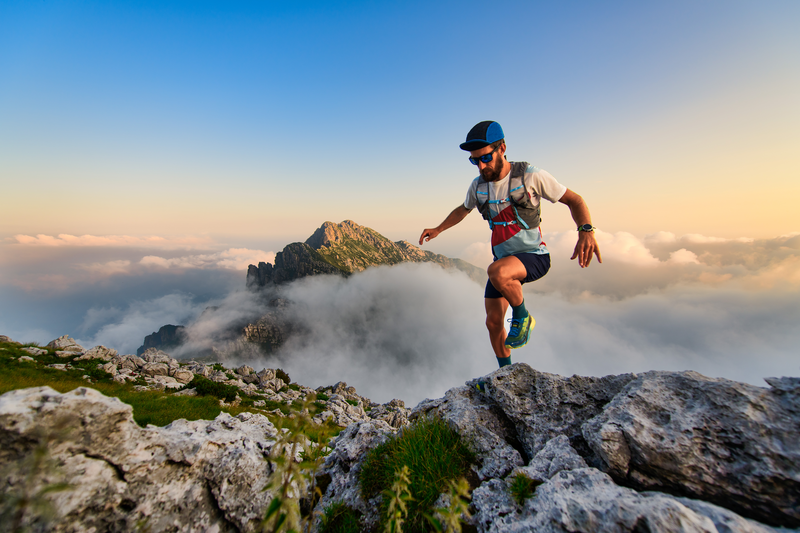
95% of researchers rate our articles as excellent or good
Learn more about the work of our research integrity team to safeguard the quality of each article we publish.
Find out more
ORIGINAL RESEARCH article
Front. Water , 30 July 2024
Sec. Water Resource Management
Volume 6 - 2024 | https://doi.org/10.3389/frwa.2024.1436169
This article is part of the Research Topic Advancements and Challenges in Sustainable Water Desalination Technologies View all 3 articles
Water scarcity is a global challenge, underscoring the importance of efficient water resource management. Solar stills offer a cost-effective method to convert brackish water into potable water but face productivity limitations. This study aims to enhance solar still productivity through modifications using different fin materials and water depth. Computational Fluid Dynamics (CFD) simulations were employed to evaluate thermal performance across four scenarios: copper and aluminum fins at water depths of 20 mm and 40 mm. Key parameters including temperature distributions, friction volume, and fluid velocity were analyzed for each configuration (MSS-I to MSS-IV). Energy and exergy efficiencies were also assessed. MSS-III, utilizing copper fins at a 20 mm depth, demonstrated the highest daily productivity (8.33 liters) compared to MSS-IV (8.02 liters), MSS-I (7.81 liters), and MSS-II (6.71 liters). Energy efficiencies were highest for MSS-III (60.10%), followed by MSS-IV (57.41%), MSS-I (55.22%), and MSS-II (52.18%). MSS-III also exhibited the highest exergy efficiency (21.50%), with MSS-I (17.15%), MSS-IV (16.43%), and MSS-II (14.12%) following. The study underscores significant improvements in thermal and energy efficiency achieved through specific design modifications of solar stills. MSS-III’s higher performance, attributed to the use of copper fins and optimized depth, highlights the critical role of material selection and structural design in enhancing solar still productivity. These findings have important implications for sustainable water resource management, emphasizing the potential of optimized solar still designs to address water scarcity challenges.
Clean water is necessary for human life, yet its availability is decreasing due to overexploitation and water pollution. Population growth and industrial expansion have increased water demand, worsening the scarcity of clean drinking water. Most people rely on polluted sources like rivers, lakes, and underground reservoirs, leading to waterborne diseases. Addressing this issue by increasing the availability of clean water is a critical and urgent need for society (Kumar et al., 2021; Vaithilingam et al., 2022). Freshwater scarcity must be addressed by desalination, but existing techniques need a lot of energy and non-renewable resources. Recent research indicates that the lifespan of petroleum resources may be extended, underscoring the necessity for innovative desalination technology. Reducing greenhouse gas emissions and ensuring secure water supply for the future may be achieved by increasing efficiency and utilizing renewable energy (Shelare et al., 2023). Freshwater is available in wetlands, lakes, glaciers, ponds, reservoirs, streams, and rivers. Desalination techniques are practical in numerous countries because they may use an infinite amount of brackish and seawater from subterranean sources. Various water sources are depicted in Figure 1.
Figure 1. Different sources of water (Tony and Nabwey, 2024).
It’s critical to understand the difference between brine and brackish water. Brine is defined as very salted water that resembles saltwater and is made up of highly concentrated salt solutions, mostly sodium chloride. Natural brines, which serve as storage spaces for vital salts like magnesium and potassium chlorides and sulfates, are typically found underground, in saline lakes, or as saltwater (Marghade et al., 2023). Table 1 shows the particular ions found in water in the ground, brackish water, and ocean. When we talk about brine, we are talking about water that has more total dissolved solids (TDS) than seawater (35,000 mg/L) or brackish water (less than 35,000 mg/L). Total dissolved solids (TDS) in seawater typically range from 15,000 mg/L to over 40,000 mg/L. TDS levels in brackish water commonly range from 1,000 to 15,000 mg/L, and TDS levels in ground water typically range from 500 to 1,000 mg/L. However, in some reservoirs, such as geothermal ones, the levels of salt can be higher, varying from 500 mg/L to 120,000 mg/L (Yenkie et al., 2022). The WHO advises that people consume water with fewer than 600 mg/L salt for tasting purposes, while there are no health-based guidelines for total dissolved solids (Dimri et al., 2008).
Table 1. Ion concentrations of different type of water (Dimri et al., 2008; Marghade et al., 2023).
Desalination methods for purifying saline water are often divided into two types: evaporative or thermal desalination and membrane desalination. Membrane method dominate current desalination processes, accounting for 69–73% of all systems installed worldwide, whereas thermal techniques account for just approximately 27%.Thermal desalination systems employ external heat to turn saltwater water into drinking water by cyclic evaporation and condensation. The most common kinds consist of multiple effect distillation (MED), multi-stage flash (MSF) desalination, thermal vapor compression (TVC), and mechanical vapor compression (MVC) (Ashour et al., 2014; Tony, 2022). Membrane desalination, on the other hand, employs permeable membranes to extract salts from saline water without causing phase change. This pressure-driven process is referred to as reverse osmosis (RO) or electrodialysis (Abed et al., 2021; Tony and Nabwey, 2024). Figure 2 highlights prominent desalination processes, including reverse osmosis (RO), multi-stage flash distillation (MSF), and maybe electrodialysis or solar desalination approaches. RO accounts for 53% of global desalination capacity, followed by MSF (25%), multiple effect distillation (8%), and electrodialysis (3%). The rest, or 11% include a variety of different methods (Shelare et al., 2023).
Figure 2. Classification of various desalination technologies (Tony and Nabwey, 2024).
Although heat or mechanical/electrical energy is used in both thermal and membrane desalination processes to extract salt from water, both processes are known for consuming a lot of energy (Kabeel, 2009; Tony and Nabwey, 2024). Membrane desalination uses membranes and high-pressure zones power units to distinguish absorbed water from salty water, which can be brackish or saltwater, whereas thermal desalination employs heat to evaporate contaminated water (Jathar et al., 2022b). Table 2 summarizes the important resource requirements for thermal and membrane desalination systems.
Table 2. Performance metrics of desalination methods (Yenkie et al., 2022).
Thermal desalination needs both heat and electrical power, whereas membrane desalination only uses electricity. RO technique typically uses 3.5–5 kWh/m3 of electricity. Recent developments in membrane technology have rendered membranes more capable of replacing thermal processes in desalination facilities (Singh and Tiwari, 2004). In Figure 3, the volume of water produced by different methods depicts the comparative efficiencies of other water production technologies.
Figure 3. Volume of water production of various desalination methods (Singh et al., 1996).
Reverse osmosis (RO), for instance, dominates the desalination market due to its high efficiency and scalability. Multi-effect distillation (MED) and membrane distillation (MD) technologies offer advantages in energy efficiency and operation under varying feedwater conditions. However, these technologies often require substantial energy inputs and face challenges related to brine disposal and environmental impact, which is not worth. Renewable energy enables long-term substitutes to fossil fuels by generating clean electricity through natural processes such as solar, wind, geothermal, marine, hydro, and bioenergy (Harijan, 2008). Photovoltaic, wind, hydropower, and bioenergy are estimated to account for 46% of electricity consumption by 2030. By then, hydropower (17%), wind energy (14%), photovoltaic solar (7%), and bioenergy (5%) will meet electricity demand. Wind, solar, waves, tidal, and geothermal energy are examples of renewable energy sources used for desalination. Figure 4 depicts the use of renewable energy resources in desalination processes to produce freshwater: solar thermal at 27%, solar PV at 43%, wind at 20%, and hybrid systems at 10% (Shelare et al., 2023).
Figure 4. The contribution of renewable energy resources in desalination (Shelare et al., 2023).
Solar energy is the predominant renewable energy source for desalination. For decades, many types of solar distillers have been widely used to desalinate water. The technology operates on a minimal natural cycle of water. Solar distillers are inexpensive to produce and operate, however they have a small amount of freshwater production. Solar distillers are praised for their poor efficiency and output capacity. Traditional solar desalination devices work best when the pond’s evaporation rate is relatively small and the glass surface has not enough condensation (Abed et al., 2022). The efficiency of solar distillers is determined by ambient conditions, device design, and operational parameters. Solar distillation output is determined by elements such as sunshine intensity, ambient conditions (temperature and humidity), wind speed, cloud cover, and glass condensation. Evaporation rates influence solar distiller efficiency directly, but basin depth has an inverse effect. Optimizing the angle of the glass cover optimizes performance; a steeper angle is encouraged in wintertime and a deeper angle in summertime for best sunshine collecting (Katekar and Deshmukh, 2020). Cost-effectiveness is critical for developing desalination plants, with the optimal approach selected based on technical and economic variables such as water conditions, distant accessibility, grid connectivity, infrastructure, and solar energy technology type. Present attempts are aimed at increasing the efficiency of solar energy systems and desalination techniques in order to affordably support medium and small-scale desalination facilities. These constraints have prompted extensive study and innovation in recent years to increase performance and investigate alternate desalination processes (Shelare et al., 2023), this study investigated nanofluids in solar energy, concentrating on advances. The study found that nanotechnology has promise for raising solar desalination efficiency by increasing evaporation rates on absorber or water surfaces. Increased nanoparticle content enhances heat transfer. Nanofluids also improve solar energy capture in direct absorption collectors due to their excellent thermal absorption capabilities (Chaudhari et al., 2024). Implementing machine learning algorithms, the researchers compared solar cooking to indoor cooking tools. It concludes that continual tracking of temperatures is critical as machine learning has the potential to transform solar cooking by boosting renewable energy and sustainable living (Pawar et al., 2024), this research attempted using machine learning approaches to better comprehend the complex relationships among nanoparticle characteristics and engine operation. The study finds that nanoparticles have a substantial influence on both the efficiency and emissions of biodiesel engines, resulting in an improvement of more sustainable and effective engine designs (Soudagar et al., 2024), this research evaluated hybrid wind-solar energy systems; highlighting the advantages of optimal designs with sophisticated control mechanisms. It analyzed current breakthroughs in PV-solar and wind-based hybrid systems and investigated future improvements, underlining the worldwide move toward environmentally friendly and resistant energy solutions (Gajbhiye et al., 2022), this research assessed advances in desalination nanotechnology and found that nanotechnology has tremendous potential for enhancing its efficiency and sustainability. Integrating nanofluids with both active and passive technologies can improve solar desalination output, allowing for more environmentally friendly procedures and efficient application of solar thermal energy (Belkhode et al., 2021), this study determined the effectiveness of several roof collector materials in a solar updraft tower. Increasing the chimney height by 33.33% (from a range 3.6 to 4.8 m) increased output power by 37.92% for glass, 36.13% for acrylic, 16.78% for crystalline, and 32.18% for polycarbonate sheet collectors. Furthermore, utilizing glass as the roof collector material increased the system’s output power by 5 to 15% over acrylic, crystalline, and polycarbonate sheets. The principles of the first law of thermodynamics drive energy analysis, providing insights on energy storage and energy transmission properties (Park et al., 2014; Sivakumar et al., 2016; Jathar and Ganesan, 2020; Kumar et al., 2022), emphasized their experimental study to enhance the nighttime production of a concave-type stepped solar still constructed from locally sourced materials such as bricks, sand, and concrete. They employed statistical tools to investigate the various weight distributions of these components and developed a linear regression model to evaluate their effect. The results showed that adding 12 kg of concrete pieces resulted in a maximum nighttime production of 435 mL and a significant distillate output of 4.06 L/day. Bricks (19.33%), sand (21.8%), and concrete fragments (25.30%) all showed considerable efficiency increases. Also, the modified solar still with 12 kg of concrete pieces had a relatively modest return on investment of 111 days, indicating its economic viability and practical use in water-scarce regions. Jathar et al. (2021), investigated and numerically analyzed a concave-type stepped solar still under various environmental circumstances. They showed that the model created using the normal values from March was very effective, with an R-square value of 0.9944, making it adequate for assessing efficiency. In March 2020, the greatest day yield recorded was 3.7 liters per square meter. This was due to the highest average radiation intensity of 1,005 W/m2 and the largest average temperature differential of 10.5°C. Jathar et al. (2022a), the concave-type stepped solar still (CTSSS) was tried in Pune with the objective of taking into account the local environment. Improved performance by using varied water depths and 0.2% quantities of MgO, Al2O3, and TiO2 nanoparticles. MgO (41.35%), Al2O3 (28.42%), and TiO2 nanofluids all resulted in significant productivity gains. The data show a negative association between water depth and productivity. Specifically, the 0.2% MgO nanofluid at 7 mm water depth produced 16.66% higher production than at 15 mm. Kabeel et al. (2019) investigated solar stills (SS) equipped with reflectors and a mixture of black gravel and PCM in their studies. Two separate sets of tests were carried out: one with SS using composite materials and the other with a solar still using PCM alone. The solar stills energy and energy efficiency increased with the use of composite materials. In comparison to employing PCM alone, the energy and energy efficiencies for the composite black gravel material were found to be 38 and 37% higher, respectively (Jathar et al., 2021). A water cooling film structure and nanoparticle application was evaluated in combination using a concave-style stepped solar still. The statistics show that adding 0.2% concentrations of MgO, Al2O3, and TiO2 nanofluids, as well as the water cooling arrangement, increased productivity by 51.28, 39.24, and 25.37%, respectively (Jathar and Ganesan, 2022). The heat-storage substances have been investigated and the results revealed that 12 kg of concrete fragments produced 4,062 mL of distillate every day, including 435 mL at night. Concrete bits outperformed brick and sand pieces in terms of heat storage by 11.16 and 7.37%, respectively. Concrete chunks had the highest overall efficiency at 28.27%. Recent advances in solar still technology have centered on improving efficiency with innovative materials and design changes. For example, the incorporation of advanced nanomaterials has shown the global application of renewable-powered desalination. For example, using advanced nanomaterials has shown promise in speeding the evaporation-condensation cycle and thereby improving freshwater yields. Integrating renewable energy into desalination operations has enormous promise for creating long-term water solutions. Ongoing research and development activities aim to increase efficiency, lower prices, and broaden the use of renewable-powered desalination systems across the world. An extensive examination of current research shows a lack of CFD studies that explore the impact of fins, especially those made of different materials, on the thermal dynamics and efficiency of solar stills. This study seeks to improve the efficiency of solar stills by altering traditional designs with fins made of various materials. The study uses CFD simulations to evaluate thermal performance in four different scenarios, which include the use of copper and aluminum fins at water depths of 20 mm and 40 mm.
A 3D model of a solar still was created using ANSYS Fluent, with dimensions specified in Table 3. The design includes various features aimed at improving efficiency and performance. The basin and sidewalls are made of 1.5 mm-thick galvanized iron sheet, which has a thermal conductivity of 72 W/m.K. To reduce heat loss, the external sides are coated with 10 mm-thick glazing wool, known for its low thermal conductivity of 0.032 W/m.K. The interior basin surface is designed to absorb 80% of solar radiation. The solar still has a base size of 1.1 m x 1.1 m, with a front wall height of 0.25 m and a back wall height of 0.9 m. It includes a 4 mm-thick glass sheet angled at 29 degrees for optimal condensation efficiency. Additionally, the design incorporates 10 square-shaped plates with aluminum and copper fins. The aluminum fins have a thermal conductivity of 205.0 W/m.K. with a 20 mm water depth, while the copper fins have 385 W/m.K. with a 40 mm water depth. The fins are strategically placed at a height of 40 mm to maximize solar radiation absorption and increase the evaporation surface area.
Different Equations 1–7 are applied to calculate energy, mass, and continuity in various phases of the system. Figure 5 demonstrates the impact of incoming solar radiation, heat loss, and water evaporation on the overall energy balance and efficiency of the solar still. This thorough examination aids in enhancing the design and performance. The CFD simulation model was developed based on the following assumptions.
• The sole internal thermal energy source in the solar still is solar radiation that enters via the glass cover.
• It is assumed that the temperature on the solar wall is steady and unchanging.
• The wind velocity was negligible due to the low ambient wind velocity.
• No vapor leaks are emanating from the last unit.
Energy equation
where keff is the effective conductivity.
Continuity equation
= Momentum equation
Conservative volume equation
Volume fractions add up to unity
Mass transfer equation
The energy for phase p and q is:
, are the enthalpies of species i, j of phase q & is the enthalpy of species i of phase
To assess the efficiency of solar stills, an analysis of the energy and exergy efficiency is necessary and it also essential for determining whether solar stills are economically feasible.
The energy efficiency of solar still is assessed using Equations 8, 9;
The exergy efficiency of solar still is assessed using Equations 10–12 (Shoeibi et al., 2022).
The exergy input of a solar still
The exergy generation of the solar still is;
In this study, ANSYS Fluent software is utilized to perform a computational fluid dynamics (CFD) analysis on solar stills using the evolving substance method. The research focuses on studying fluid flow and heat transfer within the system by applying fundamental equations like the continuity equation, conservation of momentum, and governing equations. CFD relies on mathematical algorithms designed to address fluid flow-related issues. These computational fluid dynamics programs offer advanced user interfaces that simplify inputting boundary conditions and sequentially analyzing results, providing immediate access to the solution’s capabilities. Three crucial factors need to be considered. ANSYS fluent software is employed as the CFD tool to simulate all components of the solar still. The initial step in using CFD to examine any problem involves constructing a geometric model based on design specifications. The domain of interest in this case includes the area enclosed by the saltwater surface of the still basin, the side walls, front and rear boundaries, and the transparent cover of the still. The traditional basin-type solar still was modeled in 3D using ANSYS Workbench, with the design modeler used to create geometric models corresponding to the physical domain. The cut-cell meshing technique was deemed most suitable due to the absence of curved surfaces in the solar still shape. This approach, supported by ANSYS Fluent Workbench meshing, produced accurate results within a reasonable computational timeframe. The mesh size was validated using a grid independence test, ensuring convergence conditions for continuity, velocity, k-epsilon (1e-3), and energy (1e-6) were met at each time step. The grid size was determined by gradually increasing the number of meshes until the required condition [p − (p + 1)]/p < 10–3 was satisfied, as shown in Figure 6A. The computed temperature with the current mesh size is represented by “P.” The meshed domain consisted of 1,335,561 elements and 1,535,460 nodes, considered adequate given the complexity of the task at hand. Which is shown in Figure 6B.
The study defined precise boundary conditions to solve continuity and momentum equations. The simulation lasted 10 h due to numerous time steps and computational limits. Solar radiation, ambient temperature, and water and glass temperatures were determined using a solar calculator in clear weather. Solar intensity was computed based on glass, basin, and water properties. Side-wall heat transfer remained constant for consistency. This method ensured an accurate analysis of heat transfer and fluid dynamics. Boundary conditions and parameters are detailed in Tables 4, 5. Time steps ranged from 0.001 to 1.0 s for optimal convergence and completion.
Simulations were performed using the CFD Fluent solver within the ANSYS for four configurations of modified solar stills (MSS). The simulations included four different configurations as;
i. Aluminum-finned plates at a depth of 20 mm,
ii. Aluminum-finned plates at a depth of 40 mm,
iii. Copper-finned plates at a depth of 20 mm,
iv. Copper-finned plates at a depth of 40 mm.
In a solar still, the importance of temperature control for effective water distillation. Figure 7, which shows temperature distributions on absorber plates of different solar still configurations, uses color coding to indicate variations from low (blue) to high (red) temperatures in degrees Celsius. The study focuses on how the choice of fin material (copper or aluminum) and water depth (20 mm or 40 mm) affect temperature distribution, emphasizing that fins enhance heat transfer, leading to higher temperatures near the absorber plate. Table 6 quantitatively compares the additional conduction surface area provided by each modified Solar Still (MSS) configuration, revealing that MSS-III shows the highest increase in heat transfer efficiency (38.06%), followed by MSS-IV (32.38%), MSS-II (28.37%), and MSS-I (23%). This data underscores the role of fin materials and water depths in optimizing heat absorption and improving overall solar still performance in water distillation.
Figure 8 illustrates the velocity contours of the air and water vapor mixture in the solar still basin. These contours, shown in the X-Y plane passing through the center and parallel to the sidewalls, highlight recirculation zones with varying velocity magnitudes. The maximum velocity recorded is 1.24 m/s in the basin of MSS-III. This distribution of velocities is critical for understanding how fluid dynamics influences heat transfer efficiency and the overall performance of solar stills.
The water friction study examines how different fin materials and water depths affect solar still productivity. Aluminum and copper fins, combined with varying water depths, enhance the efficiency of solar stills. Figure 9 shows the contours of water condensation distribution on the glass cover, illustrating these effects.
Hourly productivity was checked for each solar still, as shown in Figure 10. MSS-III demonstrated 17.65% higher water productivity than MSS-II, due to the increased solar intensity utilized by MSS-III. This improvement is due to the fin material attached to the absorber plate in the solar basin, which enhances solar energy absorption and optimizes productivity by increasing the basin temperature. However, water productivity decreased with greater water depth, as seen in MSS-IV, where productivity dropped by 3.68% when the water depth increased from 20 mm (MSS-III) to 40 mm (MSS-IV). This decline is attributed to the reduced temperature difference between the interior glass and the basin water. Among the tested cases, MSS-III achieved the highest productivity at 8.33 liters per day, followed by MSS-IV with 8.02 liters, MSS-I with 7.81 liters, and MSS-II with 6.71 liters per day. The use of fin material in the desalination process and the reduction in basin depth contributed to the increased productivity. Productivity was directly correlated with solar radiation, peaking at 1065.6 mL/h at noon. However, as solar radiation decreased, the energy available to heat the basin diminished, leading to a decrease in water productivity.
The thermal efficiency for each solar still is illustrated in Figure 11. The highest efficiency at peak temperature was recorded as 60.10, 57.41, 55.22, and 52.18% for MSS-III, MSS-IV, MSS-I, and MSS-II, respectively. Utilizing copper fins at a depth of 20 mm led to a 9.53% increase in efficiency. Additionally, reducing the depth of the basin from 40 mm to 20 mm resulted in a 5.19% increase in thermal efficiency. The improvement observed is attributed to the enhanced temperature difference between the water and the glass inside the basin, facilitated by the material used, which aids in absorbing more solar radiation. As a result, the optimal configuration was determined to be the one with a basin depth of 20 mm and a copper-fin solar still. Similarly, energy efficiency was computed to determine the amount of useful energy accessible to the system for specific applications like solar stills. The maximum exergy efficiency achieved was 21.50% for MSS-III, followed by MSS-I (17.15%), MSS-IV (16.43%), and MSS-II (14.12%), as depicted in Figure 5. Reducing the depth from 40 mm to 20 mm resulted in a significant 34.12% increase in energy efficiency, while incorporating aluminum and copper-finned materials led to a notable 27.26% rise in efficiency. These modifications to the design parameters brought about significant improvements in exercise efficiency. The alteration of fin materials induced substantial temperature changes, consequently enhancing the production of fresh potable water. Additionally, a reduction in the height of the basin led to enhanced exergy efficiency, a finding consistent with the study conducted by (Khafaji et al., 2022). In the current study, MSS-III was determined to be the optimal system. This configuration demonstrated performance improvements of 26.25, 50.50, and 33.12% compared to cases MSS-I, MSS-II, and MSS-IV, respectively.
A summary of the comparison of thermal efficiencies (%) achieved by different solar still configurations from various studies, including the present study, is compared in Table 7.
This comparison shows the thermal efficiencies achieved by different solar still designs as reported in various studies, culminating in the findings from the present study with copper fins at a 20-mm depth achieving the highest efficiency of 60.10%.
This paper presents a three-dimensional, multi-phase CFD model for a finned-type solar still. Integrating fins into the basin increases clean water production, while the fin material improves thermal conductivity. The findings reveal substantial improvements in both thermal and energy efficiency of solar stills achieved through particular design changes. MSS-III demonstrated the highest thermal efficiency at 60.10%, which represents a 9.53% increase attributed to copper fins placed at a 20 mm depth and a 5.19% enhancement by decreasing the basin depth from 40 mm to 20 mm. Similarly, the energy efficiency results further support the effectiveness of these design modifications. MSS-III achieved the highest exercise efficiency at 21.50%, with significant increases attributed to the reduced basin depth and the use of finned materials. The 34.12% increase in energy efficiency with a 20-mm basin depth and the 27.26% rise with copper and aluminum fins highlight the substantial impact of these changes. These results are consistent with current literature, which underscores the significance of fin materials and basin depth in boosting solar still performance. These findings are consistent with existing literature, which supports the use of advanced materials and optimized design parameters to improve solar still performance. The increase in efficiency is primarily due to better heat absorption and transfer, resulting in higher freshwater production rates. The implications of these results are profound for the design and operation of solar stills. Higher thermal and exergy efficiencies translate to more effective and sustainable freshwater production, which is critical in areas with limited water resources. This research contributes to the advancement of solar-driven water desalination technologies by providing insights into optimized design configurations. The findings are expected to inform future developments aimed at enhancing the energy efficiency and water production capabilities of solar stills.
Future research should focus on several key areas identified in this study. Firstly, exploring the long-term durability and cost-effectiveness of using different fin materials, such as aluminum and composite materials, could provide insights into practical implementation. Secondly, investigating the integration of phase change materials (PCMs) and nano porous structures on fin profiles holds promise for further enhancing thermal efficiency and daily water production. Additionally, expanding the glass area and studying the incorporation of floating materials with fins are avenues worth exploring to maximize solar energy absorption and improve overall efficiency. Moreover, leveraging advanced techniques such as computational fluid dynamics simulations and machine learning approaches could enable more precise predictions of solar still performance under varying environmental conditions. These methodologies can enhance the design optimization process and contribute to the scalability and reliability of solar still technology in addressing global water scarcity challenges.
The original contributions presented in the study are included in the article/supplementary material, further inquiries can be directed to the corresponding author/s.
RK: Conceptualization, Data curation, Formal analysis, Investigation, Methodology, Resources, Software, Validation, Visualization, Writing – original draft. LK: Conceptualization, Methodology, Project administration, Supervision, Validation, Visualization, Writing – original draft. NM: Investigation, Methodology, Resources, Supervision, Validation, Visualization, Writing – review & editing. KH: Formal analysis, Investigation, Project administration, Supervision, Validation, Visualization, Writing – review & editing.
The author(s) declare that no financial support was received for the research, authorship, and/or publication of this article.
The authors declare that the research was conducted in the absence of any commercial or financial relationships that could be construed as a potential conflict of interest.
All claims expressed in this article are solely those of the authors and do not necessarily represent those of their affiliated organizations, or those of the publisher, the editors and the reviewers. Any product that may be evaluated in this article, or claim that may be made by its manufacturer, is not guaranteed or endorsed by the publisher.
Abed, F. M., Ahmed, A. H., Hasanuzzaman, M., Kumar, L., and Hamaad, N. M. (2022). Experimental investigation on the effect of using chemical dyes on the performance of single-slope passive solar still. Sol. Energy 233, 71–83. doi: 10.1016/j.solener.2021.12.060
Abed, F. M., Zaidan, M. H., Hasanuzzaman, M., Kumar, L., and Jasim, A. K. (2021). Modelling and experimental performance investigation of a transpired solar collector and underground heat exchanger assisted hybrid evaporative cooling system. J. Build. Eng. 44:102620. doi: 10.1016/j.jobe.2021.102620
Agrawal, A., and Rana, R. (2019). Theoretical and experimental performance evaluation of single-slope single-basin solar still with multiple V-shaped floating wicks. Heliyon 5:e01525. doi: 10.1016/j.heliyon.2019.e01525
Ashour, A., Tony, M. A., and Purcell, P. J. (2014). Use of agriculture-based waste for basic dye sorption from aqueous solution: kinetics and isotherm studies. Am. J. Chem. Eng. 2, 92–98. doi: 10.11648/j.ajche.20140206.14
Belkhode, P. N., Shelare, S. D., Sakhale, C. N., Kumar, R., Shanmugan, S., Soudagar, M. E. M., et al. (2021). Performance analysis of roof collector used in the solar updraft tower. Sustain Energy Technol Assess 48:101619. doi: 10.1016/j.seta.2021.101619
Chaudhari, K., Walke, P., and Shelare, S. (2024). Comparative predictive analysis through machine learning in solar cooking technology. Int. J. Electr. Comput. Eng. Syst. 15, 543–552. doi: 10.32985/ijeces.15.6.8
Dimri, V., Sarkar, B., Singh, U., and Tiwari, G. (2008). Effect of condensing cover material on yield of an active solar still: an experimental validation. Desalination 227, 178–189. doi: 10.1016/j.desal.2007.06.024
Gajbhiye, T., Shelare, S., and Aglawe, K. (2022). Current and future challenges of nanomaterials in solar energy desalination systems in last decade. Transdiscip. J. Eng. Sci. 13:217. doi: 10.22545/2022/00217
Harijan, K. (2008). Modelling and Analysis of the Potential Demand for Renewable Resources of Energy in Pakistan.
Jathar, L. D., and Ganesan, S. (2020). Statistical analysis of brick, sand and concrete pieces on the performance of concave type stepped solar still. Int. J. Ambient Energy 1, 1–17. doi: 10.1063/5.0003821
Jathar, L. D., and Ganesan, S. (2022). Assessing the performance of concave type stepped solar still with brick, sand, and concrete pieces. Int. J. Ambient Energy 43, 3468–3484. doi: 10.1080/01430750.2020.1839551
Jathar, L. D., Ganesan, S., and Gorjian, S. (2021). An experimental and statistical investigation of concave-type stepped solar still with diverse climatic parameters. Clean. Eng. Technol. 4:100137. doi: 10.1016/j.clet.2021.100137
Jathar, L. D., Ganesan, S., and Palanimuthu, L. (2022a). Performance assessment of concave-type stepped desalination unit with nanoparticles and varying water depth: an experimental approach. Int. J. Ambient Energy 43, 5041–5054. doi: 10.1080/01430750.2021.1933589
Jathar, L. D., Ganesan, S., Shahapurkar, K., Soudagar, M. E. M., Mujtaba, M., Anqi, A. E., et al. (2022b). Effect of various factors and diverse approaches to enhance the performance of solar stills: a comprehensive review. J. Therm. Anal. Calorim. 147, 4491–4522. doi: 10.1007/s10973-021-10826-y
Kabeel, A. (2009). Performance of solar still with a concave wick evaporation surface. Energy 34, 1504–1509. doi: 10.1016/j.energy.2009.06.050
Kabeel, A., Abdelaziz, G. B., and El-Said, E. M. (2019). Experimental investigation of a solar still with composite material heat storage: energy, exergy and economic analysis. J. Clean. Prod. 231, 21–34. doi: 10.1016/j.jclepro.2019.05.200
Katekar, V. P., and Deshmukh, S. S. (2020). A review on research trends in solar still designs for domestic and industrial applications. J. Clean. Prod. 257:120544. doi: 10.1016/j.jclepro.2020.120544
Kaviti, A. K., Naike, V. R., Ram, A. S., and Thakur, A. K. (2021). Energy and exergy analysis of a truncated and parabolic finned double slope solar stills. Int. J. Ambient Energy 2021, 1–14.
Khafaji, H. Q., Abdul Wahhab, H. A., Al-Maliki, W. A. K., Alobaid, F., and Epple, B. (2022). Energy and exergy analysis for single slope passive solar still with different water depth located in Baghdad center. Appl. Sci. 12:8561. doi: 10.3390/app12178561
Kumar, L., Hasanuzzaman, M., and Rahim, N. (2022). Real-time experimental performance assessment of a photovoltaic thermal system cascaded with flat plate and heat pipe evacuated tube collector. J. Solar Energy Eng. 144:011004. doi: 10.1115/1.4051861
Kumar, P., Shah, S. F., Uqaili, M. A., Kumar, L., and Zafar, R. F. (2021). Forecasting of drought: a case study of water-stressed region of Pakistan. Atmos. 12:1248. doi: 10.3390/atmos12101248
Marghade, D., Mehta, G., Shelare, S., Jadhav, G., and Nikam, K. C. (2023). Arsenic contamination in Indian groundwater: from origin to mitigation approaches for a sustainable future. Water 15:4125. doi: 10.3390/w15234125
Omara, Z., Hamed, M. H., and Kabeel, A. (2011). Performance of finned and corrugated absorbers solar stills under Egyptian conditions. Desalination 277, 281–287. doi: 10.1016/j.desal.2011.04.042
Park, S., Pandey, A., Tyagi, V., and Tyagi, S. (2014). Energy and exergy analysis of typical renewable energy systems. Renew. Sust. Energ. Rev. 30, 105–123. doi: 10.1016/j.rser.2013.09.011
Pawar, C., Shreeprakash, B., Mokshanatha, B., Nikam, K. C., Motgi, N., Jathar, L. D., et al. (2024). Machine learning-based assessment of the influence of nanoparticles on biodiesel engine performance and emissions: a critical review. Arch. Comput. Methods Eng. 2024:10144. doi: 10.1007/s11831-024-10144-0
Rajaseenivasan, T., and Srithar, K. (2016). Performance investigation on solar still with circular and square fins in basin with CO2 mitigation and economic analysis. Desalination 380, 66–74. doi: 10.1016/j.desal.2015.11.025
Shelare, S. D., Aglawe, K. R., Matey, M. S., Shelke, K. S., and Sakhale, C. N. (2023). Preparation, applications, challenges and future prospects of nanofluid materials with a solar systems in the last decade. Mater. Today. Proc. 2023:160. doi: 10.1016/j.matpr.2023.06.160
Shelare, S., Kumar, R., Gajbhiye, T., and Kanchan, S. (2023). Role of geothermal energy in sustainable water desalination—a review on current status, parameters, and challenges. Energies 16:2901. doi: 10.3390/en16062901
Shoeibi, S., Kargarsharifabad, H., Rahbar, N., Khosravi, G., and Sharifpur, M. (2022). An integrated solar desalination with evacuated tube heat pipe solar collector and new wind ventilator external condenser. Sustain Energy Technol Assess 50:101857. doi: 10.1016/j.seta.2021.101857
Singh, S., Bhatnagar, V., and Tiwari, G. (1996). Design parameters for concentrator assisted solar distillation system. Energy Convers. Manag. 37, 247–252. doi: 10.1016/0196-8904(95)00166-B
Singh, H., and Tiwari, G. (2004). Monthly performance of passive and active solar stills for different Indian climatic conditions. Desalination 168, 145–150. doi: 10.1016/j.desal.2004.06.180
Sivakumar, V., Sundaram, E. G., and Sakthivel, M. (2016). Investigation on the effects of heat capacity on the theoretical analysis of single slope passive solar still. Desalin. Water Treat. 57, 9190–9202. doi: 10.1080/19443994.2015.1026284
Soudagar, M. E. M., Ramesh, S., Khan, T. Y., Almakayeel, N., Ramesh, R., Ghazali, N. N. N., et al. (2024). An overview of the existing and future state of the art advancement of hybrid energy systems based on PV-solar and wind. Int. J. Low Carbon Technol. 19, 207–216. doi: 10.1093/ijlct/ctad123
Srivastava, P. K., and Agrawal, S. (2013). Winter and summer performance of single sloped basin type solar still integrated with extended porous fins. Desalination 319, 73–78. doi: 10.1016/j.desal.2013.03.030
Tony, M. A. (2022). Valorization of undervalued aluminum-based waterworks sludge waste for the science of “the 5 Rs’ criteria”. Appl Water Sci 12:20. doi: 10.1007/s13201-021-01554-7
Tony, M. A., and Nabwey, H. A. (2024). Recent advances in solar still technology for solar water desalination. Appl Water Sci 14:147. doi: 10.1007/s13201-024-02188-1
Vaithilingam, S., Muthu, V., Athikesavan, M. M., Afzal, A., and Sathyamurthy, R. (2022). Energy and exergy analysis of conventional acrylic solar still with and without copper fins. Environ. Sci. Pollut. Res. 29, 6194–6204. doi: 10.1007/s11356-021-16124-2
Velmurugan, V., Gopalakrishnan, M., Raghu, R., and Srithar, K. (2008). Single basin solar still with fin for enhancing productivity. Energy Convers. Manag. 49, 2602–2608. doi: 10.1016/j.enconman.2008.05.010
Keywords: CFD simulation, distilled water, solar still, fins, energy, exergy
Citation: Kumar R, Kumar L, Mirjat NH and Harijan K (2024) CFD simulation of modified solar still for effective condensation and evaporation: energy and exergy analysis. Front. Water. 6:1436169. doi: 10.3389/frwa.2024.1436169
Received: 21 May 2024; Accepted: 12 July 2024;
Published: 30 July 2024.
Edited by:
Laxmikant Jathar, Army Institute of Technology, IndiaReviewed by:
Sagar Shelare, Priyadarshini College of Engineering, IndiaCopyright © 2024 Kumar, Kumar, Mirjat and Harijan. This is an open-access article distributed under the terms of the Creative Commons Attribution License (CC BY). The use, distribution or reproduction in other forums is permitted, provided the original author(s) and the copyright owner(s) are credited and that the original publication in this journal is cited, in accordance with accepted academic practice. No use, distribution or reproduction is permitted which does not comply with these terms.
*Correspondence: Khanji Harijan, a2hhbmppLmhhcmlqYW5AZmFjdWx0eS5tdWV0LmVkdS5waw==
Disclaimer: All claims expressed in this article are solely those of the authors and do not necessarily represent those of their affiliated organizations, or those of the publisher, the editors and the reviewers. Any product that may be evaluated in this article or claim that may be made by its manufacturer is not guaranteed or endorsed by the publisher.
Research integrity at Frontiers
Learn more about the work of our research integrity team to safeguard the quality of each article we publish.