Units include mountain ranges, which are safeguarded for wildlife or designated as state parks, and natural monuments, such as Pedra de Itaocaia and Pedra de Inoã. Sustainable Use Units serve as buffers to fully protected zones, allowing controlled urbanization. While the PPAs represent areas of full protection, the CUs can determine either full or partial protection. The CUs are vast regions primarily located mainly upstream, acting as hubs, while the riparian buffer strips of the PPAs function as links between these extensive green areas and river mouths, traversing urbanized zones. Despite their legal protection, many riparian buffer strips within PPAs are occupied by urban development.
Regarding the proposed sustainable land use management, the areas incorporated into the HIA include Lowlands, Flat Open Spaces and Riverine Flood-prone Regions. For the Lowlands, sensitive to sea level variations, the designated elevation range, according to Rio de Janeiro standards, is up to 2 meters above sea level. Consequently, all areas located in the interval between zero and two meters are included in the AIH. Most of this area surrounds the Brava Lagoon, a silted lagoon without a permanent water surface area. However, this is an officially recognized lagoon by the Rio de Janeiro estate environmental agency. While largely unoccupied, some intersections with urbanized areas exist, posing a potential future concern, mainly due to rising sea levels from climate change.
Numerous flat open spaces were identified throughout the watershed due to its low slope and the abundance of undeveloped land, as urban expansion is still in progress. These spaces are dispersed across the watershed: some are downstream near river mouths, posing natural hazards related to water discharges, while others are upstream near mountain ranges, suitable for reservoirs.
The mapping of Riverine Flood-prone Region considers terrain characteristics that make them prone to river overflow, such as longitudinal and transversal slopes relative to the waterbody. The results for the Bambu Watershed indicate that even upstream areas with low slopes are susceptible to overflow and need careful management. This criterion reinforces the connection between conservation units and river mouths in the lagoon system, integrating all other mapped criteria.
5.2 Design alternative
In this framework, the selected watershed was conceptually divided in three main spatial arches, from upstream to downstream, longitudinally connected by the rivers, until reaching the coastal lagoon system. These will be the stage for action in terms of urban flood prevention and mitigation. Figure 6 maps out the proposed interventions across the three segments and outlines the boundaries of the HIA applied to the Bambu Watershed. The set of reservoirs is illustrated in more details in Figure 7.
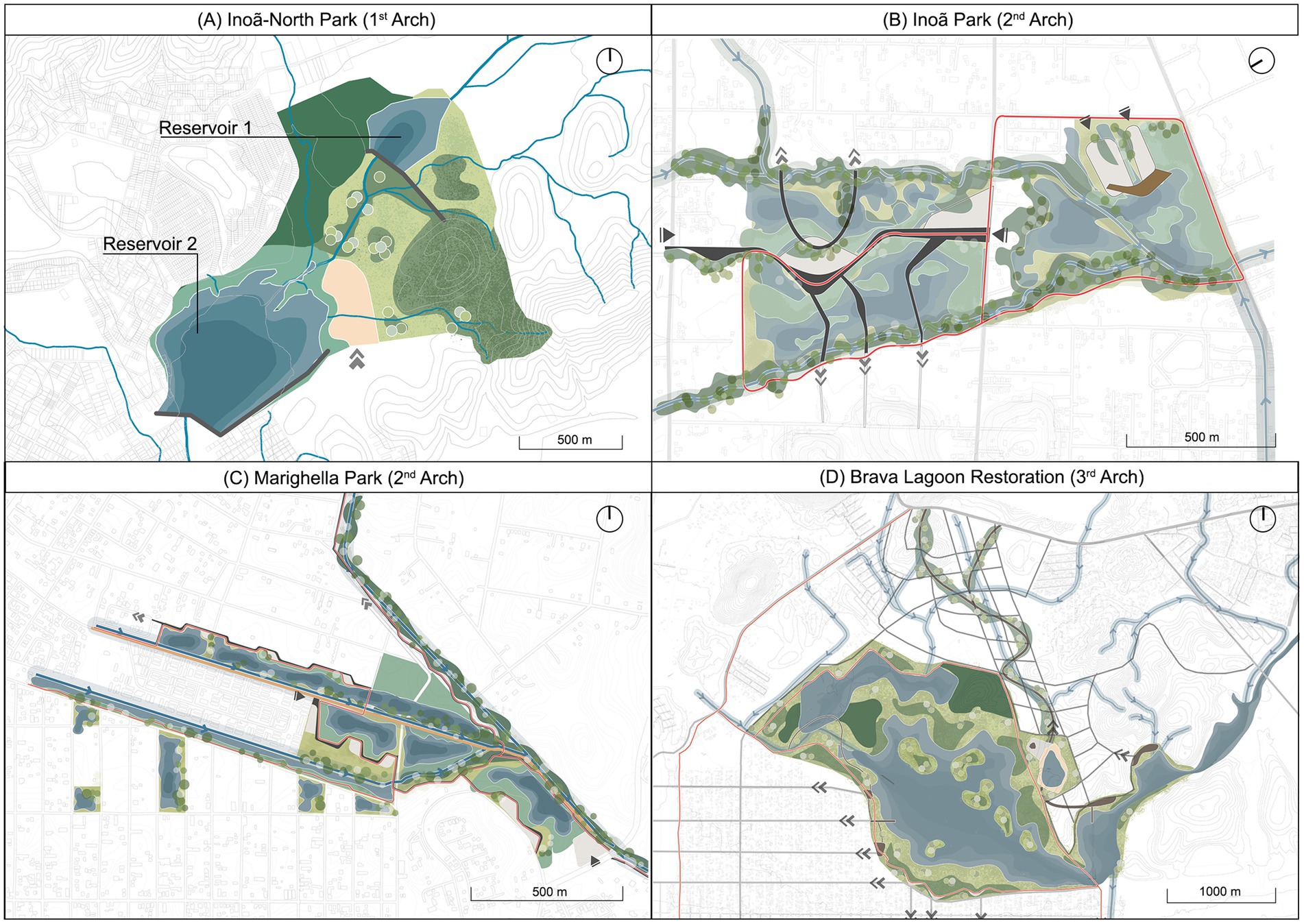
Figure 7. Set of conceptual design interventions for the Bambu Watershed. (A) Inoã-North Park (B) Inoã Park (C) Marighella Park (D) Brava Lagoon Park.
In the first arch, a set of two reservoirs located upstream of the watershed are placed to dampen flood peaks flowing in the main watercourse. They are integrated into the proposed Inoã-North Park (Figure 7A), located on the border of the rural area of the municipality. Due to its low-density urbanization surroundings, this park is proposed to serve rural functions, such as agroforestry and equestrian activities, incorporating multifunctionality although preserving more natural characteristics. Reservoir 1, located in a rural area of the Cassorotiba neighborhood, is formed by a dam in the Vigário River and has 312,760 m3 of storage capacity. Reservoir 2, situated in-between the neighborhoods of Santa Paula and Inoã, acts as a second important storage area, protecting the Jardim Atlântico Central neighborhood, reaching 165,672 m3 of capacity.
In the mid-watershed arch two interconnected multifunctional parks are proposed, integrating urban drainage solutions within the urban open space system: the Inoã Park (Figure 7B) and the Marighella Park (Figure 7C). The first is located in a large open space of approximately 72 hectares, in Chácaras de Inoã neighborhood, delimited by Taquaral and Inoã Rivers. Its plain topographic conformation contributes to the creation of a temporary pond with water levels of approximately 1.0 m, considering a 25-year return period rainfall. Due to its strategic location in the central region of the watershed and the wide availability of open green spaces, the implementation of a multifunctional park would contribute to the mitigation of floods that affect adjacent households and also mitigating floods downstream, as well as to support the creation of leisure spaces and encourage environmental awareness. Internally, the park incorporates walkable circuits for pedestrians and cyclists. Excavated material from the reservoirs is repurposed to create elevated paths and plazas, safeguarded from flooding impacts. This approach minimizes environmental disruption by utilizing excavated soil for embankment construction instead of moving it over long distances. Social and recreational amenities include a series of squares with urban furniture, recreational areas, and an observation deck offering views of the natural landscape and biodiversity.
The Marighella Park is located in the most critical flooding area of the watershed. Most importantly, this area surrounds the Carlos Marighella Housing Complex, built in 2015 under a federal social housing program for low-income populations. However, due to factors such as local geomorphology, mild slopes, soil type, low-lying buildings, and the proximity to local rivers, the housing complex occupies a naturally flood-prone area (Seabra and da Rocha-Leão, 2019). The availability of open spaces in the surrounding areas, especially to the east of the housing complex, allows the creation of a multifunctional park aimed at flood mitigation and community use. The proposed park features a set of reservoirs summing 165,672m3 in capacity. Excavation near the complex will create two multifunctional detention basins acting as temporary reservoirs and social leisure areas. Reservoirs in the eastern part of the park near Bambu/Taquaral/Vigário Rivers are designed to receive and store water during rainfall events. Additionally, a local street is proposed to be adapted to act as a levee to protect the housing complex from floods. The stored water in the detention basins return to the rivers through flap gates (one way flow). River restoration efforts include enhanced riparian vegetation and meander restoration. Marighella Park features pedestrian and cycle paths which should be connected to the other proposed parks to form a blue-green corridor and facilitate access.
Moving to the downstream segment, the third arch undertakes two major actions: restoring Brava Lagoon, a degraded water body which presently regulates Bambu River flows, and dredging the final stretch of the Bambu River near its outlet to Maricá Lagoon.
Brava Lagoon (Figure 7D) is recognized by the state environmental agency but is currently lost in the landscape, due to significant siltation reducing its water depth and altering its original form. Covering 4.4 km2, it is now primarily composed by humid lands and vegetation, in a typical wetland configuration. Its strategic location near major highways and commercial zones earmarks the area for potential urban expansion and a new administrative hub. Urban interventions, while benefiting established populations and urban fabric consolidation, could escalate environmental degradation, worsen local flooding, and hinder future solutions. Hence, the downstream arch’s conceptual project focuses on restoring and preserving the lagoon’s natural structure, recognizing its environmental and hydrological significance. The conceptual project had the Taquaral River as starting point, which crosses the original area of the Brava Lagoon. Objectives of this restoration include reinstating water bodies, reclaiming degraded areas, and creating a multifunctional landscape. Expanding the lagoon’s water surface area will alleviate current water level constraints, facilitating river discharge. The project proposal considers the premise of creating an interconnected system of lagoons, capable of not only accommodating water volumes, but creating a wetlands system that contributes to water treatment. The soil excavated in the process to restore the Brava Lagoon can be used to landfill areas to receive the new urban project related to the administrative center, ansead to build some artificial islands inside the lagoon that can act like ecological islands with phyto depurative vegetation capable of partially filtering the water and promoting local biodiversity.
However, this is not the basin’s final outlet. To ensure increased system discharge capacity, the stretch between Brava Lagoon and Maricá Lagoon must be dredged and expanded for efficient interconnection. Further measures encompass larger storm drains along east–west alignment (where local ponding occur, but there is no near river), and dredging the 14 km Costa Channel, facilitating additional system discharge directly to the sea, complementing the actions in the third arch.
Regarding the new administrative hub this new development should be conceived following concepts of a sponge city, preserving a network of green and blue spaces permeating this new development and adapting constructions to accommodate water presence, given the identified susceptibility on site. The multifunctional proposal also includes the creation of leisure areas and cycling paths to meet local demands.
Other actions like river dredging and widening are proposed to increase the discharging capacity of the basin outfall. To complement and integrate all the propositions in one joint and synergic action, a multifunctional ecological corridor is proposed. It aims to restore part of the watershed’s natural hydrological retention capacity, safeguard the river course from sedimentation, mitigate diffuse pollution from urban areas, and establish connectivity between existing conservation units and the lagoon along the river courses to ensure natural flows and support social uses.
5.3 Urban expansion estimation
The population of Maricá was estimated to be 167,668 inhabitants, by the Brazilian Institute of Geography and Statistics (IBGE), in the year of 2021. Based on this number, and considering the last census of 2010, a growth rate of 2% per year was considered, as foreseen by the 2020 Master Plan (Maricá, 2020), leading to an estimate of 212,664 inhabitants in 2033, calculated by arithmetic projection.
The population of the Bambu watershed represents 55.57% of the total current population of the Municipality, corresponding to 93,174 inhabitants in 2021, 96,939 inhabitants in 2023, and finally, 118,168 inhabitants in 2033. Therefore, the population will grow by 21,229 inhabitants between 2023 and 2033. This growth was distributed across the watershed, focusing on filling empty lots in already urbanized areas, leading to a preferential densification. Occupation in flood-prone and environmentally sensitive areas, represented by the HIA, was avoided.
5.4 Simulation and assessment
The Bambu Watershed model was designed using MODCEL and calibrated based on precipitation data from an event that occurred on February 29th, 2016, along with information about flooding locations during that event. For the evaluation, the simulations considered a 25-year return period rainfall event, which is the standard reference for Brazilian macro drainage projects (Government of Brazil, 2004). The water levels representing the restriction conditions in the downstream lagoon and sea (0.71 m and 0.60 m, respectively, related to high tide conditions) were represented by boundary conditions. Upstream boundary conditions were not necessary, since the model perform hydrologic rainfall-runoff transformation and encompasses the whole watershed. The evaluation parameter is the maximum water depth computed in each modeled flow-cell during the reference event.
Figure 8 represents the outcome of the hydrodynamic simulation, displaying the water depths in each cell in all the evaluated scenarios. From top to bottom, left to right, the first image (Figure 8A) shows the current situation, the second (Figure 8B) represents the expansion scenario, considering proper land use control in an optimistic approach, the third (Figure 8C) corresponds to the design alternative, modeled based on the urban expansion scenario, and finally the last image (Figure 8D) emphasizes the difference in water depths between the urban expansion scenario and the design alternative scenario, highlighting the efficacy of the project framework concept in reversing and mitigating floods. In Figures 8A,B,C, the water depths are represented in a blue gradient where the darkest shades represent higher values. The water depths difference is represented in a green gradient, where the darkest shades represent a greater decrease in water depths. In the design alternative scenario, river system does not overflow, and the remaining flooding spots are majorly related to minor drainage deficiencies and local problems.
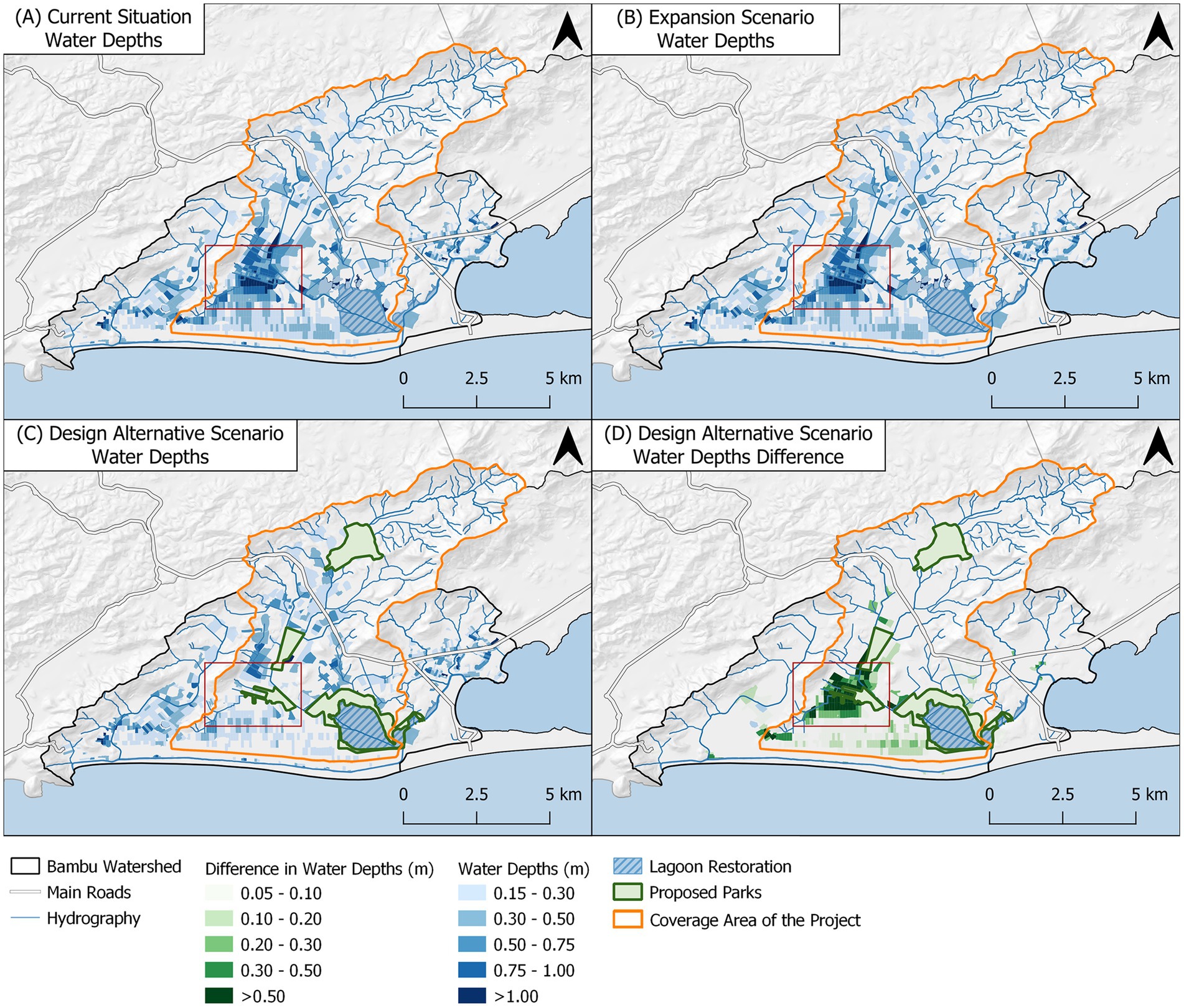
Figure 8. (A) Water depths for the current situation (B) Water depths for the urban expansion scenario (C) Water depths for the design alternative scenario (D) Water depths difference between the design alternative and the urban expansion scenarios.
Comparing Figures 8A,B, there was no significant increase in the water depths despite the population growth, demonstrating the effectiveness of controlled urban planning in flood management and underscoring the impact of proper land use, since flooding is expected to intensify when there is an increase in urbanization. In Figure 8C, which incorporates the major drainage projects into the urban open spaces in the HIA, considering the expansion scenario, the water depths are considerably lower, especially downstream in Itaipuaçu—a current critical flooding area.
The upstream reservoirs in the first arch, can significantly attenuate peak flows post-dam implementation, reducing flow from 18 m3/s to 5 m3/s in Reservoir 1 and from 12m3/s to 5m3/s in Reservoir 2, thereby mitigating downstream flooding in the more densely populated areas. Channel stretching and dredging have also effectively increased discharge capacity.
Consequently, there was a significant reduction in flood exposure. Three thresholds were established to classify potential damage: water depths over 0.15 m, which can surpass curbs and disrupt traffic; water depths over 0.30 m, which can enter buildings; and water depths greater than 1 m, with the potential of causing significant building damages both to contents and structures. In the current scenario, 33 km of roads—including highways, arterial and collector streets—are exposed to floods over 0.15 m, increasing to 35 km in the expansion scenario. In the design alternative scenario, the exposure decreases to 25 km. Regarding the building damages, over 8,000 are exposed to floods exceeding 0.30 m in both the current and expansion scenarios, but this number drops to around 3,700 in the design alternative scenarios. Finally, for major damages, 353 are buildings exposed to floods over 1.00 m in the current situation, 363 in the expansion scenario and only 2 after the project implementation. It should be noted that the project’s area of influence refers only to the main course of the Bambu River, not encompassing some peripheral areas of the watershed. Therefore, the results refer to the coverage area of the project.
The red-highlighted area represents the watershed’s most critical region, the Carlos Marighella Housing Complex and its surroundings. Currently, floods in this area can reach depths up to 1.37 m, projected to increase to 1.40 in the urban expansion scenario. Proposed interventions introduce significant changes: the majority of the urbanized area surrounding the new park experiences water depths below 0.15 m, posing minimal threat, as it is unlikely to reach the curb and affect the sidewalks. Therefore, the most critical region, in both current and urban expansion scenarios, also sees the most significant reduction in flood risks post-project implementation.
6 Discussion
The proposed methodology for defining a HIA assists in understanding the physical aspects of the terrain, enabling the understanding of stormwater behavior in the watershed and mapping ecologically significant areas. This definition provides a backdrop for interventions, serving as a robust foundation for controlling urban expansion and proposing projects. Adding new criteria to the legally protected areas for new urban zoning is significant, as there are ecologically important areas scattered throughout the watershed, beyond the large upstream green areas or riverbanks defined by law. Thus, the proposed procedure was able to build an interconnected network of green and blue elements distributed across the city.
Legally protected areas focus on upstream regions and river corridors. However, these typically cover only a fixed width based on river size—according to the Brazilian law, which is taken as an example of this study. The proposed HIA incorporates additional physical characteristics of the terrain to more precisely map potential river overflow areas, emphasizing slope as a critical factor for flood susceptibility. By incorporating the Riverine Flood-prone Region criterion on top of the already existing marginal buffer strips (PPAs), larger areas connected to waterbodies are protected. Note that, even though the PPAs are fully protected by law, and should be preserved where still unoccupied, in the mapped Potential Overflow Region urban occupation is permitted, but conditioned.
Downstream, incorporating low-elevation areas in the HIA was essential due to their increased susceptibility to flooding, especially in coastal regions where sea level variations significantly impact water accumulation. Furthermore, the threat of potential sea-level rise due to climate change underscores the importance of considering lowlands as part of the HIA. This criterion is crucial for ensuring long-term safety and sustainability in urban development strategies.
Mapping low-slope open spaces identifies not only flood-prone regions but also potential sites for interventions. This is because these areas naturally accumulate water, therefore are suitable for constructing reservoirs. These could be upstream retention reservoirs (like the ones in Inoã-North Park) or excavation reservoirs in multifunctional parks (illustrated in this study as Inoã Park and Marighella Park). Protecting these areas prevents settlement in flood-prone zones and provides solutions for urban flood issues if such designs are implemented.
Once the HIA is defined, the proposed method interprets it using the three spatial arches concept, enabling design strategies suitable for the upstream, middle, and downstream reaches of the drainage system. This approach integrates fluvial responses in a safer manner. Maricá’s amphitheater-like topography, with its central lagoon system, clearly illustrates the three intervention arches, serving as a didactic example for applying this framework.
The first arch focuses on protecting upstream natural areas—preserving its abundant green spaces - and the downstream valley from flooding, using specific open spaces to create urban flood control reservoirs. At the same time, given that the arch is bordered by environmental protected areas and rural regions, with reduced urban occupation, the immediate surroundings characteristics must be preserved to conserve both permeable spaces and the water quality of the river sources, allowing the well-functioning of the hydrological cycle.
In the mid-watershed arch, benefits of using remaining open spaces in the urban context are explored, aiming at preventing future densification and providing local storage volumes for the increased runoff generated by the urbanization itself. In fact, both selected areas, located at the confluence of rivers, are naturally floodable regions that should have more restricted policies for land occupation. However, there is an urban manage gap related to the sprawl control, given that a large number of buildings are located in wetlands or floodplains areas - this is the situation of the Carlos Marighella Housing Complex.
Therefore, the conception of Marighella and Inoã Parks aim not only to protect people in socially vulnerable situations from flooding risks, but also to provide a better quality of life to the urban population. This idealization is particularly important, given that, in developing countries, the planning of leisure areas, such as urban parks, is commonly associated with wealthier backgrounds, leading to an uneven distribution of high-quality green spaces throughout cities. Thus, the “integrate” concept, in the core of this arch, discusses the role of these solutions in connecting the natural and the urban environment’s demands, while also integrates people with their surroundings.
In the downstream arch, the concern regarding the inertia of the system was addressed by the conceptual project aiming to restore the Brava Lagoon, which lost its natural set-up during the urbanization process. From the restoration of its water surface area, fluvial discharges can reach these areas with less constraints and find a new storage area. At the same time, in view of the development of a new administrative center foreseen to this area, the concept of “sponge city” was suggested to promote a more sustainable and integrated urban development in the future urban occupation. In other words, although the downstream arch is located in the most densely populated region of the territory, the concept of “respect” deals with efforts aimed at avoiding irregular occupation and urban sprawl in areas of hydrological interest and to guarantee a more efficient passage of the fluvial discharges to their final outflow, without compromising downstream occupations (if they exist). In this case, accelerating discharges has no consequences, since the final receiving water body is a coastal lagoon connected to the sea.
In the design alternative conception, the river is taken as the main link between the three spatial arches and co-related proposed solutions, creating a blue-green axis where all the interactions take place. From upstream to downstream, the rivers can promote the ecological connectivity between parks and other green areas, as well as perform the system hydrodynamic, while allowing easy access for residents and visitors. Therefore, BGI play a key role in planning more sustainable and water sensitive cities, in which the detention basins (conceived as multifunctional parks) were the preferred typology for improving flood control in the immediate surroundings of urban areas, using the available urban open spaces organized in a functional system.
Even though the evaluation of this design alternative was mainly focused on flood reduction, it is worth pointing out that additional benefits are clearly offered, even if they were not quantified in this work. In developing areas, where open spaces usually decrease with the urban growth, it is fundamental that they accumulate more than one function, avoiding idleness—especially those downstream, in places pressed for urban growth. Some examples, regarding social functions, are increased quality of life from constant contact with the natural environment, aesthetic and cultural value, recreational opportunities and increasing the real estate value of the homes located around the parks. It is also worth mentioning the increase in biodiversity, promotion of water quality, carbon sequestration, urban cooling, among other environmental improvements, showing an excellent alignment of the proposal with the concept of Nature-based Solutions.
A possible weak point in this solution refers to the sewage system failures. In this sense it is fundamental that the design presented in this work follows measures that ensure proper sewage collection and defense against combined sewer overflows, when both stormwaters and wastewaters flow together, so that these spaces do not become a source of waterborne diseases.
7 Conclusion
This research aimed to develop and apply a conceptual framework for systematically integrating drainage solutions into the urban open space system to mitigate the adverse effects of urban flooding. The initial step involved defining a Hydrological Interest Area (HIA) to structure urban planning with a focus on stormwater management. The resulting mapping displays environmentally significant areas that should either be preserved or subjected to controlled occupation to achieve flood mitigation.
Then, the watershed was decomposed as a sum of three spatial arches, from upstream to downstream, interpretating its functional demands. The upper arch intends to protect the natural environment, usually associated with vegetated hillslopes and dampen discharges to avoid overflows in the middle and downstream reaches of the watershed. The middle arch, in moderate and plain terrain slopes, where urbanization tend to occur more intensely, is the proper stage to integrate urban and natural needs, improving the built environment quality, while using the urban open space system to project multifunctional floodable parks. The downstream arch refers to allowing discharges outflow, favoring the system emptying (since it does not transfer floods). The fluvial corridor connects all these arches.
Future land use could be adequate planned, leading to a low impact development on flood behavior, prioritizing non occupied lots to settle population growth. The HIA was used to drive the new occupation ordering, and to support decision of better places to receive flood mitigation interventions, based on stormwater accumulating. The future system design, which considers both land use planning and structural flood hazard reduction measures, could reduce flood risk, by avoiding new exposures and reducing flooding water depths. Flooding mapping shows a significant reduction followed by a decrease in exposure of buildings and roads, especially in the most critical region of the watershed.
The watershed chosen for the application of the framework is characterized by large environmentally relevant areas (both composed by forests and water bodies) and by its rapidly urban developing context. In such contexts, the systemic thinking, in which urban drainage is considered one of the key elements for structuring the territory that will receive functional cities, should be considered as a way of avoiding the densification of relevant areas to the hydrological cycle. This framework can be used in similar contexts where the increasing urban sprawl is threatening the well-functioning of ecosystem services, as well as the city welfare. Acting in advance is more effective than reversing the consequences caused by inadequate urban growth. This approach reflects the need to look at land use in a multifunctional way, where multiple systems can coexist and generate social, hydraulic and environmental benefits for the population.
Future works regarding this method should consider its application, behavior and validation to watersheds in different stages of urban development. In particular, it is recommended to test this proposition in dense core city areas, as well as in areas with initial urban occupation, mapping the limitations and opportunities that can arise from both situations.
Finally, this conceptual framework does not intend to limit or avoid urban development, but to show structured alternative ways of maintaining a harmonic relation between the natural and built environments.
Data availability statement
The raw data supporting the conclusions of this article will be made available by the authors, without undue reservation.
Author contributions
MM: Conceptualization, Formal analysis, Investigation, Methodology, Project administration, Writing – original draft, Writing – review & editing. MG: Investigation, Writing – original draft. BA: Methodology, Writing – original draft. HM: Validation, Writing – original draft. FT: Writing – original draft, Investigation, Supervision. RM: Conceptualization, Writing – original draft, Formal analysis, Investigation. AV: Writing – original draft, Conceptualization, Project administration, Supervision. MS: Validation, Writing – original draft. OR: Conceptualization, Project administration, Writing – review & editing. PM: Conceptualization, Project administration, Writing – original draft.
Funding
The author(s) declare that financial support was received for the research, authorship, and/or publication of this article. The Brazilian research council, Conselho Nacional de Desenvolvimento Científico e Tecnológico (CNPq) (Grant Number: 303862/2020-3), along with the Coordenação de Aperfeiçoamento de Pessoal de Nível Superior (CAPES) (Grant Number: Finance Code 001; 88887.805756/2023-00) and the Fundação Carlos Chagas Filho de Amparo à Pesquisa do Estado do Rio de Janeiro (FAPERJ) (Grant Number: E-26/201.404/2021(26079)), provided research scholarships. CAPES is also responsible for funding the postgraduate programs in which the authors of this manuscript conduct their research.
Acknowledgments
We acknowledge the valuable contributions of Companhia de Desenvolvimento de Maricá (CODEMAR), and the Serviços de Obras de Maricá (SOMAR) to this research project; we also acknowledge to the UNESCO Chair on Urban Drainage in Regions of Coastal Lowlands settled at the Universidade Federal do Rio de Janeiro, to which this research is linked.
Conflict of interest
The authors declare that the research was conducted in the absence of any commercial or financial relationships that could be construed as a potential conflict of interest.
Publisher’s note
All claims expressed in this article are solely those of the authors and do not necessarily represent those of their affiliated organizations, or those of the publisher, the editors and the reviewers. Any product that may be evaluated in this article, or claim that may be made by its manufacturer, is not guaranteed or endorsed by the publisher.
References
Ahern, J. (2013). Urban landscape sustainability and resilience: the promise and challenges of integrating ecology with urban planning and design. Landsc. Ecol. 28, 1203–1212. doi: 10.1007/s10980-012-9799-z
Ahmed, S., Meenar, M., and Alam, A. (2019). Designing a blue-green infrastructure (BGI) network: toward water-sensitive urban growth planning in Dhaka, Bangladesh. Land 8:4. doi: 10.3390/land8090138
Ambily, P., Chithra, N. R., and Mohammed, F. C. (2024). A framework for urban pluvial flood resilient spatial planning through blue-green infrastructure. Int. J. Disaster Risk Reduct. 103:104342. doi: 10.1016/j.ijdrr.2024.104342
Avazpour, B., Osmond, P., and Corkery, L. (2023). The dynamic challenges of mainstreaming water sensitive cities in our built environment: lessons from Australia. Cities 143:104615. doi: 10.1016/j.cities.2023.104615
Babí Almenar, J., Elliot, T., Rugani, B., Philippe, B., Navarrete Gutierrez, T., Sonnemann, G., et al. (2021). Nexus between nature-based solutions, ecosystem services and urban challenges. Land Use Policy 100:104898. doi: 10.1016/j.landusepol.2020.104898
Barbaro, G., Miguez, M. G., de Sousa, M. M., da Cruz Franco, A. B. R., de Magalhães, P. M. C., Foti, G., et al. (2021). Innovations in best practices: approaches to managing urban areas and reducing flood risk in Reggio Calabria (Italy). Sustainability 13, 1–2. doi: 10.3390/su13063463
Battemarco, B. P., Tardin-Coelho, R., Veról, A. P., de Sousa, M. M., da Fontoura, C. V. T., Figueiredo-Cunha, J., et al. (2022). Water dynamics and blue-green infrastructure (BGI): towards risk management and strategic spatial planning guidelines. J. Clean. Prod. 333:129993. doi: 10.1016/j.jclepro.2021.129993
Benedict, M. A., and McMahon, E. T. (2006). Green infrastructure: Smart conservation for the 21st century. United States: Island Press.
Brom, P., Engemann, K., Breed, C., Pasgaard, M., Onaolapo, T., and Svenning, J. C. (2023). A decision support tool for green infrastructure planning in the face of rapid urbanization. Land 12:2. doi: 10.3390/land12020415
Bueno-Suárez, C., and Coq-Huelva, D. (2020). Sustaining what is unsustainable: a review of urban sprawl and urban socio-environmental policies in North America and Western Europe. Sustainability 12, 1–3. doi: 10.3390/su12114445
Cohen-Shacham, E., Andrade, A., Dalton, J., Dudley, N., Jones, M., Kumar, C., et al. (2019). Core principles for successfully implementing and upscaling nature-based solutions. Environ Sci Policy, Elsevier 98, 20–29. doi: 10.1016/j.envsci.2019.04.014
Dabas, R., Kumar, S., and Kumar, M. (2019). “Applications of low impact development for managing the storm water surface runoff in urban areas”, advances in civil engineering and infrastructural development: Select proceedings of ICRACEID 2019. Singapore: Springer Singapore, 275–283.
de Oliveira, A. K. B., Battemarco, B. P., Barbaro, G., Gomes, M. V. R., Cabral, F. M., de Oliveira Pereira Bezerra, R., et al. (2022). Evaluating the role of urban drainage flaws in triggering cascading effects on critical infrastructure, affecting urban resilience. Inf. Dent. 7:153. doi: 10.3390/infrastructures7110153
de Oliveira, A. K. B., Carneiro Alves, L. M., Carvalho, C. L., Haddad, A. N., de Magalhães, P. C., and Miguez, M. G. (2023). A framework for assessing flood risk responses of a densely urbanized watershed, to support urban planning decisions. Sustain. Resilient Infrastruct. 8, 400–418. doi: 10.1080/23789689.2023.2175139
European Union (1992). Council directive 92/43/EEC of 21 may 1992 on the conservation of natural habitats and of wild Fauna and Flora (habitats directive). Off. J. Eur. Communities.
Folch, R. (2002). “Los conceptos socioecológlcos de partida” in El Territorio Como Sistema: Conceptos y Herramientas de Ordenación (Barcelona: Diputación de Barcelona).
Ghofrani, Z., Sposito, V., and Faggian, R. (2017). A comprehensive review of blue-green infrastructure concepts. In.t J. Environ. Sustain. 6, 17–18. doi: 10.24102/ijes.v6i1.728
Gomes, M.V.R., Ferreira, G.F., Araújo, D.F.De, Mattos, R.R.De, Veról, A.P., Magalhães, P.CDe, et al. (2023). “Environmental protection areas as a strategy to increase flood protection in metropolitan regions: a case study in Maricá, Rio de Janeiro, Brazil”, E3S Web Conf. 407, 2–3. doi: 10.1051/e3sconf/202340703004
Government of Brazil . (2000), “National System of conservation units (SNUC). Law no. 9.985, of July 18, 2000.”
Government of Brazil (2004). Manual de Drenagem Urbana Sustentável. Brasília, Brazil: Ministério das Cidades.
Government of South Africa . (2003), National Environmental Management: Protected areas act 2003. Act no. 57 of 2003.
Graham, S., and Marvin, S. (2001). Splintering urbanism: Networked infrastructures, technological Mobilities and the urban condition. Routledge, London: Routledge.
Hamel, P., and Tan, L. (2022). Blue–green infrastructure for flood and water quality Management in Southeast Asia: evidence and knowledge gaps. Environ. Manag. 69, 699–718. doi: 10.1007/s00267-021-01467-w
Havránková, L., Štych, P., Ondr, P., Moravcová, J., and Sláma, J. (2023). Assessment of the connectivity and comfort of urban Rivers, a case study of the Czech Republic. Land 12, 1–20. doi: 10.3390/land12040814
Illich, I. (2005). “Energia e Equidade” in Apocalipse Motorizado: A Tirania Do Automóvel Em Um Planeta Poluído. ed. N. Ludd. 2nd ed (São Paulo: Conrad Editora do Brasil).
Jing, J., Zhang, Z., and Li, J. (2022). Study on location decision of multi-functional rainwater storage space in high-density built-up area. Water 14, 1–2. doi: 10.3390/w14213460
Kabisch, N., Stadler, J., Korn, H., and Bonn, A. (2016). Nature-based solutions to climate change mitigation and adaptation in urban areas. BfN Skript 446. Ecol. Soc. 21:39. doi: 10.1007/978-3-319-56091-5
Karami, M., Behzadian, K., Ardeshir, A., Hosseinzadeh, A., and Kapelan, Z. (2022). A multi-criteria risk-based approach for optimal planning of SuDS solutions in urban flood management. Urban Water J. 19, 1066–1079. doi: 10.1080/1573062X.2022.2117632
Kim, H., Lee, D. K., and Sung, S. (2016). Effect of urban green spaces and flooded area type on flooding probability. Sustainability 8:2. doi: 10.3390/su8020134
Kozak, D., Henderson, H., Mazarro, A. D. C., Rotbart, D., and Aradas, R. (2020). Blue-green infrastructure (BGI) in dense urban watersheds. The case of the Medrano stream basin (MSB) in Buenos Aires. Sustainability 12, 1–3. doi: 10.3390/su12062163
Krawchenko, T., and Schumann, A. (2023). “Land use planning systems in OECD countries: trends, practices, innovations, and reforms” in The Palgrave encyclopedia of urban and regional futures (Cham: Springer International Publishing), 965–971.
Lamas, J. M. R. G. (1993). Morfologia Urbana e Desenho Da Cidade. 2nd Edn. Lisboa: Fundação Calouste Gulbenkian.
Li, H., Ding, L., Ren, M., Li, C., and Wang, H. (2017). Sponge city construction in China: a survey of the challenges and opportunities. Water 9, 1–17. doi: 10.3390/w9090594
Lourenço, I. B., Guimarães, L. F., Alves, M. B., and Miguez, M. G. (2020). Land as a sustainable resource in city planning: the use of open spaces and drainage systems to structure environmental and urban needs. J. Clean. Prod. 276:123096. doi: 10.1016/j.jclepro.2020.123096
Lovell, S. T., and Taylor, J. R. (2013). Supplying urban ecosystem services through multifunctional green infrastructure in the United States. Landsc. Ecol. 28, 1447–1463. doi: 10.1007/s10980-013-9912-y
Macdonald, N., Chester, D., Sangster, H., Todd, B., and Hooke, J. (2012). Human adjustment to floods” in the development of risk and hazard management. Prog. Phys. Geo. 36, 125–133. doi: 10.1177/0309133311414607
Majidi, A. N., Vojinovic, Z., Alves, A., Weesakul, S., Sanchez, A., Boogaard, F., et al. (2019). Planning nature-based solutions for urban flood reduction and thermal comfort enhancement. Sustainability 11, 1–2. doi: 10.3390/su11226361
McGrane, S. J. (2016). Impacts of urbanisation on hydrological and water quality dynamics, and urban water management: a review. Hydrol. Sci. J., Taylor & Francis 61, 2295–2311. doi: 10.1080/02626667.2015.1128084
Meng, X. (2022). Understanding the effects of site-scale water-sensitive urban design (WSUD) in the urban water cycle: a review. Blue Green Syst. 4, 45–57. doi: 10.2166/bgs.2022.026
Miguez, M. G., Battemarco, B. P., De Sousa, M. M., Rezende, O. M., Veról, A. P., and Gusmaroli, G. (2017). Urban flood simulation using MODCEL-an alternative quasi-2D conceptual model. Water, MDPI AG 9, 6–26. doi: 10.3390/w9060445
Miguez, M. G., Radesca, F. D., Veról, A. P., Sousa, M. M., and Oliveira, L. F. G. S. (2019). Multilayer modelling as a supporting tool for flood diagnosis and drainage system design. New Trends Urban Drain. Model, 490–495. doi: 10.1007/978-3-319-99867-1_84
Miguez, M. G., Rezende, O. M., and Veról, A. P. (2015). City growth and urban drainage alternatives: sustainability challenge. J. Urban Plan. Dev. 141:04014026. doi: 10.1061/(ASCE)UP.1943-5444.0000219
Miranda, F., Franco, A.B., Rezende, O., Costa, B.B.F.da, Najjar, M., Haddad, A.N., et al. (2023). “A GIS-based index of physical susceptibility to flooding as a tool for flood risk management”, Land 12:1408, doi: 10.3390/LAND12071408
Molné, F., Donati, G. F. A., Bolliger, J., Fischer, M., Maurer, M., and Bach, P. M. (2023). Supporting the planning of urban blue-green infrastructure for biodiversity: a multi-scale prioritisation framework. J. Environ. Manag. 342:118069. doi: 10.1016/j.jenvman.2023.118069
Monteiro, R., Ferreira, J. C., and Antunes, P. (2020). Green infrastructure planning principles: an integrated literature review. Land 9, 1–19. doi: 10.3390/land9120525
Moura Rezende, O., Ribeiro da Cruz de Franco, A. B., Beleño de Oliveira, A. K., Pitzer Jacob, A. C., and Gomes Miguez, M. (2019). A framework to introduce urban flood resilience into the design of flood control alternatives. J. Hydrol. 576, 478–493. doi: 10.1016/j.jhydrol.2019.06.063
Mullenbach, L. E., Baker, B. L., Hickerson, B., and Mowen, A. J. (2019). Assessing the relationship between community engagement and perceived ownership of an urban park in Philadelphia. J. Leis. Res. 50, 201–219. doi: 10.1080/00222216.2019.1581719
O’Donnell, E. C., Netusil, N. R., Chan, F. K. S., Dolman, N. J., and Gosling, S. N. (2021). International perceptions of urban blue-green infrastructure: a comparison across four cities. Water 13, 20–30. doi: 10.3390/w13040544
O’Donnell, E., Thorne, C., Ahilan, S., Arthur, S., Birkinshaw, S., Butler, D., et al. (2020). The blue-green path to urban flood resilience. Blue Green Syst. 2, 28–45. doi: 10.2166/bgs.2019.199
Peng, X., Heng, X., Li, Q., Li, J., and Yu, K. (2022). From sponge cities to sponge watersheds: enhancing flood resilience in the Sishui River basin in Zhengzhou, China. Water 14, 3–9. doi: 10.3390/w14193084
Pérez-Montiel, J. I., Cardenas-Mercado, L., and Nardini, A. G. C. (2022). Flood modeling in a coastal town in northern Colombia: comparing MODCEL vs. IBER. Water 14, 12–21. doi: 10.3390/w14233866
Qi, Y., Chan, F. K. S., O’Donnell, E. C., Feng, M., Sang, Y., Thorne, C. R., et al. (2021). Exploring the development of the Sponge City program (SCP): the case of Gui’an New District, Southwest China. Front. Water 3, 1–17. doi: 10.3389/frwa.2021.676965
Qiao, Y., Mengjiao, D., Haochen, L., Xizi, T., and Xiaoyan, L. (2022). Research on the integrated planning of blue-green space towards urban-rural resilience: conceptual framework and practicable approach. J. Res. Ecol. 13, 347–359. doi: 10.5814/j.issn.1674-764x.2022.03.001
Richter, M., Heinemann, K., Meiser, N., and Dickhaut, W. (2024). Trees in sponge cities—a systematic review of trees as a component of blue-green infrastructure, vegetation engineering principles, and Stormwater management. Water 16:1. doi: 10.3390/w16050655
Rodríguez-Rojas, M. I., Garrido-Jiménez, F. J., Abarca-Álvarez, F. J., and Vallecillos-Siles, M. R. (2024). Advances in the integration of sustainable drainage systems into urban planning: a case study. Sustainability 16, 1–3. doi: 10.3390/su16072658
Roggema, R., Tillie, N., and Keeffe, G. (2021). Nature-based urbanization: scan opportunities, determine directions and create inspiring ecologies. Land 10, 1–3. doi: 10.3390/land10060651
Scionti, F., Miguez, M. G., Barbaro, G., De Sousa, M. M., Foti, G., and Canale, C. (2018). Integrated methodology for urban flood risk mitigation in Cittanova, Italy. J. Water Resour. Plan. Manag. 144, 5–9. doi: 10.1061/(asce)wr.1943-5452.0000985
Seabra, V. S., and da Rocha-Leão, O. M. (2019). Razões para as enchentes e inundações no Residencial Carlos Marighella: Uma análise multitemática da bacia do rio Vigário, em Maricá-RJ. Revista Da Anpege 15, 114–137. doi: 10.5418/RA2019.1526.0005
Sharma, A. K., Pezzaniti, D., Myers, B., Cook, S., Tjandraatmadja, G., Chacko, P., et al. (2016). Water sensitive urban design: an investigation of current systems, implementation drivers, community perceptions and potential to supplement urban water services. Water 8, 2–3. doi: 10.3390/w8070272
Sowińska-Świerkosz, B., and García, J. (2022). What are nature-based solutions (NBS)? Setting core ideas for concept clarification. Nat. Based Sol. :100009. doi: 10.1016/j.nbsj.2022.100009
Tardin, R. (2013). System of open spaces: Concrete project strategies for urban territories. System of open spaces: Concrete project strategies for urban territories. New York: Springer New York.
Thorne, C. R., Lawson, E. C., Ozawa, C., Hamlin, S. L., and Smith, L. A. (2015). Overcoming uncertainty and barriers to adoption of blue-green infrastructure for urban flood risk management. J. Flood Risk Manag. 11, S960–S972. doi: 10.1111/jfr3.12218
United States Congress . (1916), “National Park Service organic act”, U.S. Code, Title 16, Chapter 1, Section 1. Available at: https://www.nps.gov/gate/learn/management/nps-organic-act-of-1916.htm
Veról, A. P., Lourenço, I. B., Fraga, J. P. R., Battemarco, B. P., Merlo, M. L., de Magalhães, P. C., et al. (2020). River restoration integrated with sustainable urban water management for resilient cities. Sustainability 12, 1–36. doi: 10.3390/su12114677
Veselinović, G., Štrbac, S., Antić, N., Ferreira, C. S. S., Dincă, L. C., Mijatović, N., et al. (2023). Connectivity approach in urban protected area management based on soil and vegetation chemical status. Environ. Geochem. Health 45, 9525–9540. doi: 10.1007/s10653-023-01553-4
Waldheim, C. (2016). Landscape as urbanism: A general theory. New Jersey: Princeton University Press.
Wang, M., Feng, S., Ikram, R. M. A., Chen, T., Sun, C., Chen, B., et al. (2023). Assessing the performance and challenges of low-impact development under climate change: a bibliometric review. Sustainability 15, 1–19. doi: 10.3390/su151813616
Wang, J., and Foley, K. (2023). Promoting climate-resilient cities: developing an attitudinal analytical framework for understanding the relationship between humans and blue-green infrastructure. Environ Sci Policy 146, 133–143. doi: 10.1016/j.envsci.2023.05.010
White, G. F. (1945). Human Ajustment to floods: a geographical aproach to the flood problem in the United States. In: Research paper no. 29. Eds. T. U. O. Chicago. Chicago: The University of Chicago, pp. 11–238.
Xia, H., Yin, R., Xia, T., Zhao, B., and Qiu, B. (2024). People-oriented: a framework for evaluating the level of green space provision in the life circle from a supply and demand perspective: a case study of Gulou District, Nanjing, China. Sustainability 16, 1–2. doi: 10.3390/su16030955
Xie, L., and Bulkeley, H. (2020). Nature-based solutions for urban biodiversity governance. Environ Sci Policy, Elsevier 110, 77–87. doi: 10.1016/j.envsci.2020.04.002
Keywords: conceptual framework, open space system, flood mitigation, multifunctional landscapes, hydrological interest area
Citation: Miguez MG, Gomes MVR, Amback BC, Mello Neto Hd, Thomaz FR, Mattos RRd, Veról AP, Sousa MMd, Rezende OM and de Magalhães PC (2024) Conceptual framework to incorporate drainage solutions in the urban open space system. Front. Water. 6:1468975. doi: 10.3389/frwa.2024.1468975
Edited by:
Kshama Gupta, Indian Institute of Remote Sensing, IndiaReviewed by:
Harsimran Kaur, Indian Institute of Technology (Banaras Hindu University) Varanasi, IndiaSatish Pipralia, Malaviya National Institute of Technology, Jaipur, India
Copyright © 2024 Miguez, Gomes, Amback, Mello Neto, Thomaz, Mattos, Veról, Sousa, Rezende and de Magalhães. This is an open-access article distributed under the terms of the Creative Commons Attribution License (CC BY). The use, distribution or reproduction in other forums is permitted, provided the original author(s) and the copyright owner(s) are credited and that the original publication in this journal is cited, in accordance with accepted academic practice. No use, distribution or reproduction is permitted which does not comply with these terms.
*Correspondence: Marcelo Gomes Miguez, bWFyY2Vsb21pZ3VlekBwb2xpLnVmcmouYnI=