- 1Department of Civil Engineering, Indian Institute of Technology (IIT) Bombay, Mumbai, India
- 2Radhe Greens, Maharashtra Industrial Development Corporation (MIDC) Zone, Hingna, Nagpur, India
- 3Department of Irrigation and Drainage Engineering, Govind Ballabh Pant University of Agriculture and Technology, Pantnagar, India
- 4Division of Agricultural Engineering, ICAR-Indian Agricultural Research Institute, New Delhi, India
- 5Department of Architecture, Vivekanand Education Society's College of Architecture (VESCOA), Mumbai, India
- 6Civil Engineering Department, Faculty of Engineering, Minia University, Mansoura, Egypt
- 7Structural Diagnostics and Analysis Research Group, Faculty of Engineering and Information Technology, University of Pécs, Pécs, Hungary
- 8Agricultural Engineering Department, Faculty of Agriculture, Mansoura University, Mansoura, Egypt
Introduction: Ancient water harvesting systems, such as those from the Indus Valley Civilization (~3500 BCE), have been vital for irrigation and climate resilience, especially in arid regions. One such prominent system in South Asia, called tank irrigation, initially thrived through community management but declined post-independence due to colonial policies and neglect in Sri Lanka and India. This study evaluates current policy frameworks and rehabilitation programs to enhance the resilience of these systems in India, develop strategies for their protection and adaptation to climate change, and integrate global lessons for sustainable development.
Methods: A systematic meta-analysis of grey literature was conducted to aggregate data on policy constraints. Policy analysis involved detailed investigations of relevant documents, regulations, and comparative analyses of frameworks at regional and national levels. Pilot projects on tank rehabilitation were assessed through reported case studies and field surveys to gauge impact. Thematic analysis was used to explore the global potential of these systems in climate resilience and overall environmental sustainability.
Results: The analysis showed that pilot projects for tank rehabilitation had limited success in achieving sustainability under current climate conditions. Tank irrigation systems are crucial for adapting to extreme weather, including floods, droughts, and heat waves, replenishing groundwater, reducing soil erosion, and ensuring reliable water supplies. Traditional water harvesting technologies support 17 Sustainable Development Goals (SDGs), including clean water access, hunger reduction, gender equality, and climate action. Integrating AI and machine learning in water management benefits disaster response, while eco-tourism aids system maintenance and cultural awareness.
Discussion: The study underscores the need for policy reforms to enhance tank rehabilitation and institutional arrangements. It calls for increased beneficiary participation and constitutional recognition of current practices. Strategic, national-scale assessments and resilience targets are recommended to improve the effectiveness of such water harvesting systems in mitigating natural hazards and enhancing environmental services.
1 Introduction
During the next few decades, India will suffer seasonally and regionally with severe water shortages. Agricultural water consumption, the most significant contributor to the demand for water, has been increasing for the past few decades in response to the growing and more affluent population. It is well known that, in India, water harvesting structures such as tanks, ponds, lakes, etc., have traditionally been used to collect water, particularly in rural settings where agriculture remains the principal occupation. Traditionally, these structures were designed to facilitate rainwater storage during the monsoon season, which is applied in various ways during the non-monsoon season (Ammar et al., 2016; Sugam et al., 2018). The ancient tank structures in South Asia, including those in Dholavira from the Indus Valley Civilization dating back to around 3,500 BCE, underscore the region’s enduring water management and tank irrigation tradition (Lal, 2002; Singh, 2008). These early systems were marked by sophisticated designs featuring large reservoirs, step wells, and channels, setting a precedent for sustainable water management through community involvement. This tradition continued and evolved throughout history, notably with the cascading tanks, designed and constructed over 2,000 years ago by rulers, philanthropists, and local communities due to their extraordinary engineering and managerial skills (Gunnell et al., 2007; Srinivasan, 2016). Numerous tanks and reservoirs were built in India and Sri Lanka during the medieval period. For instance, the Vijayanagara Empire in India (early 13th century CE to mid 17th century CE) developed extensive tank systems for agriculture and drinking water, illustrating the crucial role of community-managed water resources (Lal, 2002; Singh, 2008). Similarly, in Sri Lanka, the ancient Sinhalese civilization (3rd century BCE to the 10th century CE) constructed large-scale irrigation systems, such as the Parakrama Samudra, reflecting advanced engineering and collective management practices (Shannon and Manawadu, 2007). These tank cascade systems have been protected and maintained for several centuries by institutional arrangements that are still in place today. The sustenance of such water harvesting technologies is paramount even in the 21st century, given rising water insecurity due to environmental and climate change implications and anthropogenic stressors (Bardhan, 2000; Palanisami and Easter, 2000; Geekiyanage and Pushpakumara, 2013). Apart from the tank’s primary applications of supporting irrigation and providing water for domestic and live-stock management purposes, they have a substantial role to play in developing resilience against the negative consequences of natural hazards such as floods and droughts (Palanisami et al., 2010; Siderius et al., 2015; Jayanthi and Keesara, 2021). Even though these old systems are from generations ago, they have degenerated despite age due to unwarranted political interventions and changing socio-economic dynamics. All this eventually started during the colonial period (early 19th century) when water policy shifted from minor irrigation (having irrigated areas <2,000 hectares) to medium irrigation (between 2,000 and 10,000 hectares) and major irrigation (>10,000 hectares). These shifted policies focused more on revenue generation from these traditional structures instead of their protection and context-based applications (Mosse, 1997; Narayanamoorthy, 2007; Shah, 2008; Siderius et al., 2015). As a result of policy changes and technological advancements, local communities have been compelled or encouraged to switch from using tanks for irrigation to relying on private wells. Technologies such as energized tube wells and policies offering free pumping energy for farmers, particularly in regions like Tamil Nadu, have facilitated this shift (Srinivasan, 2015; Palanisami and Nagothu, 2024). Consequently, the ownership of common pool resources, such as tank systems, deteriorated, and village-level institutions disappeared. This management transfer from local communities to colonial governments further exacerbated the decline of traditional water management practices (Mosse, 1997; Narayanamoorthy, 2007; Shah, 2008; Siderius et al., 2015).
The unprecedented degradation of tank systems and the further expansion of groundwater-based well irrigation continued even after India’s independence in 1947 (Figure 1; Shankar et al., 2011; Narayanamoorthy and Jothi, 2019). Nonetheless, the degradation slowed down post-colonization until the 1980s. Meanwhile, the green revolution in India (which started during the 1960s) significantly experienced an unprecedented rise in pump-based well irrigation systems. This was enabled by the commencement of the energization of the groundwater extracting mechanisms in the early 1980s. Due to this, a further shift occurred from community-based water sources (like tanks) to individual-based water sources (Narayanamoorthy, 2010).
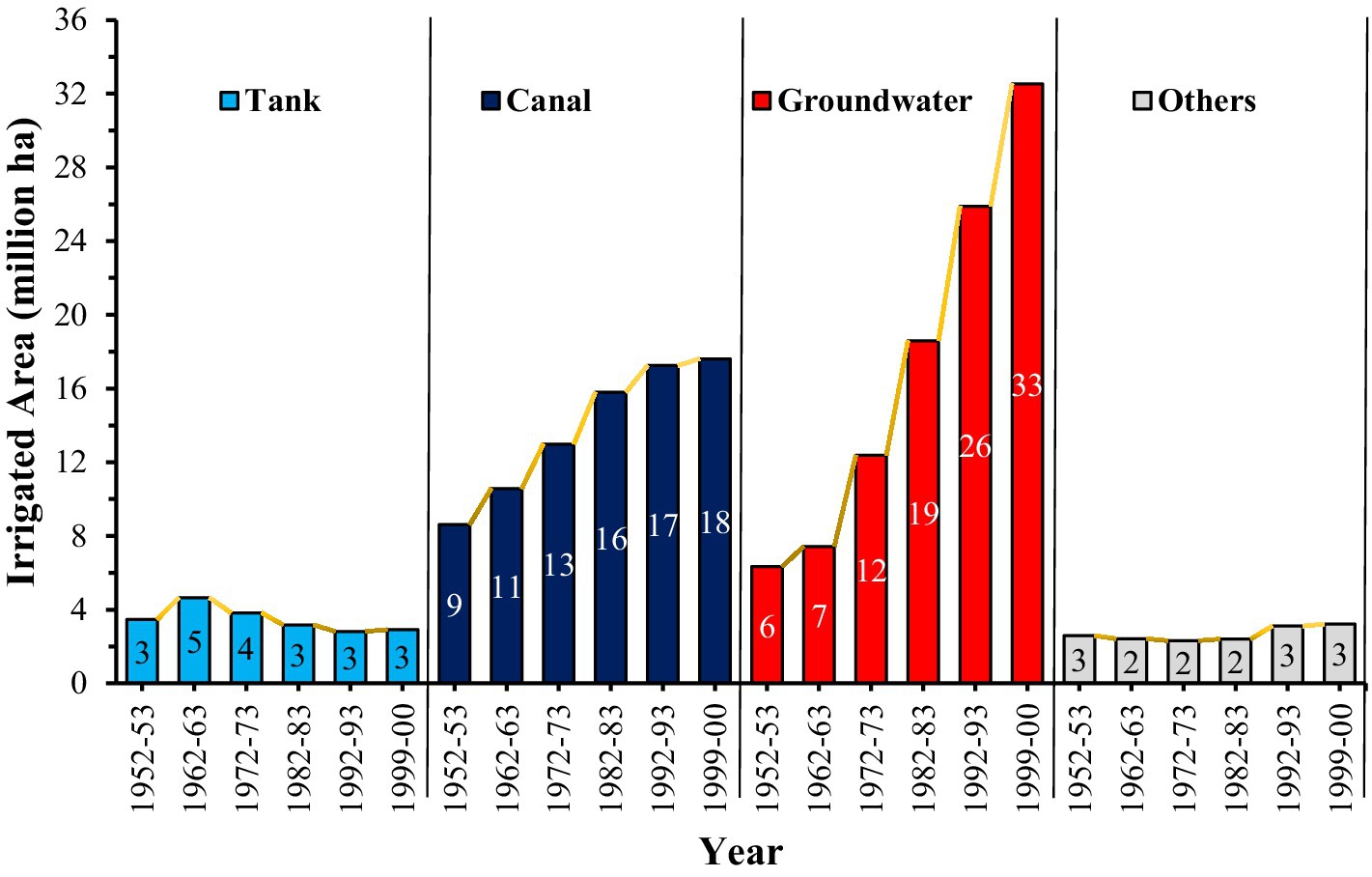
Figure 1. Source-wise area under irrigation in post-independent India (Source: Water and related statistics, central water commission).
In fact, by the 1980s, well irrigation, including both tube wells and communal open wells, accounted for the maximum area under irrigation (Sakthivadivel and Gomathinayagam, 2006). Overall, a sharp decline in tank irrigated area was observed from 3.6 million hectares in 1950 to 1.7 million hectares in 2014 (~53% decrease; also refer to (Figures 1 to 5). Other associated socio-environmental benefits, such as groundwater percolation, community-led management, maintenance of tanks, and enriching biodiversity in the vicinity of the cascading tanks, collapsed. This has created a considerable imbalance in the hydrological and ecological systems (Reddy et al., 2018; Venkatachalam and Balooni, 2018). All this eventually transmuted India as the world’s largest extractor of groundwater resources, accounting for ~25% of the global extraction (Patel et al., 2020). Despite this, more than 54% of the groundwater wells in India have been observed in a critical to overexploited state concerning depth to water level (Saha et al., 2018). Therefore, the replaceability of tanks with wells presaged a wrong conception, predominantly because tanks could create a sense of ownership for maintenance, complementing groundwater development and, thereby, water security. In general, the collective distress of the changed water policy amidst institutional neglect is a modern challenge to continuing tank-based irrigation practices, especially in the semi-arid or dry region of India (Mosse, 1997; Narayanamoorthy, 2007; Shah, 2008; Siderius et al., 2015; Reddy et al., 2018).
Water management and distribution are now centralized under the current situation. People’s reliance on the government has hampered community engagement in water management and caused the traditional water harvesting method to fail (Mane and Shinde, 2014). However, as the water situation worsens, there is an urgent need for systemic reform in water management and the revival of traditional systems (Glendenning et al., 2012). Ancient Indian tradition has a long history that can be traced back to the science of water conservation and rainwater harvesting (Laskar, 2022). Historically, various methods have been employed for rainwater collection depending on need and requirement, and civilizations have flourished close to numerous river systems. In many semi-arid areas of the world, tank cascades are the primary source of water for agriculture requirements (Jayasena et al., 2011; Ramabrahmam et al., 2021). These systems have been neglected over the last decades because of the significance put on large dams. Recently, governments and people have started to understand the adverse effects of large dams and the advantages of tank cascades (Khagram, 2004; Sakthivadivel and Gomathinayagam, 2006; Siderius et al., 2015; Reddy et al., 2018). These cascade tanks were neglected over time due to the widespread usage of agricultural bore wells, bund flagging, and siltation.
Irrigation from the tank is dwindling, and groundwater irrigation is expanding across India, especially in drought-prone areas, due to a decline in tank irrigation. Over time, a need was felt for the revival, restoration, and rehabilitation of these decaying tanks to attain surface water–groundwater security, especially those meant for irrigation and percolation purposes. As a result, scientific and technological studies are being conducted to evaluate the current situation and suggest appropriate mitigating measures to revive traditional systems and wisdom (Mbilinyi et al., 2005; Yazar and Ali, 2016). The objectives for such rehabilitation programs were driven by location-specific requirements to achieve overall sustainability in water management (Reddy et al., 2018; Roy and Kumari, 2019). However, the effectiveness of these programs was often influenced by the priorities and capacities of the implementing agencies. As a result, strategizing revival on a case-to-case basis becomes difficult in addition to changing political and socio-economic set-ups. By the early 1980s, rehabilitation programs had been initiated in support of the government, non-government, and foreign organizations across India’s semi-arid and dry states. Most of the interventions were made via repairing tank structures as a whole or part, watershed or rural-landscape development, and rebuilding institutional management systems (Sakthivadivel and Gomathinayagam, 2006; Reddy et al., 2018). According to Saxena and Brighu (2021), the revival process must be supported by public initiative and engaged public engagement. Nevertheless, the impacts of the diverse interventions on the existing tank system need to be studied, and thus, they qualify to become one of the primary objectives of this study. Additionally, as these established systems provide strategies for climate change adaptation by resisting extreme weather conditions, including floods, droughts, and heat waves, this study has further focussed on understanding how Tank Cascade Systems (TCS) and comparable techniques might be used to lessen the effects of these catastrophes and maintain a consistent water supply during environmental stress. Moreover, traditional water harvesting systems also emerge as an intriguing area of research concerning natural disasters. This paper thus also explores the evolving understanding of how these systems interact with and respond to natural disasters, contributing to disaster risk reduction strategies.
Besides, traditional water harvesting systems, exemplified by the cascading tanks and analogous methodologies worldwide, are paramount in advancing societal well-being. They may make a substantial and demonstrable contribution to socio-economic development, aligning with the 17 Sustainable Development Goals (SDGs). Thus, it becomes imperative to assert if extensive empirical evidence supports these harvesting technologies’ positive role on broader socio-economic and sustainability objectives as outlined in the SDGs. This study explores how these systems advance these SDGs’ achievement by enhancing access to clean water, increasing agricultural output, minimalizing the impacts of natural hazards, reducing poverty, empowering disadvantaged groups, and promoting gender equality. In an era of technological advancements, this study further delved into the intersection of traditional water harvesting with Artificial Intelligence (AI) and Machine Learning (ML) approaches. In order to achieve these objectives, A systematic meta-analysis of grey literature was conducted to aggregate data on policy constraints. Policy analysis involved detailed investigations of relevant policy documents, regulations, and comparative analyses of frameworks at regional and national levels. Pilot projects on tank rehabilitation were assessed through reported case studies and field surveys to gauge impact. Thematic analysis was used to explore the global potential of these systems in climate resilience and environmental sustainability. Overall, this study presents a comprehensive journey through the diverse dimensions of traditional water harvesting, shedding light on their global significance in shaping a sustainable and resilient future.
2 Extent of tank irrigation in India
In India, the distribution of tanks is uneven across states, influenced by a combination of economic and natural factors. Geological setup, hydrological conditions, and rainfall patterns significantly affect tank density (Massuel et al., 2014; Bharucha, 2019). For example, rocky substrata and undulating topography provide natural storage and gravity flow conditions ideal for establishing tanks, while the rugged granite layer beneath limits groundwater percolation. Consequently, the areas around the Deccan Plateau, encompassing parts of Central and Southern India, have required the construction of more tanks than any other region in India (Pant and Verma, 2010; Reddy et al., 2018). These regions also experience the vagaries of the Indian monsoon system, which remains highly unpredictable. Furthermore, the East coast of Southern India faces frequent storms and cyclones during the North-East monsoon season (from October to December) (Varikoden and Revadekar, 2018), making the presence of tanks crucial for managing excess rainfall and mitigating flood risks. According to the MI census of 2000–01, the number of tanks in use in India was 0.23 million (Figure 2). Additionally, an estimated 42,955 tanks were not in use in 2000–01, and this number increased to 85,807 tanks in 2010–11. It has been demonstrated that the agricultural productivity and potential of India’s water storage primarily depend on the monsoon system. However, a significant challenge exists in 40% of the landmass under arid climatic conditions, which receives annual rainfall ranging between 500 to 1,000 millimeters (Yadav and Lal, 2018). Consequently, the water supply to tank feeder channels in these regions is inadequate and unreliable. This situation is further exacerbated by urbanization and the encroachment of tank catchment and feeder channel areas (Palanisami, 2006; Palanisami et al., 2010). In general, the number of tanks and ponds has been reported to vary between 200,000 and 350,000, of which around 60% are located in the arid and semi-arid areas of the Deccan Plateau regions (Sakthivadivel and Gomathinayagam, 2006). Whereas the number of tanks from other sources has been reported to be 208,000, more than 60% (159,000) of the tanks are located alone in Southern Indian states. However, the functional tanks are limited to 35% in the South, followed by 20% in the West, 16% in the East, and 14% in the North, while the data for the North-East region are unavailable (Palanisami et al., 2010; Reddy et al., 2018). This highlights the critical situation at a national scale, as the increasing number of non-functional tanks threatens water security, agricultural productivity, and climate resilience across diverse climatic zones in India.
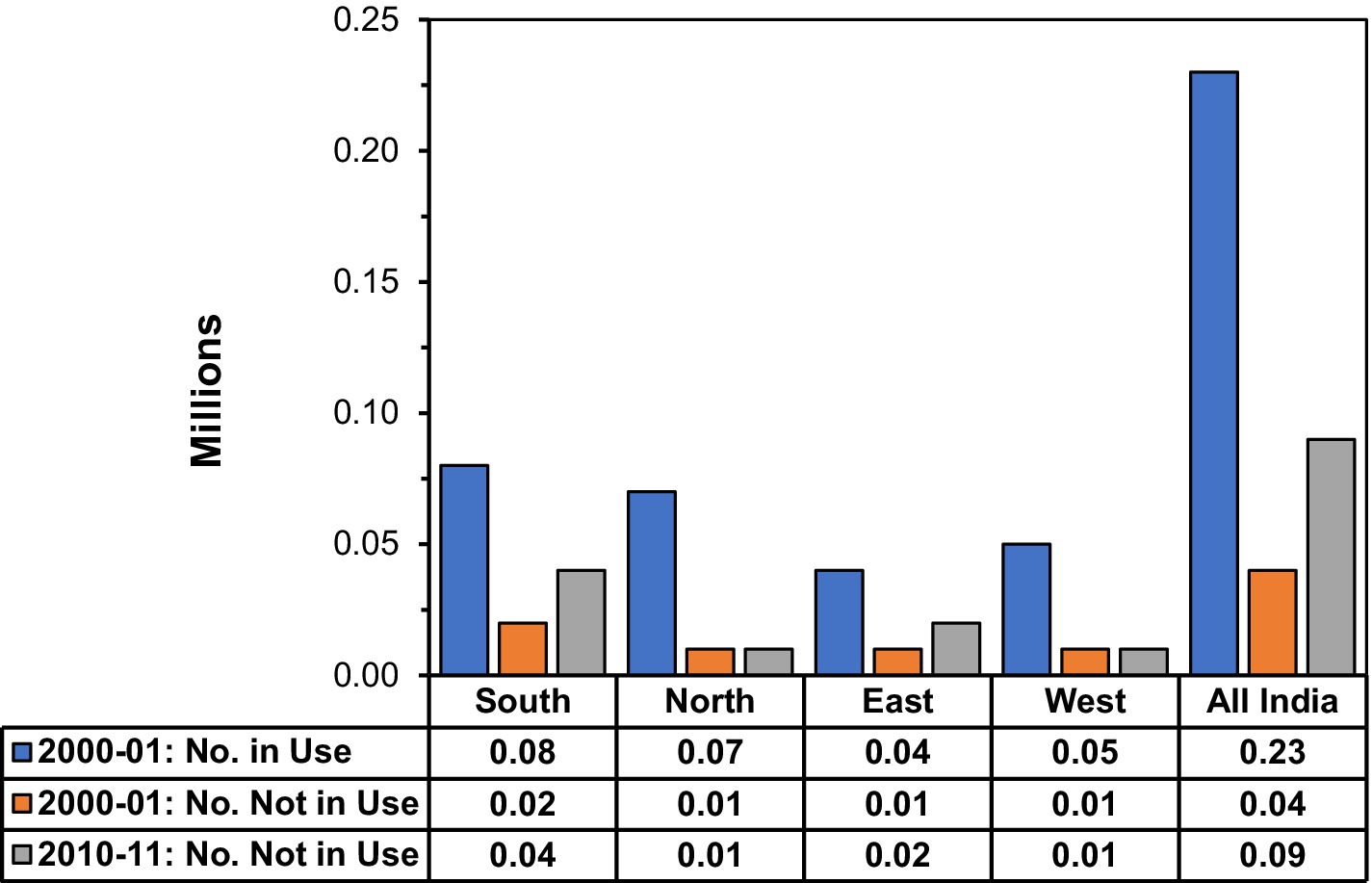
Figure 2. The number of tanks in use and not in use across the different regions of India (in millions). Source: Government of India (different years), compiled from minor irrigation census, ministry of water resources.
The threat of the declining tank numbers was reflected in the region-wise tank irrigated area (Figure 3). Across India, a decline of ~217% in tank-irrigated areas was observed between 1972 and 2014. As a result, agricultural water security in the tank-fed-based irrigation areas was impeded. However, a rise in the total irrigated area was recorded between 2003 and 2008. One reason for this anomaly was the initiatives of tank rehabilitation and modernization programs, especially in the Southern and Eastern states. Region-wise, the East declined by ~564%, the West by ~307%, and though the South remained dominant for the tank numbers (Figure 4), the irrigated area declined by ~226%. Besides this, the share of tank irrigation in the South witnessed a rapid decline from 58% in 1972 to 45% in 2003. However, revival initiatives in the last few decades lifted their share to 56% by 2014. Contrarily, both East and West recorded a rise in their share of tank irrigation from 17 to 21% and 12 to 17% between 1972 and 2008, respectively. Nevertheless, merely analyzing the region-wise percentage share of tank irrigation may be misleading since tank-irrigated areas, as a whole, declined considerably in the last four decades, except for North (Reddy et al., 2018). Therefore, the relative importance of region-wise tank irrigation against overall irrigation was analyzed to address this gap, as shown in Figure 5.
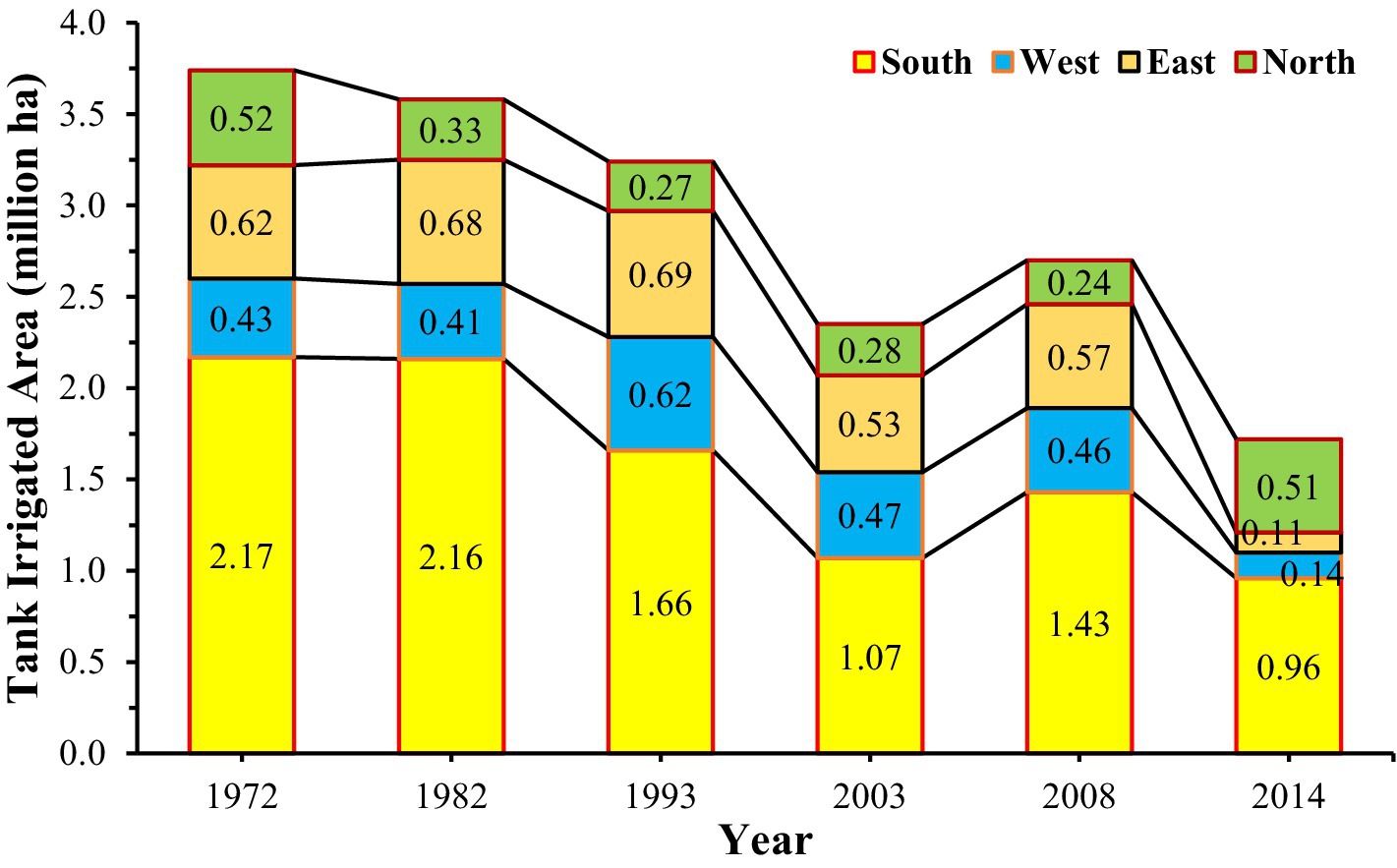
Figure 3. Region-wise tank irrigated area in million hectares (ha) across India (Source: directorate of economics and statistics, ministry of agriculture and farmers welfare).
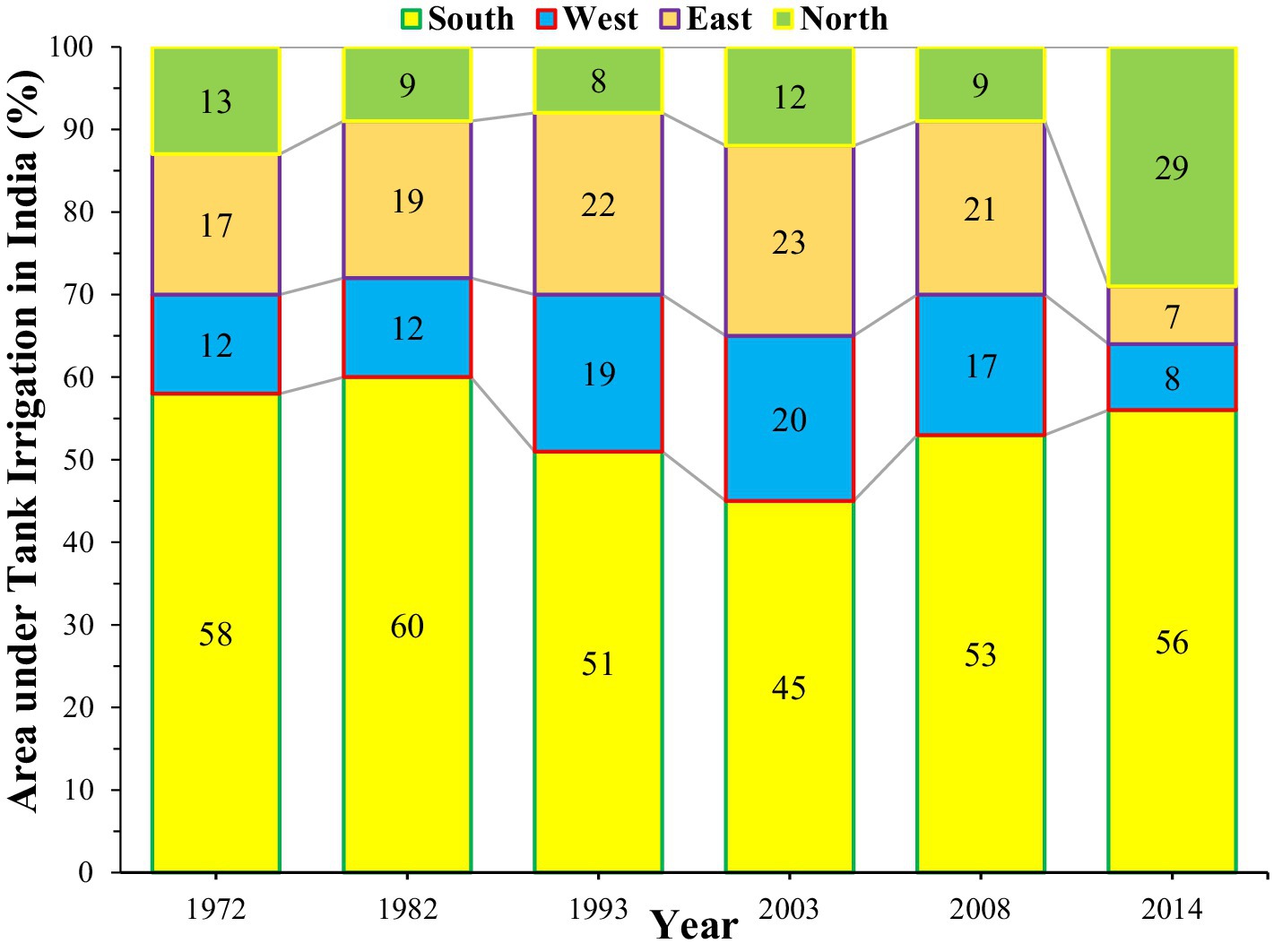
Figure 4. Percentage change in tank irrigation area relative to India’s total tank irrigated area by region. The figure displays the data across four distinct regions: East, West, North, and South, illustrating the regional variations and trends in tank irrigation over time. (Source: Directorate of Economics and Statistics, Ministry of Agriculture and Farmers Welfare).
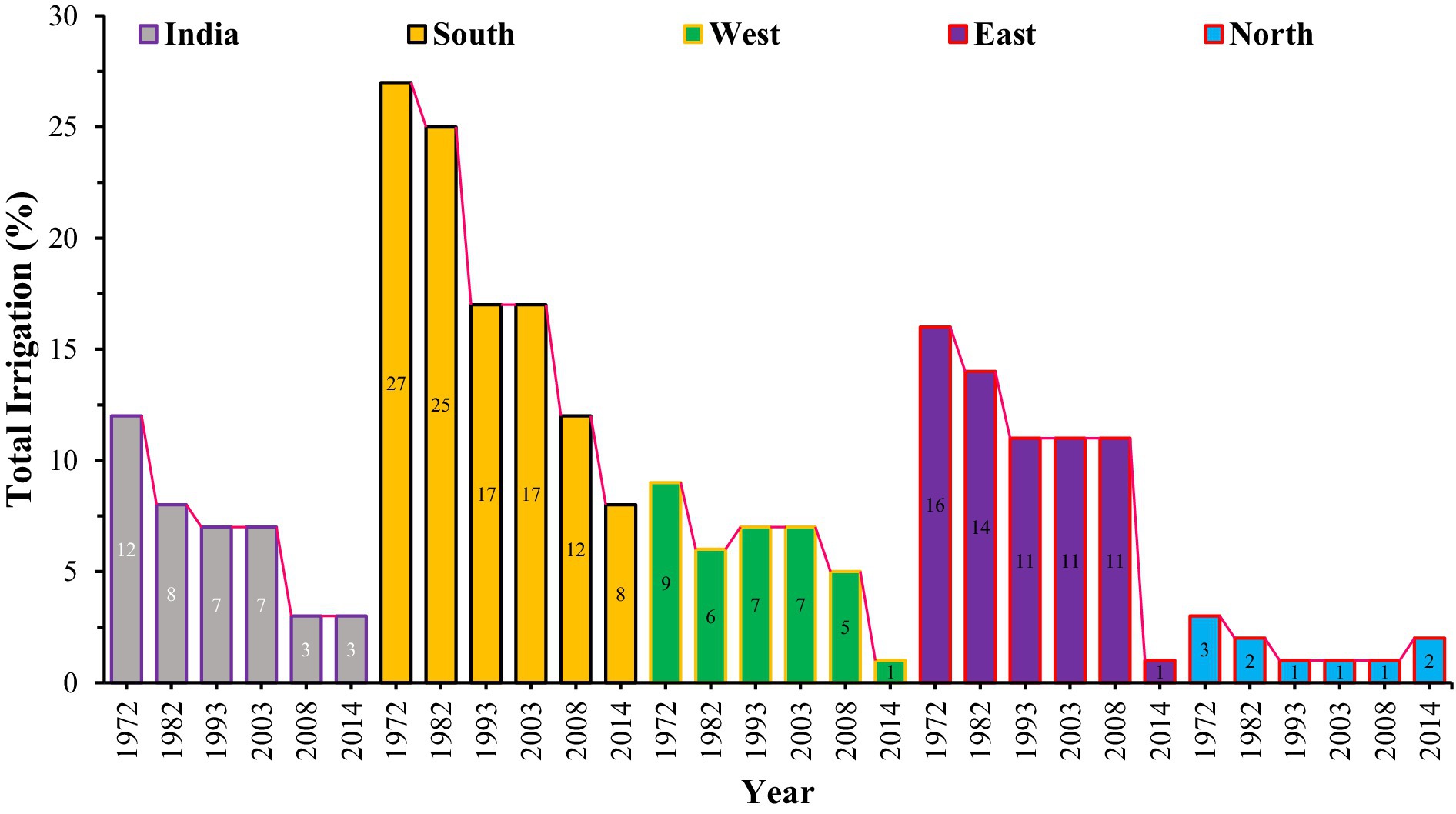
Figure 5. Region-wise percentage change in the relative importance of tank irrigation across India (Source: Directorate of Economics and Statistics, Ministry of Agriculture and Farmers Welfare).
3 Tank rehabilitation programs, policies, and implications
Over the past four decades, tank rehabilitation programs have been driven by several pressing reasons, including the benefits of tanks for groundwater recharge, as well as considerations of equity, stability, and security (Reddy et al., 2018). The knowledge of this hydrogeological behavior (groundwater storage potential) provided scope for replenishing wells in the tank command and tank bed areas. This facilitated irrigation for both tank-command farmers as well as tank-bed farmers. However, the rising struggle among the stakeholders is becoming prevalent due to changing socio-economic and market contexts. The field experience from other studies has shown that not considering the community’s perception while designing rehabilitation and revival programs may lead to a conflict of interest (Sakthivadivel and Gomathinayagam, 2006; Narayanamoorthy, 2007; Palanisami et al., 2010; Reddy et al., 2018). For instance, in Tamil Nadu, degraded tanks provided several services to the village communities, such as livestock management, obtaining silt from the tank bed for their farm, etc. According to Narayanamoorthy (2007), nearly 75% of the villagers utilized tank silt for agricultural purposes, significantly enhancing soil fertility. Under such a context, reviving tanks may be futile as it might adversely affect communities currently benefitting from them. Similarly, command area farmers and tank bed farmers were observed practicing flood irrigation in silted (degraded or unmanaged) tanks. Studies by Sakthivadivel and Gomathinayagam (2006) indicate that 60% of these farmers preferred the current state of the tanks for flood irrigation due to the easy access to water and silt. Reviving tanks in this context might go against the interest of the tank bed farmers (primarily landless) because the intervention in terms of de-siltation, catchment treatment, etc., may displace them. However, in another context, de-siltation may be an appropriate option if well irrigation in the command area is a common source of irrigation, as more percolation helps groundwater development and, thus, yields from the wells (Palanisami et al., 2010). In fact, studies have shown that de-siltation can improve groundwater recharge by up to 30%, benefiting well irrigation (Reddy et al., 2018). With such conflict of interests, it becomes apparent that designing policies for modernization and rehabilitation programs must follow Participatory Rural Appraisal (PRA) in the village context to contemplate local perspectives (Chambers, 1994). Nonetheless, the Water User’s Association (WUAs) must be part of the project in urban and rural contexts to collect wide-ranging inputs before the program implementation (Aubriot and Prabhakar, 2011; Siderius et al., 2015). WUAs are community-based organizations that manage and maintain water resources, including tank systems. Formed with local and sometimes governmental support, WUAs are governed by elected committees that handle water allocation, infrastructure maintenance, and conflict resolution. They face challenges such as limited resources and technical expertise but offer opportunities for enhanced local participation and sustainable management (Chaube et al., 2023). Coherent to these contexts, Table 1 serves as a foundation for understanding why tank rehabilitation and revival programs are necessary based on documented field experiences from elsewhere research.
Given the tank rehabilitation context, several initiatives across India have been taken since the 1980s in the direction of the tank’s development and ecosystem, as listed in Table 2. It becomes imperative at this point to analyze the inclusive implications of program policies. Tank rehabilitation started around four decades ago, with the primary objective of physical rehabilitation focusing merely on increasing productivity in agriculture. This becomes more evident from the Command Area Development (CAD) model of the 1970s and the European Economic Community (EEC) model adopted in Tamil Nadu in the 1980s. Until now, little emphasis was placed on alleviating poverty, given the wide-ranging perspective of mitigating natural hazards under environmental and climate change scenarios. During the early revival policies, the focus was limited to generating employment opportunities, local communities’ engagement for institutional development, and considering the tank cascade system as a whole rather than an individual tank. However, by the late 1990s and early 2000s, the focus of the program policy design shifted to alleviating poverty coupled with enhanced agricultural productivity, as evident from the revised EEC model adopted in Pondicherry and the creation of Jala Samvardhane Yojana Sangha (JSYS) in Karnataka under World Bank funding. Attention to the landless and poor apart from farming, fishing, Self-Help Groups (SHGs), and other local communities was given by developing Tank User’s Groups (TUGs), thereby involving them together under income generation activities via rehabilitation projects. Given water scarcity and security issues, the programs launched post-2000 focused more on cascades of tanks (system tanks) for catchment development, de-siltation, bund strengthening, and improvement of supply channel and control structures. This shift occurred due to experiencing more advantages while augmenting structural treatments under semi-arid or dry conditions in cascading tanks against the individual tank (non-system tank). For instance, canal lining for provisioning of water supply to attain last-mile connectivity in the cascading network resulted in water conservation by about 21% (Reddy et al., 2018). Altogether, the aforementioned conservation efforts paved the way for public-participatory engagement, given that tank functionalities were restored to achieve environmental and climate change resilience.
Despite several advances in rehabilitation programs across decades, threats and challenges continued to exist. In this case, a significant criticism observed in the rehabilitation works is its consideration as a single-time activity. This act has widely given rise to the absence of post-rehabilitation backing, such as for minor structural repairs or modifications, provisions of getting additional funds, and further assistance in training and capacity building. As a result, the absence of continuous support impairs the effectiveness of the rehabilitation efforts. Consequently, the program fails to achieve its objectives fully and does not deliver the intended benefits to the communities. Even though Non-Government Organizations (NGOs) play a linking role between implementation agencies and farming communities, resulting in as high as 30% of the voluntary contribution to the project budget by farmers (Sakthivadivel and Gomathinayagam, 2006), these practices are still less prevalent in recent decades. Moreover, the key concern remains the lack of effective involvement of those stakeholders for whom the tank is still the primary income source, especially in decision-making, implementation, operation, and maintenance of the rehabilitated tanks. Besides this, the modern challenges in terms of anthropogenic stressors, such as rising water demand due to demographic explosion against declining water availability (Hanjra and Qureshi, 2010) and environmental and climate change impacts in terms of unprecedented floods and recurrent droughts (Mishra, 2020), indicated the urgency of bringing coherent programs at a national scale using a participatory-bottom-up approach.
4 Strategies for policy initiatives
Numerous studies have conducted cost–benefit analyses of tank rehabilitation and have consistently demonstrated that the benefits, such as improved water availability and agricultural productivity, significantly outweigh the associated costs (Palanisami, 2006; Reddy and Behera, 2009a). However, a few studies have presented contrasting findings, suggesting that the costs of tank rehabilitation may exceed the benefits in specific contexts (Sakthivadivel and Gomathinayagam, 2006; Reddy and Behera, 2009b). Policy initiatives addressing mismanagement in tank irrigation supply have generally lacked coherence. Additionally, efforts to reconsider tanks as hazard mitigators within disaster management planning have not been systematically integrated. Furthermore, initiatives to alleviate poverty and develop primary livelihood opportunities through tank rehabilitation have been inconsistent and fragmented. It becomes even more evident that merely 10% of the total degraded tanks have been rehabilitated (Reddy et al., 2018). At the same time, approximately equal numbers of rehabilitated tanks are being degraded (Sakthivadivel and Gomathinayagam, 2006). On the flip side, the implementing agency for tank rehabilitation continued following its priorities without considering the changing socio-economic dimensions from case to case. Following the ‘one model fits all’ may not be a suitable ideology, at least from an Indian perspective, where the significance of tank irrigation varies across regions and states. Therefore, there is a need to bring policy changes considering local and regional variabilities with a primary focus on addressing collapsed livelihood opportunities for local communities (such as landless farmers), food insecurity, environmental water mismanagement, and regional and ecological imbalances in the vicinity of tanks. More specifically, strategies for real-time tank policies need to be introduced in the rational way of drafting policies. A list of possible policy-based interventions, including those from the successful pilot projects, is described in Table 3.
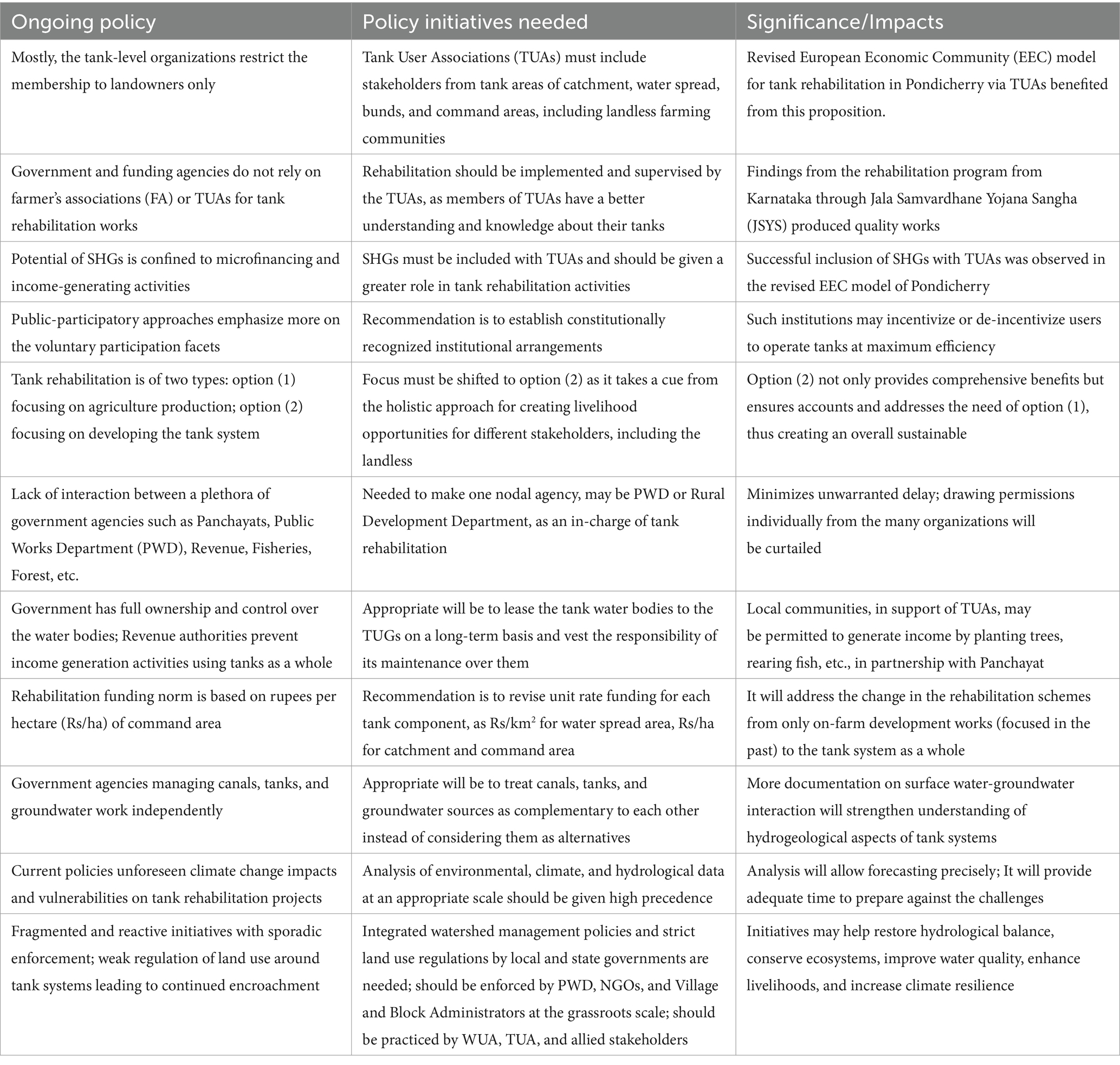
Table 3. Major policy initiatives required for sustainable tank rehabilitation programs in India (Sakthivadivel and Gomathinayagam, 2006; Reddy et al., 2018).
There are several states in India, especially from the East (for example, Odisha) and West regions, where the critical attention of tank rehabilitation is still on increasing agricultural productivity (Sakthivadivel and Gomathinayagam, 2006). The major drawback of such policies is that the benefits remain concentrated on the landholders while the landless remain merely on wages. Additionally, this increases vulnerability to agricultural drought, specifically during erratic or low rainfall scenarios, wherein poor or marginal tank-fed farmers not having access to well-irrigation abandon cropping in the midway and migrate forcefully outside their village for sub-optimal livelihood opportunities. However, states from the South, such as Tamil Nadu, have moved further to improving livelihood opportunities apart from merely focusing on agricultural outputs. Strategically, policy initiatives, in this case, are more sustainable in the modern context. This is because it takes cues from the holistic approach for creating income sources for different stakeholders, including the landless farming communities, during non-monsoon seasons and drought. As a result, every household in the village receives benefits in one way or another, reflected in their per-capita income (Chowdhury and Behera, 2018).
For improved management of the tank system, the functionalities of the elementary components must be clarified beforehand to the stakeholders. These include three components: one, service providers who may be a government through bilateral agencies; two, service facilitators such as local NGOs; and three, service users like TUGs, WUAs, and local communities. Their continuous interaction and cooperation are much needed to sustain tank irrigation. As the TUGs represent the lowest action body, federation at the tank cascade, basin, and state levels are needed for effective governance. However, instead of bringing several agencies together at the cascade level, fixing one agency as a nodal would be more appropriate. In this process of designing tank rehabilitation, capacity building is the core, and sustainability cannot be attained without it. It should start before planning rehabilitation and considering all stakeholders, including SHGs and the landless. The first part of the capacity building should focus on strengthening TUGs, creating awareness, and introducing income-generating sources to SHGs. Subsequently, NGOs can moderate their involvement in rehabilitation programs. The second part of training should start during rehabilitation, primarily emphasizing the water security, water management, operation maintenance, and natural hazard mitigation aspects of tanks. At the same time, the last part of the training should start once the rehabilitation works are completed. This part should focus on managing tanks by TUGs on their own, expanding their activities to federation levels (Sakthivadivel and Gomathinayagam, 2006). Such capacity-building strategies should consider regional requirements to cater to the tank communities’ local needs. Therefore, appropriate tank rehabilitation measures have the potential to provide immediate benefits and sustain them in the long run. In addition, such attempts, through public-participatory approaches, have the potential to buffer the negative consequences of environmental and climate change impacts in general and natural hazards in specific.
Under fiscal constraints, the crux of the rehabilitation programs should focus on achieving a balance between creating new technologies and utilizing existing technologies (tank irrigation) with obligatory modifications in the revised context. India has millions of tanks spread across vast geographical areas of arid regions. Systematic mapping of the system and non-system tanks1 by the geotagging technique and categorizing them as functional and non-functional tanks is paramount. This would allow the demarcating of actual tank boundaries, which, in turn, check encroachment, if any. Also, developing land use and land use cover maps of tank watersheds using manual and automated supervised classification techniques by employing Google Earth Pro and Geographical Information System (GIS) (Liladhar Rane et al., 2024; Pande et al., 2024) can help provide recent trends of tank deterioration, if any. Moreover, addressing encroachment of catchments and tank beds requires stringent land use regulations, proactive community engagement, and enhanced legal frameworks. Effective enforcement of these regulations is essential to prevent unauthorized activities that damage these critical areas. Active community involvement in conservation efforts and clear legal protections with penalties for violations are necessary to preserve the resilience and sustainability of traditional water harvesting systems. Integrating traditional knowledge with modern practices can further support these measures, ensuring the long-term ecological and hydrological integrity of tank irrigation systems. In general, tank revival and restoration can pave the way to enhance the scalability of national programs, even in the remotest villages. The sustenance of rehabilitation initiatives could be substantiated by capacity building and strengthening the local institutions led by farmers and local communities. Instead of substituting tanks with canal networks or well irrigation, a tank cascade network should be endorsed as a complementary source to the canal and well irrigation. Attention should be given to phenomenal aspects degrading a tank’s performance, such as siltation of the tank, including feeder channels, eradication of invasive weed infestation, and unsustainable use of groundwater resources. Mandatory provisioning of dead storage in cascading tanks can enable life irrigation of the standing crops even during non-monsoon seasons. Since the tank cascade system has been sustained for over two millennia, espousing these traditional design principles in modern-day rehabilitation schemes could allow for achieving the SDGs.
A coordinated effort involving key organizations is proposed to effectively address tank system management and rehabilitation, integrating service providers, facilitators, and users. Service providers, such as the Ministry of Jal Shakti (in India) and State Water Resources Departments, can develop and implement national and state-level policies, respectively, with the Central Water Commission providing technical guidance. Service facilitators, including local NGOs, can ensure community involvement and act as intermediaries between the government and local communities. Service users, such as TUGs, WUAs, and local communities, are crucial for the success of these programs, necessitating their active participation. Additionally, international development agencies, like the World Bank and Asian Development Bank, can offer financial and technical support. At the same time, academic and research institutions, such as the Indian Institutes of Technology (IITs), can conduct research and provide evidence-based recommendations.
5 Scope of traditional water harvesting: global perspectives
5.1 Social well-being and sustainable development
The TCS, or any analogous traditional water harvesting technique, represents a time-honored approach to water collection employed across diverse regions for centuries. In the case of TCS, it involves a series of interconnected tanks or ponds arranged in a descending fashion to collect and retain precipitation during the monsoon season. These tanks function as natural reservoirs, facilitating effective water resource management. The cascade systems provide water for fisheries and animals, prevent soil erosion, mitigate floods, control water quality, store water for irrigation, reduce vulnerability to drought, and promote soil health (Vidanage et al., 2022). These benefits align closely with the objectives of the SDGs. Traditional water harvesting systems are pivotal in enhancing societal well-being and contribute significantly to socio-economic progress through various mechanisms. The following discussion provides various dimensions on harnessing the benefits of using such traditional water harvesting techniques in the context of SDGs (further depicted in Figure 6).
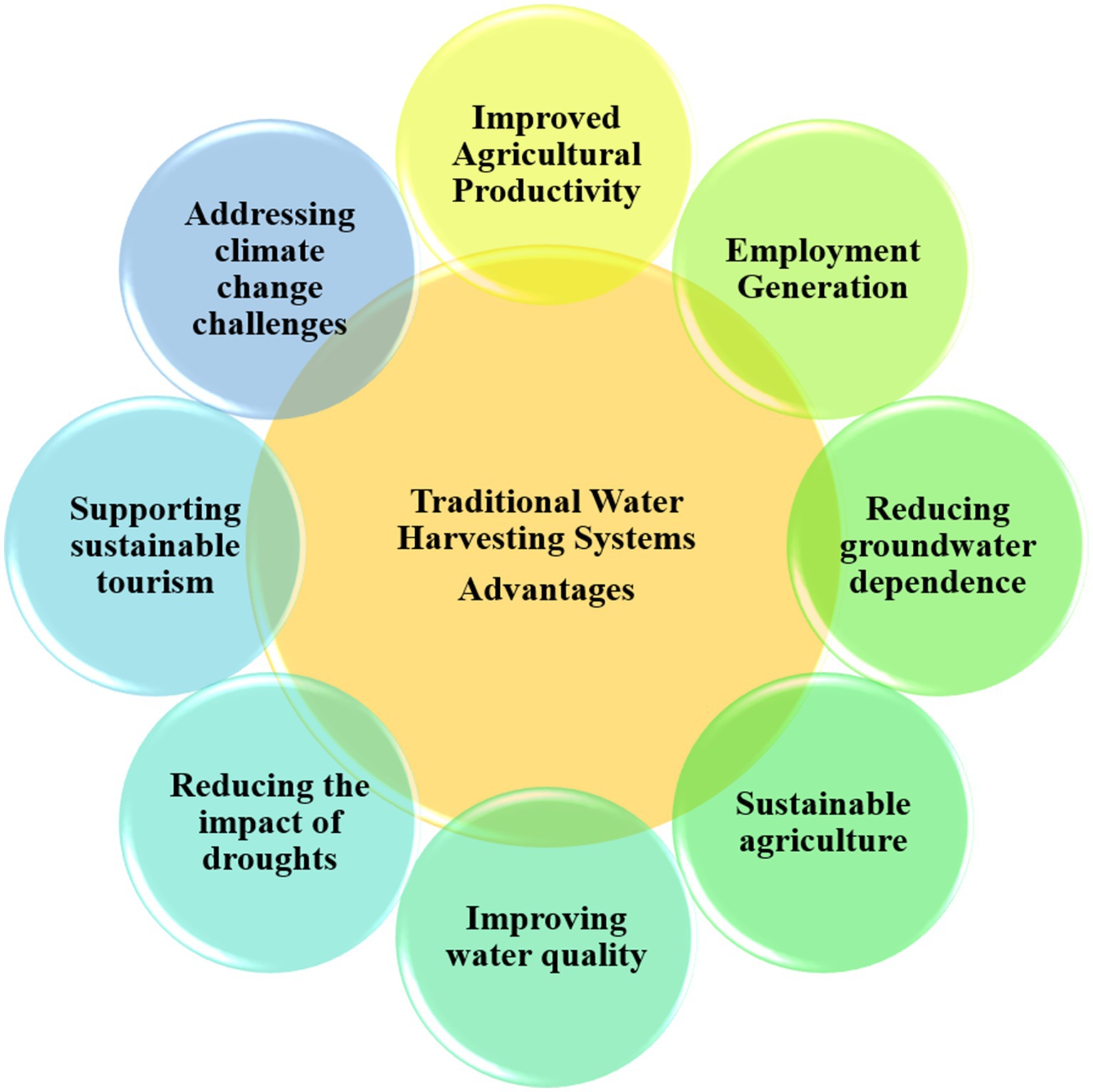
Figure 6. Benefits of traditional water harvesting system in the context of natural hazard mitigation, social well-being, and sustainable development.
5.1.1 (SDG 1) No poverty
The provision of building and maintenance services by traditional water harvesting technologies has created employment possibilities at the local level, hence contributing to the augmentation of incomes and alleviating poverty. Water storage systems facilitate water provision for irrigation, enhancing agricultural productivity and mitigating poverty within rural areas through the augmentation of food production and income-generating prospects (Gathala et al., 2020).
5.1.2 (SDG 2) Zero hunger
By capturing and storing water, traditional water harvesting technologies such as TCS allowed irrigation during dry periods, facilitating agricultural activities and thereby contributing to the promotion of food security and the mitigation of hunger in arid and semi-arid areas (Kumar et al., 2021).
5.1.3 (SDG 3) Good health and well-being
Providing clean and dependable water sources through traditional water harvesting technologies enhances sanitation and hygiene practices, reducing waterborne illnesses and improving health outcomes (Liu et al., 2021).
5.1.4 (SDG 4) Quality education
Enhanced water security and increased agricultural incomes enable households to allocate greater financial resources toward their children’s education, enhancing academic performance by mitigating student absences resulting from water-related challenges (Brewis et al., 2020).
5.1.5 (SDG 5) Gender equality
Implementing traditional water harvesting technologies has increased opportunities for education and income generation among women and girls, who typically bear the responsibility of water collection. This has contributed to the advancement of gender equality. In addition, women often took leadership roles in communally managing TCS and supporting gender equity (Tantoh et al., 2021).
5.1.6 (SDG 6) Clean water and sanitation
Implementing traditional water harvesting technologies such as TCS facilitates the storage of rainfall, thereby augmenting water availability for various purposes such as home consumption, livestock rearing, and agricultural activities. This approach effectively tackles the issue of water shortage and promotes accessible and safe water resources (Evaristo et al., 2023).
5.1.7 (SDG 7) Affordable and clean energy
TCS provides water with significant, yet underutilized, potential. Adequate attention to TCS can greatly enhance the implementation of micro-hydro initiatives, thereby promoting the adoption of environmentally sustainable and cost-effective energy alternatives in rural areas. This focus can transform rural energy solutions, supporting sustainable development and environmental conservation (Agwu et al., 2023).
5.1.8 (SDG 8) Decent work and economic growth
TCS construction and maintenance created local jobs. The use of TCS has been found to augment agricultural productivity significantly, generating more employment prospects within the farming sector and facilitating economic advancement in rural communities (Melles and Perera, 2020).
5.1.9 (SDG 9) Industry, innovation, and infrastructure
TCS assists in advancing rural infrastructure, encompassing essential components such as irrigation systems, road networks, and storage facilities. These provisions serve to enhance economic activities and foster the emergence of innovative practices (Kekulandala, 2021).
5.1.10 (SDG 10) Reduced inequality
Enhanced access to water resources facilitated by TCS yields advantageous outcomes for vulnerable populations, thereby mitigating inequalities and fostering a more comprehensive approach to development (Tantoh et al., 2021).
5.1.11 (SDG 11) Sustainable cities and communities
The implementation of TCS can significantly contribute to urban water management. By effectively lowering the strain on urban water supplies, TCS may play a pivotal role in supporting the pursuit of sustainable urbanization (Ratnayake et al., 2021).
5.1.12 (SDG 12) Responsible consumption and production
TCS advocates for properly utilizing water resources to mitigate water loss and endorse sustainable agriculture methodologies (Sirimanna et al., 2022).
5.1.13 (SDG 13) Climate action
As natural infrastructure, TCS enhances landscape resilience to climate change impacts. The storage of rainwater by TCS facilitates community adaptation to climate change by maintaining a consistent water supply during periods of drought (Ranasinghe et al., 2023). Additionally, tank organic build-up can sequester carbon, contributing to climate change mitigation by reducing atmospheric carbon dioxide levels (Kulasinghe and Dharmakeerthi, 2022).
5.1.14 (SDG 15) Life on land
TCS supports biodiversity and enhances terrestrial ecosystems within their catchment areas. By replenishing groundwater supplies and facilitating vegetation growth, TCS significantly contributes to land restoration efforts and biodiversity protection (Ratnayake et al., 2021).
5.1.15 SDG 16: Peace, justice, and strong institutions
The utilization of TCS can facilitate collaboration and encourage active participation from communities in the management of water resources. This approach can effectively contribute to promoting peace and establishing sustainable water governance systems (Kekulandala et al., 2023).
5.1.16 (SDG 17) Partnerships for the goals
The preservation and promotion of TCS necessitate the collaborative efforts of governments, non-governmental organizations (NGOs), and local people, underscoring the significance of partnerships in attaining sustainable development (Tantoh et al., 2021; Kulasinghe and Dharmakeerthi, 2022; Sirimanna et al., 2022; Kekulandala et al., 2023).
5.1.17 Sustainable agriculture and rural development as a source of employment
Implementing traditional water harvesting technologies in sustainable agriculture and rural development offers significant employment opportunities and rural advancement (Melles and Perera, 2020; Kekulandala, 2021). These activities include constructing and maintaining rainwater harvesting systems, conserving soil and water, and developing small-scale irrigation systems. Such efforts enhance agricultural productivity, generate higher incomes, and foster employment and entrepreneurship (Karalliyadda et al., 2023). Revitalizing traditional water systems also aids cultural preservation and environmental sustainability (Perera et al., 2021; Ratnayake et al., 2021). Systems like TCS are vital for sustainable agriculture, providing employment, reducing groundwater reliance, and improving water quality. Integrating modern technologies like geographical information systems, remote sensing, artificial intelligence, and machine learning (discussed in Section 5.3) can optimize traditional systems, while community-based monitoring can ensure sustainability. These systems increase agricultural productivity, create employment, reduce groundwater use, and promote sustainable agriculture. They also improve water quality, mitigate drought impacts, support sustainable tourism, and address climate change by promoting sustainable water management, as was reflected in SDGs. The diverse benefits of traditional water harvesting methods are illustrated in Figure 7.
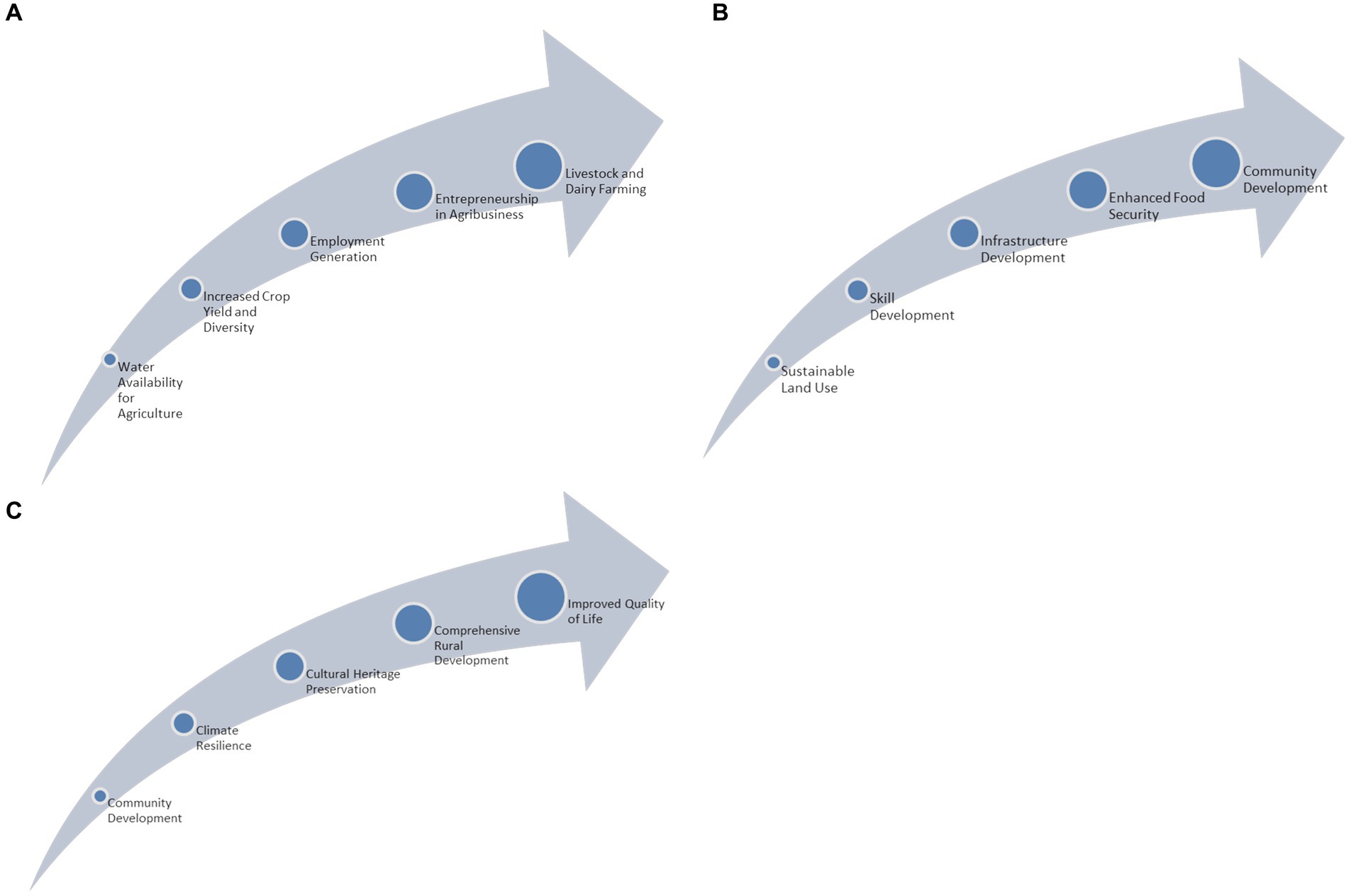
Figure 7. A growth chart indicating the scope of development due to better utilization of available water resources (A), suitable and sustainable adoption of land use land cover pattern (B), and practice of decentralized water governance amidst the applications of traditional water harvesting systems (C).
5.2 Adaptation amidst climate change
Traditional methods of harnessing surface water can mitigate water scarcity, although they may not provide a complete solution in all cases. A systematic study of these methods is thus needed to raise awareness of these alternative water sources among policy-makers. Efforts should also be made to educate rural people about these techniques, using locally available and low-cost construction materials. An integrated approach to the upkeep of traditional water harvesting structures is essential for sustainable livelihoods and ecological balance in drought-affected regions globally. TCS and traditional water harvesting systems can help to bring climate change resilience in the following ways (depicted in Figure 8):
• Flood mitigation: By collecting and storing more precipitation, TCS and conventional water harvesting devices can assist in minimizing floods. Adopting this can decrease the chance of floods and associated infrastructure and property damage without constructing the additional dedicated structures.
• Drought resilience: TCS and conventional water collecting systems can contribute to drought resilience by offering a steady water supply during dry spells. This can lessen the impact of drought stress on animals and crops and assist modern structures such as dams.
• Heatwave adaptation: Providing access to water for cooling and irrigation is one way to respond to a heat wave. This can enhance the quality of life during heat waves and lower the risk of heat-related diseases.
• Reduced dependence on external water sources: TCS and conventional water harvesting devices can aid in lowering a community’s reliance on rivers and groundwater. As a result, communities may become more resistant to the effects of climate change, such as drought and groundwater depletion.
• Enhanced ecosystem services: TCS and conventional water collecting systems can support enhancing ecosystem functions, including soil conservation and groundwater recharging. This might increase a community’s ability to withstand the effects of climate change, such as drought and soil erosion.
Traditional water harvesting systems offer multifaceted benefits with significant implications for government, policymakers, administration, and society. For governments and policymakers, these systems provide opportunities for resource optimization by streamlining water management and bolstering climate resilience against floods, droughts, and heat waves. Administration stands to gain from cost savings in infrastructure development and potential reductions in healthcare expenditures through the health benefits of heatwave adaptation. In society, adopting these systems directly enhances the quality of life by providing water for cooling and irrigation, making communities more resilient to extreme weather events, and contributing to environmental sustainability by reducing dependence on external water sources. Implementing traditional water harvesting systems yields economic, social, and environmental advantages, aligning with sustainable development goals and fostering community well-being.
5.3 Role of artificial intelligence and machine learning approaches: a proposal
Given the limited applications of Artificial Intelligence (AI) and Machine Learning (ML) in enhancing TCS, there exists a substantial opportunity to re-evaluate traditional water harvesting approaches through the lens of advanced AI-ML technologies, which are revolutionizing scientific applications. Hence, this section proposes revisiting TCS through the lens of AI-ML to advance water management and disaster resilience. Addressing the growing water demands in agriculture, balancing industrial, urban, and rural water needs, and tackling water scarcity necessitate innovative water governance strategies (De Fraiture and Wichelns, 2010; Di Vaio et al., 2021). Traditional water harvesting methods such as TCS, a time-tested technology, can significantly benefit from AI-ML integration. AI-ML techniques can enhance TCS efficiency, particularly in forecasting extreme weather events such as floods and droughts (Arora et al., 2021; Elbeltagi et al., 2024). AI-ML models trained on historical data can predict the frequency and severity of these events, enabling proactive management of TCS. For instance, if an AI-ML model predicts a flood, tank gates can be opened to release excess water, mitigating flood risks. Conversely, if a drought is forecasted, tank gates can be closed to conserve water. Additionally, AI-ML models can optimize irrigation schedules to maximize crop yields with minimal water use and develop water distribution plans that ensure equitable access during scarcity (Elbeltagi et al., 2022).
The application of AI in intelligent water management systems holds significant potential for improving water supply and service delivery efficiency (Jenny et al., 2020). The integration of AI, Deep Learning (DL), and ML with Internet-of-Things (IoT) technologies is anticipated to address complexities in water management systems (Nasser et al., 2020; Alam et al., 2022). AI-ML models have demonstrated effectiveness in wastewater treatment, water pollution control, smart agriculture, optimizing water use, and monitoring water quality and levels (Jain et al., 2021; Akhund et al., 2022; Lowe et al., 2022). The recent socioeconomic growth and ecological sustainability depend on effective water resources management to alleviate poverty and promote equity. Moreover, Adaptive Intelligent Dynamic Water Resource Planning (AIDWRP) exemplifies AI’s potential in sustainable water management. Combining numerical AI methods with human intelligence enhances data-driven decision-making and water efficiency (Xiang et al., 2021; Krishnan et al., 2022). AIDWRP utilizes the Markov Decision Process (MDP) to optimize environmental planning, balancing water supply and demand while supporting local economic efficiency. Integrating AI-ML techniques with TCS could significantly enhance their functionality by optimizing water distribution and improving predictive capabilities for extreme weather events. This approach can transform traditional water harvesting practices, fostering more resilient and efficient management of water resources in rural areas.
Furthermore, the collaboration of AI with Information and Communication Technologies (ICT) can yield effective water management strategies, enhancing distribution and reducing costs (Jenny et al., 2020). AI’s application to water management can help formulate strategies to minimize water leakage and address water scarcity, considering population density. Integrating AI-ML with traditional water harvesting systems like TCS offers a strategic advantage for disaster preparedness and response, optimizing water management and infrastructure resilience (Drogkoula et al., 2023). This synergistic approach optimizes water management and fine-tunes infrastructure and disaster recovery efforts. By harnessing the predictive capabilities of AI and the adaptive learning algorithms of ML, these systems empower communities to manage and mitigate the impact of natural disasters proactively. The infusion of such technologies extends beyond immediate disaster response, fostering long-term resilience by providing invaluable insights for future planning. This advanced integration stands as a testament to the potential of technology not merely as a tool for emergency response but as a cornerstone for enhancing societal well-being and environmental sustainability.
5.4 Global lessons
The restoration and rehabilitation of traditional water harvesting systems, such as the TCS, offer significant visions for global water management, environmental sustainability, and climate resilience. Key lessons derived from the study include:
• The successful revival of traditional water harvesting systems, exemplified by TCS, demonstrates that combining ancient knowledge with modern technology can create sustainable solutions for water management. These systems provide reliable water sources for agriculture and drinking, enhance groundwater recharge, reduce soil erosion, and improve water quality. Regions experiencing water scarcity can adopt similar practices to ensure a more sustainable and resilient water supply.
• TCS and similar systems mitigate the impacts of climate change by capturing and storing rainwater, reducing the vulnerability of communities to droughts and floods. These systems offer a steady water supply during dry spells and manage excess water during heavy rains, building resilience to climate variability. This approach can be replicated globally to enhance adaptive capacity to climate change.
• The success of TCS rehabilitation projects underscores the critical role of community involvement in water management. Engaging local communities in planning, implementation, and maintenance ensures the sustainability and effectiveness of these systems. Empowering communities with knowledge and resources fosters improved water governance and resilience to environmental changes.
• Integrating traditional water harvesting methods with modern technologies, such as GIS, remote sensing, and artificial intelligence, optimizes the efficiency and effectiveness of these systems. This hybrid approach provides precise data for better decision-making and enhances overall water resource management, demonstrating the value of leveraging both traditional knowledge and modern innovation.
• The rehabilitation of TCS has resulted in significant economic and social benefits, including increased agricultural productivity, employment generation, and improved livelihoods. Revitalizing traditional water systems can lead to higher incomes, foster entrepreneurship, and reduce groundwater dependency, promoting long-term economic sustainability. These benefits highlight the potential for similar projects to contribute to rural socio-economic development worldwide.
• Supportive policies and institutional frameworks have been instrumental in the successful implementation of TCS rehabilitation projects. Governments and organizations must prioritize the conservation and rehabilitation of traditional water systems through policies that promote sustainable practices. Institutional support, funding, and capacity-building initiatives are essential for the successful scaling and replication of these projects in various regions.
• TCS contributes to broader environmental conservation efforts by reducing water runoff and promoting groundwater recharge, helping to maintain ecological balance, preserve biodiversity, and enhance ecosystem resilience. Adopting similar practices can support global efforts to protect natural resources and promote environmental sustainability.
• Educating communities about the importance of traditional water harvesting systems and their role in sustainable water management is crucial. Awareness programs and educational initiatives can help communities understand the benefits of these systems and encourage active participation in conservation efforts. Continuous education and outreach are essential to foster a culture of sustainable water management.
In summary, the rehabilitation of traditional water harvesting systems provides lessons that can be applied globally. By integrating traditional knowledge with modern technology, involving communities, and providing policy and institutional support, regions around the world may enhance water security, build climate resilience, and achieve sustainable development.
6 Conclusion
Traditional water harvesting systems in South Asia have historically been integral to rural economies, playing a crucial role in sustaining agriculture and managing water resources. However, these systems have experienced significant decline due to shifts in policy, political interventions, and socio-economic changes, particularly during colonial rule. The Tank Cascade System (TCS), a prominent example, is critical for mitigating natural hazards such as floods and droughts. Despite their historical significance, modern neglect and insufficient integration into contemporary water management practices often compromise these systems’ efficacy, undermining their potential to contribute to sustainable development and climate resilience.
The alignment of TCS with the 17 Sustainable Development Goals (SDGs) underscores their multifaceted role in advancing social well-being and environmental sustainability. These systems contribute to improved water access, increased agricultural productivity, community empowerment, and gender equality while exhibiting notable resilience to extreme climatic events. Their integration into SDG frameworks highlights their potential to enhance adaptive capacity and socio-economic development. Recent research advocates incorporating traditional water harvesting technologies into disaster risk reduction frameworks and eco-tourism initiatives. The resurgence of interest in tank rehabilitation and modernization, bolstered by government support in India, reflects their significance in preserving biodiversity, maintaining hydro-ecological stability, and enhancing climate resilience.
To ensure the continued relevance and effectiveness of traditional practices such as TCS, adopting a holistic approach to their management is essential. Modern strategies should prioritize stakeholder engagement and critically evaluate the dependency on large-scale dam projects, considering traditional systems’ ecological and socio-economic advantages. Effective rehabilitation and sustainability of tank irrigation require comprehensive policies that recognize the intricate significance of these systems and facilitate their adaptation to current and future challenges. By integrating traditional knowledge with modern technologies and practices, we can create resilient water management systems that support sustainable development and mitigate the impacts of climate change.
Data availability statement
The original contributions presented in the study are included in the article, further inquiries can be directed to the corresponding authors.
Author contributions
SJ: Formal analysis, Investigation, Validation, Visualization, Writing – original draft, Writing – review & editing. AmS: Conceptualization, Data curation, Formal analysis, Investigation, Validation, Visualization, Writing – original draft, Writing – review & editing. DV: Investigation, Visualization, Writing – review & editing. JR: Investigation, Visualization, Writing – review & editing. NR: Investigation, Visualization, Writing – review & editing. AlS: Formal analysis, Supervision, Validation, Writing – review & editing. AE: Formal analysis, Supervision, Validation, Writing – review & editing.
Funding
The author(s) declare that no financial support was received for the research, authorship, and/or publication of this article.
Acknowledgments
The authors thank the Directorate of Economics and Statistics under the Ministry of Agriculture and Farmers Welfare and Central Water Commission under the Ministry of Jal Shakti, Government of India, for providing secondary data to conduct this research. Primary data from the Asian Development Bank’s report entitled ‘Rehabilitation and Management of Tanks in India’ are gratefully acknowledged. The authors further acknowledge the work of V. Ratna Reddy, M. Srinivasa Reddy, and K. Palanisami entitled ‘Tank rehabilitation in India: Review of experiences and strategies’ which motivated the authors to conduct this work. Besides, during the preparation of this work, the authors used the ‘OpenAI - Cloud Computing Platform Version 4’ in order to improve language. After using this service, the authors reviewed and edited the content as needed and took full responsibility for the content of the publication. Finally, the authors would like to acknowledge the substantial efforts of various Indian institutions (besides those which are enlisted inside this article) in restoring, rehabilitating, and reviving traditional water harvesting systems, such as (not limited to) the Centre for Water Resources Development and Management (CWRDM), Tamil Nadu Water Supply and Drainage Board (TWAD), National Institute of Hydrology (NIH), Rural Technology Action Groups (RuTAG) of various Indian Institute of Technology (IIT), Centre for Technology Alternatives for Rural Areas (CTARA), Mahatma Gandhi National Rural Employment Guarantee Scheme (NREGS) of Ministry of Rural Development (MoRD), WaterAid India, Development of Humane Action (DHAN) Foundation, Professional Assistance for Development Action (PRADAN), Arghyam, Society for Integrated Rural Development (SIRDO), and Gram Vikas.
Conflict of interest
The authors declare that the research was conducted in the absence of any commercial or financial relationships that could be construed as a potential conflict of interest.
Publisher’s note
All claims expressed in this article are solely those of the authors and do not necessarily represent those of their affiliated organizations, or those of the publisher, the editors and the reviewers. Any product that may be evaluated in this article, or claim that may be made by its manufacturer, is not guaranteed or endorsed by the publisher.
Footnotes
1. ^System tanks refer to tanks that are part of a larger, interconnected network of water bodies designed for collective water management and distribution, often found in traditional tank cascade systems. These systems enable the efficient use and reuse of water resources across multiple tanks, ensuring a sustainable supply for irrigation, drinking, and other purposes. On the other hand, non-system tanks are standalone tanks that do not have such interconnections and function independently. These tanks often rely on local rainfall and runoff and do not benefit from the integrated water management provided by system tanks.
References
Agwu, A. E., Raheem, D., Muteba, M. C., and Foster, S. N. (2023). Micro-hydropower systems for smallholder farmers in rural communities of Taraba state, Nigeria: socioeconomic assessment of needs and perceptions (part I). Energy Nexus 10:100191. doi: 10.1016/j.nexus.2023.100191
Akhund, T. N., Newaz, N. T., Zaman, Z., Sultana, A., Barros, A., and Whaiduzzaman, M. (2022). “IoT-based low-cost automated irrigation system for smart farming” in Intelligent Sustainable Systems. Lecture Notes in Networks and Systems. eds. A. K. Nagar, D. S. Jat, G. Marín-Raventós, and D. K. Mishra, vol. 333 (Singapore: Springer).
Alam, G., Ihsanullah, I., Naushad, M., and Sillanpää, M. (2022). Applications of artificial intelligence in water treatment for optimization and automation of adsorption processes: recent advances and prospects. Chem. Eng. J. 427:130011. doi: 10.1016/j.cej.2021.130011
Ammar, A., Riksen, M., Ouessar, M., and Ritsema, C. (2016). Identification of suitable sites for rainwater harvesting structures in arid and semi-arid regions: a review. Int. Soil Water Conserv. Res. 4, 108–120. doi: 10.1016/j.iswcr.2016.03.001
Arora, A., Arabameri, A., Pandey, M., Siddiqui, M. A., Shukla, U. K., Bui, D. T., et al. (2021). Optimization of state-of-the-art fuzzy-metaheuristic ANFIS-based machine learning models for flood susceptibility prediction mapping in the middle ganga plain, India. Sci. Total Environ. 750:141565. doi: 10.1016/j.scitotenv.2020.141565
Aubriot, O., and Prabhakar, P. I. (2011). Water institutions and the “revival” of tanks in South India: what is at stake locally? Water Altern. 4, 325–346.
Bardhan, P. (2000). Irrigation and cooperation: an empirical analysis of 48 irrigation communities in South India. Econ. Dev. Cult. Chang. 48, 847–865. doi: 10.1086/452480
Bharucha, Z. P. (2019). This is what nature has become: tracing climate and water narratives in India’s rainfed drylands. Geoforum 101, 285–293. doi: 10.1016/j.geoforum.2018.09.032
Brewis, A., Workman, C., Wutich, A., Jepson, W., and Young, S.Household Water Insecurity Experiences - Research Coordination Network (HWISE-RCN) (2020). Household water insecurity is strongly associated with food insecurity: evidence from 27 sites in low-and middle-income countries. Am. J. Hum. Biol. 32:e23309. doi: 10.1002/ajhb.23309
Brewis, A., Workman, C., Wutich, A., Jepson, W., and Young, S.Household Water Insecurity Experiences – Research Coordination Network (HWISE‐RCN) (2021). Role of traditional ethnobotanical knowledge and indigenous communities in achieving sustainable development goals. Sustain. For. 13:3062.
Castro-Arce, K., and Vanclay, F. (2020). Transformative social innovation for sustainable rural development: an analytical framework to assist community-based initiatives. J. Rural. Stud. 74, 45–54. doi: 10.1016/j.jrurstud.2019.11.010
Chambers, R. (1994). The origins and practice of participatory rural appraisal. World Dev. 22, 953–969. doi: 10.1016/0305-750X(94)90141-4
Chaube, U. C., Pandey, A., and Singh, V. P. (2023). “Farmers’ participation” in Canal irrigation Systems in India. Water science and technology library. ed. U. C. Chaube, vol. 126 (Cham: Springer).
Chowdhury, K., and Behera, B. (2018). Is declining groundwater levels linked with the discontinuity of traditional water harvesting systems (tank irrigation)? Empirical evidence from West Bengal, India. Groundw. Sustain. Dev. 7, 185–194. doi: 10.1016/j.gsd.2018.05.007
De Fraiture, C., and Wichelns, D. (2010). Satisfying future water demands for agriculture. Agric. Water Manag. 97, 502–511. doi: 10.1016/j.agwat.2009.08.008
Di Vaio, A., Trujillo, L., D'Amore, G., and Palladino, R. (2021). Water governance models for meeting sustainable development goals: a structured literature review. Util. Policy 72:101255. doi: 10.1016/j.jup.2021.101255
Drogkoula, M., Kokkinos, K., and Samaras, N. (2023). A comprehensive survey of machine learning methodologies with emphasis in water resources management. Appl. Sci. 13:12147. doi: 10.3390/app132212147
Elbeltagi, A., Srivastava, A., Ehsan, M., Sharma, G., Yu, J., Khadke, L., et al. (2024). Advanced stacked integration method for forecasting long-term drought severity: CNN with machine learning models. J. Hydrol. 53:101759. doi: 10.1016/j.ejrh.2024.101759
Elbeltagi, A., Srivastava, A., Kushwaha, N. L., Juhász, C., Tamás, J., and Nagy, A. (2022). Meteorological data fusion approach for modeling crop water productivity based on ensemble machine learning. Water 15:30. doi: 10.3390/w15010030
Evaristo, J., Jameel, Y., Tortajada, C., Wang, R. Y., Horne, J., Neukrug, H., et al. (2023). Water woes: the institutional challenges in achieving SDG 6. Sustain. Earth Rev. 6:13. doi: 10.1186/s42055-023-00067-2
Gathala, M. K., Laing, A. M., Tiwari, T. P., Timsina, J., Islam, M. S., Chowdhury, A. K., et al. (2020). Enabling smallholder farmers to sustainably improve their food, energy and water nexus while achieving environmental and economic benefits. Renew. Sust. Energ. Rev. 120:109645. doi: 10.1016/j.rser.2019.109645
Geekiyanage, N., and Pushpakumara, D. K. N. G. (2013). Ecology of ancient tank cascade systems in island Sri Lanka. J. Mar. Island Cult. 2, 93–101. doi: 10.1016/j.imic.2013.11.001
Glendenning, C. J., Van Ogtrop, F. F., Mishra, A. K., and Vervoort, R. W. (2012). Balancing watershed and local scale impacts of rain water harvesting in India—a review. Agric. Water Manag. 107, 1–13. doi: 10.1016/j.agwat.2012.01.011
Gunnell, Y., Anupama, K., and Sultan, B. (2007). Response of the south Indian runoff-harvesting civilization to northeast monsoon rainfall variability during the last 2000 years: instrumental records and indirect evidence. The Holocene 17, 207–215. doi: 10.1177/0959683607075835
Hanjra, M. A., and Qureshi, M. E. (2010). Global water crisis and future food security in an era of climate change. Food Policy 35, 365–377. doi: 10.1016/j.foodpol.2010.05.006
Jain, H., Buch, M., and Babu, P. (2021). “Water Management System Using Machine Learning” in Data engineering and intelligent computing. Advances in intelligent systems and computing. eds. V. Bhateja, S. C. Satapathy, C. M. Travieso-González, and V. N. M. Aradhya, vol. 1407 (Singapore: Springer).
Jayanthi, S. L. S. V., and Keesara, V. R. (2021). Climate change impact on water resources of medium irrigation tank. ISH J. Hydraul. Eng. 27, 322–333. doi: 10.1080/09715010.2019.1649605
Jayasena, H. A. H., Chandrajith, R., and Gangadhara, K. R. (2011). Water management in ancient tank Cascade systems (TCS) in Sri Lanka: evidence for systematic tank dis-tribution. J. Geol. Soc. Sri Lanka 14, 29–34.
Jenny, H., Alonso, E. G., Wang, Y., and Minguez, R. (2020). Using artificial intelligence for smart water management systems.
Kakatiya, M. (2015). Manual on construction procedure. Irrigation and CAD Department, Government of Telangana State. Available at: http://missionkakatiya.cgg.gov.in/jsp/manual.pdf (Accessed January 15, 2021).
Karalliyadda, S. M. C. B., Kazunari, T., and Fujimura, M. (2023). Managing rain-fed uplands of cascaded tank village systems: what stakeholders really suggest? Agric. Syst. 208:103643. doi: 10.1016/j.agsy.2023.103643
Kekulandala, B. (2021). Adaptive co-management of small tank cascade systems in Sri Lanka in a changing climate (order no. 30610653). Available from publicly available content Database, No. 2833612314. Available at: https://www.proquest.com/dissertations-theses/adaptive-co-management-small-tank-cascade-systems/docview/2833612314/se-2 (Accessed August, 2024).
Kekulandala, B., Cunningham, R., and Jacobs, B. (2023). Exploring social networks in a small tank cascade system in northcentral Sri Lanka: first steps to establishing adaptive governance. Environ. Dev. 46:100847. doi: 10.1016/j.envdev.2023.100847
Khagram, S. (2004). Dams and development: Transnational struggles for water and power. Ithaca, NY: Cornell University Press.
Krishnan, S. R., Nallakaruppan, M. K., Chengoden, R., Koppu, S., Iyapparaja, M., Sadhasivam, J., et al. (2022). Smart water resource management using artificial intelligence—a review. Sustain. For. 14:13384. doi: 10.3390/su142013384
Kulasinghe, T. N., and Dharmakeerthi, R. S. (2022). Effects of land use type and tank components on soil properties and sustainability of tank cascade system in the dry zone of north Central Sri Lanka. Agric. Syst. 201:103474. doi: 10.1016/j.agsy.2022.103474
Kumar, M. D., and Rao, N. (2017). “The hydro-institutional challenge of managing tanks: a study of tanks in rural Andhra Pradesh” in Ecosystem functions and management. ed. H. Sandhu (Cham: Springer International Publishing), 155–191.
Kumar, A., Kumar, S., Ramchiary, K. N., and Singh, P. (2021). Role of traditional ethnobotanical knowledge and indigenous communities in achieving sustainable development goals. Sustainability. 13:3062. doi: 10.3390/su13063062
Lal, B. B. (2002). The Saraswati flows on: The continuity of Indian culture. New Delhi: Aryan Books International.
Laskar, N. (2022). Reviving traditional rain-water harvesting system and artificial ground-water recharge. Sādhanā 47, 1–15. doi: 10.1007/s12046-022-02035-6
Liladhar Rane, N., Purushottam Choudhary, S., Saha, A., Srivastava, A., and Pande, C. B. (2024). Delineation of environmentally sustainable urban settlement using GIS-based MIF and AHP techniques. Geocarto International. 39. doi: 10.1080/10106049.2024.2335249
Liu, H. Y., Jay, M., and Chen, X. (2021). The role of nature-based solutions for improving environmental quality, health and well-being. Sustain. For. 13:10950. doi: 10.3390/su131910950
Lowe, M., Qin, R., and Mao, X. (2022). A review on machine learning, artificial intelligence, and smart technology in water treatment and monitoring. Water 14:1384. doi: 10.3390/w14091384
Mane, S. P., and Shinde, A. S. (2014). A study changing pattern of rain water harvesting management an ancient to modern age in India-geographical analysis. Rev. Res. 3, 1–6.
Massuel, S., Perrin, J., Mascre, C., Mohamed, W., Boisson, A., and Ahmed, S. (2014). Managed aquifer recharge in South India: what to expect from small percolation tanks in hard rock? J. Hydrol. 512, 157–167. doi: 10.1016/j.jhydrol.2014.02.062
Mbilinyi, B. P., Tumbo, S. D., Mahoo, H. F., Senkondo, E. M., and Hatibu, N. (2005). Indig-enous knowledge as decision support tool in rainwater harvesting. Phys. Chem. Earth Parts A B C 30, 792–798. doi: 10.1016/j.pce.2005.08.022
Melles, G., and Perera, E. D. (2020). Resilience thinking and strategies to reclaim sustainable rural livelihoods: Cascade Tank-Village system (CTVS) in Sri Lanka. Challenges 11:27. doi: 10.3390/challe11020027
Mishra, V. (2020). Long-term (1870–2018) drought reconstruction in context of surface water security in India. J. Hydrol. 580:124228. doi: 10.1016/j.jhydrol.2019.124228
Mosse, D. (1997). The symbolic making of a common property resource: history, ecology and locality in a tank-irrigated landscape in South India. Dev. Chang. 28, 467–504. doi: 10.1111/1467-7660.00051
Narayanamoorthy, A. (2007). Tank irrigation in India: a time series analysis. Water Policy 9, 193–216. doi: 10.2166/wp.2006.063
Narayanamoorthy, A. (2010). India’s groundwater irrigation boom: can it be sustained? Water Policy 12, 543–563. doi: 10.2166/wp.2010.042
Narayanamoorthy, A., and Jothi, P. (2019). Water saving and productivity benefits of SRI: a study of tank, canal and groundwater irrigated settings in South India. Water Policy 21, 162–177. doi: 10.2166/wp.2018.199
Nasser, A. A., Rashad, M. Z., and Hussein, S. E. (2020). A two-layer water demand prediction system in urban areas based on micro-services and LSTM neural networks. IEEE Access 8, 147647–147661. doi: 10.1109/ACCESS.2020.3015655
Palanisami, K. (2006). Sustainable Management of Tank Irrigation Systems in India. J. Dev. Sustain. Agric. 1, 34–40. doi: 10.11178/jdsa.1.34
Palanisami, K., and Easter, K. W. (2000). Tank irrigation in the 21st century: What next? New Delhi: Discovery Publishing House.
Palanisami, K., Meinzen-Dick, R., and Giordano, M. (2010). Climate change and water supplies: options for sustaining tank irrigation potential in India. Econ. Polit. Wkly. 45, 183–190.
Palanisami, K., and Nagothu, U. S. (2024). “Sustaining groundwater irrigation” in India's water future in a changing climate. Advances in geographical and environmental sciences. ed. K. Palanisami (Singapore: Springer).
Pande, C. B., Srivastava, A., Moharir, K. N., Radwan, N., Mohd Sidek, L., Alshehri, F., et al. (2024). Characterizing land use/land cover change dynamics by an enhanced random forest machine learning model: a Google Earth Engine implementation. Environmental Sciences Europe. 36:84. doi: 10.1186/s12302-024-00901-0
Pant, N., and Verma, R. K. (2010). Tanks in eastern India: a study in exploration. IWMI-TATA water policy research program (Hyderabad) and Centre for Development Studies (Lucknow).
Patel, P. M., Saha, D., and Shah, T. (2020). Sustainability of groundwater through community-driven distributed recharge: an analysis of arguments for water scarce regions of semi-arid India. J. Hydrol. 29:100680. doi: 10.1016/j.ejrh.2020.100680
Perera, K. T. N., Wijayaratna, T. M. N., Jayatillake, H. M., Manatunge, J. M. A., and Priyadarshana, T. (2021). Framework for the sustainable development of village tanks in cascades as an adaptation to climate change and for improved water security, Sri Lanka. Water Policy 23, 537–555. doi: 10.2166/wp.2021.262
Raju, K. V., Gaur, A., and Wani, S. P. (2015). Poverty reduction approach in South Asia: Rejuvenating centuries old water bodies to improve rural livelihoods, a case of Karnataka state. India: International Crops Research Institute for the Semi-Arid Tropics (ICRISAT).
Ramabrahmam, K., Keesara, V. R., Srinivasan, R., Pratap, D., and Sridhar, V. (2021). Flow simulation and storage assessment in an ungauged irrigation tank Cascade system using the SWAT model. Sustain. For. 13:13158. doi: 10.3390/su132313158
Ranasinghe, R. D. A. K., Korale-Gedara, P. M., and Weerasooriya, S. A. (2023). Climate change adaptation and adaptive capacities of dairy farmers: evidence from village tank cascade systems in Sri Lanka. Agric. Syst. 206:103609. doi: 10.1016/j.agsy.2023.103609
Ratnayake, S. S., Khan, A., Reid, M., Dharmasena, P. B., Hunter, D., Kumar, L., et al. (2022). Land use-based participatory assessment of ecosystem services for ecological restoration in village tank cascade systems of Sri Lanka. Sustain. For. 14:10180. doi: 10.3390/su141610180
Ratnayake, S. S., Kumar, L., Dharmasena, P. B., Kadupitiya, H. K., Kariyawasam, C. S., and Hunter, D. (2021). Sustainability of village tank cascade systems of Sri Lanka: exploring cascade anatomy and socio-ecological nexus for ecological restoration planning. Challenges 12:24. doi: 10.3390/challe12020024
Reddy, V. R., and Behera, B. (2009a). Economic viability of rehabilitation of traditional water harvesting system: empirical evidence from South India. Q. J. Int. Agric. 48, 321–336.
Reddy, V. R., and Behera, B. (2009b). The economic and ecological impacts of tank restoration in South India. Eur. J. Dev. Res. 21, 112–136. doi: 10.1057/ejdr.2008.12
Reddy, V. R., Reddy, M. S., and Palanisami, K. (2018). Tank rehabilitation in India: review of experiences and strategies. Agric. Water Manag. 209, 32–43. doi: 10.1016/j.agwat.2018.07.013
Roy, D., and Kumari, S. (2019). Social actors in traditional tank rehabilitation: a case study of Tamil Nadu (India). Sustain. Water Resour. Manag. 5, 587–597. doi: 10.1007/s40899-018-0221-0
Saha, D., Marwaha, S., and Mukherjee, A. (2018). “Groundwater resources and sustainable management issues in India” in Clean and sustainable groundwater in India. eds. D. Saha, S. Marwaha, and A. Mukherjee (Singapore: Springer Singapore), 1–11.
Sakthivadivel, R., and Gomathinayagam, P. (2006). Rehabilitation and management of tanks in India. Asian Development Bank: Publication Stock, 122605.
Sakurai, T., and Palanisami, K. (2001). Tank irrigation management as a local common property: the case of Tamil Nadu, India. Agric. Econ. 25, 273–283. doi: 10.1111/j.1574-0862.2001.tb00207.x
Saxena, K., and Brighu, U. (2021). “Jal Swavlamban: Water Independence” in The Palgrave handbook of climate resilient societies. ed. R. C. Brears (Cham: Palgrave Macmillan).
Shah, T. (2002). Rethinking rehabilitation: socio-ecology of tanks in Rajasthan, north-West India. Water Policy 3, 521–536. doi: 10.1016/S1366-7017(02)00015-6
Shah, E. (2003). Social design: Tank irrigation technology and agrarian transformation in Karnataka. South India: Wageningen University and Research.
Shah, E. (2008). Telling otherwise: a historical anthropology of tank irrigation Technology in South India. Technol. Cult. 49, 652–674. doi: 10.1353/tech.0.0054
Shankar, P. S. V., Kulkarni, H., and Krishnan, S. (2011). India’s groundwater challenge and the way forward. Econ. Polit. Wkly. 46, 37–45.
Shannon, K., and Manawadu, S. (2007). Indigenous landscape urbanism: Sri Lanka's reservoir & tank system. J. Landsc. Archit. 2, 6–17. doi: 10.1080/18626033.2007.9723384
Siderius, C., Boonstra, H., Munaswamy, V., Ramana, C., Kabat, P., van Ierland, E., et al. (2015). Climate-smart tank irrigation: a multi-year analysis of improved conjunctive water use under high rainfall variability. Agric. Water Manag. 148, 52–62. doi: 10.1016/j.agwat.2014.09.009
Singh, U. (2008). A history of ancient and early medieval India: From the stone age to the 12th century. Delhi: Pearson Education India.
Sirimanna, S., Kahathuduwa, K. K. P. N., and Prasada, D. V. P. (2022). Are cascade reservoir systems sustainable agroecosystems? A comparative assessment of efficiency, effectiveness and resource footprint in a Sri Lankan micro-cascade. Agric. Syst. 203:103493. doi: 10.1016/j.agsy.2022.103493
Srinivasan, V. (2015). Reimagining the past—use of counterfactual trajectories in socio-hydrological modelling: the case of Chennai, India. Hydrol. Earth Syst. Sci. 19, 785–801. doi: 10.5194/hess-19-785-2015
Srinivasan, T. M. (2016). Agricultural practices as gleaned from the Tamil literature of the Sangam age. Indian J. Hist. Sci. 51, 167–189. doi: 10.16943/ijhs/2016/v51i2/48430
Sugam, R., Gupta, B., and Deka, D. (2018). Dying traditional water bodies in India struggling to survive against unplanned development. J. Water Resour. Prot. 10, 539–558. doi: 10.4236/jwarp.2018.106030
Tantoh, H. B., McKay, T. T., Donkor, F. E., and Simatele, M. D. (2021). Gender roles, implications for water, land, and food security in a changing climate: a systematic review. Front. Sustain. Food Syst. 5:707835. doi: 10.3389/fsufs.2021.707835
Thakkar, H. (1998). The World Bank’s-the Orissa water resources consolidation project: A critique. Bhubaneshwar: OXFAM.
Varikoden, H., and Revadekar, J. V. (2018). Relation between the rainfall and soil moisture during different phases of Indian monsoon. Pure Appl. Geophys. 175, 1187–1196. doi: 10.1007/s00024-017-1740-6
Venkatachalam, L., and Balooni, K. (2018). Water transfer from irrigation tanks for urban use: can payment for ecosystem services produce efficient outcomes? Int. J. Water Resour. Dev. 34, 51–65. doi: 10.1080/07900627.2017.1342610
Venot, J.-P., Reddy, V. R., and Umapathy, D. (2010). Coping with drought in irrigated South India: farmers’ adjustments in Nagarjuna Sagar. Agric. Water Manag. 97, 1434–1442. doi: 10.1016/j.agwat.2010.04.009
Vidanage, S. P., Kotagama, H. B., and Dunusinghe, P. M. (2022). “Sri Lanka’s small tank Cascade systems: building agricultural resilience in the dry zone” in Climate change and community resilience. eds. A. K. E. Haque, P. Mukhopadhyay, M. Nepal, and M. R. Shammin (Singapore: Springer).
Xiang, X., Li, Q., Khan, S., and Khalaf, O. I. (2021). Urban water resource management for sustainable environment planning using artificial intelligence techniques. Environ. Impact Assess. Rev. 86:106515. doi: 10.1016/j.eiar.2020.106515
Yadav, S. S., and Lal, R. (2018). Vulnerability of women to climate change in arid and semi-arid regions: the case of India and South Asia. J. Arid Environ. 149, 4–17. doi: 10.1016/j.jaridenv.2017.08.001
Keywords: agriculture, climate change, sustainable development goals, rainwater harvesting, irrigation, water resources management, natural hazard mitigation, public policy and governance
Citation: Jain S, Srivastava A, Vishwakarma DK, Rajput J, Rane NL, Salem A and Elbeltagi A (2024) Protecting ancient water harvesting technologies in India: strategies for climate adaptation and sustainable development with global lessons. Front. Water. 6:1441365. doi: 10.3389/frwa.2024.1441365
Edited by:
Nevil Wyndham Quinn, University of the West of England, United KingdomReviewed by:
Sreenath Dixit, International Crops Research Institute for the Semi-Arid Tropics (ICRISAT), IndiaMark Everard, University of the West of England, United Kingdom
Copyright © 2024 Jain, Srivastava, Vishwakarma, Rajput, Rane, Salem and Elbeltagi. This is an open-access article distributed under the terms of the Creative Commons Attribution License (CC BY). The use, distribution or reproduction in other forums is permitted, provided the original author(s) and the copyright owner(s) are credited and that the original publication in this journal is cited, in accordance with accepted academic practice. No use, distribution or reproduction is permitted which does not comply with these terms.
*Correspondence: Ali Salem, c2FsZW0uYWxpQG1pay5wdGUuaHU=