- 1Department of Meteorology and Climatology, Taras Shevchenko National University of Kyiv, Kyiv, Ukraine
- 2Chair of Hydrology and Climatology, University of Potsdam, Potsdam, Germany
- 3Research Department II: Climate Resilience, Potsdam Institute for Climate Impact Research, Potsdam, Germany
- 4Institute of Water Problems and Land Reclamation of NAAS, Kyiv, Ukraine
This article focuses on water security in Mykolaiv, a city of 0.5 million inhabitants in southern Ukraine, in the situation of scarcity of usable water resources caused by climate change and military operations. This problem arose after the Dnipro-Mykolaiv water pipeline was destroyed in April 2022 as a result of military operations and the supply of drinking water to the city was cut off. To ensure that the city’s population has constant access to sufficient water of acceptable quality, a search for alternative water sources and a climate risk assessment were carried out for the new municipal water supply system from the Southern Bug River. The possible change in flow and its intra-annual distribution under the influence of climate change was modeled using the WaterGAP2 hydrological model and climate projections under the SSP1-2.6 and SSP5-P8.5 scenarios. It was found that under the SSP1-2.6 scenario, the reduction in river flow will be insignificant (up to a maximum of 14% in the far future) and there will be no restrictions on the city’s water supply from this section of the river in the near (2021-2050) and far (2051-2080) period. The maximum water withdrawal for municipal water supply and the minimum environmental flow will reach their maximum value only in August (56% of the projected flow), which is not critical. Under the SSP5-8.5 scenario, in the long-term perspective of 2051-2080, the largest decrease in runoff will occur from May to October, and the water withdrawal will increase to 40-79% of the projected flow. The use of the research results not only in water management, but also in municipal administration, and their dissemination in territorial communities will contribute to the successful adaptation of socio-economic and environmental processes in the region and can bring successful benefits not only to the economy, but also to communities.
1 Introduction
UN Water (2013) defines water security as the ability of the population to guarantee sustainable access to sufficient water of acceptable quality for livelihoods, human well-being and socio-economic development, to ensure protection from water-related pollution and disasters, and to preserve ecosystems in peace and political stability.
Gunda et al. (2019) have noted that most water security studies (Gain et al., 2016; Tal, 2006; Vogel et al., 2015) focus on the quantity of water, without delving into the assessment of water quality and its suitability for meeting the basic needs of society, which are defined by the very concept of water security.
Very often the quantity of available water can be of poor quality. Water quality refers to its suitability for various uses and the health of aquatic ecosystems. Water quality has a significant impact on water supply and often determines supply options (Guidelines for drinking-water quality, 2017). Surface and groundwater within the Black Sea Lowland in southern Ukraine (where the city of Mykolaiv is located) or in the Azov Sea region is unsuitable for drinking water supply or irrigation. The poor quality of these waters is due to the high content of various salts and high salinity of up to 3,000 mg/L or more (Khilchevskyi et al., 2018; Shestopalov et al., 2019).
The poor quality of water resources, combined with the reduction of water available for consumption due to climate change in many regions of the world, causes water stress and significantly weakens water security. In recent decades, climate change has become a serious threat to water security, especially in arid and semi-arid zones. Observed changes in temperature, rainfall, and evapotranspiration over recent decades have already affected dryland extent and water security in many areas of the world, including Ukraine (Khokhlov and Yermolenko, 2013; Pokhrel et al., 2021; Semenova et al., 2015; Semenova and Vicente-Serrano, 2024; Shevchenko et al., 2022; Stringer et al., 2021; Quandt et al., 2022).
Water security can also depend on the degree of anthropogenic modification of the river ecosystem, in particular the degree of water flow regulation by numerous dams, which can lead to a decrease in natural water flow, deterioration of water quality, and pose a threat to human well-being (Ekka et al., 2020; McCully, 1996; Poff et al., 1997; Renöfält et al., 2010; Sarker, 2021; Singhal et al., 2024; Yuan et al., 2022).
Another problem related to water security is political instability and wars. Water can be used as a weapon or as a target of armed conflict (Gleick, 1993, 2019).
If water is used as a weapon, it can lead to the complete destruction of water infrastructure and the water security system (Gleick et al., 2023; Snizhko et al., 2023a; Vyshnevskyi et al., 2023; Vyshnevskyi and Kutsiy, 2024).
The combination of all the above factors determines the water security of the region under study in southern Ukraine. In the semi-arid regions of southern Ukraine, the water stress index (Falkenmark et al., 2019) according to the World Resources Institute (2024) was already very high before the start of the Russian-Ukrainian war, reaching 40–80%, indicating a low level of water security.
Since the beginning of the war, water security in the region has deteriorated dramatically, as Russian troops have repeatedly used water as a weapon by destroying critical water infrastructure.
On April 12, 2022, as part of the attack of the Russian forces against Ukraine, the drinking water supply source (or intake) of Mykolaiv was destroyed and, since then, the city with a population of 470,000 people (01.01.2022) (State Statistics Service of Ukraine, 2022) has had no sustainable water supply (Figure 1A).
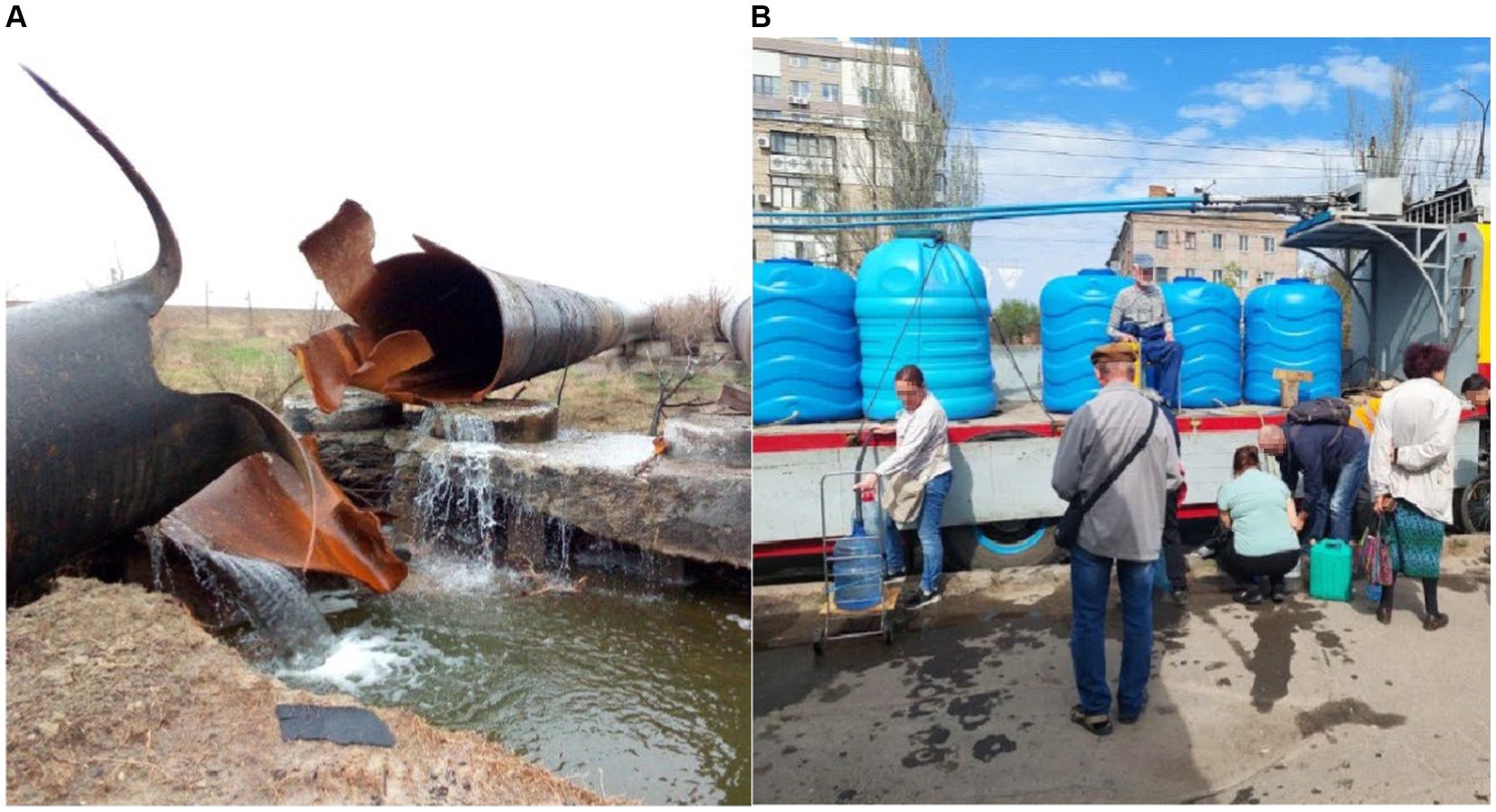
Figure 1. The destroyed water pipeline Dnipro-Mykolaiv (A) and delivery of water by road transport from other territories of Ukraine (B). Photo from texty.org.ua (2022).
As a temporary solution, dozens of clean water points were established with water provided by neighboring cities (Figure 1B) or international aid agencies (The Washington Post, 2022) and organized water abstraction from the downstream part of the Southern Bug River near the city. Nevertheless, due to the high salinity in the lagoon (South Bug River), the abstracted water could be used only for technical purposes. Before the attack, the water supply system was mainly carried out by abstracting water from the Dnipro River near Kherson city and transferring it by two pipelines 73 km long (Figure 2) to Mykolaiv (Mozgoviy, 2021; NPR, 2022).
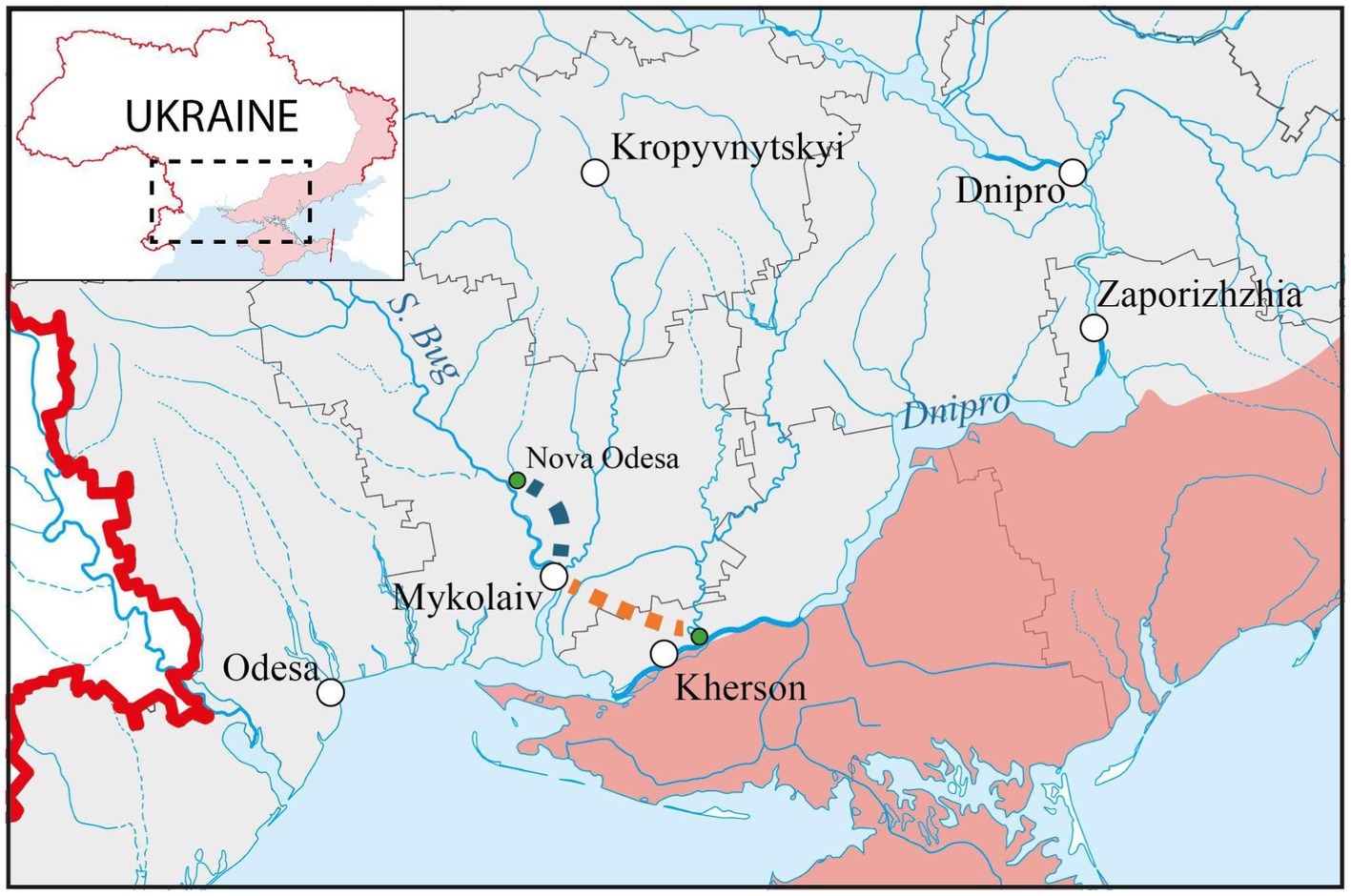
Figure 2. The possible freshwater sources for Mykolaiv City. Red area—occupied territory of Ukraine; orange line—schematic illustration of the former freshwater pipeline; dark green line—schematic illustration of the alternative freshwater pipeline; green circle—water abstraction sites.
At this moment, there is no possibility of repairing the water pipelines because they are located close to the frontline within the area of Russian artillery strikes (Figure 2). In addition, the territory has to be de-mined before the engineers can fix the damaged sections (BBC, 2022; NPR, 2022; The Washington Post, 2022).
An alternative solution for a sustainable water source for Mykolaiv could be an abstraction of water in the upper part of the Southern Bug River near Nova Odesa (Figure 2) and build a new water pipeline to the city. The city is located in a semi-arid climate zone, where the limited amount of water resources is already an issue, and climate change may put additional pressure on future water availability in the region. The region is already affected by climate change. Since investments in water management have been planned over decades, the water strategy must take climate change into account, especially the risk of prolonged droughts. What is needed is science-based decision support.
Decision-making should be based on the study of all available information on the region’s water resources, on the use of all available databases on water quantity and quality, on the study of the hydrological regime of water bodies and the operation of water management facilities, on the results of hydrological modeling using several climate projection to reduce the uncertainty of modeling results and ensure sustainable water security in the future. It should be consistent with the basic principles of Integrated Water Resources Management (IWRM).
The main objective of the research is to select an alternative water supply source based on hydrological and climatological studies to guarantee sustainable access to drinking water for the population of Mykolaiv, taking into account the water management needs of the region and the stability of the ecosystems of the lower part of the Southern Bug basin. To accomplish this task, we performed an analysis of potential water supply sources. The hypothesis was tested that groundwater, brackish water from the Bug estuary surrounding the city of Mykolaiv, and river water from the Southern Bug River upstream of the city were suitable for the new water supply system in terms of quantitative and qualitative indicators.
The current state of water resources of the Southern Bug River as the most suitable source of water supply was investigated, and the long-term variability and intra-annual distribution of the river’s water flow was studied.
The final task of the research was to assess the climate risk to the water security of Mykolaiv at least for the near term (2021–2050) and (optionally) for the long term period (2051–2080) by analyzing the projections of water flow, water balance parameters and environmental flow in the lower section of the river. To achieve this goal the mean annual and intra-annual river discharge for two projected periods was modeled using the global hydrological model (GHM) WaterGAP2 under two socio-economic scenarios SSP1-RCP2.6 (minimum risks to water resources) and SSP5-RCP8.5 (maximum risks to water resources). To simulate the future water flow of the river, climate data from five Global Climate Models (GCMs) were used, which were bias-corrected to reduce inherent simulation uncertainties and errors yielding the “CMIP6 climate forcing.”
2 Materials and methods
2.1 Analysis of potential water supply sources for Mykolaiv
Before the destruction of the Kherson-Mykolaiv water pipeline, Mykolaiv’s domestic water supply came from the Dnipro River. Groundwater was also a source of water supply for the city and surrounding areas, but due to pollution and increased salinity, it became less important over time. In 2020, the enterprise “Mykolaivvodokanal” provided 44.6 million m3 of water annually from the Dnipro River to Mykolaiv (Ministry of Development of Communities and Territories of Ukraine, 2021; Mykolaiv Regional Council, 2021). The water supply was destroyed during war in 2021–2022. The city of Mykolaiv is supplied with water through a 73 km-long pipeline that runs from the Dnipro River. The intake point is located in the village of Mykilske, in the Kherson region, below the dam of the Kakhovka hydropower plant (HPP). The pipeline was designed to have a capacity of 280 thousand m3 of water per day, but due to a decline in water consumption, only 122.2 thousand m3 of water per day was transported in 2020. The reduced water demand in the city can be attributed to economic factors such as the reduction or cessation of production processes in large enterprises. As a result, the structure of water consumption has changed significantly. Currently, only 20% of water is used for production purposes, while the remaining 80% is consumed by the population. It is essential to note that any attack or damage to the current water supply source can be seen as a use of water as a weapon against the city’s civilian population.
In some remote areas of the city, water is supplied from several wells that have been out of use since the late 1980s. However, this water is not safe for drinking as it does not meet the required standards. While the underground aquifers are free from bacterial contamination, they do not meet the standards for drinking water in terms of salt composition. The water’s dry residue, hardness, sulfates, and chlorides exceed the permissible limits by 2–3 times. Currently, water is delivered to these areas each day via road transport to ensure the residents have access to safe drinking water.
The city’s wells can provide a maximum of 5–10 thousand m3 of water per day, with a total capacity of 20 thousand m3 per day. However, the city’s population consumed at least 80 thousand m3 of water daily before the water supply system was destroyed. Even with the current wells, the water supply system cannot be fully replenished. As a result, it was decided to fill the water supply system with water from the Southern Bug River to meet the technical water needs of the city’s population. It’s important to note that the water quality in the Bug estuary near Mykolaiv is unstable due to the influence of the sea, which makes it more like seawater than river water (Table 1). It is quite clear that water quality is a limiting factor in water use from this source. To supply the city with appropriate clean water, it is necessary to explore other sources of fresh water with optimal quantitative and qualitative indicators.
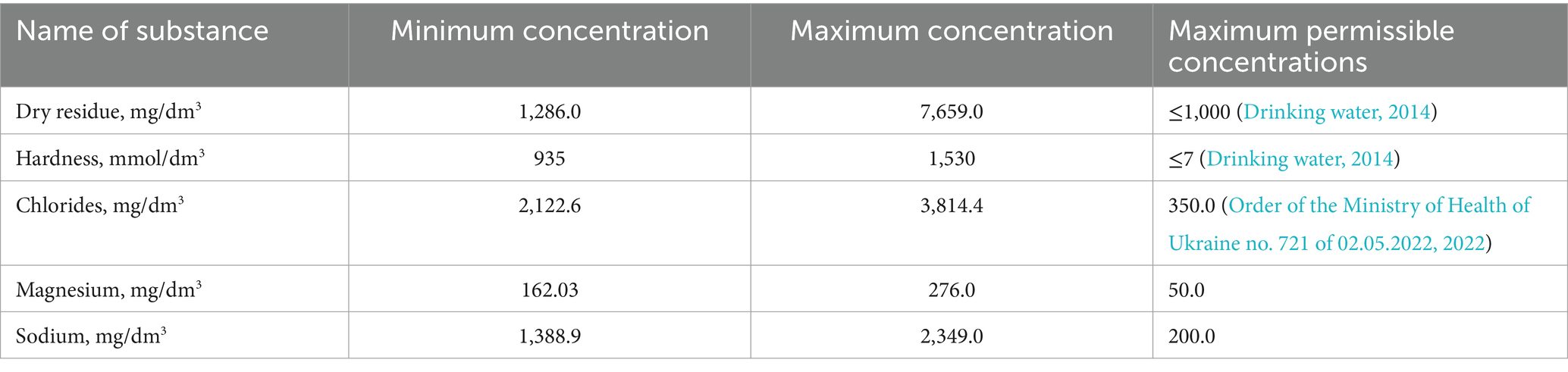
Table 1. Chemical composition of water in the South Bug River near Mykolaiv (Regional Office of Water Resources in Mykolaiv Oblast, 2014).
In 2019, experts from the Institute of Geological Sciences of the National Academy of Sciences of Ukraine (Shestopalov et al., 2019) studied the possibility of water supply to Mykolaiv from groundwater of the Upper Sarmatian aquifer in case of emergency. A hydrogeological model of the Mykolaiv groundwater deposit was created and the possibility of forced exploitation of groundwater from this aquifer was assessed, depending on the duration of the emergency period, taking into account the protection of fresh groundwater from brackish water from the Bug Estuary. They found that under certain technical conditions of groundwater extraction in the Balabanivsko-Svyatotroitska area, the maximum possible water supply to the population for 1 week is 45 thousand m3 per day (90 dm3/day per 1 city resident). This amount of water can be transferred to the population for 600 days without exceeding the established standard of water salinity (1.5 g/dm3). Unfortunately, this study offers only a temporary alternative and only a partial solution to the city’s water supply problem.
If we consider the use of highly mineralized, in fact, seawater from the Southern Bug estuary, at least several problems arise: first, the search for investments to build a seawater desalination plant; second, the problem of temporarily solving the issue of providing the city with drinking water for a long and uncertain period of construction of such a plant; third, the expected high cost of desalinated water production. For example, when using the most common seawater desalination technology, continuous reverse osmosis, the cost of 1 m3 of desalinated water will cost from $0.68 to $2.5 per cubic meter (World Bank, 2019).
To solve the problem, the best option is to use the water from the Southern Bug River. This can be achieved by setting up a water intake above the point where salty sea water affects the water quality of the river. A new water supply pipeline also needs to be built. However, before making the final decision, it is important to conduct hydrological calculations that take into account the potential impact of climate risks. This will help determine the availability of local water resources from surface runoff in the short, medium, and long term.
2.2 Description of the study area
The Southern Bug is the third longest in Ukraine located entirely within Ukraine, making it the only first-order river basin with such distinction (Figure 3). The river originates in the Volyn-Podilska Highlands and flows into the Dnipro-Buzky Firth. It is situated in the south-west region of the country and spans across two geomorphological regions. The upper and middle parts of the river are located on the Volyn-Podilska Highlands and the Dnieper Highlands, while the lower part is on the Black Sea Lowlands (Lyuta and Sanina, 2022).
The catchment area of the river is about 63,700 km2. The river stretches over a length of 806 km with a drop of 328 m, and its average stream gradient is approximately 0.4‰ (Lyuta and Sanina, 2022). The total resources of surface runoff formed in the Southern Bug basin are 3.4 km3, and in extremely dry years (95% probability of exceedance) they decrease to 1.4 km3. In high-water years, the water resources of the basin’s rivers are 2–5 times greater, and in low-water years, 3–9 times less than in average years (Yatsyk and Khorev, 2000). The basin spans across forest-steppe and steppe zones with a moderately continental climate influenced by the Black Sea. The significant geographical spread causes variations in annual temperatures between 7.1 and 10.0°C. Annual precipitation varies based on location, with an average of 669 mm in forest-steppe areas and 470–540 mm in steppe areas (Lipinsky et al., 2003).
The Southern Bug River Basin is a significant resource for Ukraine’s national economy, particularly for surface sources of fresh water. The river basin is mainly located within the Vinnytsa (62% of the region) and Mykolaiv (59.5%) regions. There are 16 dams on the Southern Bug River and its tributaries, and over 10 thousand ponds. The territory of the Southern Bug River Basin is inhabited by 3.24 million people, with 58% being urban dwellers and the remaining 42% residing in rural areas (Shakhman and Bystriantseva, 2021).
Water use in the Southern Bug River Basin has seen a significant reduction, falling by over four times from 1.185 billion m3 in 1995 to 279.2 million m3 in 2019. The reduction in water consumption occurred as a result of the shutdown of a certain number of large industrial enterprises in connection with the collapse of the Soviet Union in 1991. The surface and underground water abstraction from the Southern Bug River Basin within the Vinnytsa region in 2019 was 94.5 million m3, whereas it was 103.8 million m3 for the Mykolaiv region. The main sectors using water from the basin are agriculture (39%), industry (37%), and utilities (23%) (Shakhman and Bystriantseva, 2021).
2.3 Research methodology
The research methodology of the current study is divided into three main parts: (1) Analysis of the historical long-term variability and runoff formation of the Southern-Bug River. (2) Assessment of climate change impacts on water variability under different climate scenarios. Both parts involve the collection and analysis of data regarding water resources and climate. The second part applies the Global Hydrological Model (GHM) driven by a set of climate projections for two scenarios. Figure 4 presents the study workflow.
The historical data has been collected from various Ukrainian and international databases, including the Global Runoff Data Centre database (GRDC, 2023), long-term runoff data (1914–2020) for the Southern Bug River sourced from the Boris Sreznevsky Central Geophysical Observatory in Ukraine, data from the State Cadaster of Ukraine on the use of water resources (Open data portal, 2022), and operational and analytical data from annual reports by state institutions regarding the state and use of water resources in Ukraine.
To analyze the variability of flow, we used the method of constructing integral curves. The integral curve’s ordinates are calculated as an accumulated sum, Σ(Ki − 1)/Cv, where K is the modular coefficient (K = Qi/Qmean), Cv is the coefficient of variation, and Qi is mean annual river discharge for year i, Q mean is the mean annual multi-year period water discharge.
2.3.1 Climate data analysis
This study uses results from five Global Climate Models (GCMs) from the ISIMIP 3b project (ISIMIP, 2023). These GCMs are the GFDL-ESM4, UKESM1-0-LL, MPI-ESM1-2-HR, IPSL-CM6A-LR, MRI-ESM2-0. Their results were bias-corrected to reduce inherent simulation uncertainties and errors yielding the “CMIP6 climate forcing.”
Regarding future climate conditions, the study uses two distinct SSP-RCP scenarios: SSP1-2.6 and SSP5-P8.5. SSP1.26 aligns with the climate goals set out in the Paris Agreement, while SSP5.85 represents a ‘business as usual’ scenario. These choices allow us to analyze a “range of possible climate futures” based on different emission trajectories.
The climate data used has a spatial resolution of 0.5 × 0.5 degrees. The model’s time series is divided into two parts: historical simulations covering the period from 1861 to 2005, and future projections for the period 2006–2099, incorporating the effects of greenhouse gas emissions.
Climate projections from the GCMs were used to simulate future river discharge patterns, offering insights into the potential impacts of climate change on water resources.
For analysis, the future time series has been divided into a reference period and two future periods. The reference period is from 1986 to 2015, which provides a base-line for comparisons. The future periods of 2021–2050 and 2051–2080 allow us to evaluate both near and far future implications of climate change.
2.3.2 Hydrological modeling
The study utilized WaterGAP2, a global water availability and water use model that includes modules for various water uses like irrigation, domestic, manufacturing, livestock, and cooling of thermal power plants. Its capacity to simulate water fluxes, storages, and impacts of anthropogenic alterations at a 0.5 × 0.5 degrees spatial resolution and daily temporal resolution was instrumental in our hydrological assessments. The model’s basin-specific calibration approach aims to replicate observed river discharge in 1,319 basins globally, resulting in a plausible macro-scale representation of river discharge (ISIMIP, 2023; Krysanova et al., 2020; Müller Schmied et al., 2014; Müller Schmied et al., 2016).
Additionally, its performance was evaluated in a recent study by Didovets et al. (2020). The study found that WaterGAP2 has the best representation of river discharge among other global hydrological models for the territory of Ukraine considered in the study. This further confirms its suitability for our research.
3 Results
3.1 The current state of water resources of the Southern Bug River
The optimal location for a new water intake on the Southern Bug River is in the area of Nova Odesa. This area is approximately 40 km away from Mykolaiv and 50 km from the river mouth, as shown in Figure 5. The nearest hydrological gauge with a long period of observation is located in Oleksandrivka village, which is 137 km from the river mouth. There is no significant impact from natural factors such as lateral inflow or economic activity such as water withdrawal or discharge on the hydrological regime of the river between Oleksandrivka and Nova Odesa, with a population of 14,000. Therefore, the quantitative flow parameters available for Oleksandrivka village in this section of the river will remain unchanged.
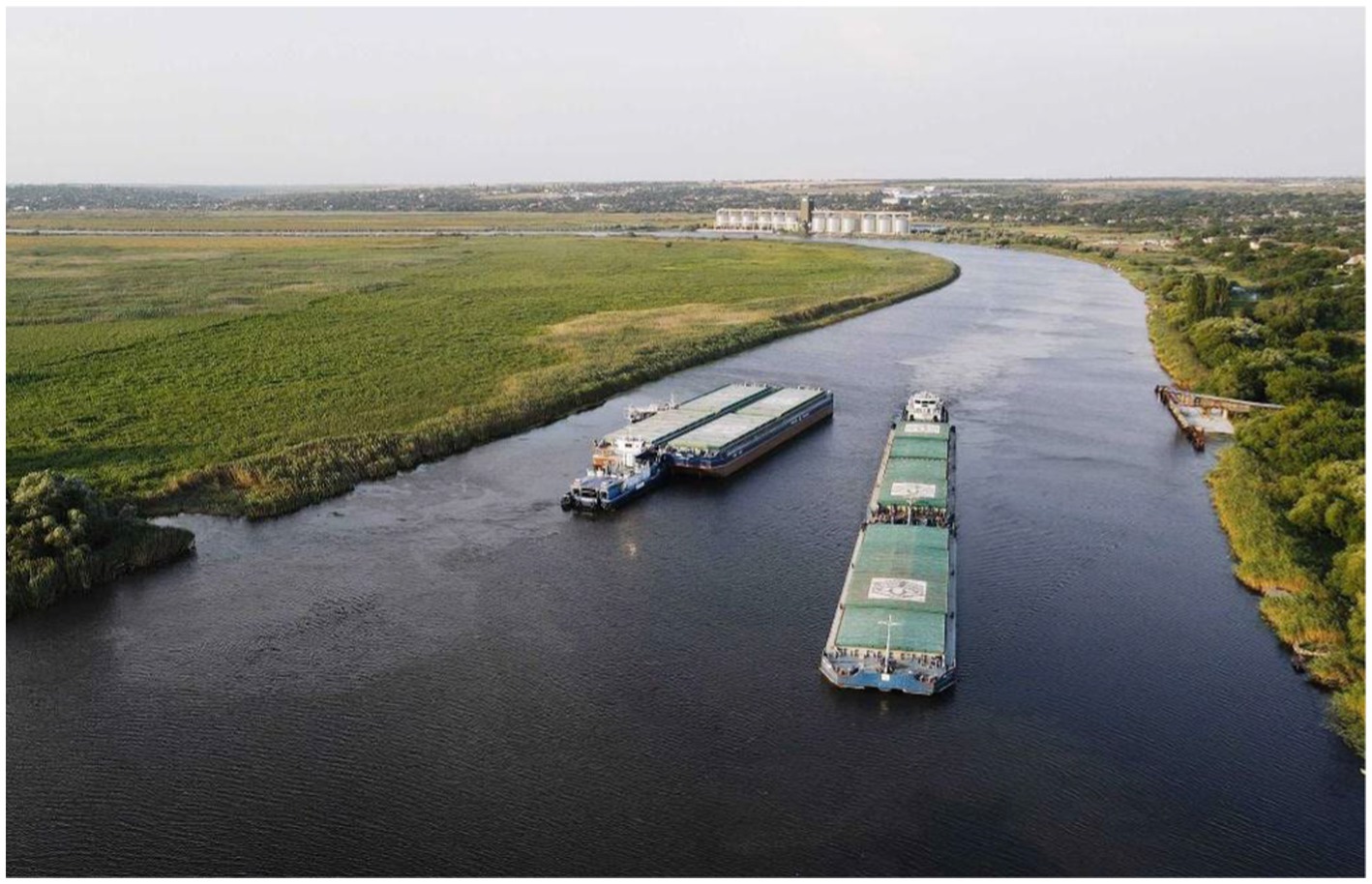
Figure 5. River Southern Bug near Nova Odesa. Photo from Facebook (2023).
An important argument for locating the water intake in this area is the fact that this section of the river receives water of improved quality from the Oleksandrivka reservoir. This reservoir is one of the largest in the Southern Bug and serves a complex purpose. The reservoir receives water from the upper reaches of the basin, which contains a significant amount of pollutants due to untreated wastewater from the large cities of Khmelnytskyi and Vinnytsia being discharged directly into the river. The water in the reservoir settles and removes some suspended solids with absorbed heavy metals and microorganic pollutants. As the water passes through the dam and turbines of the Oleksandrivka HPP, it becomes saturated with oxygen, which promotes oxidation and decomposition of organic pollutants. As a result, the quality of the river water in the area below the reservoir and in the area of Nova Odesa is satisfactory, according to Afanasуev et al. (2014).
3.2 Long-term variability of water flow
To assess the hydrological regime of the river in the area of a possible new water intake and the availability of water resources for water supply in Mykolaiv, it is possible to use the data of long-term observations of water flow at the Oleksandrivka hydrological station. Overview water flow data in the Oleksandrivka gauging station for the long-term observation period from 1914 to 2020 are presented in Table 2.

Table 2. Overview of water flow data of the Southern Bug River at the gauge Oleksandrivka (Vyshnevskyi and Kutsiy, 2022).
The analysis of the long-term dynamics of water flow (Figure 6A) shows that the river flow during the study period from 1914 to 2020 varied in a wide range. To get a clearer idea of the periodicity of the river flow formation, a graph of normalized difference-integral curves was constructed (Figure 6B). The analysis of this curve allows us to determine three periods of water flow formation in the Southern Bug River based on the change of negative or positive trend of the curve:
1. 1914–1966—the period of natural flow;
2. 1967–1995—the first period of regulated flow (creation of channel storage tanks on the rivers of the basin);
3. 1996–2020—the second period of regulated flow (the flow of the lower reaches of the Southern Bug River is regulated by the Oleksandrivka Reservoir).
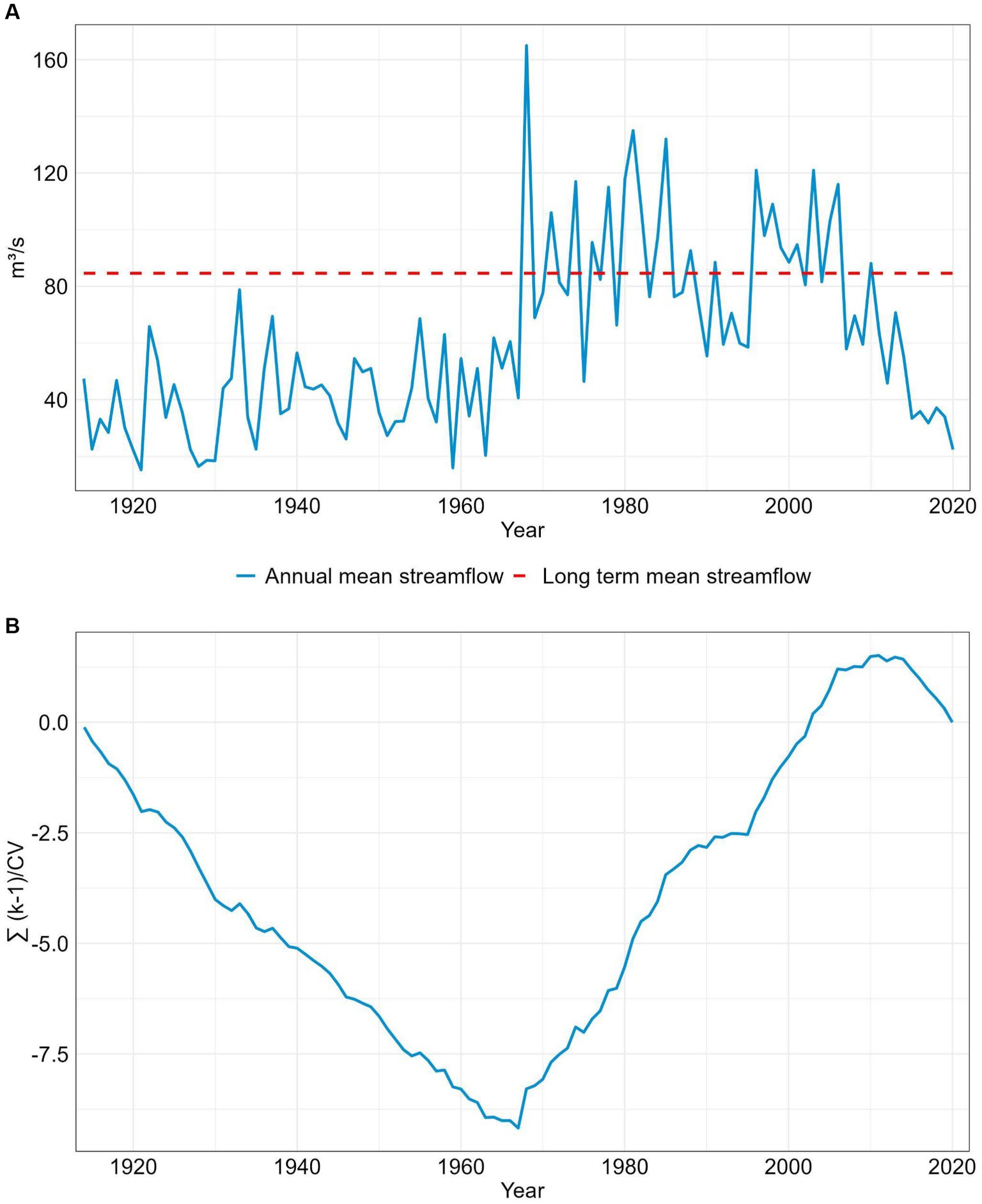
Figure 6. Changes in the streamflow during from 1914 to 2020 in the Southern Bug River. (A) Comparison of annual mean streamflow variability with the long term mean streamflow. (B) Long-term variability of flow depicted as the normalized difference-integral curve.
Figure 7 clearly shows that at the beginning of the study period, the river flow was significantly lower than the mean annual multi-year period water flow (Q mean = 84.6 m3/s), in the middle of the period the river water flow increased (to 168 m3/s), and at the end of the period it began to decrease significantly (to 22.4 m3/s). The statistical parameters of water flow in different periods of time are shown in Table 3.
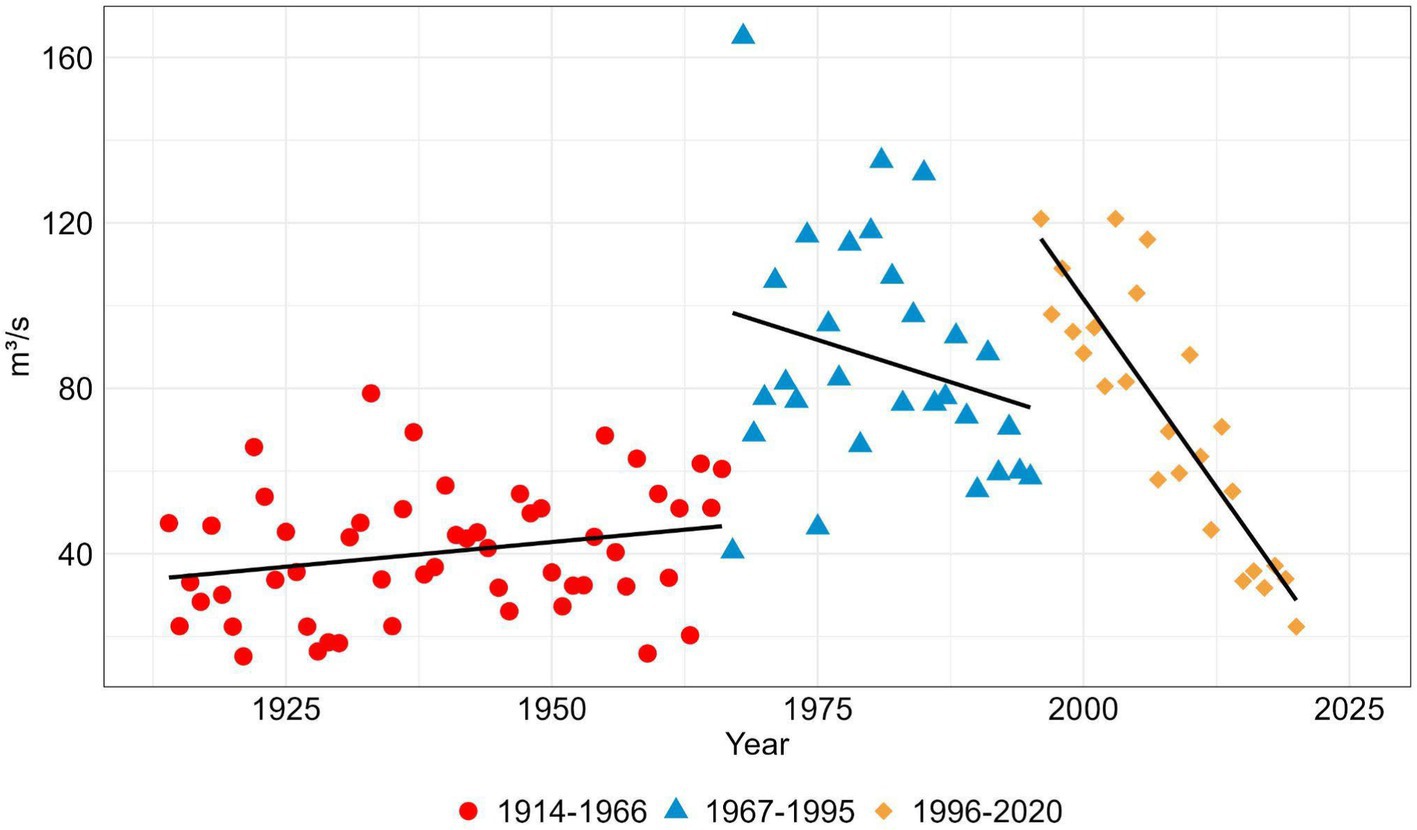
Figure 7. Comparison of annual streamflow trends across three historical periods in the Southern Bug River.
3.3 Intra-annual distribution of the water flow
The characteristics of intra-annual flow distribution are extremely important for planning and implementing water management measures. The analysis of changes in the intra-annual water flow distribution from 1914 to 2020 was performed by comparing flow hydrographs for three characteristic periods using average monthly water flows (Figure 8). This Figure clearly shows that the natural flow of the river (1914–1966) was characterized by a significant peak of spring floods in February, during which 50% of the annual river flow occurred. In 1967–1995, due to the accumulation of flood runoff in constructed ponds and reservoirs, the annual share of runoff in this month decreased to 29.5%, and in 1996–2020, due to the accumulation of water in the Oleksandrivka Reservoir, it decreased to 11.5%. At the same time, the share of runoff in summer, autumn, and winter months increased due to the artificial regulation of water supply to the lower part of the river by the Oleksandrivka HPP dam (Figure 9). Figure 9 shows that since the 1980s, artificial flow control has stabilized the intra-annual flow distribution, which is still characteristic of the river today. Therefore, to calibrate the hydrologic model to calculate runoff projections for the medium and long term, it is sufficient to use daily observation data for the last 30-year period, which began in 1991.
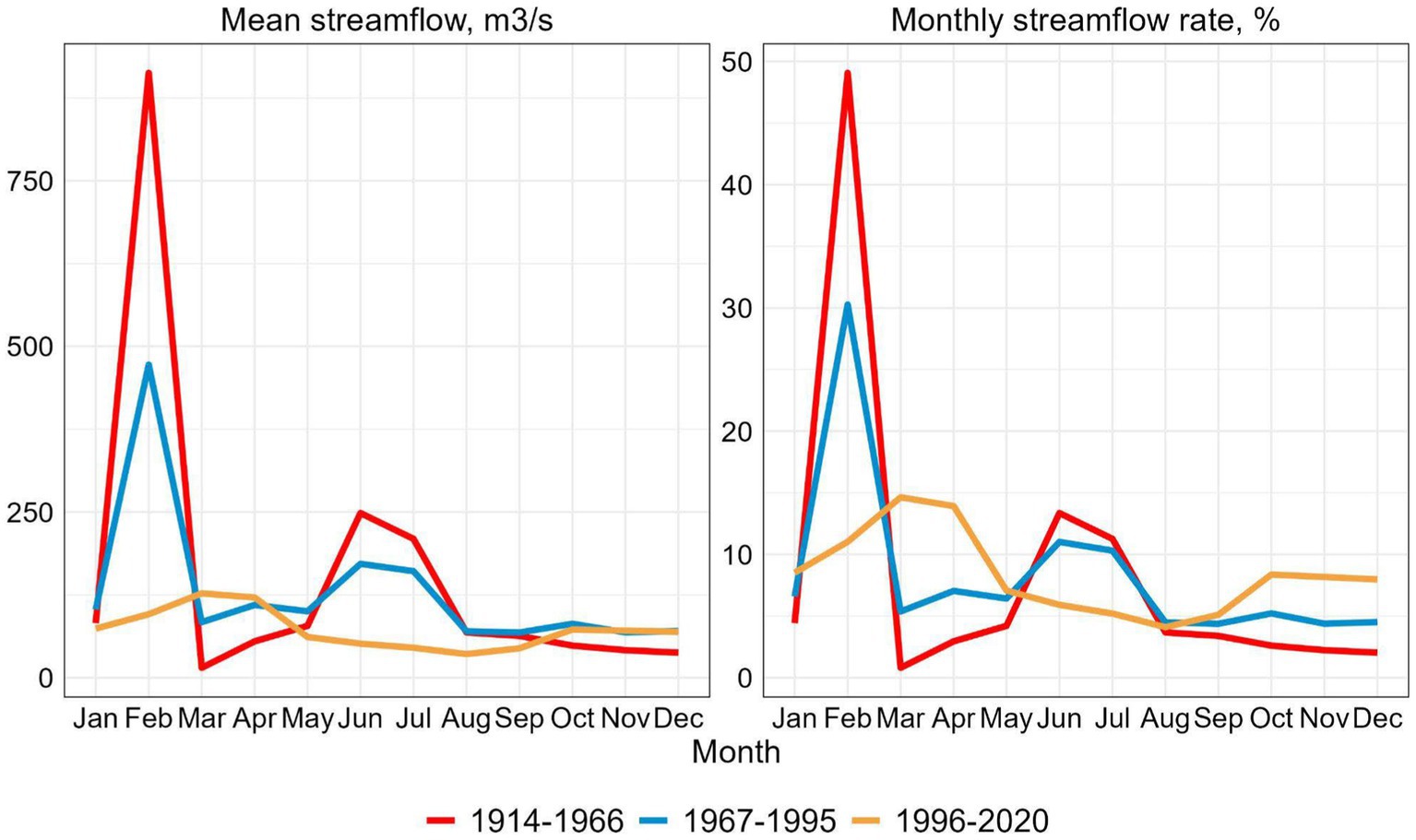
Figure 8. Change of intra-annual distribution of the Southern Bug River streamflow at the gauge Oleksandrivka as a result of flow regulation. Mean monthly streamflow (left) and monthly streamflow rate in % (right).
3.4 Results for projected changes in climate and water flow
3.4.1 Climate projections
In Ukraine, climate change projections suggest a notable warming trend over the 21st century. Under the mitigation pathway SSP1, the mean annual temperature is projected to rise by 2 degrees until the mid-21st century and subsequently stabilize. However, the SSP5 scenario, often characterized as ‘business as usual’, projects a more drastic temperature increase, potentially reaching a 6-degree rise by the end of the century. Notably, precipitation patterns do not show significant alterations under either scenario (Figure 10).
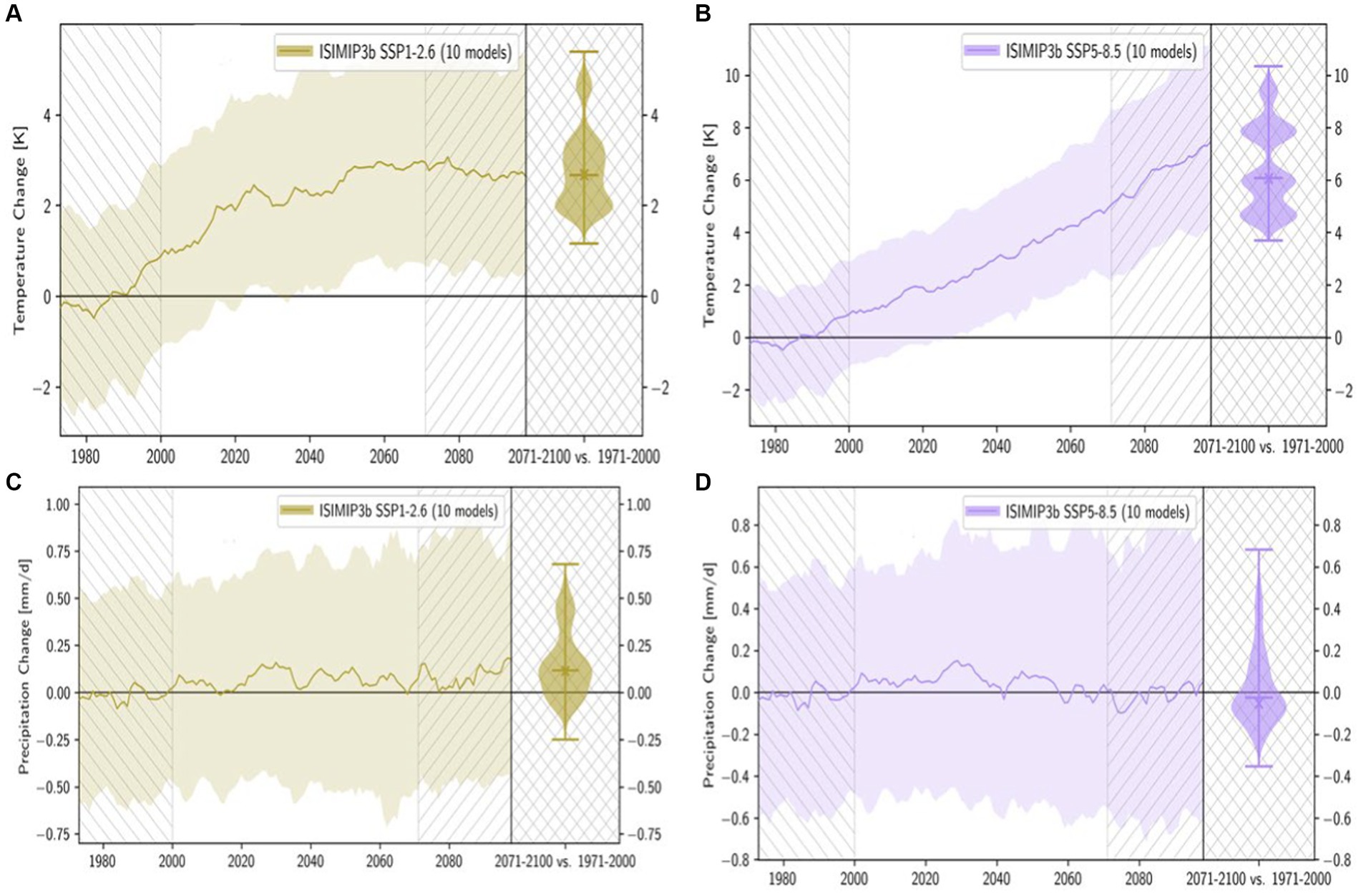
Figure 10. Projected changes of mean annual temperature for SSP1-2.6 (A) and SSP5-8.5 (B) and annual precipitation for SSP1-2.6 (C) and SSP5-8.5 (D) across Ukraine based on ISIMIP3b projections.
3.4.2 Projected river flow changes
Figure 11 depicts the modeled alterations in both the annual average and long-term monthly mean river discharge across the Southern Bug basin. These simulations are based on two prospective timeframes, 2021–2050 and 2051–2180, under scenarios SSP1-2.6 and SSP5-P8.5. The outcomes were derived from the hydrological model driven by four bias-adjusted General Circulation Models from ISIMIP3b.
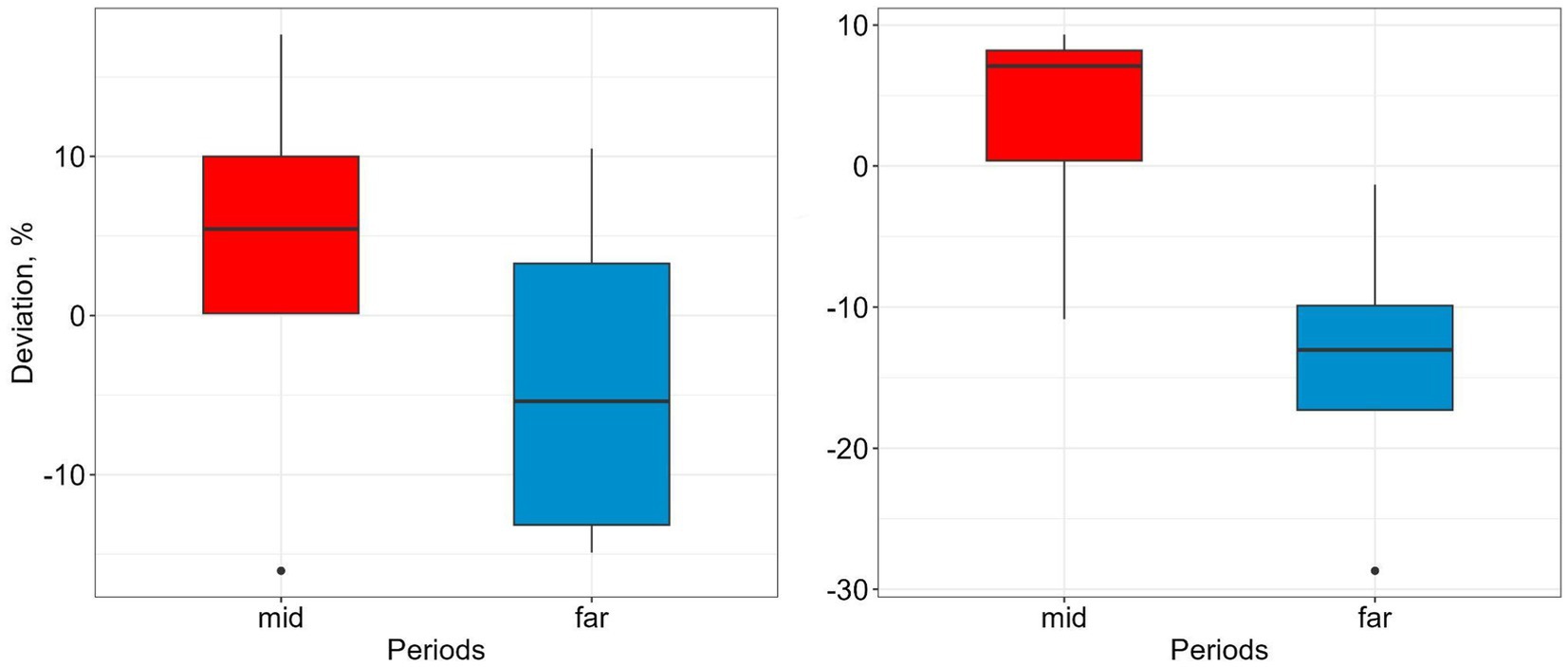
Figure 11. Projected changes in the mean annual river discharge for two future periods (2021–2050—mid, 2051–2080—far) under SSP1-2.6 (left) and SSP5-8.5 (right).
In the hydrological gauge Oleksandrivka, located downstream of the basin, distinct changes in river discharge are projected based on multiple future scenarios. According to multi-model median projections, under the relatively mitigated scenario of SSP1-2.6, the mean annual discharge is anticipated to increase by approximately 5% in the mid-future. Conversely, under the more severe scenario of SSP5-P8.5, the increase could reach up to 7%.
However, the long-term horizon paints a different picture. For the far future, projections indicate a decrease in river discharge, ranging from 4% under SSP1-2.6 to a substantial 14% under the SSP5-P8.5 scenario (Figure 11).
Projected decreases in long-term mean monthly discharge are expected for most seasons and months, except for winter. According to both outlined scenarios, there will be an increase in streamflow during winter months in the mid-future, ranging from a modest 5% to a more significant 30% (Figure 12). Interestingly, from April to June, no discernible changes in river discharge are projected for the mid-future under either scenario. However, from July through November, the majority of months under both scenarios show a decline in streamflow of up to 10–12%.
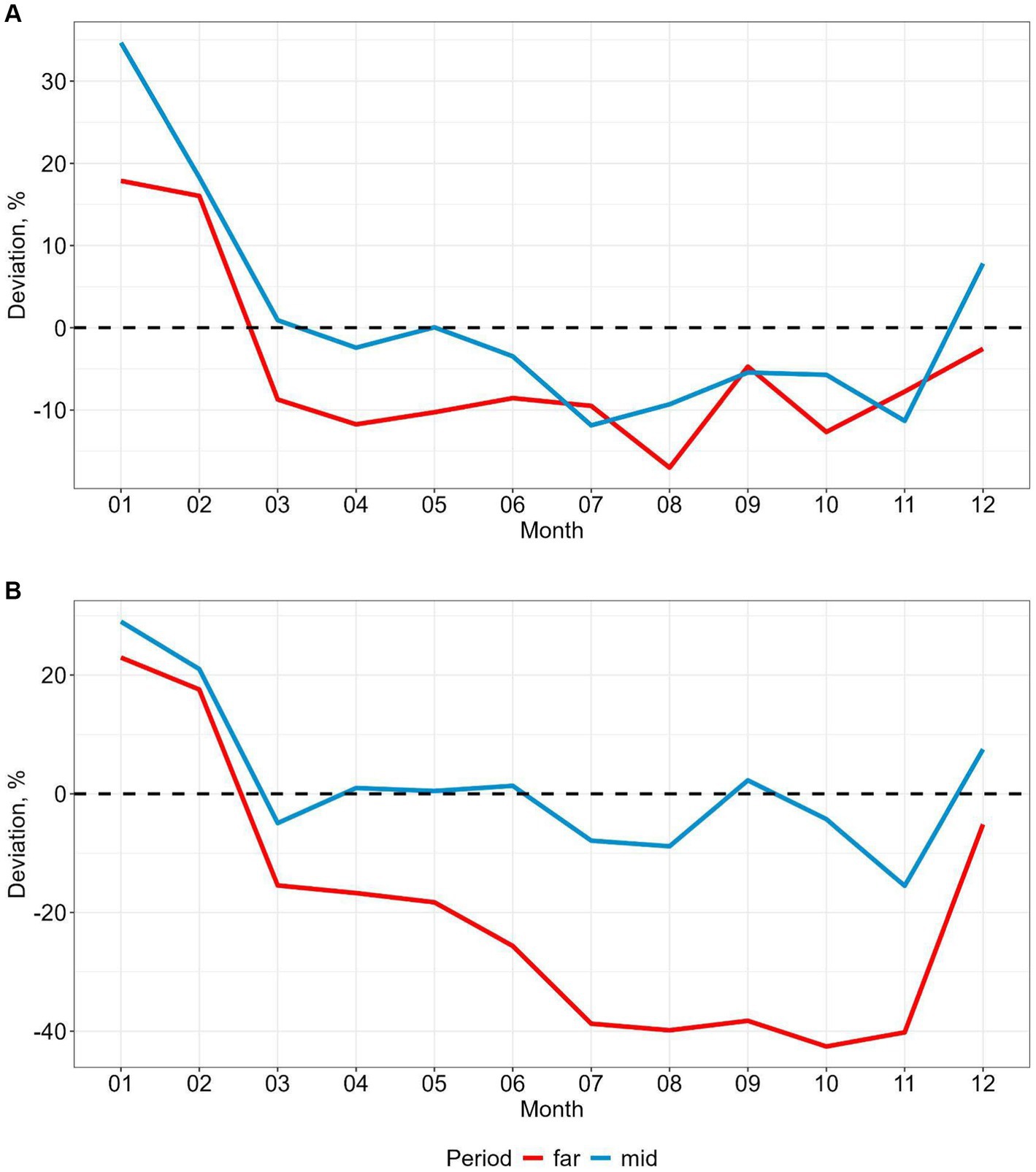
Figure 12. Projected changes in the long-term mean monthly river discharge for two future periods under SSP1-2.6 (A) and SSP5-8.5 (B).
Looking further into the future, the projections suggest more pronounced changes. There is an expected decline in water availability from March to the end of the year under both scenarios, reaching a significant decrease of up to 40% during the summer and early fall months.
3.4.3 Assessment of water availability and climatic risks for water supply
To assess the availability of water on the selected river section in the area of Nova Odesa for the water supply of Mykolaiv, taking into account climate change, the results on the projected water flow for the mid and far future were used following SSP1-2.6 and SSP5-8.5. The needs for alternative water supply to Mykolaiv were calculated, taking into account the minimum environmental flow. The total value of water distraction and minimum environmental flow was compared with the projected flow in the lower reaches of the Southern Bug River in the Oleksandrivka gauging station. For ease of comparison, all calculated values were converted to the same hydrological unit (m3/s). The monthly average water consumption in the city 1.41 m3/s, before the water supply was cut off. We also took into account the value of the mandatory minimum water flow in the Oleksandrivka stream, which is 17.0 m3/s. The results of the comparison are presented in the form of a combined diagram in Figure 13.
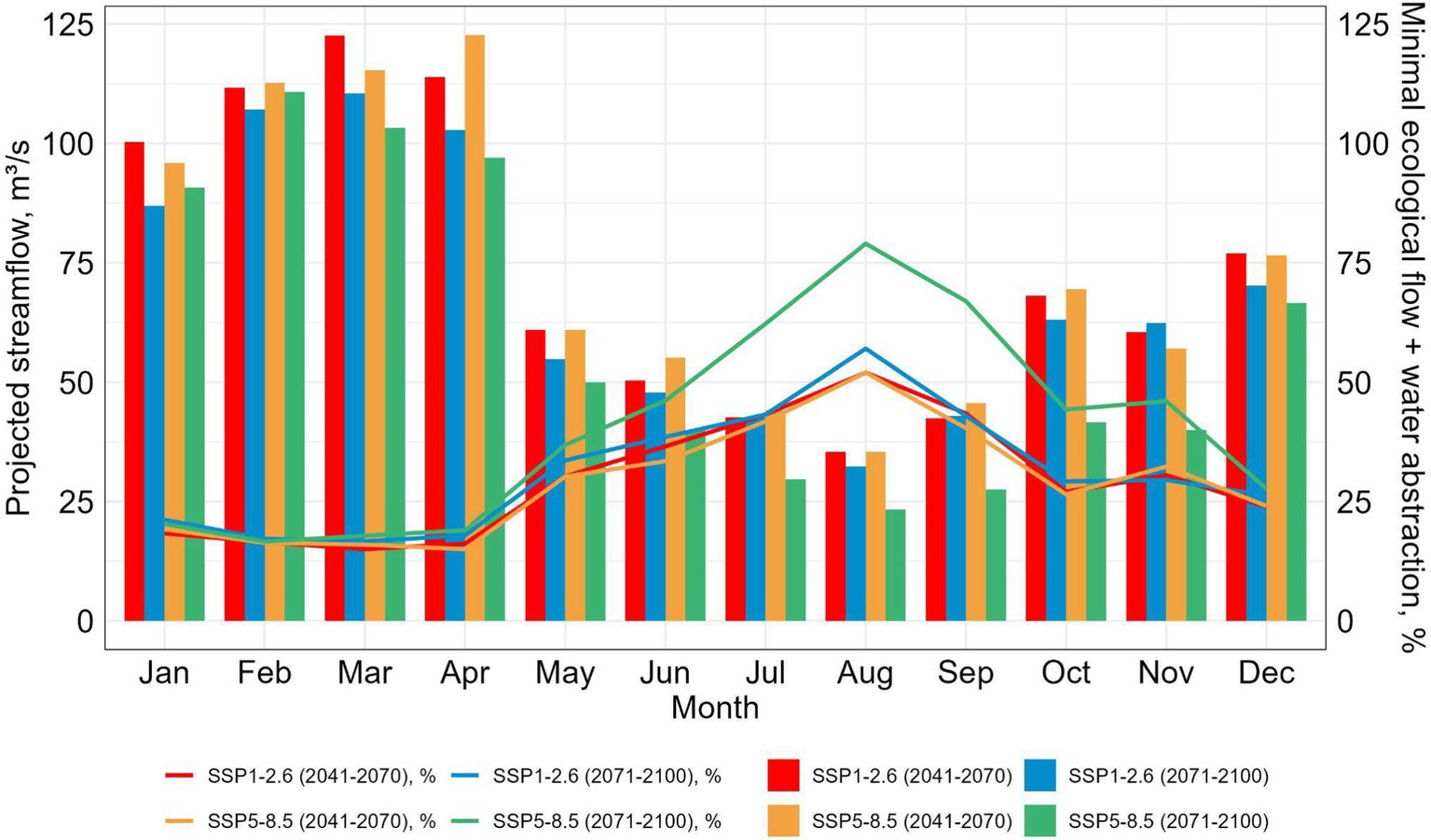
Figure 13. Comparison of the total needs for ensuring alternative water supply of Mykolaiv city and the minimum environmental flow in the lower section of the Southern Bug River with the projected flow at the gauge Oleksandrivka based on SSP1-2.6 and SSP5-8.5 for two future periods.
According to calculations, both the minimum ecological flow and the amount of water that will be withdrawn for water supply purposes, amount to a total of 18.41 (17.0 + 1.41) m3/s. Depending on the water flow throughout the year in the Oleksandrivka section, this value will range from 10 to 15% of the river flow during winter months (2021–2050), and from 30 to 52% from May to November. This means that the projected water flow of the river will meet both the minimum climate flow and the water supply needs of the city, taking into account both climate scenarios. In 2051–2080, according to the SSP1-2.6 projection, the intra-annual flow distribution will be similar to the period 2021–2050 with a slight decrease in August. As a result, the share of the required amount of water for water supply together with ecological runoff from the river flow will increase slightly (up to 57%).
The largest decrease in the river flow is projected under the SSP5.85 scenario in the long-term perspective of 2051–2080 from May to October (Figure 13), so an increase to 40–79% of the share of water that needs to be directed to ensure ecological flow and water supply to the city should be expected. However, even in August, when the water runoff may decrease to critical values of 23 m3/s, there will still be enough water for these needs.
4 Discussion
The impact of climate change is increasingly important for the design, construction, and maintenance of water sector infrastructure (Danilenko et al., 2010; Sarker, 2022). Climate change is having a significant impact on river flows. The last decade, especially the years after 2015, have seen the warmest temperatures recorded not only in Ukraine but also across the Northern Hemisphere. In some years, the increase in average annual air temperature has exceeded 2.0°C with the highest recorded temperatures being 2.2°C in 2007, 2.3°C in 2015, and 2.7°C in 2019, according to the World Bank (2021) report (World Bank, 2021). In Ukraine, the average annual air temperature has been exceeding the normal temperature by 1.5°C since 2007 (World Bank, 2021). Air temperatures are expected to rise further in Ukraine, especially in its southern part (Snizhko et al., 2020). Our earlier research (Shevchenko et al., 2020) showed that during 1981–2010, the southern part of the Southern Bug basin was characterized by the highest annual number of heat waves in Ukraine. Rising temperatures, droughts, and heat waves have contributed to increased evapotranspiration from the surface of reservoirs and reduced water availability in the Southern Bug River basin. A statistically significant relationship between the SPEI drought index and the minimum river flow of the Southern Bug River at the gauge Oleksandrivka for the period 1950–2010 was established (Ovcharuk et al., 2020).
Many researchers note that the influence of climate on the amount of precipitation can be critical in arid and semi-arid areas (Marcos-Garcia et al., 2017; Marcos-Garcia and Pulido-Velazquez, 2017; Rubio-Martin et al., 2023). A study by Zabulonov et al. (2018) revealed that fluctuations in the annual runoff of the Southern Bug River are highly affected by long-term precipitation dynamics. According to Levkovska and Zubko (2021), in Mykolaiv region, the average monthly precipitation has been steadily decreasing from 2015 to 2020, decreasing by 20%, and in the summer months (July and August) by 20–30% in recent years.
The study of long-term series of water discharges (Section 3.2) showed that there are three specific periods of river flow formation. In the first period (1914–1966), when the water flow in the Oleksandrivka section depended solely on natural factors, the long-term value of the water flow was almost half of the next two periods.
The impact of climate change on the formation of the river’s water flow is also felt in winter, when the air temperature often rises and the snow cover melts rapidly. This leads to the formation of maximum annual runoff earlier than usual. The value of this runoff decreases and the duration and volume of spring flood runoff also decrease. However, the possibility of accumulating enough water in reservoirs for use in summer increases. This redistribution of the maximum river flow in the Southern Bug basin is consistent with the trends projected by studies conducted by Gorbachova et al. (2021a, 2021b), Ovcharuk and Gopchenko (2022), and Snizhko et al. (2023b), which indicate a decreasing spring flood flow. Under future warming conditions, the decrease in spring runoff in the Southern Bug basin is expected to be 50% (Ovcharuk et al., 2020), which may cause difficulties in filling all artificial reservoirs in the basin with water. Our research results, on the other hand, indicate that there will be a 10–20% decrease in spring runoff only in the far-future period.
Climate change is not the only reason behind water shortages in the lower part of the Southern Bug River basin. The second reason is the high degree of flow regulation in creating water reserves for use during dry summer and fall seasons, along with increased water consumption in the agricultural and energy sectors.
Artificial flow regulation began to have a significant impact on the river’s water flow starting in 1967 (the beginning of the second period of flow formation). In the early 1960s, almost 10,000 artificial reservoirs with a total volume of more than 1.5 km3 were created in the river basin, which is almost equal to the river flow in a low-water year of 95% exceedance probability (Southern Bug River Basin Water Resources Management, 2023). It is known that 56% of the river’s flow is formed in the upper and middle parts of the basin. Most of the reservoirs (106 out of 187) are located there, accumulating water runoff that is largely used by local water users (Southern Bug River Basin Water Resources Management, 2023). In addition, the large water surface area of these reservoirs (30 thousand hectares), combined with the arid climate of the region, leads to significant water losses due to evapotranspiration.
During spring floods, water was accumulated in ponds and reservoirs, while in dry months and seasons, it was supplied to the lower section of the river in more significant amounts than the natural flow in the previous period. As a result, the average annual runoff value nearly doubled compared to the first period and approached the long-term flow rate (Table 3).
The most recent flow formation period (1996–2020) is of particular interest in this study. Although the flow rate during this period (72.5 m3/s) is similar to the flow rate of the previous period (1967–1995), the average annual water flow has started to decrease significantly over the past 25 years, beginning in 1996 as shown in Figure 7. The reason for this recent runoff trend is the combination of climate change with runoff regulation and increased water use.
In 1986, the construction of the Oleksandrivka Reservoir on the Southern Bug River began, and it was filled with water until 1990. In 1999, the Oleksandrivka HPP was launched with a water level of 8.0 m. In the spring of 2006, the Oleksandrivka reservoir was filled to a level of 14.7 m, and in 2010 to a level of 16.0 m, which increased its management capacity to 20.95 million m3 and its regulatory capacity to 14.0 million m3 (State Scientific and Engineering Center for Control and Emergency Response Systems, 2016).
In this period water consumption for irrigation has increased by 1.3–1.4 times annually compared to the 1980s due to rising air temperatures and an increase in evapotranspiration (Romashchenko, 2012). The agricultural business is developing rapidly, and this is contributing to the water consumption. The Oleksandrivka Reservoir operated by the South Ukrainian Energy Complex and the Tashlyk Reservoir, which serves as a cooler for the South Ukrainian Nuclear Power Plant (NPP), also contribute to the reduction in the flow of water in the Southern Bug. Irreversible losses of water in the Southern Bug River due to its use for the South Ukrainian NPP and Tashlytsky hydroelectric power plant (HPP) in August–September and in low-water years with a 95% probability of exceedance of flow are estimated at 10–11% (Romas et al., 2006). Although the volume of water accumulated in artificial reservoirs currently satisfies the existing water consumption needs, it is a limiting factor for further development of the region’s agricultural sector.
In the last two decades, the river’s water flow has decreased due to the combined effects of climate change and economic activity.
If this trend continues, the water flow will decrease by another 10–12% in the mid-future period and up to 40% in the far-future period as shown in Sections 3.4.2 and 3.4.3. It should be noted that the obtained results of the simulation of water flow are not devoid of uncertainty, like all other simulations of the climate impact on the water sector. These uncertainties are due to various reasons, among which the quality of the initial data, the imperfection of modern climate and hydrological models, the uncertainty of the applied socio-economic pathways, and others (Kundzewicz et al., 2018; Bennett et al., 2012).
However, we have made some efforts to significantly reduce the contribution of uncertainty to the results of our calculations. For this purpose, we chose not one climate model, but an ensemble of 5 models and carried out bias correction of their results in order to reduce inherent simulation uncertainties and errors yielding the “CMIP6 climate forcing.”
Significant preliminary work was carried out on the selection of the most suitable hydrological model for the territory of Ukraine (Didovets et al., 2020). Of the six models (LPJmL, MATSIRO, H08, WaterGAP2, DBH, and PCR-GLOBWB), the WaterGAP2 model demonstrated the best results of water flow simulation. The model selection procedure also contributed to reducing the uncertainty of the final result. Two SSP-RCP scenarios were also selected in order to obtain a certain most probable range of possible future flow changes: SSP1-2.6SSP1-RCP2.6 and SSP5-P8.5SSP5-RCP8.5. SSP1.26 aligns with the climate goals set out in the Paris Agreement, while SSP5.85 represents a ‘business as usual’ scenario. These choices allow us to analyze a “range of possible climate futures” based on different emissions trajectories.
The results of flow design obtained according to this technological scheme and comparing them with the water management needs of the region showed that in 2021–2050, 10–15% of the river flow during winter months and from 30 to 52% will be sufficient for water supply needs and to guarantee environmental flow from May to November (Figure 13). That is, a critical situation for the functioning of the water intake is not foreseen for the next 30 years. If even predicting the impact of forecasting uncertainty in this result, there remains a fairly large reserve of water flow for use.
In 2051–2080, according to the SSP1-2.6SSP1-RCP2.6 forecast, the share of the required amount of water for water supply and environmental runoff in summer may reach a maximum of 57%, and according to SSP5.85 even 79% (August), although in other months of the year, the need for water withdrawal will not exceed 25–30% of the projected flow in the Oleksandrivka catchment (Figure 13). Even in this case, a correction of the result due to the possible uncertainty is permissible while maintaining the guarantee of the required water selection without harming the environment.
Construction, filling, and operation of Oleksandrivka Reservoir and HPP began in the 1990s, changing the intra-annual runoff distribution scheme (Figure 9). During the winter and spring months, excess water resulting from runoff is collected in the reservoir. This water is then released through the hydroelectric power plant’s dam in the summer. The release of water is done in larger quantities than the natural runoff to compensate for the shortage of water resources in the dry season. Proper use of artificial reservoirs on snow-fed rivers can significantly mitigate the consequences of climate-induced reductions in snowpack accumulation for agriculture and the environment (Baah-Kumi et al., 2024; Kim et al., 2019).
The State Agency of Water Resources of Ukraine has proposed a scheme for artificial regulation of river flow. This scheme aims to distribute the accumulated runoff in reservoirs evenly over the months of the year, accounting for different water availability in different years (Open data portal, 2022). On average, in a low-water year with a 50% reliability of flow, this value is 96.6 m3/s, in a low-water year with a 75% reliability of flow—71.0 m3/s, and in a low-water year with a 95% reliability of flow—57.9 m3/s per month.
If this regulatory scheme had been followed, there would be no doubt that Mykolaiv’s water supply and minimal environmental flow to the lower part of the river would have been ensured. However, from 2001 to 2019, climate change led to an increase in the average annual air temperature in the region by about 2°C and a sharp decline in river flow. During this period, there were 16 dry years, and the average annual runoff decreased from 2.88 km3 in the previous period to 2.15 km3 today, and in the dry year (2017) to 1.0 km3 (Landau and Chernomorov, 2020).
Based on recent hydrological observations at the Oleksandrivka HPP, it has been noted that during the summer and autumn seasons, the water discharge of the Southern Bug River has been declining for an extended period. This decline has resulted in critical values ranging from 5.98 to 13.3 m3/s, which is significantly lower than the mandatory minimum environmental flow value (as shown in Figure 14). Based on the daily water discharge data collected between 1991 and 2020, our calculations indicate that the duration of low water levels with discharges less than 18.41 m3/s (the minimum environmental flow) can last up to 20 days in a calendar year (Figure 14).
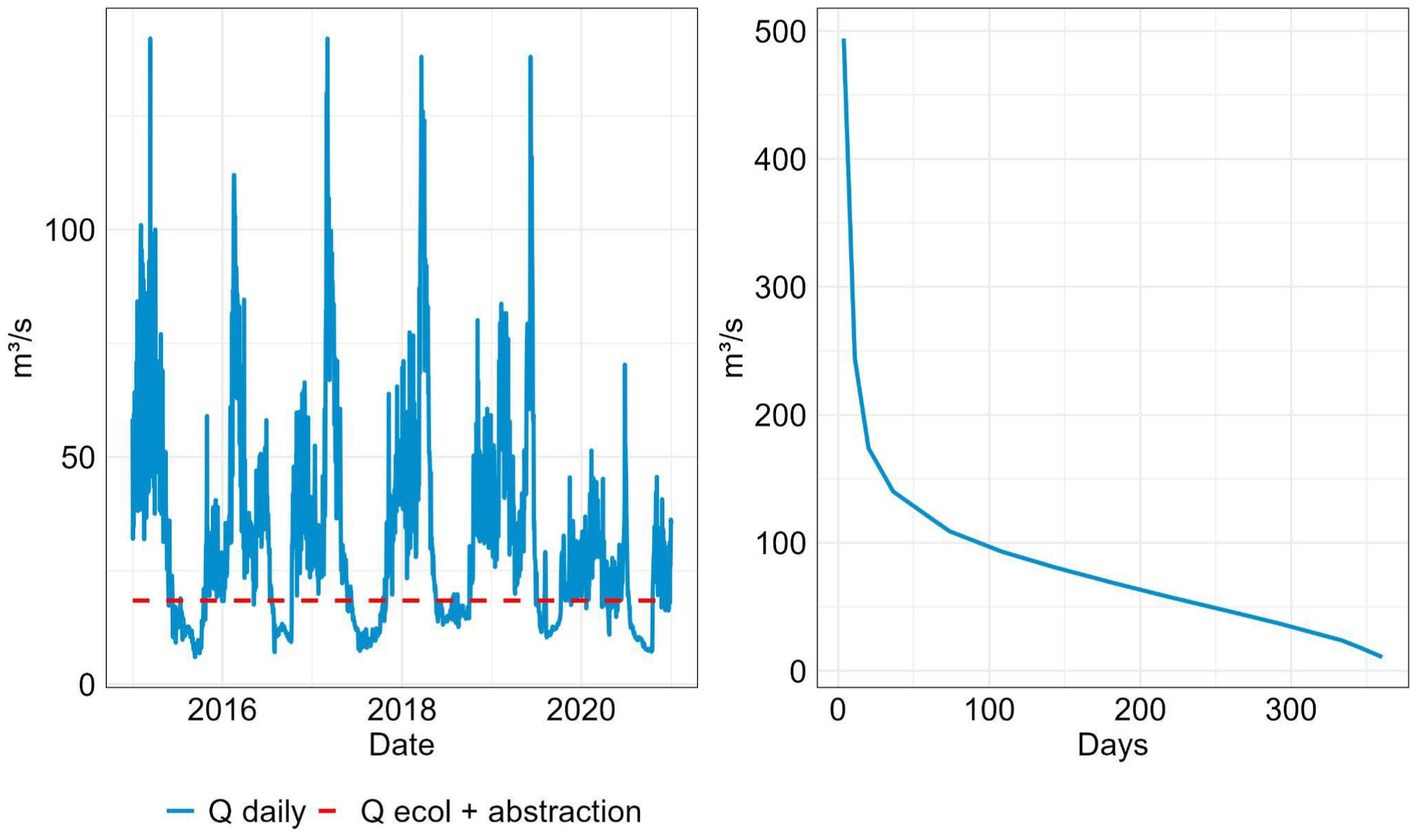
Figure 14. Daily streamflow of the Southern Bug River at the gauge Oleksandrivka. Daily time series of low-water years from 2015 to 2020 (left) and flow-duration curve based on the daily streamflow time series from 1991 to 2020 (right).
The State Agency of Water Resources in Ukraine conducts an annual review of the established modes of reservoir operation (Basin management of water resources of the South Bug River, 2021) to ensure that the water needs of all users and environmental flows in the lower part of the river are met. As a result, the minimum environmental flow was reduced to 12.1 m3/s in 2021. In recent years, the Oleksandrivka HPP reservoir has not been able to fully accumulate spring runoff in reservoirs for redistribution in subsequent seasons of the year in accordance with the established reservoir operation modes (Basin management of water resources of the South Bug River, 2021). It is impossible to increase water reserves in the Oleksandrivka Reservoir due to its small volume, although hydrological parameters of the flow in the Oleksandrivka Reservoir, even in recent low-water years during the spring flood, indicate the presence of significant volumes of water with maximum flow rates of 70.0–142.0 m3/s, which pass through the reservoir in winter months without being retained in it. A significant part of the river’s water flow is accumulated during spring floods in 188 small and medium-sized reservoirs in the upper and middle parts of the Southern Bug basin and is used in summer for local water users. These reservoirs cannot be used to improve the water management situation in the lower part of the river basin.
The energy sector proposed a solution to address the problem of additional water storage to provide water to all water users in the lower reaches of the Southern Bug River. The proposal included raising the level of the Oleksandrivske reservoir from 16.0 m to 16.9 m and then to 20.7 m, creating a usable volume of 46 million m3. This would have ensured a minimum environmental flow of the river in summer and autumn. However, such a decision could have negative environmental consequences.
Therefore, the Ministry of Environmental Protection and Natural Resources of Ukraine did not support this proposal, as it could cause irreparable damage to the natural and cultural heritage of Ukraine in this region.
Our research will help improve water security and environmental conditions. In recent years, many countries and large cities around the world have been experiencing increasing water scarcity as a result of climate change associated with global warming, population growth, and demand exceeding supply (Orimoloye et al., 2019; Quandt et al., 2022). Our study considers the case where access to sufficient water resources is limited by aspects of water quality and lack of infrastructure (broken water supply), rather than availability of resources. We focused on the primary problem of the region, which arose in connection with military actions and consists in ensuring adequate access to safe drinking water. However, the issue of water security cannot be solved without taking into account environmental issues.
The implications of using the research results in terms of environmental protection and climate change can be direct and indirect.
Direct effects will be determined by the preservation of channel and basin ecological systems by ensuring the minimum environmental flow necessary for their functioning. Seasonal characteristics and forecasts of climate and runoff for the near and long term, as well as water balances based on them, can be used in water management as a key element to achieve the necessary water security, greater sustainability and resilience of the water sector in the face of climate change.
An indirect environmental impact of the study results could be the use of runoff modeling data to implement both short-term and long-term water management plans. The use of long-term forecasts will help water managers and local administrations to respond in advance to seasonal deterioration by implementing water conservation measures in the public utilities sector, new irrigation technologies in agriculture, and introducing temporary restrictions for the energy sector, while ensuring minimal environmental flow losses. The use of the research results not only in water management, but also in municipal administration, and their dissemination in territorial communities will contribute to the successful adaptation of socio-economic and environmental processes in the region and can bring successful benefits not only to the economy, but also to communities.
5 Conclusion
This study examines the issue of ensuring water security for the population of the city of Mykolaiv in southern Ukraine, in the context of a shortage of usable water resources that has arisen under the influence of climate change and military operations. The Southern Bug River is being considered as a potential source of water for the city of Mykolaiv, Ukraine, after the primary water supply from the Dnipro River was interrupted by Russian armed aggression.
Based on the hydrological model WaterGAP2, driven by bias-corrected global climate models (GCMs) and two shared socio-economic pathways (SSPs), the results of this study suggest that the Southern Bug basin will experience significant variations in river discharge in the future under SSP1-2.6 and SSP5-P8.5 scenarios. According to the projections, the mean annual discharge could increase by about 5–7% in the medium future. In the far future, however, river flows could decrease from 4% to an alarming 14%.
Our research indicates that there will be changes in the long-term average monthly river discharge. While most seasons and months will experience a decrease in river discharge, there will be an increase of 5% to over 30% in winter months during the mid-future. From April to June, river discharge levels are expected to remain constant for the mid-future under both scenarios. However, from July to November, a decrease in streamflow of up to 10–12% is projected. Further into the far future, the reduction in water availability could reach up to 40% during the summer and early fall months.
The projections have shown that in the mid-future (2021–2050), only 10–15% of the river flow will be used during winter months to provide water supply to Mykolaiv. From May to November, up to 30–52% of the projected river flow will be used. In the far future (2051–2080), based on the SSP1-2.6 projection, the distribution of flow throughout the year will be similar to the period 2021–2050, with a slight decrease in August. This will result in a slight increase (up to 57%) in the amount of water required for water supply and environmental runoff from the river flow during summer. Only under the SSP5-8.5 scenario, in the far future of 2051–2080, will the largest decrease in flow occur from May to October, and the share of water for urban water supply and minimum environmental flow will increase to 40–79%.
This study is an important step in understanding the impacts of climate change on water resources in the Southern Bug Basin. It highlights the need for a sustainable approach to water management, given the region’s semi-arid climate and the increasing pressures of climate change. The resulting projections of future water flows high-light the urgent need to adapt water management strategies in the Southern Bug Basin to mitigate the potential impacts of climate change and ensure sustainable water availability in the region.
Research results are of great importance for the development of water management in the region, mitigation measures and adaptation of the water sector to climate change. They can be used in municipal administration and territorial communities to make decisions on socio-economic development and environmental protection.
Data availability statement
The original contributions presented in the study are included in the article/supplementary material, further inquiries can be directed to the corresponding author.
Author contributions
SS: Conceptualization, Data curation, Formal analysis, Investigation, Methodology, Visualization, Writing – original draft, Writing – review & editing. ID: Data curation, Formal analysis, Investigation, Methodology, Validation, Visualization, Writing – original draft, Writing – review & editing. OS: Writing – original draft, Writing – review & editing. MY: Data curation, Investigation, Writing – original draft, Writing – review & editing. FH: Writing – original draft, Writing – review & editing. AB: Conceptualization, Project administration, Supervision, Writing – original draft, Writing – review & editing.
Funding
The author(s) declare that financial support was received for the research, authorship, and/or publication of this article. This work was supported in part by a grant from the Volkswagen Stiftung. SS has received research support from the Volkswagen Stiftung (Ref. No. 9C084) to implement the project “Analysis of the availability of water resources in Ukraine taking into account climate variability for the reconstruction of the destroyed water sector in the postwar period.”
Conflict of interest
The authors declare that the research was conducted in the absence of any commercial or financial relationships that could be construed as a potential conflict of interest.
Publisher’s note
All claims expressed in this article are solely those of the authors and do not necessarily represent those of their affiliated organizations, or those of the publisher, the editors and the reviewers. Any product that may be evaluated in this article, or claim that may be made by its manufacturer, is not guaranteed or endorsed by the publisher.
Supplementary material
The Supplementary material for this study can be found online at: https://www.frontiersin.org/articles/10.3389/frwa.2024.1447378/full#supplementary-material
References
Afanasуev, S., Peters, A., Stashuk, V., and Iarochevitch, O. (2014). Southern Bug River basin management plan: river basin analysis and actions. Interservice: Kyiv, Ukraine.
Baah-Kumi, B., Boisramé, G. F. S., Taylor, M. H., and Carroll, R. W. H. (2024). Can reservoir and groundwater storage buffer the impacts of climate-induced declines in snowpack storage for agriculture and the environment? Insights from the walker river basin. J. Hydrol. 630:130781:130781. doi: 10.1016/j.jhydrol.2024.130781
Basin management of water resources of the South Bug River. (2021). Reservoir operation regimes. Operation modes of the complex reservoirs and water management systems of the South Bug River basin area for the period of spring flood passage and spring-summer period of 2021. Available at: https://buvrpb.davr.gov.ua/diialnist/rezhymy-roboty-vodoskhovyshch (Accessed December 9, 2023).
BBC. (2022). Russia accused of sabotaging Ukraine water pipe to Mykolaiv. Available at: https://www.bbc.com/news/world-europe-63383605 (Accessed December 7, 2023).
Bennett, K., Werner, A., and Schnorbus, M. (2012). Uncertainties in hydrologic and climate change impact analyses in headwater basins of British Columbia. J. Clim. 25, 5711–5730. doi: 10.1175/JCLI-D-11-00417.1
Danilenko, A., Dickson, E., and Jacobsen, M. (2010). Climate change and urban water utilities: challenges and opportunities. Water working notes; note no. 24. Washington, D.C.: World Bank Group.
Didovets, I., Krysanova, V., Hattermann, F. F., Del Rocío Rivas López, M., Snizhko, S., and Müller Schmied, H. (2020). Climate change impact on water availability of Main River basins in Ukraine. J. Hydrol. Reg. Stud. 32:100761. doi: 10.1016/j.ejrh.2020.100761
Ekka, A., Pande, S., Jiang, Y., and der Zaag, P. (2020). Anthropogenic modifications and river ecosystem services: a landscape perspective. Water 12:2706. doi: 10.3390/w12102706
Facebook. (2023). Nibulon company page. Available at: https://www.facebook.com/nibulonltd (Accessed December 9, 2023).
Falkenmark, M., Lindh, G., Mageed, Y. A., and Chow, V. T. (2019). Water fora starving world. New York: Routledge.
Gain, A. K., Giupponi, C., and Wada, Y. (2016). Measuring global water security towards sustainable development goals. Environ. Res. Lett. 11:124015. doi: 10.1088/1748-9326/11/12/124015
Gleick, P. H. (1993). Water and conflict: fresh water resources and international security. Int. Secur. 18, 79–112. doi: 10.2307/2539033
Gleick, P. H. (2019). Water as a weapon and casualty of armed conflict: a review of recent water-related violence in Iraq, Syria, and Yemen. WIREs Water 6:e1351. doi: 10.1002/wat2.1351
Gleick, P., Vyshnevskyi, V., and Shevchuk, S. (2023). Rivers and water systems as weapons and casualties of the Russia-Ukraine war. Earth’s Future 11:e2023EF003910. doi: 10.1029/2023EF003910
Gorbachova, L. O., Prykhodkina, V. S., and Khrystiuk, B. F. (2021b). Spring flood frequency analysis in the Southern Bug River Basin, Ukraine. J. Geol. Geogr. Geoecol. 30, 250–260. doi: 10.15421/112122
Gorbachova, L. O., Prykhodkina, V. S., Khrystiuk, B. F., Zabolotnia, T. O., and Rozlach, V. O. (2021a). Statistical analysis of maximum runoff of the Southern Bug River using the method of “indicators of hydrologic alteration”. Ukrain. Hydrometeorol. J. 27, 42–54. doi: 10.31481/uhmj.27.2021.05
GRDC. (2023). Global runoff data base. Available at: http://grdc.bafg.de (Accessed December 7, 2023).
Guidelines for drinking-water quality (2017). Geneva: World Health Organization (WHO). Available at: https://www.who.int/publications/i/item/9789241549950
Gunda, T., Hess, D., Hornberger, G. M., and Worland, S. (2019). Water security in practice: the quantity-quality-society nexus. Water Secur. 6:100022. doi: 10.1016/j.wasec.2018.100022
ISIMIP. (2023). Impact model: WaterGAP2. Available at: https://www.isimip.org/impactmodels/details/134/ (Accessed December 7, 2023).
Khilchevskyi, V. K., Kurylo, S. M., and Sherstyuk, N. P. (2018). Chemical composition of different types of natural waters in Ukraine. J. Geol. Geogr. Geoecol. 27, 68–80. doi: 10.15421/111832
Khokhlov, V., and Yermolenko, N. (2013). On the relationship of the average annual runoff of the Southern Bug River with droughts in the period 1951-2010. Bull. Odessa State Ecol. Univ. 16, 51–59.
Kim, D., Eum, H.-I., Kaluarachchi, J. J., and Chun, J. A. (2019). A sensitivity-based analysis for managing storage capacity of a small agricultural reservoir under drying climate. Agric. Water Manag. 213, 410–418. doi: 10.1016/j.agwat.2018.10.040
Krysanova, V., Zaherpour, J., Didovets, I., Gosling, S. N., Gerten, D., Hanasaki, N., et al. (2020). How evaluation of global hydrological models can help to improve credibility of river discharge projections under climate change. Clim. Chang. 163, 1353–1377. doi: 10.1007/s10584-020-02840-0
Kundzewicz, Z., Krysanova, V., Benestad, R., Hov, Ø., Piniewski, M., and Otto, I. (2018). Uncertainty in climate change impacts on water resources. Environ. Sci. Pol. 79, 1–8. doi: 10.1016/j.envsci.2017.10.008
Landau, Y., and Chernomorov, A. (2020) Southern bug: How to improve water supply in Mykolaiv region. Government courier. Available at: https://ukurier.gov.ua/uk/articles/pivdennij-bug-yak-polipshiti-vodozabezpechennya-mi/ (Accessed December 7, 2023).
Levkovska, L., and Zubko, A. (2021). Current trends in the development of the zone of risk agriculture in the conditions of climate change. Effect. Econ. 3, 16–34. doi: 10.32702/2307-2105-2021.9.12
Lipinsky, V. M., Dyachuk, V. A., and Babichenko, V. M. (2003). Climate of Ukraine. Kyiv, Ukraine: Rayevsky Publishing House.
Lyuta, N., and Sanina, І. (2022). Conditions for the formation of quality state of aquifers in the Southern Bug River Basin. Visnyk Taras Shevchenko Natl. Univ. Kyiv Geology 4, 73–79. doi: 10.17721/1728-2713.99.10
Marcos-Garcia, P., Lopez-Nicolas, A., and Pulido-Velazquez, M. (2017). Combined use of relative drought indices to analyze climate change impact on meteorological and hydrological droughts in a Mediterranean Basin. J. Hydrol. 554, 292–305. doi: 10.1016/j.jhydrol.2017.09.028
Marcos-Garcia, P., and Pulido-Velazquez, M. (2017). Climate change and water planning: is a single reduction coefficient appropriate for the whole river basin district? Ingeniería Del Agua 21:35. doi: 10.4995/ia.2017.6361
McCully, P. (1996). Silenced Rivers: the ecology and politics of large dams. New York, NY, USA: Zed Books.
Ministry of Development of Communities and Territories of Ukraine (2021). National report on the quality of drinking water and the state of drinking water supply in Ukraine in 2020. Kyiv, Ukraine: Ministry of Development of Communities and Territories of Ukraine.
Mozgoviy, A. (2021). Report on the strategic environmental assessment of the program of economic and social development of Mykolaiv for 2022–2024. Mykolaiv, Ukraine: Mykolaiv City Administration.
Müller Schmied, H., Adam, L., Eisner, S., Fink, G., Flörke, M., Kim, H., et al. (2016). Variations of global and continental water balance components as impacted by climate forcing uncertainty and human water use. Hydrol. Earth Syst. Sci. 20, 2877–2898. doi: 10.5194/hess-20-2877-2016
Müller Schmied, H., Eisner, S., Franz, D., Wattenbach, M., Portmann, F. T., Flörke, M., et al. (2014). Sensitivity of simulated global-scale freshwater fluxes and storages to input data, hydrological model structure, human water use and calibration. Hydrol. Earth Syst. Sci. 18, 3511–3538. doi: 10.5194/hess-18-3511-2014
Mykolaiv Regional Council. (2021). Regional program “drinking water of Mykolaiv region” for 2021–2025. Decision dated: 29 September 2021. Available at: https://www.mk-oblrada.gov.ua/User%20Files/decree/1633434255615c3a8f16115.pdf (Accessed December 9, 2023).
NPR. (2022). A Ukrainian city struggles after Russian forces blew up its water supply. Available at: https://www.npr.org/2022/10/08/1127303154/ukraine-mykolaiv-water-supply (Accessed December 9, 2023).
Open data portal. (2022). General indicators of the use of water resources of Ukraine. Datasets. Available at: https://data.gov.ua/dataset/cadastre-water-use (Accessed December 9, 2023).
Order of the Ministry of Health of Ukraine no. 721 of 02.05.2022. (2022). On approval of hygienic water quality standards for water bodies to meet drinking, domestic, and other needs of the population. Available at: https://zakon.rada.gov.ua/laws/show/z0524-22#Text (Accessed December 9, 2023).
Orimoloye, I. R., Ololade, O. O., Mazinyo, S. P., Kalumba, A. M., Ekundayo, O. Y., Busayo, E. T., et al. (2019). Spatial assessment of drought severity in Cape Town area, South Africa. Heliyon 5:e02148. doi: 10.1016/j.heliyon.2019.e02148
Ovcharuk, V., and Gopchenko, E. (2022). Chapter 18 - Engineer substantiation of estimated characteristics of maximum rivers runoff during floods under climate change. Challenges and Management Strategies. doi: 10.1016/B978-0-323-85045-2.00018-2
Ovcharuk, V., Gopchenko, E., Kichuk, N., Shakirzanova, Z., Kushchenko, L., and Myroschnichenko, M. (2020). Extreme hydrological phenomena in the Forest steppe and steppe zones of Ukraine under the climate change. Proc. Int. Assoc. Hydrol. Sci. 383, 229–235. doi: 10.5194/piahs-383-229-2020
Poff, N., Allan, J., Bain, M., Karr, J., Prestegaard, K., Richter, B., et al. (1997). The natural flow regime: a paradigm for conservation of riverine ecosystems. Bioscience 47, 769–784. doi: 10.2307/1313099
Pokhrel, Y., Felfelani, F., Satoh, Y., Boulange, J., Burek, P., Gädeke, A., et al. (2021). Global terrestrial water storage and drought severity under climate change. Nat. Clim. Chang. 11, 226–233. doi: 10.1038/s41558-020-00972-w
Quandt, A., O’Shea, B., Oke, S., and Ololade, O. (2022). Policy interventions to address water security impacted by climate change: adaptation strategies of three case studies across different geographic regions. Front. Water 4:935422. doi: 10.3389/frwa.2022.935422
Regional Office of Water Resources in Mykolaiv Oblast. (2014). Available at: https://mk-vodres.davr.gov.ua/derzhavnij-monitoring-poverkhnevikh-vod-basejnu-r-pivdennij-bug-po-mikolajivskij-oblasti-shcho-provoditsya-laboratorieyu-monitoringu-vod-ta-gruntiv-mikolajivskogo-regionalnogo-upravlinnya-vodnikh-resursiv-na-kinets-2014-roku (Accessed December 9, 2023).
Renöfält, B. M., Jansson, R., and Nilsson, C. (2010). Effects of hydropower generation and opportunities for environmental flow management in Swedish riverine ecosystems. Freshw. Biol. 55, 49–67. doi: 10.1111/j.1365-2427.2009.02241.x
Romas, M., Chunarev, O., and Shevchuk, I. (2006). On the issue of water supply of nuclear power plants in Ukraine when new capacities are commissioned. Sci. Works UkrNDGMI 255, 257–265.
Romashchenko, M. (2012). Scientific principles of land irrigation development in Ukraine. Agrarian Sci. 12, 14–16.
Rubio-Martin, A., Llario, F., Garcia-Prats, A., Macian-Sorribes, H., Macian, J., and Pulido-Velazquez, M. (2023). Climate services for water utilities: lessons learnt from the case of the urban water supply to Valencia, Spain. Climate Serv. 29:100338. doi: 10.1016/j.cliser.2022.100338
Sarker, S. (2021). Investigating topologic and geometric properties of synthetic and Natural River networks under changing climate. Electronic Theses and Dissertations, 965. Available at: https://stars.library.ucf.edu/etd2020/965/ (Accessed September 16, 2024).
Sarker, S. (2022). Fundamentals of climatology for engineers: lecture note. Eng 3, 573–595. doi: 10.3390/eng3040040
Semenova, I. G., Ovcharuk, V. A., and Traskova, A. V. (2015). Droughts and their relationship with some phases of the streamflow regime for Ukrainian Rivers. Eur. Sci. Rev. 8, 1–2. doi: 10.20534/ESR-15-1.2-8-10
Semenova, I., and Vicente-Serrano, S. M. (2024). Long-term variability and trends of meteorological droughts in Ukraine. Int. J. Climatol. 44, 1849–1866. doi: 10.1002/joc.8416
Shakhman, I., and Bystriantseva, A. (2021). Water quality assessment of the surface water of the Southern Bug River Basin by complex indices. J. Ecol. Eng. 22, 195–205. doi: 10.12911/22998993/128858
Shestopalov, V. M., Stetsenko, B. D., and Rudenko, Y. F. (2019). Groundwater of upper Sarmatian aquifer as reserve source for potable water supply to Mykolayiv (Ukraine). Geol. J. 5–17. doi: 10.30836/igs.1025-6814.2019.2.169930
Shevchenko, O., Oliynyk, R., Snizhko, S., Svintsitska, H., and Kostyrko, I. (2020). Indexing of heat waves in Ukraine. Water 12:962. doi: 10.3390/w12040962
Shevchenko, O., Snizhko, S., Zapototskyi, S., and Matzarakis, A. (2022). Biometeorological conditions during the august 2015 mega-heat wave and the summer 2010 mega-heat wave in Ukraine. Atmos. 13:99. doi: 10.3390/atmos13010099
Singhal, A., Jaseem, M., Divya, S. S., Prajapati, P., Singh, A., and Jha, S. (2024). Identifying potential locations of hydrologic monitoring stations based on topographical and hydrological information. Water Resour. Manag. 38, 369–384. doi: 10.1007/s11269-023-03675-x
Snizhko, S., Bertola, M., Ovcharuk, V., Shevchenko, O., Didovets, I., and Blöschl, G. (2023a). Climate impact on flood changes – an Austrian-Ukrainian comparison. J. Hydrol. Hydromech. 71, 271–282. doi: 10.2478/johh-2023-0017
Snizhko, S., Shevchenko, O., Didovets, I., Krukivska, A., and Kostyrko, I. (2020). “Assessment of changes in the main climatic parameters over the territory of Ukraine during the XXI century according to scenarios based on representative concentration pathways (RCP)” in XIV International Scientific conference “monitoring of geological processes and ecological condition of the environment” (Kyiv, Ukraine: European Association of Geoscientists & Engineers), 1–5.
Snizhko, S., Zapototskyi, S., Shevchenko, O., Olexienko, I., Didivets, I., and Bronstert, A. (2023b). Assessment of the water resource potential of the lower Dnipro after the destruction of the Kakhovka reservoir taking into account the impact of climate change. XVII international scientific conference “monitoring of geological processes and ecological condition of the environment”. 7–10 November 2023. Kyiv, Ukraine.
State Statistics Service of Ukraine. (2022). Number of Present Population of Ukraine, as of January 1, 2022. http://db.ukrcensus.gov.ua/PXWEB2007/ukr/publ_new1/2022/zb_%D0%A1huselnist.pdf (Accessed 10 September 2024).
Southern Bug River Basin Water Resources Management. (2023). Available at: https://buvrpb.davr.gov.ua/vodni-resursy/hidrohrafichna-merezha (Accessed December 9, 2023).
State Scientific and Engineering Center for Control and Emergency Response Systems. (2016). Informational and analytical review of the materials of the environmental impact assessment of raising the level of the Oleksandrivka reservoir to the design level of 20.7 m, Ukraine: Kyiv.
Stringer, L. C., Mirzabaev, A., Benjaminsen, T. A., Harris, R. M. B., Jafari, M., Lissner, T. K., et al. (2021). Climate change impacts on water security in global drylands. One Earth 4, 851–864. doi: 10.1016/j.oneear.2021.05.010
Tal, A. (2006). Seeking sustainability: Israel’s evolving water management strategy. Science 313, 1081–1084. doi: 10.1126/science.1126011
texty.org.ua. (2022). On water, but without water. How half a million people in Mykolaiv live without the usual water supply and are trying to restore it. Available at: https://texty.org.ua/articles/107273 (Accessed December 7, 2023).
The Washington Post. (2022). Mykolaiv, port city in Ukraine, shows toll of months without fresh water. Available at: https://www.washingtonpost.com/world/2022/11/09/mykolaiv-water-infrastructure-ukarine-war/ (Accessed June 5, 2024).
Vogel, R. M., Lall, U., Cai, X., Rajagopalan, B., Weiskel, P. K., Hooper, R. P., et al. (2015). Hydrology: the interdisciplinary science of water. Water Resour. Res. 51, 4409–4430. doi: 10.1002/2015WR017049
Vyshnevskyi, V., and Kutsiy, A. (2022). Long-term changes in the water regime of rivers of Ukraine. Kyiv, Ukraine: Naukova dumka.
Vyshnevskyi, V., and Kutsiy, A. (2024). Emergency situations in Ukraine. Kyiv, Ukraine: Nika-Center.
Vyshnevskyi, V., Shevchuk, S., Komorin, V., Oleynik, Y., and Gleick, P. (2023). The destruction of the Kakhovka dam and its consequences. Water Int. 48, 631–647. doi: 10.1080/02508060.2023.2247679
World Bank (2019). The role of desalination in an increasingly water-scarce world. Washington, DC: World Bank.
World Bank (2021). Ukraine: building climate resilience in agriculture and forestry. Washington DC: World Bank.
World Resources Institute. (2024). Aqueduct, water risk atlas. Available at: https://www.wri.org/aqueduct (Accessed May 17, 2024).
Yuan, G. Y., Sarker, S., Sarker, T., and Leta, O. (2022). Analyzing the critical locations in response of constructed and planned dams on the Mekong River basin for environmental integrity. Environ. Res. Commun. 4:101001. doi: 10.1088/2515-7620/ac9459
Zabulonov, Y., Boychenko, S., Zholudenko, O., and Buhera, M. (2018). The tendencies of climate change and the runoff in the middle-lower part in the basin of Southern Bug River (in the region of location of the south-Ukrainian nuclear power plant). Geofizicheskiy Zhurnal 40, 286–300. doi: 10.24028/gzh.0203-3100.v40i5.2018.147498
Keywords: water supply, water as a weapon, climate risk assessment, water availability, WaterGAP2
Citation: Snizhko S, Didovets I, Shevchenko O, Yatsiuk M, Hattermann FF and Bronstert A (2024) Southern Bug River: water security and climate changes perspectives for post-war city of Mykolaiv, Ukraine. Front. Water. 6:1447378. doi: 10.3389/frwa.2024.1447378
Edited by:
Johannes A. C. Barth, Friedrich-Alexander-Universität Erlangen-Nürnberg, GermanyReviewed by:
Shiblu Sarker, Virginia Department of Conservation and Recreation, United StatesDavid Robert Piatka, Karlsruhe Institute of Technology (KIT), Germany
Copyright © 2024 Snizhko, Didovets, Shevchenko, Yatsiuk, Hattermann and Bronstert. This is an open-access article distributed under the terms of the Creative Commons Attribution License (CC BY). The use, distribution or reproduction in other forums is permitted, provided the original author(s) and the copyright owner(s) are credited and that the original publication in this journal is cited, in accordance with accepted academic practice. No use, distribution or reproduction is permitted which does not comply with these terms.
*Correspondence: Sergiy Snizhko, c25pemhrb0BrbnUudWE=