- 1Department of Environmental Science and Disaster Management, Bangabandhu Sheikh Mujibur Rahman Science and Technology University, Gopalganj, Bangladesh
- 2Department of Environmental Science and Engineering, Bangladesh University of Textiles (BUTEX), Dhaka, Bangladesh
- 3Department of Marine Environmental Engineering, Gyeongsang National University, Gyeongsangnam-do, Republic of Korea
Every day, the amount of quality fresh water decreases notably due to contamination of drinking water. As a result, people use commercially supplied jar water in the southern part of Bangladesh as well as in the Gopalganj municipal area. This study aims to investigate the physicochemical parameters, trace elements, and microbial parameters for assessing human health risks from oral ingestion of these elements. This study gathered commercially supplied jar water samples from 15 companies in the Gopalganj municipal area during the post-monsoon season. Temperature, potential of hydrogen (pH), total dissolved solids (TDS), electrical conductivity (EC), and salinity showed significant variation among the samples, and the level was within the national and international standard limits. The concentrations of iron (Fe) and arsenic (As) were measured using a UV–VIS spectrophotometer and atomic absorption spectroscopy (AAS), respectively. The microbial analysis was conducted by the membrane filtration method. The study found that the water samples had an average concentration of 0.16 mg/L in terms of Fe and 0.016 mg/L in terms of As, with the mean value of As exceeding the standard limits. On the other hand, the mean Fe concentration value did not exceed the standards. Probable human health risk from heavy metal in the jar water was determined by hazard quotient (HQ), hazard index (HI), and carcinogenic risk (CR) assessments. A child (HI = 3.5914) is more vulnerable to non-carcinogenic human health risks than an adult (HI = 1.6931). Furthermore, pollution in water samples was found to pose a high carcinogenic risk, with children (CR = 1.6 × 10−3) being more vulnerable to carcinogenic risk than adults (CR = 7.5 × 10−4). In the microbial analysis, 100% of the samples exceeded the total coliform and fecal coliform standard limits, and 80% of the samples exceeded the Escherichia coli standard limits. Among the samples, 7% present high risk, around 47% present medium risk, 26% show low risk, and 20% show no risk based on the E. coli standard limits. This study did not find any samples that exceeded the extremely high limit for E. coli concentration. This study found that drinking jar water samples had inadequate quality, which may increase the risk of water-borne infections such diarrhea as well as heart disease, diabetes, and cancer. As a result, the manufacturer of drinking water in jars must carry out the essential quality control procedures, and the government should regularly monitor the procedures.
1 Introduction
Water is an essential element that has allowed us to survive in the world (Hasan et al., 2019). However, human activity causes more water scarcity and puts risk on drinking water sources every day (Molden, 2020). Water scarcity affects two-thirds of the world’s population at least once a year, and 1.6 billion people currently have an economic water deficit (Mekonnen and Hoekstra, 2016). Stress and the scarcity of water have both gotten worse in recent years. By 2025, more than 1.8 billion people worldwide may face a total water shortage (UNESCO World Water Assessment Programme, 2012). Global development, industrial activities, and climate change have contributed to the contamination of water bodies due to floating plastic bags, synthetic waste, heavy metals, and salinity intrusion (Nayak et al., 2023; Rahman et al., 2020; Sakamoto et al., 2019; Walker et al., 2019; Zamora-Ledezma et al., 2021). To overcome the problem, the United Nations proposed SDG 6.1, which states that “widespread and equal availability of safe and affordable drinking water for all by 2030” (UNICEF, 2019).
Over 97% of the people of Bangladesh, a developing nation in South Asia, have access to improved water supplies (UNICEF and WHO, 2015). However, inadequately managed water services serve less than 40% of the population. Furthermore, the World Bank (2018) discovered that hazardous bacteria, salt, or heavy metals had polluted about half of the delivered water. However, naturally occurring groundwater has significant levels of geogenic arsenic (As) (Saha et al., 2019). At least 59 out of 64 districts in Bangladesh have reported arsenic issues, exposing 30 million people to levels of arsenic above the national standard of 0.05 mg/L (Huq et al., 2020; Rahman et al., 2016). Furthermore, there are seasonal and geographical variations in the country’s water service distribution, and the service quality is typically worse in hydro-geologically sensitive and difficult-to-reach places (Mondal, 2015). Thus, achieving the SDG for Bangladesh requires giving extra consideration to hydro-geologically critical, difficult-to-reach water-insecure places (Hossain et al., 2021).
Gopalganj district holds significant importance in terms of water-quality risk in Bangladesh’s central south. Gopalganj is not particularly close to any coasts, yet there have recently been reports of high salinity in the surface water and groundwater systems (Shammi et al., 2017). Several important variables, including stream flow, poor watershed management, climate variability, and human-induced activities, are contributing to the diminishing quality of groundwater in Gopalganj (Shaibur et al., 2024a). Contamination has been increasing in surface water and groundwater (Shaibur et al., 2024b). As and manganese (Mn) also negatively impact the south-central regions of Bangladesh, which are important in terms of water quality (Atikul Islam et al., 2017). Prior investigations focused on Gopalganj and the adjacent areas indicated the distribution of heavy metals in the south-central region (Atikul Islam et al., 2017; Shaibur et al., 2024b). Gopalganj found both carcinogenic and noncarcinogenic human health risks associated with arsenic, iron, nitrate, copper, manganese not only for adults but also for children. Most of the samples from Gopalganj and 85% of Kashiani upazila revealed chronic human health risks (Rahman et al., 2018; Shaibur et al., 2024a).
In recent years, the practice of drinking bottled water has expanded in upper-middle-class and high-income countries due to a variety of factors, including contaminated ground and surface water (Hu et al., 2011; Ward et al., 2009). However, 1.8 billion people worldwide use fecally contaminated jars and bottled water (Bain et al., 2014; Onda et al., 2012). Packaged water from low and lower-middle-income countries was 4.6 and 13.6 times more likely to have total coliforms and fecal indicator bacteria, respectively, than packaged water from upper-middle and high-income countries (Williams et al., 2015). Restaurants, medical facilities, diagnostic centers, schools, residential areas, and nearly all offices and industries in Bangladesh receive commercially supplied jars and bottled water (Zuthi et al., 2009). In Chittagong, a study found that the majority of people used supplied jar water, with 60.53% of samples contaminated by microbial entities such as total coliform, fecal coliform, and 11 bacterial species (Mina et al., 2018). Moreover, fecal coliform was found in the supplied jar water in Nakla, Sherpur, where the heterotrophic plate count was 2.4 × 105 cfu/100 mL (Sarker et al., 2019).
Contaminated drinking water causes about 17% of global communal diarrhea, according to a previous study (Levy, 2015). In developing nations, water-related illnesses account for around 80% of all illnesses and more than one-third of all fatalities (Mazurkiewicz et al., 2020). Globally, almost 7 million people die from different diseases related to water every year (WHO, 2017). An estimated 2,000 children under the age of five pass away from water related disease every day throughout the world (UNICEF, 2013). The estimated annual death toll from waterborne infections was 1.8 million individuals worldwide, the majority of whom were children from developing nations (WHO, 2022a). Poor sanitation and contaminated water supplies were responsible for the majority of the fatalities (Rochelle-Newall et al., 2015).
Microbial and trace element contamination in potable drinking water in Bangladesh, along with Gopalganj, is increasing day by day. As a result, people use improved and treated commercially supplied jar water for their daily lives. To prevent further pollution, it is now critical to monitor the quality of water sources, particularly microbial characteristics. Typically, we employ chemical and microbiological indicators to evaluate quality, but from a public health perspective, microbial quality is more crucial as some pathogenic organism-caused epidemic outbreaks can spread rapidly. On the other hand, trace element-contaminated water can cause cancer in the human body. The study evaluates the microbiological quality of commercially supplied jar water in the Gopalganj municipal area focusing on the presence of total coliform bacteria and fecal bacteria such as Escherichia coli (E. coli), as well as the concentration of trace elements like iron and arsenic and the health risks associated with them.
2 Materials and methods
2.1 Study area
Thirteen areas in the Gopalganj municipal area were selected for the study (Table 1). In the region. different companies sell drinking water in jars and they collect water from sallow or deep-water tubewell. Gopalganj is located on the Madhumati riverbank at 23°00′47.67″ N and 89°49′21.41″ E. The Gopalganj municipal area is composed of 15 wards and 46.88 km2 (Bangladesh Bureau of Statistics, 2011). Gopalganj appears to be less than 2 m above sea level. The following floodplains make up Gopalganj: 23% Old Meghna, 41% Gopalganj-Khulna Beels, 5% Ganges, 30% active low Ganges River, and 1% others (Molla, 2019; Shaibur and Howlader, 2020). The main productive aquifer, which is between 35 and 145 feet below the surface, is roughly 40 feet thick (Ferdous et al., 2016).
2.2 Sampling
A total of 15 water samples were collected in the post monsoon season from different companies in the Gopalganj Municipal Area that were used for drinking and supplied through commercial jars among the consumers. Several distinct companies in the municipality supplied the drinking water, and this study aimed to include all of them. Four sets of sterile and cap-secured glass bottles were used for sampling, one set for physicochemical analysis, one set for microbial analysis, and the rest of the sets for trace element analysis. For microbial analysis, bottles were autoclaved at 121°C for 30 min, and the collected samples were immediately transferred to the laboratory. Furthermore, during sampling, 65% concentrated HNO3 acid was added to the trace metals analysis sample immediately to bring the pH level to 2 to minimize precipitation and adsorption onto the bottle walls (Haque et al., 2020). A calibrated multi-parameter water quality meter was used to measure the physicochemical parameters such as temperature, pH, TDS, electrical conductivity (EC), and salinity (HQ40d multi, HACH International, United States). For determining the arsenic (As) concentration, this study used atomic absorption spectroscopy (AAS) (AA-7000, Shimadzu Corporation). Samples were digested to remove organic matter interference and change particulate-associated metals into an AAS-detectable state (Sankararamakrishnan and Mishra, 2018). A UV–VIS spectrophotometer (UV-1900i, A125358, Shimadzu Corporation) was used to measure the amount of iron (colorimetric method). The samples were filtered through glass fiber filter (GF/F; 0.45 μm) paper. The number of coliform bacteria present in a water sample was determined by the coliform test using the membrane filtration method (Pant et al., 2016). The water was pumped through the filter paper using a vacuum or suction pump (AS20). The filters were cultured for 24 h at 35°C or 44.5°C in Petri dishes that were filled with MFC broth. After incubation, the petri dishes were taken out of the incubator, and coliform colonies were counted on each plate. All the samples were analyzed in triplicate to ensure reproducibility and statistical validity.
2.3 Human health risk assessment of metal contamination
Risk assessment is the process of determining how likely it is that any particular magnitude of harmful health effects will occur over a certain time period, depending on the hazard and exposure (Bortey-Sam et al., 2015). Each trace element or metalloid’s health risk assessment is often based on quantification of the risk level and expressed as a cancer-causing or non-carcinogenic health risk (US EPA, 2009a). Two primary toxicity risk variables are the slope factor (SF) to determine cancer risk and the reference dose (RfD) to determine cancer prevention risk (Supplementary Table S1; Lim et al., 2008). According to Liu et al. (2011), the exposure mechanisms for metals include the dermal absorption of pollutants in water, which adhere to exposed skin, and direct swallowing of water. This study calculated the chronic daily intake from oral and skin absorption pathways using (Equation 1), which was adapted from the US Environmental Protection Agency (Ecetoc, 2001; Giri and Singh, 2015; USEPA, 2004; Wongsasuluk et al., 2014):
Here, CDI = chronic daily intake (μg/kg/day), CW = concentration of trace metal in water (μg/L), IR = ingestion rate (L/day; 2.2 for adults, 1 for children), EF = exposure frequency (days/year, 365), ED = exposure duration (year; oral = 70 for adults, 10 for children), BW = body weight (kg; 70 kg for adults, 25 kg for children), AT = average time (days; 25,550 for adults, 3,650 for children) [Ecetoc, 2001; EPA, 2001; Kavcar et al., 2009; US Environmental Protection Agency (USEPA), 1999; USEPA, 2009a; USEPA, 2004; Weyer et al., 2001].
This research evaluated the health risks of drinking groundwater by looking at its long-term (noncancerous) and short-term (cancerous) effects. It was done by calculating the CDI and toxicity values for each heavy metal or metalloid. The hazard quotient (HQ) was used in this study to look at any possible non-cancerous risks that might come with being exposed to pollutants that are cause for concern (Equation 2). The HQ is calculated by dividing the estimated contaminant intakes from each oral exposure route by the reference dose (RfD). If the value of HQ exceeds 1, the risk of negative non-carcinogenic health impacts is undesirable, but acceptable if it is less than 1 (Giri and Singh, 2015; Wongsasuluk et al., 2014).
This study creates the Hazard Index (HI) by combining the separate HQs for elemental risk evaluation (Equation 3). There may be possible non-cancerous consequences for health if the values of HQ and HI exceed 1, whereas HI less than 1 implies there is no danger of health problems (Supplementary Table S2; Haque et al., 2021; Sikder et al., 2013).
The incremental chance that a person will eventually acquire cancer as a result of exposure to a suspected carcinogen is known as carcinogenic risk (CR) (Habib et al., 2020; Islam et al., 2020). The accepted specific slope factor (CSF) of the carcinogens in Equation 4 was used to evaluate the risk of exposure.
Where CR is the cancer risk for each metal for the specific routes, CSF is called the slope factor of cancer-causing contaminants (μg/kg/day)−1. CSF can vary for various routes and one of them is oral, the CSF value of As for adults and children is 0.0015 and 0.00038 μgKg−1 day−1, respectively (De Miguel et al., 2007; EPA, 2001; Gao et al., 2019). A risk value <10−6 represents no carcinogenic risk to health, while a risk value >1 × 10−4 suggests a high risk of developing cancer. A risk value ranging from 1 × 10−6 to 1 × 10−4 signifies an acceptable risk to human health (Boateng et al., 2019; Habib et al., 2020; USEPA, 2009b).
2.4 Microbial health risk assessment
According to WHO (2006), total coliform, fecal coliform specially E. coli must not be detected in the water. This study divides drinking water samples into five risk categories based on the E. coli concentration. These categories are (i) no risk (E. coli concentration < 1 CFU/100 mL), (ii) low risk (E. coli concentration 1–10 CFU/100 mL), (iii) intermediate risk (E. coli concentration 11–100 CFU/100 mL), (iv) high risk (E. coli concentration 101–1,000 CFU/100 mL), and (v) very high risk (E. coli concentration > 10,000 CFU/100 mL) (Odonkor and Mahami, 2020).
2.5 Statistical analysis
Bivariate statistical analysis namely Pearson’s correlation matrix (PCM) was performed by SPSS (V.22). Microsoft Excel 2016 was also used for statistical analysis and other calculations.
3 Results and discussion
3.1 Physico-chemical properties of water
Table 2 displays the physicochemical parameters of the collected samples, including temperature, pH, TDS, EC, salinity, Fe, and As. The average value of pH was 6.73 ± 0.49. According to WHO (2022b), almost all the samples, ~73.33%, were within the drinking limit, while ~26.67% were below the range (Figure 1A). A previous study portrayed that the surface water and groundwater pH of Gopalganj District were 8.24 (7.50–9.60) and 7.15 (6.89–7.78), respectively (Shammi et al., 2016). On the other hand, the pH value of Dhaka city groundwater varied from 6.66 to 8.19 with an average of 7.32 (Bodrud-Doza et al., 2020), whereas the pH in Rajshahi city varied from slightly acidic to neutral (Mostafa et al., 2017). These differences were most likely based on geographical locations, as Dhaka and Rajshahi are farther from the coastal zone than this study. From the temperature data, the average value was 25.9 ± 1.14°C (Figure 1B). In this study, the water collected from the jar at room temperature. The TDS of drinking water samples has an average value of 4.42 ± 7.90 mg/L (Figure 1C) which were within the acceptable limit prescribed by WHO (2022b). In Dhaka city, groundwater TDS values varied from 32.9 to 211.0 mg/L, with an average of 72.22 (Bodrud-Doza et al., 2020). In Khulna City, the average value of tubewell water was 1188.7 mg/L (Mahmud et al., 2020), and another study reported the range from 237.0 to 3,112 mg/L with an average of 1,556.05 mg/L (Islam et al., 2017). For commercially supplied jar water in Chittagong City, TDS varied from 0 to 0.00015 g/L (Mina et al., 2018). These variations were most likely caused by the location of the sampled regions and the depth of the water sources. The EC value of this study was 9.60 ± 16.81 μS/cm (Figure 1D). The groundwater of Gopalganj city’s EC was 3206.95 (pre-monsoon) and 3218.47 μs/cm (post-monsoon); the EC values ranged from 600 to 9,380 μs/cm during pre-monsoon and 274–9,190 μs/cm during post-monsoon (Rahman et al., 2018). The study showed that EC ranged from 55 to 353 μs/cm, with a mean of 120.64 μs/cm in the groundwater of Dhaka (Bodrud-Doza et al., 2020) and a mean of 1,650 μs/cm in Khulna (Mahmud et al., 2020). Changes in the depths of the water sources most likely caused the fluctuation in EC, although their locations may also have played a role. In addition, low salinity was found throughout the study area, with an average of 0.003 ± 0.01 ppt (Figure 1E). A previous study reported that the range of groundwater was 0.2–5.2 ppt, with an average of 1.70 ppt (Rahman et al., 2018).
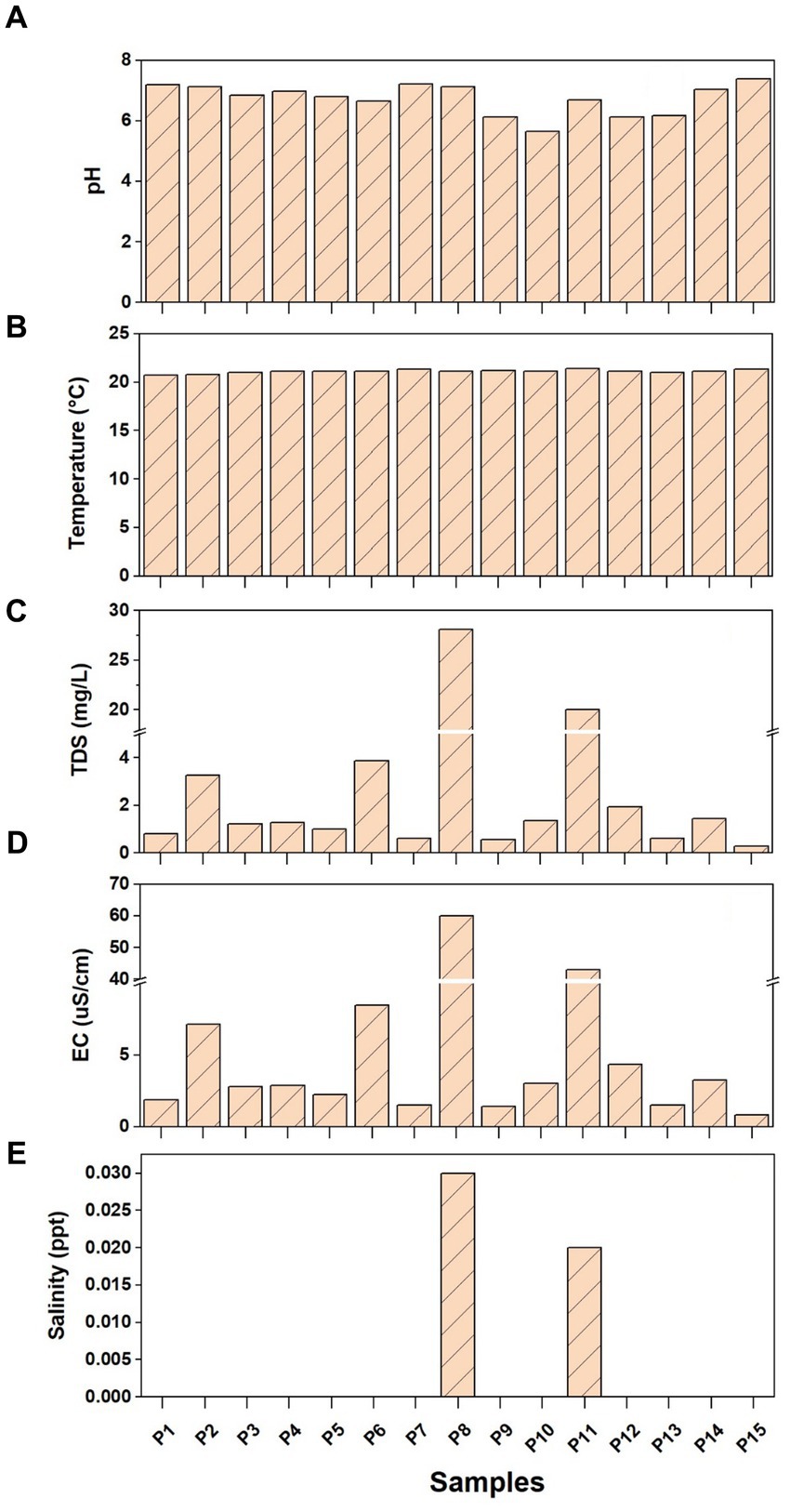
Figure 1. The physicochemical parameters of the studied samples, whereas (A) pH, (B) Temperature, (C) Total Dissolved Solids (TDS), (D) Electrical Conductivity (EC), and (E) Salinity.
This study further investigated the trace metals of the studied samples (Figure 2). The mean value of arsenic was 0.016 ± 0.05 mg/L (Figure 2A) where almost 87% were safe for drinking. Rahman et al. (2018) investigated that, in the groundwater of Gopalganj during the pre-monsoon, the As concentration ranged from 5 to 198 μg/L (mean 50.3 μg/L), whereas in the post-monsoon, it ranged from 5 to 102.5 μg/L (mean 37.17 μg/L). The observed seasonal changes in arsenic levels in groundwater were attributed to a mixture of mineral dissolution, microbiological activity, and river-water flow (Rahman et al., 2018). In this study, Figure 2B describes mean value of Fe which was 0.16 mg/L. According to Rahman et al. (2018), average concentrations of Fe in the groundwater of Gopalganj at pre-monsoon and post-monsoon were 5.12 and 3.31 mg/L, respectively. Iron is essential for human nutrition, but excessive amounts of drinking water can cause lethargy and chronic anemia (Søgaard et al., 2017). Most of the samples were within the standard limit, but some samples were not because of the age or malfunctioning of the treatment machines. The variation of arsenic and iron might be caused by the treatment process. This study treated all commercially supplied jar water, while other studies directly collected samples from various sources. This study treated all commercially supplied jar water, while other studies directly collected samples from various sources. In treated jar water in Chittagong, a lower amount of Fe was found, but there was no trace of As, Pb, or Cr (Mina et al., 2018).
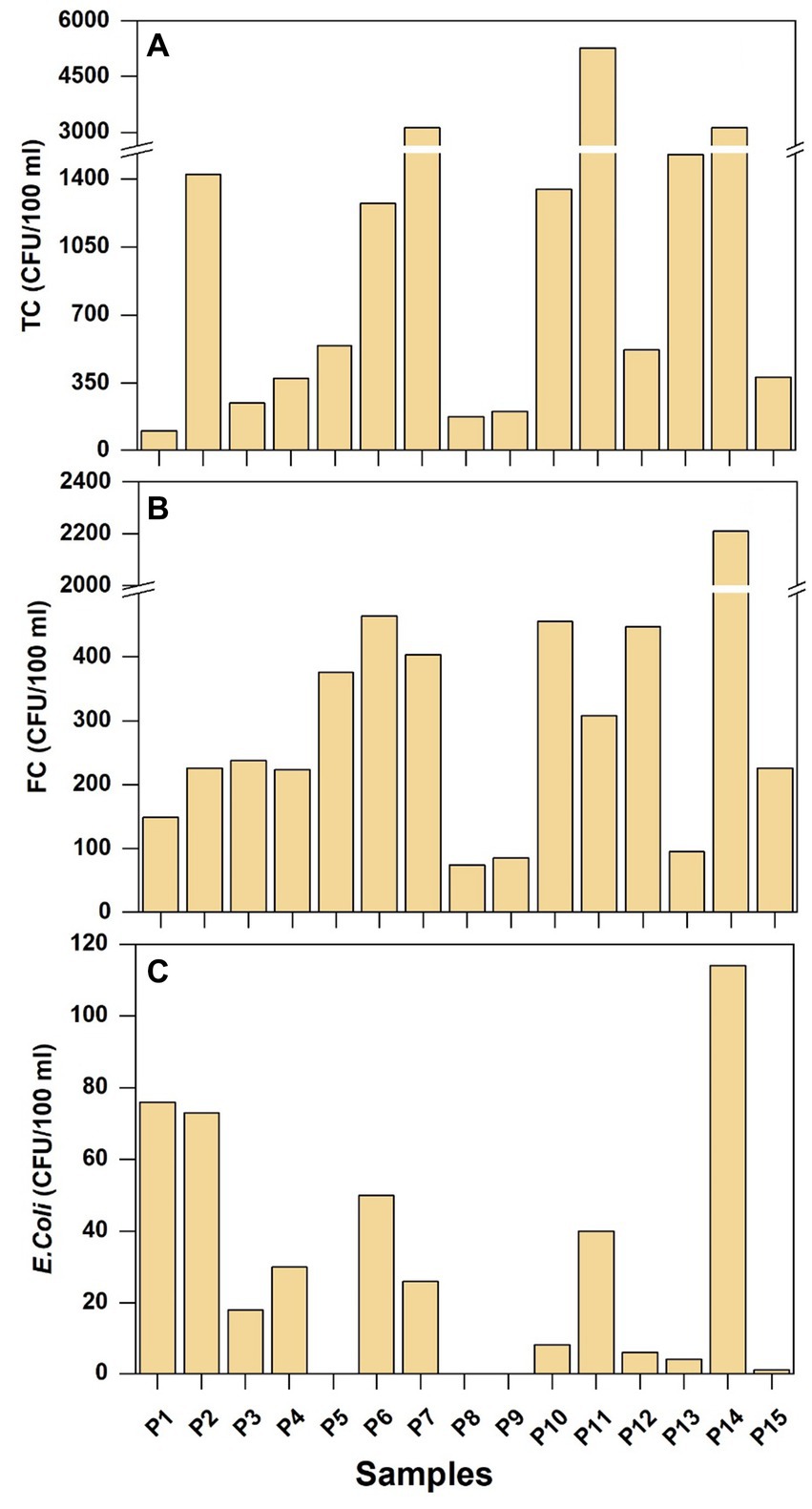
Figure 3. The microbial parameters of studied samples, where as (A) Total Coliform (TC), (B) Fecal Coliform (FC), and (C) Escherichia coli.
3.2 Overview of microbial parameter
This study collected drinking water samples from 15 different sources. Table 3 presents the concentration of indicator bacteria in water samples from each source. The studied water sample describes the range of total coliform, which was 99–5,265 CFU/100 mL. The mean was 1309.33±1472.25 CFU/100 mL, while the 25% quartile was 248 and the 75% was 1,525 (Figure 3A). The maximum count of the fecal coliform was 2,210 CFU/100 mL, and the minimum count was 74 CFU/100 mL (Figure 3B). The mean of the parameter was 398.73 ± 519.22 CFU/100 mL (Figure 3C). The range of E. coli was 0–5,265 CFU/100 mL. The mean was 29.73 ± 34.80 CFU/100 mL, while the 25% quartile was 1 and 75% was 50 CFU/100 mL. According to the WHO, there must be no E. coli in the drinking water (WHO, 2006). Around 20% of the water sample was within the standard range, whereas 80% of the sample exceeded the limit range. According to PCR analysis and a cultural test, the prevalence of bacteria in commercially provided drinking jar water in Chittagong City, Bangladesh, was 60.53 and 50% of the sample, respectively (Mina et al., 2018). Deep-tube well water and commercially supplied water are devoid of bacteria that cause illness such as water-borne disease, diarrhea, and vomiting (Shaibur et al., 2012). Poor environmental conditions and worker personal hygiene significantly contribute to the pollution of commercially supplied drinking water in developing countries (Mina et al., 2018). The presence of indicator organisms in the water poses a serious threat to the community, as all these earlier studies emphasized, and they urged processors and handlers to employ proper manufacturing methods (Mina et al., 2018).
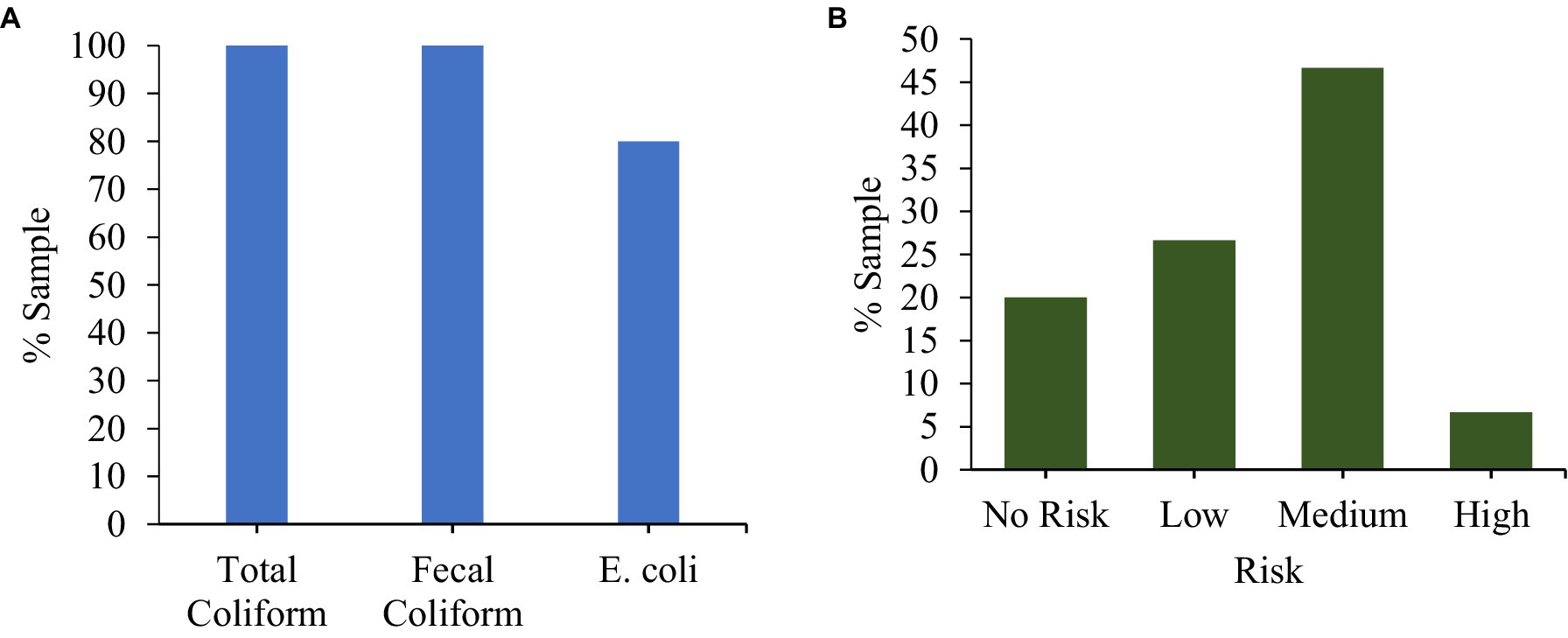
Figure 5. (A) Percentage of samples exceeding the standard limit; and (B) Percentage of samples which has human health risk due to microbial contamination.
3.3 Human health risk assessment
3.3.1 Risk due oral ingestion
Table 4 presents a summary of non-carcinogenic and carcinogenic risks for adults and children. The average HI of these trace elements was greater than 4, which indicates a higher chronic risk for these non-carcinogenic elements. Compared to adults, HI values were almost three times higher in the child section. This suggests that children were more vulnerable than adults in the sampling areas. The carcinogenic risk for both adults and children exceeded the standard limit (10−6 to 10−4) (USEPA, 2004).
However, the mean (range) HQ oral (adult) for As and Fe were 1.6765.513 (0.104–21.58) and 0.169 ± 0.016 (0.0023–0.689), respectively. In addition, the mean (range) HQ oral (children) for As and Fe was 3.556 ± 11.693 (0.222–45.77) and 0.0359 ± 0.034 (0.0049–0.1461), respectively. The average strength of HQ oral values in the study area ranked As higher than Fe for both adults and children. The HI calculation was also done for adults and children. It indicates that there is a non-carcinogenic risk for both adults and children. In this case, children were more vulnerable than adults. Furthermore, approximately 73% of the samples were within the acceptable range with respect to arsenic carcinogenic risk. The other four samples contain 27%, which poses a significant carcinogenic risk to adults and children (Figure 4).
Researchers have proven that oral ingestion of drinking water increases the risk of cancer in both adults and children (Das et al., 2009). Additionally, increased threats at low concentrations of As exposure result in deaths from cancer cardiovascular disease, and other infectious diseases in Bangladesh (Sohel et al., 2009). Moreover, iron has a potential link to several chronic illnesses, including diabetes and heart disease (Ghosh et al., 2020).
According to Rahman et al. (2018), the groundwater in Gopalganj poses a significant health risk to adults and children, with mean Hazard Quotient and Hazard Index values based on Fe, As, Mn, NO3−, B, and F-fluctuating depending on seasonal change. In the pre-monsoon and post-monsoon seasons, the mean HQ of Fe, As, Mn, NO3−, B, and F− were 1.14, 11.18, 0.67, 0.41, 1.42, 0.43 and 0.54, 5.27, 0.31, 0.20, 0.67, and 0.20, respectively. In both seasons, there was a high risk of As-related cancer in adults (65.20%) and children (69.57%). Another study found that the carcinogenic risk (CR) in Kashiani Upazila under the Gopalganj district was extremely high. The CR ranged from 1.11 × 10−3 to 9.0 × 10−2 for adults and 2.7 × 10−6 to 4.2 × 10−2 for children (Shaibur et al., 2024a). According to a recent examination, in Manikganj district, a higher level of As was found in groundwater, which was related to a higher CR for children (1.94 × 10−3) in comparison to adults (9.20 × 10−4) (Rahman et al., 2020). In the Bengal basin, the study area’s geology (Das et al., 2009; Rahman et al., 2016) and human activity (Kundu et al., 2008) were the two possible sources of As. Furthermore, children in Bangladesh’s capital are at risk for carcinogenic health problems if they consume contaminated groundwater over an extended period of time (Bodrud-Doza et al., 2020).
3.3.2 Microbial contamination
According to the WHO guideline, all the samples of total coliform and fecal coliform exceeded the standard limit, causing serious human health effects. Moreover, 80% of samples containing E. coli exceed the standard limit (Figure 5A). It can cause severe stomach cramps, bloody diarrhea, and vomiting (Alizadeh Behbahani et al., 2019). In Gopalganj, substantial rates of acute watery diarrhea-related morbidity and even mortality—which is uncommon in Bangladesh—were reported to be very high (Khan et al., 2023) which might be due to microbial contamination. According to WHO (2006) and based on E. coli, there were 7% of samples with high risk, around 47% with medium risk, 26% with low risk, and 20% with no risk (Figure 5B). The investigation found no samples that exceeded the extremely high limit. The graph indicates a concerning level of contamination in the treated jar water. Mina et al. (2018) conducted research and found that, in Chittagong, there were 26.32 and 18.42% of total and fecal coliform, respectively, in commercially supplied jar water. Furthermore, the authors discovered E. coli and other microbial organisms in the jar water. Mahbub et al. (2012) reported that the Dhaka WASA-supplied drinking water tested positive for E. coli bacteria in 51.11% of samples and positive for coliform in 57.78% of tests. Another study revealed that E. coli contaminated 46% of Dhaka’s drinking water (Saima et al., 2023). In Chittagong, treated water supplied by WASA for drinking was highly contaminated with E. coli, with a load of more than 8 × 103 CFU/mL (Debnath et al., 2022). In the drinking water that Khulna City Corporation supplies, total coliform counts at Nirala, Sonadanga, Rupsa, and Hadispark were 23, 93, 23, and 460 CFU/100 mL, respectively (Nasrin et al., 2022). Mou et al. (2023) investigated whether TC, FC, and E. coli were present in the drinking water provided at various restaurants. While E. coli infected 70% of the samples, TC and FC contaminated 100% of them. According to earlier research, the majority of Bangladesh’s drinking water filtration processes were insufficient in their ability to eliminate microbiological contamination. The primary reason for this was improper management of the instruments and processes (Nishat et al., 2023). Another contributing factor was the prolonged use of unwashed bottles (jars). Research has shown that poor personal hygiene and sanitation of transporters and handlers, along with environmental sanitation, significantly contribute to the contamination of commercially supplied drinking water in developing nations (Ashbolt, 2004; Dada, 2009; Mina et al., 2018). The Bangladesh Standards and Testing Institute (BSTI) provided guidelines and clearance for the manufacturing of commercially supplied drinking water, including handling, processing, and delivery from factory to customer. However, many companies were unable to meet these requirements. As a result, disease-causing bacteria and pathogens have infiltrated the drinking water in the provided jars. Furthermore, a large number of the defaulting manufacturers lacked the necessary licenses to operate, which could be the cause of additional contamination in the supplied drinking water (Mina et al., 2018). The suppliers claimed to follow the treatment process, as depicted in Figure 6. However, a field survey revealed that they did not adhere to the entire process, and the majority of the RO machines had expired. Effective adherence to the BSTI’s implemented processes is crucial.
3.4 Correlation matrix
This study used bivariate statistics to carry out Pearson’s correlation matrix (PCM) and determine the influence of parameter pairs on drinking water quality. Table 5 presents the results of the correlation analyses. Several pairs have a strong relationship with a very high level of significance. This study found a perfect correlation between EC-TDS (r = 1.000) and strong correlations between EC-Salinity (r = 0.992). Besides, in terms of microbial analysis, there was a strong correlation between E. coli and fecal coliform (r = 0.656). The pH showed a positive correlation with TDS, EC, salinity, and other microbial and heavy metal parameters, while temperature showed a negative correlation. TDS showed a very high positive correlation (r = 0.992) with salinity, as well as a positive correlation with other parameters. It had strong negative correlations with As (r = −0.095), FC (r = −0.160), and E. coli (r = −0.104). However, it had weak positive correlations with temperature (r = 0.255), Fe (r = 0.075), and TC (r = 0.268). In addition, there was also a negative correlation between EC, As, Fc, and E. coli. Salinity also had a positive correlation with temperature, Fe, and TC, while it had a negative correlation with As, Fc, and E. coli (Table 5). The temperature was very low, negligible, and exhibited a low positive correlation with As (r = 0.25), FC (r = 0.081), and TC (r = 0.485), while it had a negative correlation with Fe (r = −0.103) and E.coli (r = −0.391). In terms of As, it was negatively corelated with Fe, FC, and TC, whereas there was a minor positive correlation with E.coli (r = 0.006). Fe was negatively associated with three other microbial parameters (FC, TC, and E. coli). Fe had a low positive correlation with TC (r = 0.405) and a moderate positive correlation with E. coli (r = 0.656). Furthermore, there was a positive correlation between TC and E. coli (r = 0.384).
4 Conclusion
The surface and groundwater in the Gopalganj municipal area are not suitable for drinking because salinity and pollution are increasing. In their daily lives, people used to drink commercially supplied jar water. The study monitored microbial and trace element contamination in commercially supplied drinking water, as well as the human health risks associated with these factors in the post-monsoon season. The study also determines the physicochemical condition of the water, and it appears that the majority of the drinking water samples meet the standard limits set by the WHO and DoE. However, the results of the evaluation of the human health risk associated with metal contamination through oral ingestion are extremely concerning. The noncarcinogenic risk associated with the water is medium, but the carcinogenic risk is very high for children and high for adults. Moreover, the microbial condition of the water sample is very alarming, where 100% of the samples exceed the standard guidelines of the WHO for total coliform and fecal coliform and 80% of E. coli samples are outside the standard limit. The study also reveals a strong correlation between salinity, TDS, and EC in terms of physicochemical parameters and a strong relationship between fecal coliform and E. coli. Finally, this study suggests that Gopalganj lacks sufficient water quality monitoring, necessitating periodic chemical analysis to assess the water’s suitability for human consumption.
Data availability statement
The original contributions presented in the study are included in the article/Supplementary material, further inquiries can be directed to the corresponding authors.
Author contributions
PB: Data curation, Formal analysis, Investigation, Methodology, Software, Visualization, Writing – original draft. SA: Conceptualization, Data curation, Investigation, Methodology, Supervision, Validation, Writing – original draft. MH: Formal analysis, Investigation, Software, Supervision, Visualization, Writing – original draft, Writing – review & editing. MK: Resources, Software, Visualization, Writing – review & editing.
Funding
The author(s) declare that no financial support was received for the research, authorship, and/or publication of this article.
Acknowledgments
The authors extend their gratitude to Environmental Science Discipline, Khulna University for providing laboratory support for the research. The authors are grateful to Anik Ray, Department of Soil and Environmental Sciences, University of Barishal and Abdullah Bin Kaizer, Department of Environmental Science and Disaster Management, Bangabandhu Sheikh Mujibur Rahman Science and Technology University for their assistance in sample collection and laboratory works. The authors thank to Achinta Biswas and Jonayed Ahmed, Department of Environmental Science and Disaster Management, Bangabandhu Sheikh Mujibur Rahman Science and Technology University for their support in data collection.
Conflict of interest
The authors declare that the research was conducted in the absence of any commercial or financial relationships that could be construed as a potential conflict of interest.
Publisher’s note
All claims expressed in this article are solely those of the authors and do not necessarily represent those of their affiliated organizations, or those of the publisher, the editors and the reviewers. Any product that may be evaluated in this article, or claim that may be made by its manufacturer, is not guaranteed or endorsed by the publisher.
Supplementary material
The Supplementary material for this article can be found online at: https://www.frontiersin.org/articles/10.3389/frwa.2024.1441313/full#supplementary-material
References
Alizadeh Behbahani, B., Noshad, M., and Falah, F. (2019). Inhibition of Escherichia coli adhesion to human intestinal Caco-2 cells by probiotic candidate Lactobacillus plantarum strain L15. Microb. Pathog. 136:103677. doi: 10.1016/j.micpath.2019.103677
Ashbolt, N. J. (2004). Microbial contamination of drinking water and disease outcomes in developing regions. Toxicology 198, 229–238. doi: 10.1016/j.tox.2004.01.030
Atikul Islam, M., Zahid, A., Rahman, M. M., Rahman, M. S., Islam, M. J., Akter, Y., et al. (2017). Investigation of groundwater quality and its suitability for drinking and agricultural use in the south central part of the coastal region in Bangladesh. Expo. Health 9, 27–41. doi: 10.1007/s12403-016-0220-z
Bain, R., Cronk, R., Hossain, R., Bonjour, S., Onda, K., Wright, J., et al. (2014). Global assessment of exposure to faecal contamination through drinking water based on a systematic review. Trop. Med. Int. Health 19, 917–927. doi: 10.1111/tmi.12334
Boateng, T. K., Opoku, F., and Akoto, O. (2019). Heavy metal contamination assessment of groundwater quality: a case study of Oti landfill site, Kumasi. Appl Water Sci 9:33. doi: 10.1007/s13201-019-0915-y
Bodrud-Doza, M., Islam, S. M. D.-U., Rume, T., Quraishi, S. B., Rahman, M. S., and Bhuiyan, M. A. H. (2020). Groundwater quality and human health risk assessment for safe and sustainable water supply of Dhaka City dwellers in Bangladesh. Groundw. Sustain. Dev. 10:100374. doi: 10.1016/j.gsd.2020.100374
Bortey-Sam, N., Nakayama, S. M. M., Ikenaka, Y., Akoto, O., Baidoo, E., Mizukawa, H., et al. (2015). Health risk assessment of heavy metals and metalloid in drinking water from communities near gold mines in Tarkwa, Ghana. Environ. Monit. Assess. 187, 397–312. doi: 10.1007/s10661-015-4630-3
Dada, A. (2009). Sachet water phenomenon in Nigeria: assessment of the potential health impacts. Afr. J. Microbiol. Res. 3, 15–21.
Das, B., Rahman, M. M., Nayak, B., Pal, A., Chowdhury, U. K., Mukherjee, S. C., et al. (2009). Groundwater arsenic contamination, its health effects and approach for mitigation in West Bengal, India and Bangladesh. Water Qual Expo Health 1, 5–21. doi: 10.1007/s12403-008-0002-3
De Miguel, E., Iribarren, I., Chacón, E., Ordoñez, A., and Charlesworth, S. (2007). Risk-based evaluation of the exposure of children to trace elements in playgrounds in Madrid (Spain). Chemosphere 66, 505–513. doi: 10.1016/j.chemosphere.2006.05.065
Debnath, P., Mamun, M.M.A.Al, Karmakar, S., Uddin, M.S., and Nath, T.K. (2022). Drinking water quality of Chattogram city in Bangladesh: an analytical and residents’ perception study. Heliyon 8::e12247. doi: 10.1016/j.heliyon.2022.e12247
DoE (2019). Environmental Law Collection. Department of. Environment, Dhaka, Government of the People's Republic of Bangladesh.
EPA (2001). Baseline human health risk assessment. Vasquez Boulevard and 1-70 superfund site Denver, Denver (co). United States Environmental Protection Agency. United States Environmental Protection Agency. VIII.
Ferdous, F., Rafiq, M. R., and Mahmud, M. I. (2016). Aquifer geometry and water quality in relation to occurrence and distribution of peat in Baghia-Chanda Beel, Bangladesh. J. Sci. Res. 8, 355–370. doi: 10.3329/jsr.v8i3.25360
Gao, B., Gao, L., Gao, J., Xu, D., Wang, Q., and Sun, K. (2019). Simultaneous evaluations of occurrence and probabilistic human health risk associated with trace elements in typical drinking water sources from major river basins in China. Sci. Total Environ. 666, 139–146. doi: 10.1016/j.scitotenv.2019.02.148
Ghosh, G. C., Khan, M. J. H., Chakraborty, T. K., Zaman, S., Kabir, A. H. M. E., and Tanaka, H. (2020). Human health risk assessment of elevated and variable iron and manganese intake with arsenic-safe groundwater in Jashore, Bangladesh. Sci. Rep. 10:5206. doi: 10.1038/s41598-020-62187-5
Giri, S., and Singh, A. K. (2015). Human health risk assessment via drinking water pathway due to metal contamination in the groundwater of Subarnarekha River Basin, India. Environ. Monit. Assess. 187:63. doi: 10.1007/s10661-015-4265-4
Habib, M. A., Islam, A. R. M. T., Bodrud-Doza, M., Mukta, F. A., Khan, R., Bakar Siddique, M. A., et al. (2020). Simultaneous appraisals of pathway and probable health risk associated with trace metals contamination in groundwater from Barapukuria coal basin, Bangladesh. Chemosphere 242:125183. doi: 10.1016/j.chemosphere.2019.125183
Haque, M. M., Niloy, N. M., Khirul, M. A., Alam, M. F., and Tareq, S. M. (2021). Appraisal of probabilistic human health risks of heavy metals in vegetables from industrial, non-industrial and arsenic contaminated areas of Bangladesh. Heliyon 7:e06309. doi: 10.1016/j.heliyon.2021.e06309
Haque, M. M., Niloy, N. M., Nayna, O. K., Fatema, K. J., Quraishi, S. B., Park, J.-H. H., et al. (2020). Variability of water quality and metal pollution index in the Ganges River, Bangladesh. Environ. Sci. Pollut. Res. 27, 42582–42599. doi: 10.1007/s11356-020-10060-3
Hasan, M. K., Shahriar, A., and Jim, K. U. (2019). Water pollution in Bangladesh and its impact on public health. Heliyon 5:e02145. doi: 10.1016/j.heliyon.2019.e02145
Hossain, M. J., Chowdhury, M. A., Jahan, S., Zzaman, R. U., and Islam, S. L. U. (2021). Drinking water insecurity in southwest coastal Bangladesh: how far to SDG 6.1? WaterSA 13:3571. doi: 10.3390/w13243571
Hu, Z., Morton, L. W., and Mahler, R. L. (2011). Bottled water: United States consumers and their perceptions of water quality. Int. J. Environ. Res. Public Health 8, 565–578. doi: 10.3390/ijerph8020565
Huq, M. E., Fahad, S., Shao, Z., Sarven, M. S., Khan, I. A., Alam, M., et al. (2020). Arsenic in a groundwater environment in Bangladesh: occurrence and mobilization. J. Environ. Manag. 262:110318. doi: 10.1016/j.jenvman.2020.110318
Islam, A. R. M. T., Al Mamun, A., Rahman, M. M., and Zahid, A. (2020). Simultaneous comparison of modified-integrated water quality and entropy weighted indices: implication for safe drinking water in the coastal region of Bangladesh. Ecol. Indic. 113:106229. doi: 10.1016/j.ecolind.2020.106229
Islam, S. M. D.-U., Bhuiyan, M. A. H., Rume, T., and Azam, G. (2017). Hydrogeochemical investigation of groundwater in shallow coastal aquifer of Khulna District, Bangladesh. Appl Water Sci 7, 4219–4236. doi: 10.1007/s13201-017-0533-5
Islam, M. A., Rahman, M. M., Bodrud-Doza, M., Muhib, M. I., Shammi, M., Zahid, A., et al. (2018). A study of groundwater irrigation water quality in south-Central Bangladesh: a geo-statistical model approach using GIS and multivariate statistics. Acta Geochim. 37, 193–214. doi: 10.1007/s11631-017-0201-3
Kavcar, P., Sofuoglu, A., and Sofuoglu, S. C. (2009). A health risk assessment for exposure to trace metals via drinking water ingestion pathway. Int. J. Hyg. Environ. Health 212, 216–227. doi: 10.1016/j.ijheh.2008.05.002
Khan, A. I., Islam, M. T., Amin, M. A., Khan, Z. H., and Qadri, F. (2023). Outbreak of diarrheal diseases causes mortality in different geographical locations of Bangladesh during the 2021 COVID-19 era. Front. Public Health 11:1103518. doi: 10.3389/fpubh.2023.1103518
Kundu, M. C., Mandal, B., and Sarkar, D. (2008). Assessment of the potential hazards of nitrate contamination in surface and groundwater in a heavily fertilized and intensively cultivated district of India. Environ. Monit. Assess. 146, 183–189. doi: 10.1007/s10661-007-0070-z
Levy, K. (2015). Does poor water quality cause diarrheal disease? Am. J. Trop. Med. Hyg. 93, 899–900. doi: 10.4269/ajtmh.15-0689
Lim, H. S., Lee, J. S., Chon, H. T., and Sager, M. (2008). Heavy metal contamination and health risk assessment in the vicinity of the abandoned Songcheon Au-Ag mine in Korea. J. Geochem. Explor. 96, 223–230. doi: 10.1016/j.gexplo.2007.04.008
Liu, N., Ni, T., Xia, J., Dai, M., He, C., and Lu, G. (2011). Non-carcinogenic risks induced by metals in drinking source water of Jiangsu Province, China. Environ. Monit. Assess. 177, 449–456. doi: 10.1007/s10661-010-1646-6
Mahbub, K., Nahar, A., Ahmed, M., and Chakraborty, A. (2012). Quality analysis of Dhaka WASA drinking water: detection. J. Environ. Sci. Nat. Resour. 4, 41–49. doi: 10.3329/jesnr.v4i2.10133
Mahmud, A., Sikder, S., and Joardar, J. C. (2020). Assessment of groundwater quality in Khulna city of Bangladesh in terms of water quality index for drinking purpose. Appl Water Sci 10:226. doi: 10.1007/s13201-020-01314-z
Mazurkiewicz, J., Mazur, A., Mazur, R., Chmielowski, K., Czekała, W., and Janczak, D. (2020). The process of microbiological remediation of the polluted Słoneczko reservoir in Poland: for reduction of water pollution and nutrients management. WaterSA 12:3002. doi: 10.3390/w12113002
Mekonnen, M. M., and Hoekstra, A. Y. (2016). Four billion people facing severe water scarcity. Sci. Adv. 2:e1500323. doi: 10.1126/sciadv.1500323
Mina, S. A., Marzan, L. W., Sultana, T., Akter, Y., Mina, S. A., Marzan, L. W., et al. (2018). Quality assessment of commercially supplied drinking jar water in Chittagong City, Bangladesh. Appl Water Sci 8:24. doi: 10.1007/s13201-018-0673-2
Molden, D. (2020). Scarcity of water or scarcity of management? Int. J. Water Resour. Dev. 36, 258–268. doi: 10.1080/07900627.2019.1676204
Molla, R. S. (2019). Distribution of arsenic and heavy metals and correlation among them in groundwater of south Fukra, Kashiani, Gopalganj, Bangladesh. Environ. Biol. Res. 1, 84–105.
Mondal, M. S. (2015). Groundwater resources in the hard to reach areas of Bangladesh: constraints for drinking water supply and strategies for sustainable use. Eur. Water 50, 43–57.
Mostafa, M. G., Uddin, S. M. H., and Haque, A. B. M. H. (2017). Assessment of hydro-geochemistry and groundwater quality of Rajshahi City in Bangladesh. Appl Water Sci 7, 4663–4671. doi: 10.1007/s13201-017-0629-y
Mou, S. I., Swarnokar, S. C., Ghosh, S., Ridwan, M. T., and Ishtiak, K. F. (2023). Assessment of drinking water quality served in different restaurants at Islam Nagor road adjacent to Khulna university campus, Bangladesh. J. Geosci. Environ. Prot. 11, 252–267. doi: 10.4236/gep.2023.119017
Nasrin, S., Saha, S. K., and Rahman, M. M. S. (2022). Assessment of supply water quality of Khulna City Corporation, Khulna, Bangladesh. Khulna Univ. Stud. 6, 13–19. doi: 10.53808/KUS.2005.6.1and2.0341-L
Nayak, A., Matta, G., Prasad Uniyal, D., Kumar, A., Kumar, P., and Pant, G. (2023). Assessment of potentially toxic elements in groundwater through interpolation, pollution indices, and chemometric techniques in Dehradun in Uttarakhand state. Environ. Sci. Pollut. Res. 31, 36241–36263. doi: 10.1007/s11356-023-27419-x
Nishat, S., Zahurul, S., Asna, H., Karmaker, M., and Sarker, U. J. (2023). Microbial contamination in different water sources in Dhaka City. Bangladesh J. Heal. Allied Sci. 1, 7–12.
Odonkor, S. T., and Mahami, T. (2020). Escherichia coli as a tool for disease risk assessment of drinking water sources. Int. J. Microbiol. 2020, 1–7. doi: 10.1155/2020/2534130
Onda, K., LoBuglio, J., and Bartram, J. (2012). Global access to safe water: accounting for water quality and the resulting impact on MDG progress. Int. J. Environ. Res. Public Health 9, 880–894. doi: 10.3390/ijerph9030880
Pant, N. D., Poudyal, N., and Bhattacharya, S. K. (2016). Bacteriological quality of bottled drinking water versus municipal tap water in Dharan municipality, Nepal. J. Health Popul. Nutr. 35:17. doi: 10.1186/s41043-016-0054-0
Rahman, M. M., Bodrud-Doza, M., Muhib, M. I., Binte, K. F., Hossain, M. S., Akter, S., et al. (2020). Human health risk assessment of nitrate and trace metals via groundwater in Central Bangladesh. Pollution 6, 253–266. doi: 10.22059/POLL.2019.288090.691
Rahman, M. M., Islam, M. A., Bodrud-Doza, M., Muhib, M. I., Zahid, A., Shammi, M., et al. (2018). Spatio-temporal assessment of groundwater quality and human health risk: a case study in Gopalganj, Bangladesh. Expo. Health 10, 167–188. doi: 10.1007/s12403-017-0253-y
Rahman, M. M., Sultana, R., Shammi, M., Bikash, J., Ahmed, T., Maruo, M., et al. (2016). Assessment of the status of groundwater arsenic at singair upazila, Manikganj Bangladesh; exploring the correlation with other metals and ions. Expo. Health 8, 217–225. doi: 10.1007/s12403-016-0196-8
Rochelle-Newall, E., Nguyen, T. M. H., Le, T. P. Q., Sengtaheuanghoung, O., and Ribolzi, O. (2015). A short review of fecal indicator bacteria in tropical aquatic ecosystems: knowledge gaps and future directions. Front. Microbiol. 6:308. doi: 10.3389/fmicb.2015.00308
Saha, R., Dey, N. C., Rahman, M., Bhattacharya, P., and Rabbani, G. H. (2019). Geogenic arsenic and microbial contamination in drinking water sources: exposure risks to the coastal population in Bangladesh. Front. Environ. Sci. 7:57. doi: 10.3389/fenvs.2019.00057
Saima, S., Ferdous, J., Sultana, R., Rashid, R. B., Almeida, S., Begum, A., et al. (2023). Detecting enteric pathogens in low-risk drinking water in Dhaka, Bangladesh: an assessment of the WHO water safety categories. Trop. Med. Infect. Dis. 8:321. doi: 10.3390/tropicalmed8060321
Sakamoto, M., Ahmed, T., Begum, S., and Huq, H. (2019). Water pollution and the textile industry in Bangladesh: flawed corporate practices or restrictive opportunities? Sustain. For. 11:1951. doi: 10.3390/su11071951
Sankararamakrishnan, N., and Mishra, S. (2018). “A Comprehensive review on various analytical methods for the determination of inorganic and organic arsenic in environmental samples,” Environmental Contaminants. Energy, Environment, and Sustainability. eds. T. Gupta, A. Agarwal, R. Agarwal, and N. Labhsetwar (Singapore: Springer), 21–41.
Sarker, S., Mahmud, S., Sultana, R., Biswas, R., Sarkar, P. P., Munayem, M. A., et al. (2019). Quality assessment of surface and drinking water of Nakla Paurosova, Sherpur, Bangladesh. Adv. Microbiol. 9, 703–727. doi: 10.4236/aim.2019.98043
Shaibur, M. R., Anzum, M. N., Rana, M. S., and Khan, M. A. S. (2012). Assessment of supplied water quality at Jessore municipality (Pourashava), Bangladesh. Bangladesh J. Environ. Res. 10, 69–87.
Shaibur, M., and Howlader, M. (2020). Groundwater composition at some unions in Kashiani and Kotalipara Upazila of south-central coastal Gopalganj District, Bangladesh. Environ. Biol. Res. 2, 22–36.
Shaibur, M. R., Howlader, M., Ahmmed, I., Sarwar, S., and Hussam, A. (2024a). Water quality index and health risk assessment for heavy metals in groundwater of Kashiani and Kotalipara upazila, Gopalganj, Bangladesh. Appl Water Sci 14:106. doi: 10.1007/s13201-024-02169-4
Shaibur, M. R., Howlader, M., Nahar, N., Hossain, M. S., Mamun, A. M., Shohan, M. H., et al. (2024b). Application of pollution indices to determine pollution intensities in the groundwater of Gopalganj (south-central part). Bangladesh. Groundw. Sustain. Dev. 26:101206. doi: 10.1016/j.gsd.2024.101206
Shammi, M., Rahman, M. M., Islam, M. A., Bodrud-Doza, M., Zahid, A., Akter, Y., et al. (2017). Spatio-temporal assessment and trend analysis of surface water salinity in the coastal region of Bangladesh. Environ. Sci. Pollut. Res. 24, 14273–14290. doi: 10.1007/s11356-017-8976-7
Shammi, M., Rahman, R., Rahman, M. M., Moniruzzaman, M., Bodrud-Doza, M., Karmakar, B., et al. (2016). Assessment of salinity hazard in existing water resources for irrigation and potentiality of conjunctive uses: a case report from Gopalganj District, Bangladesh. Sustain. Water Resour. Manag. 2, 369–378. doi: 10.1007/s40899-016-0064-5
Sikder, M. T., Kihara, Y., Yasuda, M., Yustiawati Mihara, Y., Tanaka, S., Odgerel, D., et al. (2013). River water pollution in developed and developing countries: judge and assessment of physicochemical characteristics and selected dissolved metal concentration. Clean Soil Air Water 41, 60–68. doi: 10.1002/clen.201100320
Søgaard, K. L., Ellervik, C., Svensson, J., and Thorsen, S. U. (2017). The role of Iron in type 1 diabetes etiology: a systematic review of new evidence on a long-standing mystery. Rev. Diabet. Stud. 14, 269–278. doi: 10.1900/RDS.2017.14.269
Sohel, N., Persson, L. Å., Rahman, M., Streatfield, P. K., Yunus, M., Ekström, E.-C., et al. (2009). Arsenic in drinking water and adult mortality. Epidemiology 20, 824–830. doi: 10.1097/EDE.0b013e3181bb56ec
UNESCO World Water Assessment Programme (2012). United Nations world water development report 4: Managing water under uncertainty and risk. UNESCO.
UNICEF (2013). Children dying daily because of unsafe water supplies and poor sanitation and hygiene. OCHA.
US Environmental Protection Agency (USEPA) (1999). A risk assessment-multiway exposure spreadsheet calculation tool.
US EPA (2009a). Guidance on the Development, Evaluation, and Application of Environmental Models. Council for Regulatory Environmental Modeling U.S. Environmental Protection Agency. Washington, DC: USEPA Publication.
US EPA (2009b). National Primary Drinking Water Regulations, United States Environmental Protection Agency.
USEPA (2004). Risk assessment guidance for superfund (RAGS). Volume I. Human health evaluation manual (HHEM). Part E. Supplemental guidance for dermal risk assessment. USEPA 1.
USEPA (2009a). National Primary/Secondary and Drinking Water Regulations. Washington, D.C.: U.S. Environmental Protection Agency.
Walker, D. B., Baumgartner, D. J., Gerba, C. P., and Fitzsimmons, K. (2019). “Surface water pollution” in Environmental and Pollution Science. eds. B. L. Brusseau, I. L. Pepper, and C. P. Gerba (Elsevier), 261–292.
Ward, L. A., Cain, O. L., Mullally, R. A., Holliday, K. S., Wernham, A. G., Baillie, P. D., et al. (2009). Health beliefs about bottled water: a qualitative study. BMC Public Health 9:196. doi: 10.1186/1471-2458-9-196
Weyer, P. J., Cerhan, J. R., Kross, B. C., Hallberg, G. R., Kantamneni, J., Breuer, G., et al. (2001). Municipal drinking water nitrate level and cancer risk in older women: the Iowa women’s health study. Epidemiology 12, 327–338. doi: 10.1097/00001648-200105000-00013
WHO (2006). Guidelines for Drinking Water Quality. First Addendum to Third Edition, Volume 1: Recommendations.
WHO (2017). Diarrhoeal disease [WWW Document]. Available online at: https://www.who.int/news-room/fact-sheets/detail/diarrhoeal-disease (August 16, 2023).
WHO (2022a). Drinking-water. Available online at: https://www.who.int/news-room/fact-sheets/detail/drinking-water (Accessed September 1, 2023).
Williams, A. R., Bain, R. E. S., Fisher, M. B., Cronk, R., Kelly, E. R., and Bartram, J. (2015). A systematic review and meta-analysis of fecal contamination and inadequate treatment of packaged water. PLoS One 10:e0140899. doi: 10.1371/journal.pone.0140899
Wongsasuluk, P., Chotpantarat, S., Siriwong, W., and Robson, M. (2014). Heavy metal contamination and human health risk assessment in drinking water from shallow groundwater wells in an agricultural area in Ubon Ratchathani province, Thailand. Environ. Geochem. Health 36, 169–182. doi: 10.1007/s10653-013-9537-8
World Bank (2018). Promising progress: a diagnostic of water supply, sanitation, hygiene, and poverty in Bangladesh. World Bank, Washington, DC.
Zamora-Ledezma, C., Negrete-Bolagay, D., Figueroa, F., Zamora-Ledezma, E., Ni, M., Alexis, F., et al. (2021). Heavy metal water pollution: a fresh look about hazards, novel and conventional remediation methods. Environ. Technol. Innov. 22:101504. doi: 10.1016/j.eti.2021.101504
Keywords: jar water, supplied water, trace elements, microbial contamination, E. coli, human health risks
Citation: Brahma PP, Akter S, Haque MM and Khirul MA (2024) Probabilistic human health risk assessment of commercially supplied jar water in Gopalganj municipal area, Bangladesh. Front. Water. 6:1441313. doi: 10.3389/frwa.2024.1441313
Edited by:
Geonildo Rodrigo Disner, Butantan Institute, BrazilReviewed by:
Gagan Matta, Gurukul Kangri University, IndiaSunitha Vangala, Yogi Vemana University, India
Copyright © 2024 Brahma, Akter, Haque and Khirul. This is an open-access article distributed under the terms of the Creative Commons Attribution License (CC BY). The use, distribution or reproduction in other forums is permitted, provided the original author(s) and the copyright owner(s) are credited and that the original publication in this journal is cited, in accordance with accepted academic practice. No use, distribution or reproduction is permitted which does not comply with these terms.
*Correspondence: Sharmin Akter, sharminakter.env@gmail.com; Md. Morshedul Haque, morshdeulhaq@gmail.com,;morshedulhaq@ese.butex.edu.bd
†ORCID: Md. Morshedul Haque, https://orcid.org/0000-0001-9799-6948