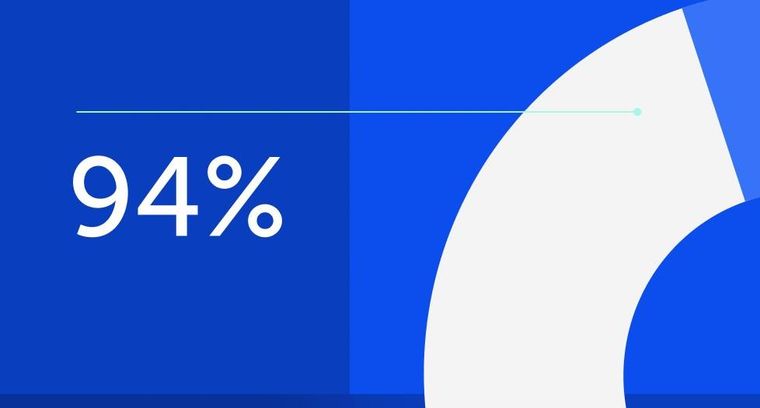
94% of researchers rate our articles as excellent or good
Learn more about the work of our research integrity team to safeguard the quality of each article we publish.
Find out more
EDITORIAL article
Front. Water, 09 September 2024
Sec. Water and Built Environment
Volume 6 - 2024 | https://doi.org/10.3389/frwa.2024.1438086
This article is part of the Research TopicDesigning, Operating, and Rebuilding Infrastructures and Lifelines for Resilience to Extreme FloodingView all 5 articles
Editorial on the Research Topic
Designing, operating, and rebuilding infrastructures and lifelines for resilience to extreme flooding
Recent flood events demonstrate that communities, cities, and nations are not yet prepared for hydroclimate extremes (Eisenberg, 2021; Tanoue et al., 2021). Infrastructure adaptation, where it exists, has largely focused on fail-safe engineering that emphasizes large, gray infrastructure projects while neglecting safe-to-fail solutions on smaller, more adaptive scales that are less destructive when they fail (Kim et al., 2017, 2019, 2022). When seawalls, dams, and drainage systems become overwhelmed by flooding exceeding design tolerances, the results can be catastrophic. Impacts can be compounded by social vulnerabilities and increasingly interdependent infrastructure systems that sacrifice resilience in the interest of efficiency. Population growth and urban development patterns increase threat exposure further, creating a perfect storm of factors and demanding new design paradigms and creative solutions cutting across science, engineering, policy, and community engagement. However, every failure provides opportunities to advance resilient infrastructures through design, to study failure patterns, and to understand the often community-driven successes that help protect against the worst impacts.
Hydroclimate extremes are worsening due to a confluence of factors. The atmosphere is warming, allowing air to hold more moisture, leading to more frequent and intense rainfall events (Martinkova and Kysely, 2020). Compound coastal flooding is projected to increase due to extreme storm surge and high tides coinciding with intense precipitation (Bevacqua et al., 2020). Simultaneously, land use changes are increasing the severity of flood impacts (Rogger et al., 2017). As cities grow into regional urban agglomerations, decreases in surface permeability prevent runoff from absorbing into the soil, intensifying pluvial and fluvial flooding. Furthermore, excessive groundwater abstraction is depleting aquifers and inducing land subsidence, increasing threat exposure for millions of people (Ajjur and Al-Ghamdi, 2022).
These exacerbating factors pose great risk to infrastructure systems, often concentrated in urban areas most prone to flooding. A resilience imperative emerges to rebound and adapt infrastructure to attenuate the future impacts of extreme flooding, and to reinforce and create alternatives to impacted lifeline systems in flood-prone regions (Woods, 2015). However, achieving resilience is difficult as infrastructure systems have hidden interdependencies, feedback effects, and emergent phenomena (Almoghathawi et al., 2019). The design paradigm with which many infrastructure systems are built prioritize capital and operational efficiency; recently, an inherent tradeoff between efficiency and resilience was identified (Brede and de Vries, 2009; Ganin et al., 2017), and with it the realization that maximizing efficiency produces fragile systems prone to cascading failures triggered by local disruptions (Wang et al., 2019). This tradeoff has been identified across diverse critical systems beyond infrastructure and is an unavoidable issue in managing future floods (Woods, 2018).
Past and recent events in the United States demonstrate that attempts to improve infrastructure resilience to floods can prove ineffectual. A classic example of catastrophic infrastructure failures induced by extreme flooding is New Orleans after Hurricane Katrina in 2005, where the low-lying placement of hospital backup generators within the flood zone resulted in the failure of life support systems. With evacuation routes inundated, significant loss of life resulted. In response, the US federal government passed the Post-Katrina Emergency Management Reform Act of 2006 which led to a restructuring of the US Department of Homeland Security (DHS) to support more effective preparedness and response to such storms (United States Congress., 2006). Then, Superstorm Sandy in 2012 further demonstrated that major metropolitan areas like New York City, NY were vulnerable to flooding. In response to Superstorm Sandy, the US federal government adopted new critical infrastructure security and resilience policies that guided federal, state, local, and tribal decision-makers in the upgrade and protection of infrastructure systems (The White House, 2013a,b). Despite these efforts, in New York City in September 2023, two storm systems combined to drop more than 8′′ of rain with some rainfall rates exceeding 3′′ per hour (see Figure 1). With the city's sewer system designed to handle a maximum of 1.75′′ per hour (Office of the New York City Comptroller., 2024), massive flooding resulted. Rail, road, and air transportation systems were disrupted, and more than 150 schools flooded. Total damage was estimated at millions USD. Recent updates to US federal policies with National Security Memorandum 22 now explicitly consider interdependencies across infrastructure systems but do little to address tradeoffs in adaptation decisions (The White House., 2024) (e.g., fail-safe vs. safe-to-fail).
Figure 1. A Brooklyn resident attempts to clear a storm drain on Albemarle Road in Flatbush, New York, during flash flooding on September 29, 2023. Record rainfall overwhelmed the city's sewer system leading to widespread floods. Credit: Wil540, licensed under the Creative Commons Attribution-Share Alike 4.0 International license.
Difficulties involved in adapting our critical infrastructure systems to a changing climate can be broadly categorized into three divisions: uncertainty in climate and weather projections; uncertainty in modeling infrastructure impacts; and the lasting effects of a design paradigm in which efficiency is prioritized above all else, leading to highly fragile systems with understudied interdependencies and modes of failure. The articles in this special issue each tackle one aspect of infrastructure resilience to extreme flooding.
Raub et al. explores resilience on a conceptual level, combining aspects of two related paradigms—resilience and the food-energy-water nexus—to improve the formulation, and thus solvability, of problems encountered in infrastructure resilience. The authors argue that this underexplored integration of two complementary approaches to managing flood risk provides a theoretical foundation capable of addressing three key challenges in complex systems: coordination, scale, and heterogeneity. Furthermore, the authors offer examples of existing resilience plans and how to improve upon them to tackle these three challenges. In Miami, it's suggested that points of failure with the potential to cascade across sectors can be removed, mitigating the severity of failures and increasing the efficacy of post-flood recovery. To reduce the impact of post-disaster supply chain failures in urban areas, recommendations are made to increase the local sourcing of food, in accordance with a safe-to-fail design paradigm.
Bartuska and Beighley assess the ability of multi-satellite data products to capture rainfall events across North Carolina, using as ground truth observations from stream gauges across the state over a twenty-year period. Their findings show relatively high performance when evaluating the multi-satellite dataset in terms of error rate, false positive rate, and detection probability. This suggests its suitability for use in rapid-response applications such as water supply monitoring and flood warning systems.
Richardson and Beighley develop and validate methods for improved flood warning systems and rapid flood mapping with a reduced rate of false negatives. The authors use river discharges in combination with Height Above Nearest Drainage (HAND) metrics to develop a rapid flood mapping technique. Comparing their results to FEMA flood maps showed relatively high agreement, with HAND maps tending to overestimate flood extents in non-agreement locations. This represents an improvement: a higher false negative rate may lead to underinvestment in flood adaptation measures, leading to unanticipated system disruptions, while the consequences of a higher false positive rate are limited to inefficient resource allocation due to overinvestment in unnecessary adaptation measures.
Friedland et al. establishes a framework for assessing the vulnerability of industrial process facilities to flood damage from tropical storms, using petrochemical infrastructure in the Gulf of Mexico as a case study. Arguing that existing qualitative assessments are insufficient for identifying vulnerabilities, the authors propose a quantitative framework for assessing susceptibility to floodwater damage relying on a component-level methodology. The authors use this to demonstrate how industry-standard qualitative loss evaluations can be translated to quantitative, granular assessments of potential loss, providing a bridge necessary for robust risk characterization and resource allocation for mitigating the potential impacts of flooding. Such an assessment framework makes anticipative disaster mitigation strategies possible, achieving resilience by increasing facilities' failure tolerance. By modeling a range of failure modes cascading failures can be identified and addressed, recognizing the inevitability of disruption impacts and redesigning systems to be safe-to-fail.
These articles demonstrate the complementary aspects needed to build resilience: a forward-thinking, rigorous conceptual framing; identification and characterization of threats considering uncertainty; and quantification of resilience in engineered systems to such a threat space. On their own, necessary but insufficient; together, a powerful toolset for adapting to a changing world.
Many challenges remain. Developing and testing protective infrastructures like dams and levees, drainage networks, and nature-inspired solutions must continue. Impacted infrastructures—the critical lifelines we rely upon—need to be retrofitted and reconfigured to increase adaptive capacity to avoid the worst consequences of extreme weather and flooding. Implications need to be conceptualized in an end-to-end lifecycle framing from infrastructure design to retrofitting, maintenance, and operations, and ultimately to restoration and salvage. Finally, and perhaps the greatest challenge, is to account for and constrain an uncertain future; any lasting solutions will need to consider socioeconomic, climatological, epistemic, and geopolitical uncertainties alongside the possibility of fundamental surprises. Comprehensive, lasting solutions need to cut across disciplines and involve collaboration between academia and public and private sectors to translate research into flexible adaptation.
JW: Writing – original draft, Writing – review & editing. DE: Writing – review & editing. RA: Writing – review & editing. UB: Writing – review & editing. SC: Writing – review & editing. MG: Writing – review & editing. AM: Writing – review & editing. AP: Writing – review & editing. NY: Writing – review & editing. AG: Writing – review & editing.
The author(s) declare financial support was received for the research, authorship, and/or publication of this article. Partial support was provided by the US DoD SERDP grant RC20-1183 titled Networked Infrastructures under Compound Extremes (NICE).
NY was employed by Microsoft (United States).
The remaining authors declare that the research was conducted in the absence of any commercial or financial relationships that could be construed as a potential conflict of interest.
The author(s) declared that they were an editorial board member of Frontiers, at the time of submission. This had no impact on the peer review process and the final decision.
All claims expressed in this article are solely those of the authors and do not necessarily represent those of their affiliated organizations, or those of the publisher, the editors and the reviewers. Any product that may be evaluated in this article, or claim that may be made by its manufacturer, is not guaranteed or endorsed by the publisher.
Ajjur, S. B., and Al-Ghamdi, S. G. (2022). Exploring urban growth–climate change–flood risk nexus in fast growing cities. Sci. Rep. 12:12265. doi: 10.1038/s41598-022-16475-x
Almoghathawi, Y., Barker, K., and Albert, L. A. (2019). Resilience-driven restoration model for interdependent infrastructure networks. Reliab. Eng. Syst. Safety 185, 12–23. doi: 10.1016/j.ress.2018.12.006
Bevacqua, E., Vousdoukas, M. I., Zappa, G., et al. (2020). More meteorological events that drive compound coastal flooding are projected under climate change. Commun. Earth Environm.1:47. doi: 10.1038/s43247-020-00044-z
Brede, M., and de Vries, B. J. (2009). Networks that optimize a trade-off between efficiency and dynamical resilience. Phys. Lett. A 373, 3910–3914. doi: 10.1016/j.physleta.2009.08.049
Eisenberg, D. (2021). The need to consider residual risk. Nat. Clim. Chang. 11, 803–804. doi: 10.1038/s41558-021-01129-z
Ganin, A. A., Kitsak, M., Marchese, D., Keisler, J. M., Seager, T., and Linkov, I. (2017). Resilience and efficiency in transportation networks. Sci. Adv. 3:e1701079. doi: 10.1126/sciadv.1701079
Kim, Y., Carvalhaes, T., Helmrich, A., Markolf, S., Hoff, R., Chester, M., et al. (2022). Leveraging SETS resilience capabilities for safe-to-fail infrastructure under climate change. Curr. Opin. Environm. Sustainab. 54:101153. doi: 10.1016/j.cosust.2022.101153
Kim, Y., Chester, M. V., Eisenberg, D. A., and Redman, C. L. (2019). The infrastructure trolley problem: Positioning safe-to-fail infrastructure for climate change adaptation. Earth's Future 7, 704–717. doi: 10.1029/2019EF001208
Kim, Y., Eisenberg, D. A., Bondank, E. N., Chester, M. V., Mascaro, G., and Underwood, B. S. (2017). Fail-safe and safe-to-fail adaptation: decision-making for urban flooding under climate change. Clim. Change 145, 397–412. doi: 10.1007/s10584-017-2090-1
Martinkova, M., and Kysely, J. (2020). Overview of observed Clausius-Clapeyron scaling of extreme precipitation in midlatitudes. Atmosphere 11:786. doi: 10.3390/atmos11080786
Office of the New York City Comptroller. (2024). Is New York City Ready for Rain? Available at: https://comptroller.nyc.gov/reports/is-new-york-city-ready-for-rain/#_edn16 (accessed May 23, 2024).
Rogger, M., Agnoletti, M., Alaoui, A., Bathurst, J. C., Bodner, G., Borga, M., et al. (2017). Land use change impacts on floods at the catchment scale: challenges and opportunities for future research. Water Resour. Res. 53, 5209–5219. doi: 10.1002/2017WR020723
Tanoue, M., Taguchi, R., Alifu, H., and Hirabayashi, Y. (2021). Residual flood damage under intensive adaptation. Nat. Clim. Chang. 11, 823–826. doi: 10.1038/s41558-021-01158-8
The White House (2013a). Presidential Policy Directive/PPD-21: Critical Infrastructure Security and Resilience. Washington, DC: The White House Office of the Press Secretary.
The White House (2013b). Executive order 13636: improving critical infrastructure cybersecurity. Fed. Regist. 78, 11739–11743.
The White House. (2024). National Security Memorandum on Critical Infrastructure Security and Resilience. Available at: https://www.whitehouse.gov/briefing-room/presidential-actions/2024/04/30/national-security-memorandum-on-critical-infrastructure-security-and-resilience/ (accessed May 24, 2024).
United States Congress. (2006). Post-Katrina Emergency Management Reform Act of 2006. Public Law 109–295.
Wang, W., Yang, S., Stanley, H. E., and Gao, J. (2019). Local floods induce large-scale abrupt failures of road networks. Nat. Commun. 10, 2114. doi: 10.1038/s41467-019-10063-w
Woods, D. D. (2015). Four concepts for resilience and the implications for the future of resilience engineering. Reliab. Eng. Syst. Safety 141, 5–9. doi: 10.1016/j.ress.2015.03.018
Keywords: flooding, infrastructure, lifelines, design, operations
Citation: Watson JR, Eisenberg DA, Anderson R, Bhatia U, Chatterjee S, Gonzalez MC, Mishra A, Pal AK, Yadav N and Ganguly AR (2024) Editorial: Designing, operating, and rebuilding infrastructures and lifelines for resilience to extreme flooding. Front. Water 6:1438086. doi: 10.3389/frwa.2024.1438086
Received: 25 May 2024; Accepted: 26 July 2024;
Published: 09 September 2024.
Edited and reviewed by: Hiba Baroud, Vanderbilt University, United States
Copyright © 2024 Watson, Eisenberg, Anderson, Bhatia, Chatterjee, Gonzalez, Mishra, Pal, Yadav and Ganguly. This is an open-access article distributed under the terms of the Creative Commons Attribution License (CC BY). The use, distribution or reproduction in other forums is permitted, provided the original author(s) and the copyright owner(s) are credited and that the original publication in this journal is cited, in accordance with accepted academic practice. No use, distribution or reproduction is permitted which does not comply with these terms.
*Correspondence: Jack R. Watson, d2F0c29uLmphY0Bub3J0aGVhc3Rlcm4uZWR1
Disclaimer: All claims expressed in this article are solely those of the authors and do not necessarily represent those of their affiliated organizations, or those of the publisher, the editors and the reviewers. Any product that may be evaluated in this article or claim that may be made by its manufacturer is not guaranteed or endorsed by the publisher.
Research integrity at Frontiers
Learn more about the work of our research integrity team to safeguard the quality of each article we publish.