- 1Applied Microbiology Laboratory, Center for Rural Development and Technology, Indian Institute of Technology Delhi, New Delhi, India
- 2Department of Biosciences and Bioengineering (BSBE), Indian Institute of Technology Roorkee, Roorkee, India
- 3School of Civil Engineering, University of Sydney, Darlington, NSW, Australia
- 4Department of Chemical and Biological Engineering, Princeton UniversityPrinceton, NJ, United States
- 5Lewis-Sigler Institute of Integrative Genomics and Department of Molecular Biology, Princeton University, Princeton, NJ, United States
The study investigates the feasibility and performance of an indigenously designed algal-bacterial biofilm reactor (ABR) for treating graywater in rural areas. Focusing on both treatment efficacy and sustainability, the research explored two key areas: optimizing the ABR for efficient graywater treatment and evaluating the use of readily available, low-cost materials for its construction. To achieve this, ABR was optimized to handle the specific characteristics of rural graywater, including potential seasonal variations in nutrient concentrations. The optimized ABR’s adaptability was rigorously assessed across various operational modes—batch, fed-batch, and continuous—demonstrating consistent removal efficiencies for key pollutants (COD, nitrate-nitrogen, total dissolved phosphate, and total ammoniacal nitrogen) under changing conditions. The fiber support-based ABR demonstrated significant pollutant removal from rural graywater: 81% COD reduction, 49.5% nitrate-nitrogen (NO3-N), 88.46% total dissolved phosphate (TDP), and 83.42% total ammoniacal nitrogen (TAN) in batch mode. Moreover, there was almost complete removal of total suspended solids (from 142 mg L−1 on Day 1 to 0 mg L−1 on Day 6) and total coliform (39,000 CFU mL−1 to 30 CFU mL−1). Notably, these removal efficiencies remained consistent across fed-batch (and continuous operation modes), showcasing the ABR’s adaptability. This adaptability facilitated a significant increase in treatment capacity, scaling from 3 L treated in batch mode (6 days) to 40 L in fed-batch mode (12 days) and a continuous treatment rate of 20 L per day. Further enhancing the sustainability of this approach, the study successfully employed readily available natural materials like bamboo pipes and sheets for constructing the ABR. This innovative design resulted in a remarkable increase in biomass productivity (9.8 g m−2 day−1) compared to polypropylene fiber-based reactors (4.5 g m−2 day−1) with enhanced treatment potential for all the parameters in batch mode (77.7% COD removal, 86% NO3-N removal, 81% TDP removal, 92% TAN removal, and 100% removal of total coliforms). This is the first reported algae-bacterial biofilm system using bamboo for wastewater treatment, promoting local resource utilization and a bio-based sustainable approach for rural graywater treatment.
Highlights
• Development of an eco-friendly bamboo-based wastewater treatment system for rural areas.
• Multi-mode performance evaluation of algal-bacterial biofilm reactors for graywater treatment.
• More efficient contaminant removal in bamboo-based reactor (77.7% COD, 86% NO3-N, 81% TDP, and 92% TAN).
• 2.4 fold biomass productivity in bamboo-based reactor vs. polypropylene fiber-based reactor.
• Proposed algae farming model for integrated wastewater management and valorization.
1 Introduction
Limited access to freshwater resources for agriculture poses a significant challenge in developing countries with large rural populations. Graywater, originating from domestic activities like laundry, dishwashing, and bathing, cattle washing offers a readily available source for non-potable water needs. However, the direct application of untreated graywater poses several environmental and public health risks. Chemical pollutants, such as salts, phosphates, and surfactants, present in commonly used household detergents can accumulate in soil with prolonged irrigation using untreated graywater (Mousavi and Khodadoost, 2019). This can lead to alterations in soil pH, potentially hindering plant growth and disrupting the delicate balance of soil microbial communities crucial for nutrient cycling and overall soil health (Leonel and Tonetti, 2021). Furthermore, untreated graywater can harbor pathogenic microorganisms, including bacteria, viruses, and parasites. These pathogens pose a potential health risk to consumers if they contaminate crops irrigated with untreated graywater. In the context of livestock management, the use of untreated graywater for drinking or cleaning can similarly introduce pathogens, potentially leading to animal illness and subsequent food safety concerns for humans consuming these animal products (Wardrop et al., 2018). Salinity buildup from detergent residues in graywater is another potential long-term consequence. Continued irrigation with untreated graywater can lead to increased soil salinity, rendering it unsuitable for many crops and ultimately impacting agricultural productivity in these resource-limited areas (Qadir et al., 2023). Therefore, while graywater reuse offers a promising approach for water conservation in rural areas, its direct application without appropriate treatment methods can have significant negative consequences. Further action is necessary to explore and evaluate effective graywater treatment technologies suitable for resource-constrained rural areas.
One promising strategy for mitigating these risks and promoting sustainable graywater management is algal farming as a wastewater treatment technology. Microalgae, microscopic aquatic organisms, offer a unique solution by simultaneously treating the diverse wastewater and producing valuable biomass (Naaz et al., 2019; Dalvi et al., 2021). Unlike conventional methods relying on harsh chemicals, algal farming utilizes natural biological processes, minimizing the environmental impact and potential health risks associated with chemical use (Gupta et al., 2017). Microalgae in synergy with the bacterial community, generally present with algae community, have shown a remarkable ability to absorb and utilize nutrients like nitrogen and phosphorus present in graywater (Mohit and Remya, 2023). Certain algal species can effectively reduce bacterial and viral pathogens present in graywater through various mechanisms, including competition for nutrients and direct predation (Delanka Pedige et al., 2019). The treated water from the algal treatment system can be potentially reused for irrigation or other non-potable purposes, reducing pressure on freshwater resources.
The economic viability of algal farming for wastewater treatment hinges on developing efficient and low-cost cultivation systems. This is crucial for establishing this technology beyond agricultural settings and into rural communities where the treated water can be directly used for irrigation. In India, for instance, several algae-based startups and small businesses are already demonstrating the potential of this approach. Phycolinc Technologies, a Gujarat-based company, utilizes microalgae for in situ remediation of polluted water bodies and industrial effluents, effectively removing pollutants like ammonia, COD, and BOD. Similar efforts are underway by Aurospirul in Tamil Nadu and Caradora in Rajasthan, who focus on cultivating algae for nutraceutical applications. These examples highlight the growing interest and potential of algal farming in India, not just for wastewater treatment but also for generating valuable biomass products. By developing cost-effective cultivation systems, algal farming can become a sustainable and economically attractive solution for rural communities facing water scarcity and limited wastewater treatment options. Using this valuable biomass generated post wastewater treatment for different applications may lead to a self-sustained circular bio-based economy, which helps in complete utilization of resources leading to reduced greenhouse gas emissions (Vishwakarma et al., 2022). The generated algal biomass also holds significant value as a potential fertilizer or biofuel in terms of biogas and biocrude (Choudhary et al., 2017). This two-pronged approach, offering wastewater treatment and resource recovery, strengthens the case for adopting algae farming as a sustainable solution for water management and agricultural development in rural areas.
Microalgal biofilms offer a promising alternative to traditional biofilm systems for wastewater treatment due to the presence of naturally occurring microalgal-bacterial consortia (Qv et al., 2023). This synergistic partnership offers many advantages: in situ oxygen production by microalgae through photosynthesis directly supports the aerobic biodegradation of pollutants by bacteria (Ji et al., 2018; Wang et al., 2023). Furthermore, microalgae act as carbon dioxide sequesters, utilizing the CO2 released by bacteria during respiration for their own growth, creating a closed-loop system within the biofilm. Studies have shown that microalgae-bacteria consortia can achieve significantly higher organic matter removal rates compared to solely bacterial biofilms (Ashraf et al., 2023; Zhang et al., 2023).
Though algal-bacterial biofilm reactors offer a promising approach to sustainable wastewater treatment, its success relies on optimizing a confluence of factors (Nguyen et al., 2024). This includes the physical and chemical properties of the support material, operational conditions for optimal algal growth, the selection of a suitable microalgal strain with strong biofilm-forming abilities, and the intricate interactions between the algae, the support, and the wastewater (Li et al., 2023; Wu et al., 2023; Qian et al., 2024). Different microalgae have varying biofilm-forming abilities, indicating the importance of selecting the right microalgal strain for effective biofilm-based wastewater treatment (Choudhary et al., 2017). Effective algal biofilm-based wastewater treatment relies heavily on optimizing operational parameters like hydraulic retention time (HRT) and flow rate. A number of lab scale studies have demonstrated that an optimal HRT ensures sufficient time for pollutant removal while avoiding excessively short residence times that compromise treatment or long durations that inflate operational costs (Akao et al., 2021; Katam and Bhattacharyya, 2021; Arcila et al., 2023; Jones et al., 2023). Studies have demonstrated that optimizing wastewater flow rate requires careful consideration of the design of algae biofilm reactors to achieve a balance between efficient nutrient delivery and maintaining a healthy and active algal population within the biofilm reactor (Rodrigues de Assis et al., 2020; Katam and Bhattacharyya, 2021; Jones et al., 2023). Particularly, when implementing wastewater treatment systems in low resource settings of rural areas, the ease of operation, local availability of materials and robustness of the systems are important. While diverse reactor designs exist (e.g., vertical, rotating drums, and fiber sheets) (Choudhary et al., 2017; Podola et al., 2017; Wang et al., 2021), rural applications necessitate a specific material selection approach. In context of algal bacterial biofilm system for rural wastewater treatment, the selection of the support material would play a critical role in ensuring efficient reactor operation, promoting both algal growth and effective wastewater treatment (Choudhary et al., 2017; Podola et al., 2017; Wang et al., 2021). Durability is essential, as the material needs to withstand long-term use, potentially under harsh outdoor conditions. Furthermore, it must be non-toxic to avoid harming the algae or introducing contaminants into the treated wastewater. Additionally, selecting a sustainable and readily available material aligns with the principles of eco-friendliness. Finally, affordability is crucial for resource-constrained rural communities. Studies have demonstrated the potential of utilizing readily available, sustainable materials like pine sawdust as biofilm carriers (Zhang et al., 2020). Though, this approach aligns well with the principles of eco-friendly and cost-effective wastewater treatment solutions for rural areas, the performance of other native materials like bamboo-based structures has not been evaluated yet. Further research is required to explore the performance of various sustainable and affordable materials in diverse rural environments, considering all the above stated design and operational factors. Optimizing biofilm growth and pollutant removal while maintaining these key characteristics will be crucial for widespread adoption of algal biofilm technology in rural wastewater treatment applications.
While algal-bacterial biofilm reactors (ABRs) hold promises for wastewater treatment, existing research often focuses on lab-scale or optimized conditions. Existing studies on algal-bacterial biofilms (ABRs) for wastewater treatment often focus on specific settings or operational modes. There is a gap in knowledge regarding the feasibility of implementing ABRs in real-world settings, particularly rural areas with limited resources and infrastructure.
This study addresses a key gap by investigating the optimization, testing, and feasibility of an indigenous algal biofilm reactor (ABR) for treating graywater in rural areas. The study unfolds in three phases. The initial phase focuses on selecting and optimizing the algal biofilm reactor system. Following optimization, the developed reactor was tested in various operational modes, including batch, fed-batch, and continuous operation, to assess its wastewater treatment efficacy. The final phase demonstrates the use of readily available natural materials like bamboo pipes and sheets for constructing the attached algal biofilm reactor system. As a readily available and sustainable resource in many rural areas, bamboo is both cost-effective and eco-friendly. To validate this innovative approach, the study also tested the potential of bamboo-based algal biofilm reactor for rural wastewater management.
2 Materials and methods
2.1 Wastewater collection and characterization
Wastewater samples were collected from the drains through which the water flows into the low-lying pond area on the outskirts of a village site (Mubarakpur, Farukhnagar, District Gurgaon) in Haryana. The sampling site (Supplementary Figure S1) was roughly of dimensions 45 cm × 40 cm (width × depth) with an average flow rate of water 0.81 m3 min−1. Samples were collected three times in intervals of 2 months based on the different climatic conditions, i.e., May (summer), July (during Monsoon), and September (End of Monsoon), to monitor the effect of temperature on the quality of wastewater. The wastewater was collected in pre-cleaned plastic jars (5 L) and was passed through a clean muslin cloth to remove any coarse particles before any analysis. Wastewater samples were analyzed within 2 h of collection for various parameters like, total dissolved solid (TDS), pH, chemical oxygen demand (COD), nitrate-nitrogen (NO3-N), total dissolved phosphate (TDP), total ammoniacal nitrogen (TAN), total suspended solids (TSS), and electrical conductivity (EC). Electrical conductivity (HANNA) and pH (Eutech CyberScan PC510) were measured using specific probes. TDS was calculated as EC x 0.67 × 1,000 (Ali et al., 2012). COD, TDP, TAN, and NO3-N were determined using standard HACH protocols as reported in earlier study (Choudhary et al., 2016). TSS was measured using Aquasensor Datastick (RT2142, Thermo). Dissolved oxygen (DO) was measured using a DO probe (HANNA). The wastewater was stored at 4°C for further experiments. All the experiments were conducted in triplicates and the results were expressed as Mean ± Standard Error (S.E.).
2.2 Algal-bacterial culture conditions
An algal-bacterial consortium PA6, dominated by Chlorella sp. and Phormidium sp. along with presence of bacteria of various families previously isolated from wastewater (Choudhary et al., 2016), was used in the present study for the wastewater treatment in attached biofilm reactor. The protocol for inoculum preparation given in our previous study was followed (Choudhary et al., 2017). Initially, microalgal cells were cultivated in TAP water medium (suspension) containing Na2CO3 (0.02 g L−1); NaNO3 (0.15 g L−1); and KH2PO4 (0.0125 g L−1) under control conditions (T = 24–27°C; light intensity: 50–80 μmol m−2 s−1) till the optical density at 680 nm (OD680) reached up to 2.0. The algal culture having OD680 2.0 was used as inoculum throughout the experiments, unless stated otherwise.
2.3 Design and development of algal-bacterial biofilm system
The design of algal-bacterial biofilm reactor previously reported in our studies (Choudhary et al., 2017) was used in the present study, where a polypropylene fiber board covered with non-woven spunbound fabric (70 GSM) was used as support system for biofilm formation. Precisely, the algal-bacterial biofilm support system assembly comprised of a 30 cm × 21 cm wide fiber board base on which a PVC pipe (Diameter-2 cm) with multiple perforations (2 mm at every 1.5 cm interval along the length) was fixed with a strong adhesive along the breath of the board. This biofilm support system was fixed at an inclination of 19° onto a recirculation tank (4 L capacity) containing a submersible pump and a silicon pipe connected to biofilm support material. These perforations on PVC pipe were made to redistribute nutrient medium (or wastewater) evenly through the turf surface.
Alternatively, an alternative attached biofilm reactor was designed to utilize some locally available resources to fabricate an algal biofilm assembly. The material selected was bamboo considering its local availability in villages as well as strength and durability of material. The design was similar to previous system, except replacement of PVC tube and fiber sheet by bamboo for customized dripping system and solid support for biofilm attachment material, respectively. The selected attachment material was placed on bamboo sheet with an effective area of 670 cm2. A uniform slit throughout the width of the bamboo pipe served as a dripping system unlike perforations in PVC pipes in the previous design made up of polypropylene fiber board. Rest of the experimental conditions (growth conditions, inclination, flow rate, working volume, nutrient medium, and inoculum preparation) were kept similar to polypropylene fiber based attached biofilm reactor.
2.3.1 Development and quantification of algal-bacterial biofilm
Selected algal culture was a consortium, PA6 mainly dominated by Chlorella sp. and Phormidium sp. These two species are thoroughly studied in the literature and were found to perform well in a range of wastewater system (Choudhary et al., 2016). Bacteria were indigenously grown within the algal biofilm and played a synergistic role in the growth and waste water treatment. To begin with, the recirculation tank containing TAP media (Na2CO3, 0.02 g L−1; NaNO3, 0.15 g L−1; KH2PO4, 0.0125 g L−1) was inoculated with 10% (v/v) exponentially growing algal culture (0.7 g L−1). The biofilm was grown under controlled conditions (T = 24–27°C; light intensity: 50–80 μmol m−2 s−1) of green house. White light was provided by Light Emitting Diodes (LEDs), positioned outside of the growth system. Harvesting of algal-bacterial biomass was done on sixth day of growth based on the previous optimization of harvesting frequency (Choudhary et al., 2017). The scanning electron microscopy (ZEISS EVO 50) of the developed and harvested algal-bacterial biofilm was performed. The harvested biofilm was washed using phosphate buffer saline (0.1 M, pH 7.2) followed by fixing the biomass in 2.5% gluteraldehyde. The fixed algal-bacterial biomass was freeze dried using a lyophilizer (Allied Frost FD3) before subjecting to SEM viewing at 10,000X (Bhattacharya et al., 2017). The thickness of the biofilm was estimated for each harvest (6 days). The harvested biomass was dried in a hot air oven at 55°C in a pre-weighed container. The container with dried biomass was weighed again and the difference of both was calculated (empty container and container with dried biomass). The biomass productivity was expressed in terms of dry cell weight (g) per m2 per day.
2.4 Wastewater treatment in algal-bacterial biofilm reactors
The wastewater treatment was performed in three types of reactor operation: Batch, Fed-batch and Continuous mode. All the experiments were conducted in triplicates and the results were expressed as Mean ± Standard Error (S.E.). This was done to increase wastewater treatment capacity using the same biofilm reactor.
2.4.1 Batch and fed-batch reactor
For batch operation of the reactor, about 2–3 L of wastewater (gray water) was poured into the recirculation tank. A 2 W submersible pump was used to pump up the water at the rate of 2 L min−1 from the recirculation tank to the inlet hole of the PVC pipe. The PVC pipe redistributed the nutrient medium along the turf surface which then by virtue of gravity falls back to the recirculation tank. Thus, the cyclic motion of nutrient medium was maintained. This batch reactor was operated under 3 conditions, i.e., (i) Controlled condition—in green house maintained at 25°C and approximately 65 μmol m−2 s−1; (ii) Semi-outdoor condition—placed in a shed (outside green house) with average temperature = 27–34°C; light intensity: 45–50 μmol m−2 s−1 provided through artificial white light illumination (by two 28 W LED bulbs run 24 h); and (iii) Outdoor condition—average temperature range = 25–34°C; with day light intensity variation between 11.1 and 875.05 μmol m−2 s−1.
For the fed-batch system, the wastewater was pumped from a 20 L container and was allowed to flow down through the biofilm into the recirculation tank from where a 2 W submersible pump pressured water back into the 20 L container. The 20 L container was covered to block light to avoid growth of algae within the container. Two cycles were consecutively run for the fed-batch treatment, where the wastewater (20 L) was added on the seventh day of treatment. The treated water (on seventh day) from previous cycle was stored in a separate storage bottle.
2.4.2 Continuous reactor
Continuous setup consisted of two 20 L containers each to act as inlet and outlet tanks. Two peristaltic pumps were used; the first peristaltic pump was used to constantly feed gray water from the inlet tank to the recirculation tank. The second peristaltic pump was used to pump out water from the recirculation tank to the effluent tank. Both pumps were adjusted to maintain a flow rate of 2 mL min−1. The biofilm assembly was placed within the recirculation tank. The gray water was recirculated through the algal-bacterial biofilm using a 2 W submersible pump as explained in section 2.4.1.
2.4.3 Experimental conditions and wastewater treatment in the reactor set-ups
All three types of reactors were initially run in control conditions within a green-house [Temperature = 24–27°C; light intensity: 50–80 μmol m−2 s−1; white light provided by light emitting diodes (LEDs), positioned above growth surface for 24 h]. For batch reactor, the samples were withdrawn from the recirculation vessel, for fed-batch from the 20 L container and for the continuous reactor the samples were taken from the outlet tank. For all the setups, after every 24 h, 50 mL samples were collected in plastic centrifuge tubes to monitor COD, TDP, TAN, TSS, pH, DO and NO3−-N. For biomass productivity, the biomass was harvested for each reactor after every cycle (at the end of 6th day) by manual scraping. The collected biomass was weighed (wdry) and then oven dried at 50°C for 24 h. The dry weight of the harvested biomass was found out. The biomass productivity was calculated as follows:
Fecal coliform test was also performed in samples procured in the beginning and end of each cycle to find out the total coliform removal. Coliform plates were prepared using M-FC Agar base media (Himedia) mixed with 1% Rosolic Acid (FD058). For coliform analysis, 1–2 mL samples were collected in micro centrifuge tubes. Dilutions (101, 102, 103) were prepared and 100 μL of each dilution were spread on coliform plates (performed in duplicates). The inoculated plates were incubated at 45°C for 24 h. After the incubation period, the coliform colonies were counted to calculate the coliform count using following formula:
3 Results and discussion
In the present study, polypropylene based algal-bacterial biofilm reactor was used to investigate the treatment efficiency of wastewater collected from Mubarakpur village, Haryana (India) under multi-mode operations as well as different environmental conditions to establish the consistent performance. To improve the environmental footprints and enhance the local resource utilization, a bamboo-based ABR was developed and evaluated for the wastewater treatment efficacy. An algal farming model has been proposed to indicate the feasibility of such treatment systems in the rural settings.
3.1 Effect of climatic variation in the wastewater quality
The village selected for the study, Mubarakpur, Haryana, consisted of a total population of 4000 (approximately) with 500 number of households. The daily gray water generation capacity was estimated to be 96,000 L day−1. The gray water generated due to household activities and was discharged in a small unlined and uncovered drain to the low-lying pond area on outskirts of the village through narrow streams. This gray water was collected from the drain for further experiments in the present study. The characteristics of the gray water collected from the sampling site along with the ambient temperatures have been summarized in Table 1. In summer months (May–June), high nutrient accumulations due to high temperature was recorded. Water collected during Monsoon season (July–August) showed less levels of nutrients due to dilution with additional water from rain. The COD reduced from 275 ± 9.25 mg L−1 to 52 ± 3.77 mg L−1 and nitrate was decreased from 11.8 ± 0.825 to 5.5 ± 0.68 mg L−1, when season was changed from summer to monsoon. Similarly, TAN and TDP was also reduced from 71.2 ± 4.26 and 39 ± 1.06 mg L−1 to 36.8 ± 2.87 and 16.8 ± 0.92 mg L−1, respectively, in monsoon season. This wastewater majorly consisted of domestic water coming from the rural households (from kitchen and washing area), animal washing etc. The known industrial drain near this village is Outfall Drain no. 8, which is located approximately 6 Km (areal distance) away from Mubarakpur village. However, the water from this drain does not flow toward this village. There is an irrigation canal 1 km away from the village, which flows outside the agricultural fields and then drains into this main drain no. 8 (Supplementary Figure S1). Hence, no industrial/urban wastewater is mixed with the wastewater selected in the study. Moreover, the construction companies, automobile industries, electronic industry or petrochemical industries are mostly present in Bawal, Rewari, Dharuhera and Manesar cities of Haryana, which are approximately 33 to 67 km away from Mubarakpur, Haryana.
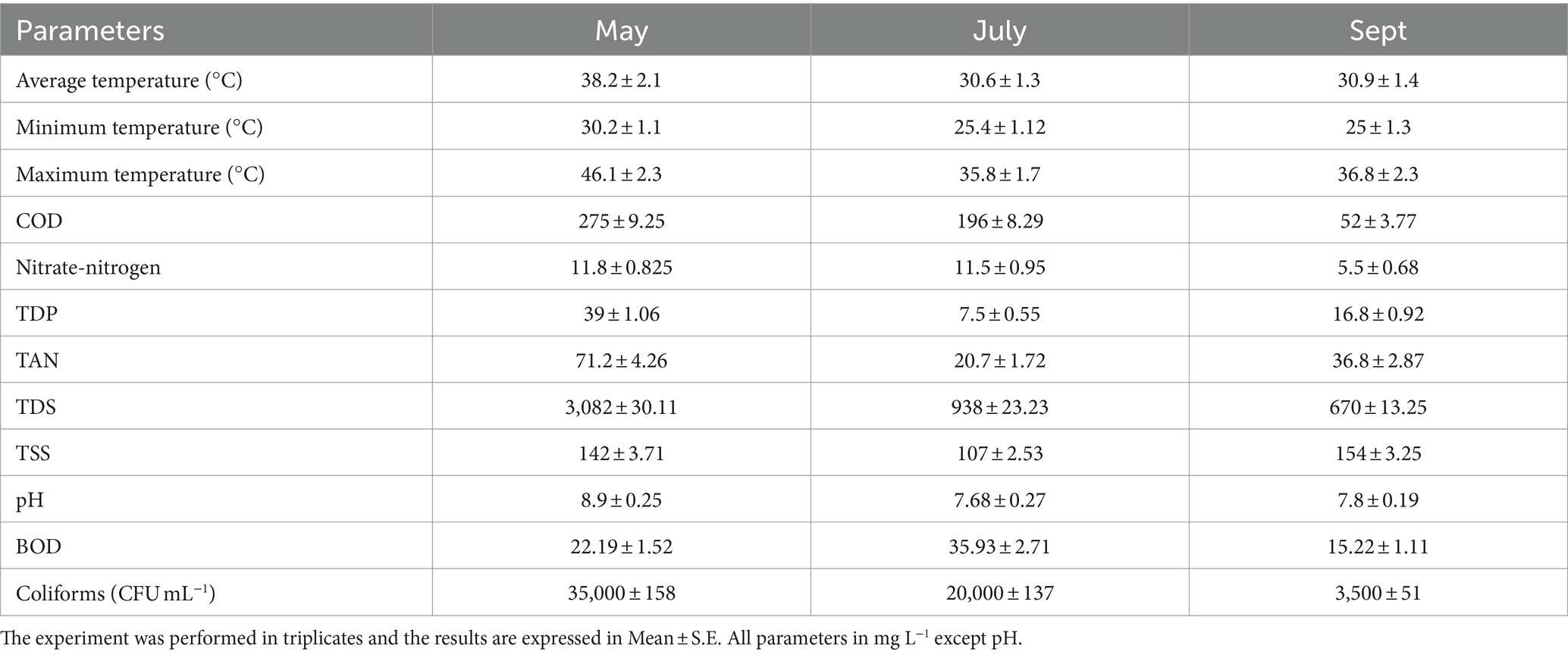
Table 1. Physico-chemical properties of gray water collected from Mubarakpur village, Haryana in different months.
3.2 Wastewater treatment in algal-bacterial biofilm reactor
To perform the wastewater treatment experiments, the bioreactors were initially allowed to run on conventional TAP media and biofilm was grown. The scanning electron microscopic studies clearly indicated the presence of algal (including rounded and filamentous algae) and bacterial communities (Supplementary Figure S2). Before the start of wastewater treatment experiments, the media grown biofilm was harvested after 6 days of cultivation using manual scraping followed by addition of gray water for treatment. The biomass productivity after 6 days of growth was found to be 2.89 g m−2 day−1.
3.2.1 Batch study
The changes in nutrient profile during gray water treatment in algal-bacterial attached biofilm reactor operated in batch mode during the total experimental was monitored for the duration of 6 days (Figure 1; Table 2). The total treatment capacity in batch study was 3 L. It was observed that COD showed a sharp decrease within 2 days of treatment from 275 ± 9.25 mg L−1 to 92 ± 3.28 mg L−1, followed by gradual reduction up to 50 mg L−1 after 6 days. The resultant removal efficiency for COD was found to be 81.81%. Similar trend was observed for TAN and TDP removal, which showed 83.42 and 88.46% removal efficiencies after 6 days, respectively. However, in case of nitrate-nitrogen, the concentration fluctuated from low to high within first 4 days, followed by its reduction on 5 and 6th day (49.15%). There was 100% reduction in TSS and 99.92% reduction in total coliforms. Dissolved oxygen was also found to be slightly improved from 7.1 ± 0.15 to 7.47 ± 0.23 mg L−1. A significant reduction in several parameters was observed leading to the improvement in water quality.
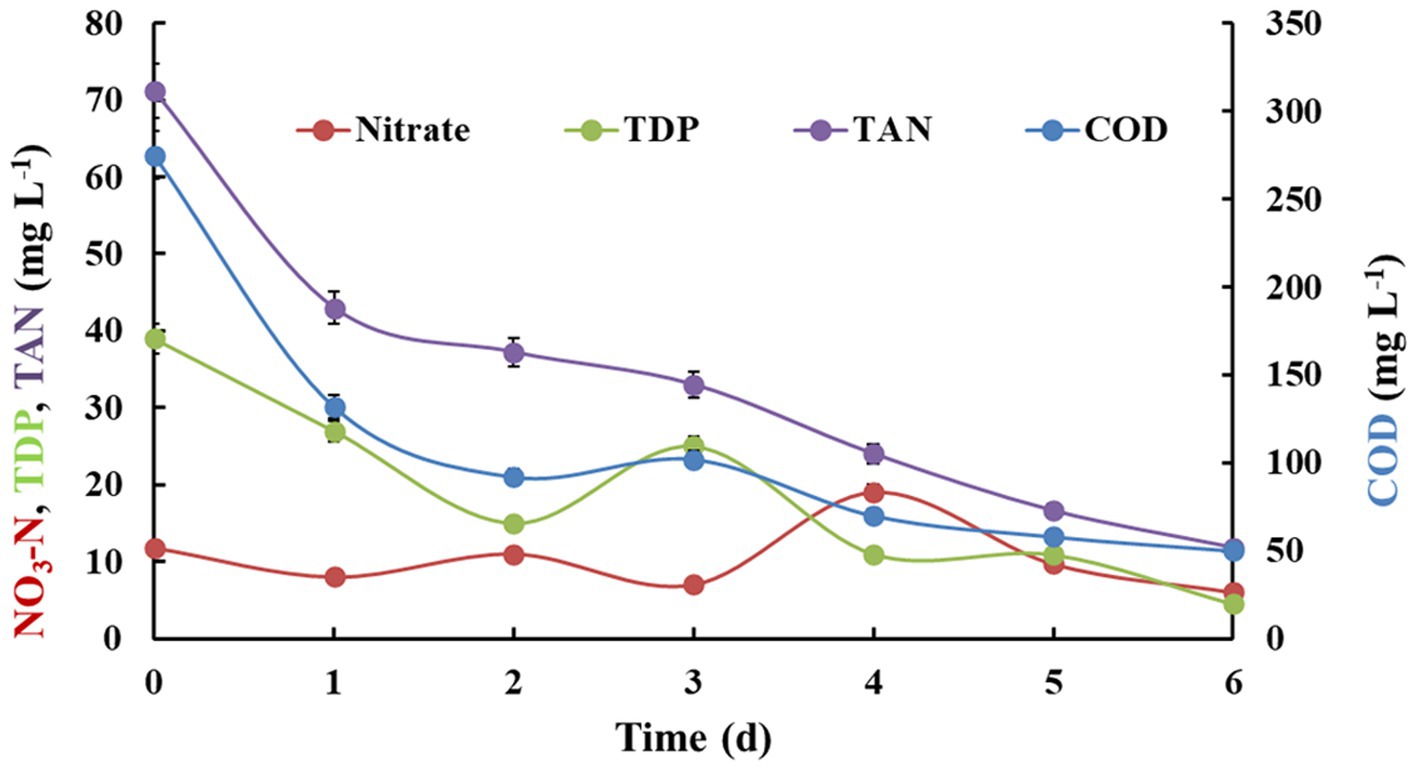
Figure 1. Nutrient removal by algal-bacterial biofilm from gray water in a batch reactor setup. The experiment was performed in triplicates and the results are expressed in Mean ± S.E.
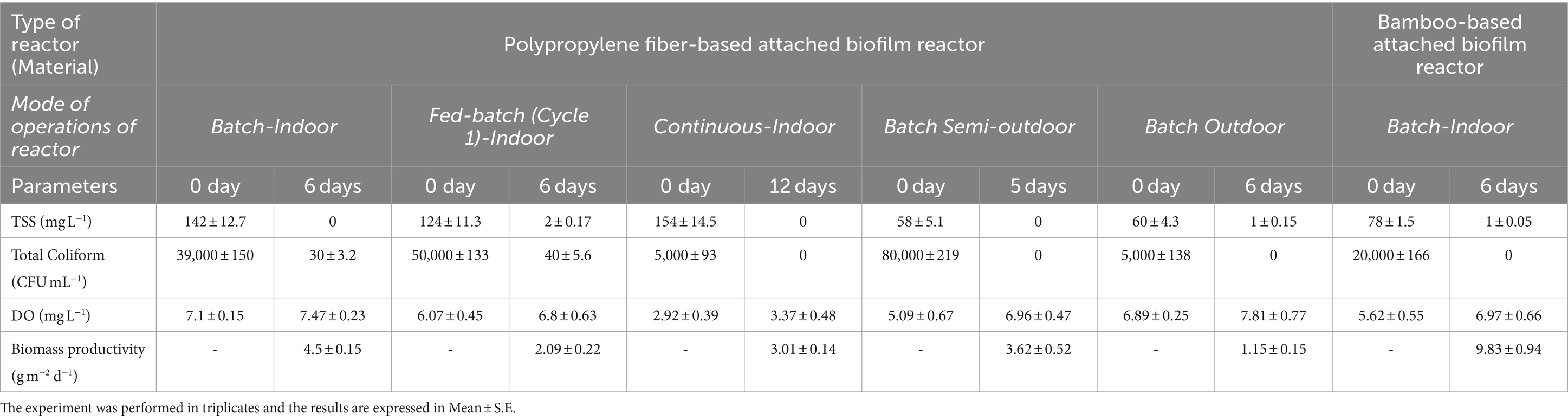
Table 2. Biomass productivity and concentrations of total suspended solids (TSS), total coliforms, dissolved oxygen (DO) after treatment with algal-bacterial biofilm in multi-mode operational conditions.
In our previous studies, the wastewater collected from Mubarakpur, Haryana were treated using suspended systems by several microalgal consortia and pure cultures in the flask level suspended microalgal systems (Prajapati et al., 2013; Choudhary et al., 2016). The above study depicted efficient removal in the suspended system followed by the use of resultant microalgal biomass for biogas production (Prajapati et al., 2013). However, biomass was harvested from the suspension using a centrifuge. In spite of having an efficient removal potential, the feasibility of using suspended biomass for wastewater treatment in rural area is limited due to involvement of high cost, energy and infrastructural facility. Hence, an alternative bioreactor system was chosen for wastewater treatment, which has already been established in our previous study (Choudhary et al., 2017) and also by other researchers (Hodges et al., 2017; Katam and Bhattacharyya, 2021).
The nutrient removal rates of the developed system showed comparable efficiency with the reported biofilm systems (Liu et al., 2024). At the end of 7th day cultivation period, the biofilm was harvested by manual scraping and kept in oven for drying for approximately −24 h. The biomass productivity of the biofilm was found to be 4.5 ± 0.15 g m−2 day−1, which was significantly higher than the reported studies (Posadas et al., 2013; Liu et al., 2024). The mitigation of nutrients can be clearly correlated by the nutrition requirements of the microalgae for its propagation. The nitrate-nitrogen (NO3-N) and ammonia are generally utilized as the nitrogen source (N) (Biller et al., 2012). Moreover, another research has interpreted that microalgal cells utilize ammonia as their preferred source of nitrogen rather than nitrate (Salbitani and Carfagna, 2021). It has been reported that inorganic nitrogen is assimilated in the microalgal cells during heterotrophic and autotrophic growth (Renuka et al., 2015).
Synergy of algae and bacteria plays a crucial role in the ammonia removals as it involves rapid oxidation of ammonia to nitrite and nitrate followed by assimilation in algal or bacterial biomass. The nitrification process, i.e., conversion of ammonia to nitrate or nitrate is being mediated by nitrifying autotrophic bacteria. Heterotrophic bacteria and algal biomass tend to assimilate these nitrified forms within itself, thereby removing the ammonia from the surrounding (Fallahi et al., 2021). Similar pattern was also observed in our previous study on wastewater treatment in algal biofilm reactor (Choudhary et al., 2017). Similarly, phosphate from the cultivation medium/wastewater acts as the phosphorus source (P) for growth of algae as it is involved in many cellular and metabolic processes (Franchino et al., 2013). Hence, there was an exponential decrease in the phosphate concentrations with increase in the biomass film. The dramatic decrease in COD levels from 275 mg L−1 to 92 mg L−1 on second day of growth and then to 50 mg L−1 after 6 days suggest that COD solubilization and conversion to CO2 is being mediated by the synergistic growth of algae and bacteria present in the algal consortium, which is acting upon the organic substrates present in wastewater (Hasnain et al., 2023). The metagenomic studies conducted for PA6 (data not shown) also depicted algae as the most dominant community (Chlorella variabilis) and the bacterial community consisting of Rhizobiaceae, Enterococcaceae, Brucellaceae, Cyclobacteriaceae, Porphyromonadaceae, Sphingobacteriaceae, and Pseudomonadaceae as the predominant bacterial families. These bacterial families might be acting as positive correlation with algae to treat wastewater nutrients by the mechanisms stated above.
3.2.2 Fed-batch study
The fed-batch study was performed to increase the treatment capacity to 20 L (Figure 2; Table 2). For this, the nutrient removal efficiency was monitored for 2 cycles of 6 days each (24 h HRT at 2 mL min−1 flow rate). For both the cycles, all parameters eventually were reduced in the range of 50–90%. In case of nitrate-nitrogen concentration, a similar trend during the cycles was observed as that in batch studies. In the first cycle (0–6 days), it first increased upto 51.5 ± 2.75 mg L−1 and then decreased to 11.6 ± 1.33 mg L−1 after 6 days. Similarly, for second cycle, a sharp increase was observed on the eighth day then gradually reduced till 9.5 mg L−1 after 12th day (56%). The net removal efficiency of other parameters like COD, TDP, and TAN for 1st cycle was, respectively, 57.7, 61.9, and 76.7%, which was improved during the second cycle (76.3, 93.3, and 60.1% respectively). In addition to nutrient removal, algal-bacterial biofilm reactor was able to reduce TSS up to 98% in cycle 1 and 91% in cycle 2. The total coliform was found to be removed by 100%. The DO levels were found to be significantly improved from 6.07 ± 0.45 mg L−1 to 6.8 ± 0.63 mg L−1 after the first cycle and then from 3.4 to 8.01 mg L−1 after the second cycle. The biomass productivity of the biofilm after first cycle was estimated to be 2.09 ± 0.22 g m−2 day−1 while for second cycle the productivity slightly increased to 2.15 ± 0.17 g m−2 day−1. The increased biomass productivity is in accordance with the nutrient removal efficiency, which was observed to be higher for cycle 2.
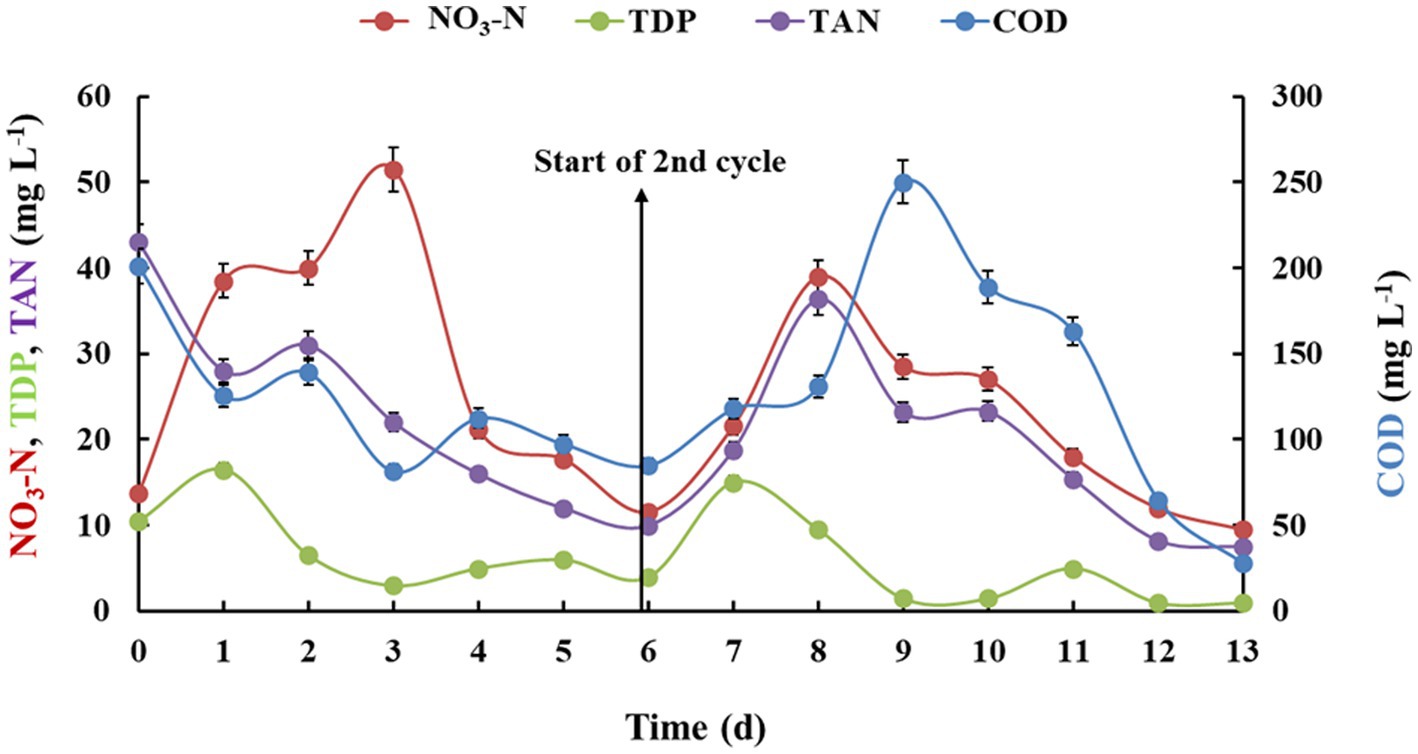
Figure 2. Nutrient removal by algal-bacterial biofilm from gray water in a fed-batch reactor setup at control conditions. The experiment was performed in triplicates and the results are expressed in Mean ± S.E.
Algal biofilm developed on a ceramic foam filter (silicon carbide) have been reported to integrate with the activated sludge process (in the aeration tank known as modified aeration tank) to improve the domestic wastewater treatment (Katam and Bhattacharyya, 2021). For this, pregrown algal concentrates (5,000 mg L−1 total solids) on the foam filter were added and the performance of aeration tank was monitored. The trace metal elements were fed in the tank every day to provide essential nutrient for microalgal growth. LED lights were increased in different phases and operated to increasing light intensities. The results indicated significant increase in removal efficiencies of total carbon (67%), nitrogen (63%) and phosphorus (70%) in the modified aeration tank as compared to aeration tank without algae (55, 48, and 37%, respectively). The present study also depicted a better removal efficiency in Cycle 2 after addition of wastewater nutrient, which would have helped the microalgal-bacterial biomass to grow better in presence of available nutrient with higher biomass productivity.
Several fed-batch studies for wastewater treatment using microalgae have been reported in suspended microalgal systems (Beydeş and Karapinar, 2018; Delanka-Pedige et al., 2020), but very few reports on biofilm reactors are available (Zhang et al., 2021). Zhang et al. (2021) have reported removal of polyacrylamide and other nutrients like COD and nitrogen from wastewater produced during petroleum extraction using fed-batch revolving algal biofilm reactor. It was observed that the removal efficiencies of COD (30%) and polyacrylamide (40%) were lower in the wastewaters (without external nitrogen) but increased to 58 and 64%, respectively on addition of external carbon source (KNO3). Addition of external nitrogen did not affect the absorption of total nitrogen with removal efficiencies of 85% in both the cases. This phenomenon is true for the urban or industrial wastewaters containing harsh chemicals like polyacrylamide, where nitrogen assimilation is necessary for the removal of such chemicals. However, in the present study wastewater from rural sector was collected, which consists of only gray water coming from households and animal washing. No industrial effluent/urban effluent was mixed in the pond or drains from where the wastewater samples were collected.
3.2.3 Continuous study
The continuous study (performed during monsoon season) was done as advancement to the batch and fed-batch study in order to increase the treatment capacity to 20 L per day till 12 days (HRT 24 h with flow rate of 2 mL min−1). The reactor was designed in such a way that water was continuously fed from an inlet tank to recirculation tank which was then subjected to algal-bacterial treatment. The treated water on the other side was simultaneously discharged into the outlet tank at the same rate. The nutrient removal profile under continuous system is shown in Figure 3. COD depicted a gradual decrease in concentration per day till 12 days, with a final removal efficiency of 53.5%. TDP showed a sharp decrease on the second day of the continuous cycle from 16.8 ± 1.75 to 2.9 ± 0.55 mg L−1 and dropped down to 0 in 12 days. Similarly, the daily removals of TAN were also effectively high, which showed a decrease from 28.6 ± 1.67 to 5.38 ± 0.13 mg L−1 in 12 days. Similar to the previous results of batch and fed-batch, nitrate-nitrogen concentrations fluctuated during the treatment and then finally reduced from 11.8 ± 1.83 mg L−1 upto 8.8 ± 1.09 mg L−1 after 12 days. All the above parameters were observed to approach a steady state after eighth day. The TSS content was also reduced from 154 ± 14.5 mg L−1 to 5 ± 0.15 mg L−1 at the end of growth cycle. The treated water depicted slightly better DO level (3.37 ± 0.48 mg L−1) than the initial value (2.92 ± 0.39 mg L−1). No coliforms were detected at the end of the experiment in the treated water. The biomass productivity after 12 days of experiment was found to be 3.01 ± 0.14 g m−2 day−1 (Table 2).
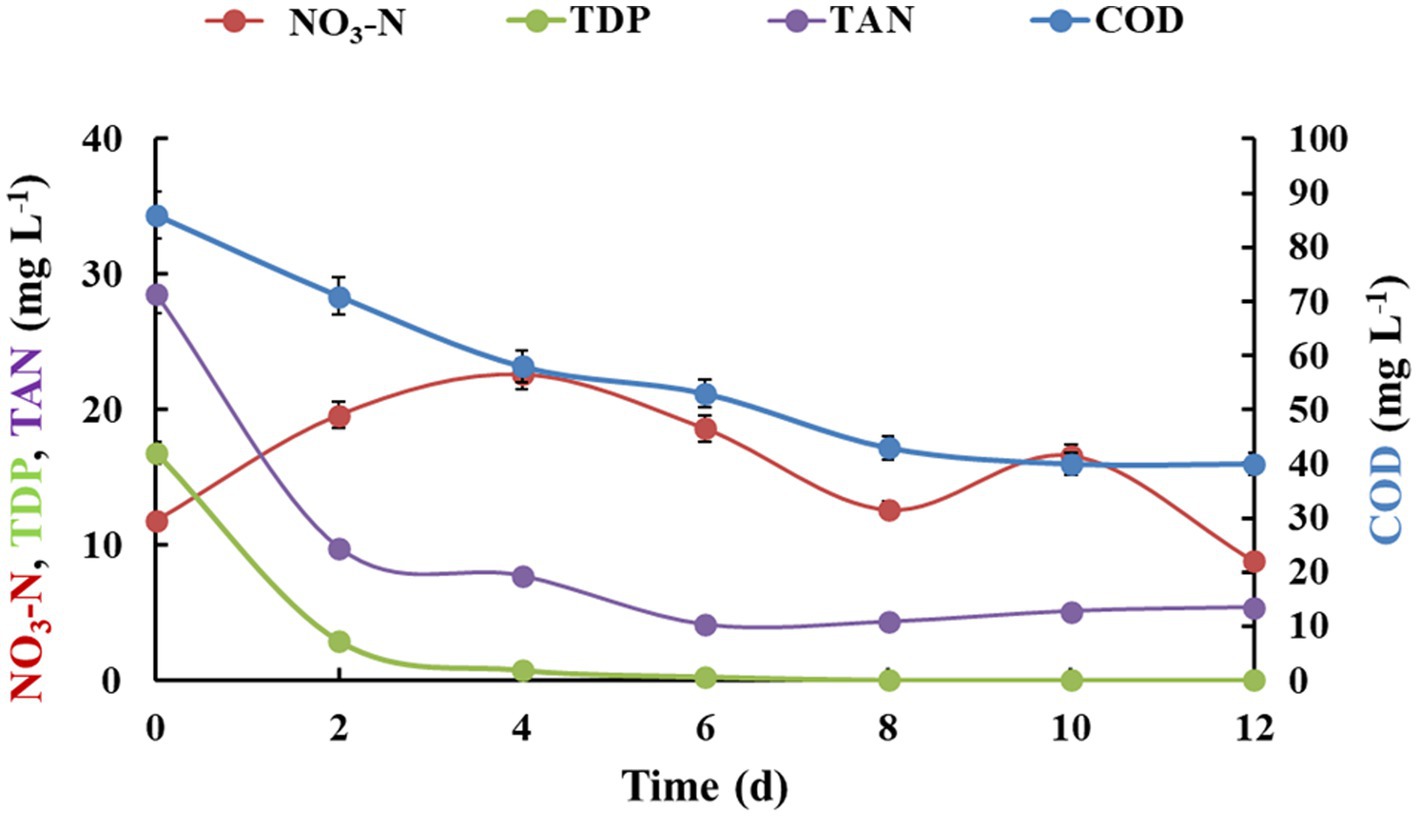
Figure 3. Nutrient removal by algal-bacterial biofilm from gray water in a continuous reactor setup. The experiment was performed in triplicates and the results are expressed in Mean ± S.E.
Algal-bacterial consortium was studied for domestic wastewater treatment (Posadas et al., 2013) in a continuous mode with different HRTs (10.4, 5.2, and 3.1 days). The study depicted highest removal efficiencies of carbon (91%), nitrogen (70%) and phosphorus (85%) with 10.4 days HRT. The present study depicted comparable removals of these nutrients in HRT of 1 day only, which could be further improved upon optimization and long-term operations. A hybrid wastewater treatment system has been reported which consisted of a continuous High Rate Algal Ponds (HRAP) in sequence with a biofilm reactor (Rodrigues de Assis et al., 2020). The results indicated removal of 59% COD, 78% ammonia nitrogen, 16% total phosphorus, and 1 log unit of Escherichia coli bacteria from wastewater with HRT of 10 days. The previous studies and the results of present in continuous in continuous mode depicts the necessity of further optimization and operations of the system for increasing the efficiency.
3.2.4 Batch studies of algal-bacterial biofilm reactor in semi-outdoor and outdoor conditions
The batch studies in semi-outdoor and outdoor conditions were performed to mimic the actual onsite environmental conditions with respect to the temperature, sunlight and other factors. The algal-bacterial biofilm system exposed to semi outdoor conditions, witnessed a good biomass productivity compared to controlled conditions. After 6 days of experimental run, the productivity was 3.62 ± 0.52 g m−2 day−1 which was slightly reduced from the controlled condition (4.5 ± 0.15 g m−2 day−1). This could be due to the decreased nutrient concentration in the feed wastewater as these experiments were performed in the monsoon season. This experiment faced the growth of red colored worm on the biofilm after fifth day of experiment which created a menace in the experiment. It could be due to availability of the reactor for the breeding of these worms in the hot and humid semi-outdoor conditions (27–34°C). Hence, an herbal insecticidal formulation previously developed by our research group (Chauhan et al., 2015, 2016) was added to the water in bioreactor, to kill the worms. Hence, the data of nutrient removal efficiency up to fifth day is considered for comparison, as some of the parameter showed an increase after addition of the insecticidal formulation (Figure 4; Table 2). The nutrient removal was found to be highly efficient after fifth day as the COD levels were dropped down from 52 ± 2.18 mg L−1 to 25 ± 1.36 mg L−1 and nitrate-nitrogen reduced from 5.5 ± 0.95 to 1.8 ± 0.21 mg L−1. The dissolved phosphates (TDP) showed complete removal at fourth day and TAN decreased up to 1.47 ± 0.87 mg L−1 after fifth day. The treated water showed 98.27% decreased TSS levels with improved DO levels (5.09 ± 0.67 to 6.96 ± 0.47 mg L−1), without any detectable coliform bacteria.
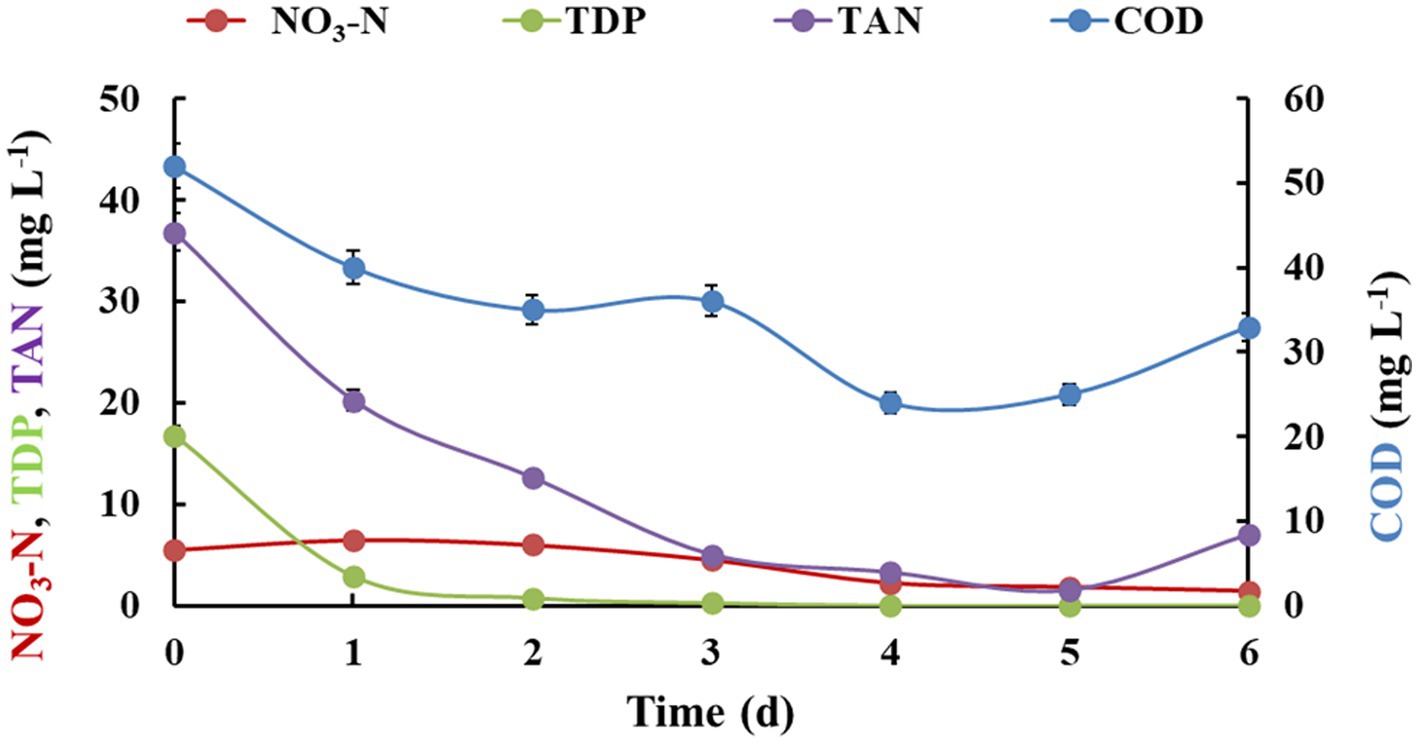
Figure 4. Nutrient removal by algal-bacterial biofilm from gray water in a fed-batch reactor setup in semi-outdoor. The experiment was performed in triplicates and the results are expressed in Mean ± S.E.
In the outdoors, biomass productivity was not as good as the one run in the semi-outdoor. The weather conditions were mostly harsh during the experimental run, with frequent heavy rains and cloudy sky. The light intensity fluctuated from as low as 1.11 μmol m−2 s−1 during night to average 103 μmol m−2 s−1 during a cloudy day, which sometimes reached as high as 880.6 μmol m−2 s−1 under direct sun exposure. After 6 days of experimental run, the productivity was only 1.15 ± 0.15 g m−2 day−1, which was almost 74% less from the control conditions. Interestingly, this reactor system in outdoor conditions did not observe any red worm growth during the experiment. The nutrient removal efficiencies showed similar trends to that of semi-outdoor conditions. The COD removal under outdoor set-up (55%) was better than semi-outdoor (52%) but not as good as control conditions (81%). Nitrate-nitrogen was reduced from 6.1 ± 1.01 mg L−1 to 2.1 ± 0.43 mg L−1, whereas TAN showed removal from 37.5 ± 3.15 mg L−1 to 3.1 ± 0.77 mg L−1. TDP was completely removed within 4 days of treatment (Figure 5). Almost complete removal of TSS and coliform bacteria was observed in the treated water with improvement in DO levels (Table 2).
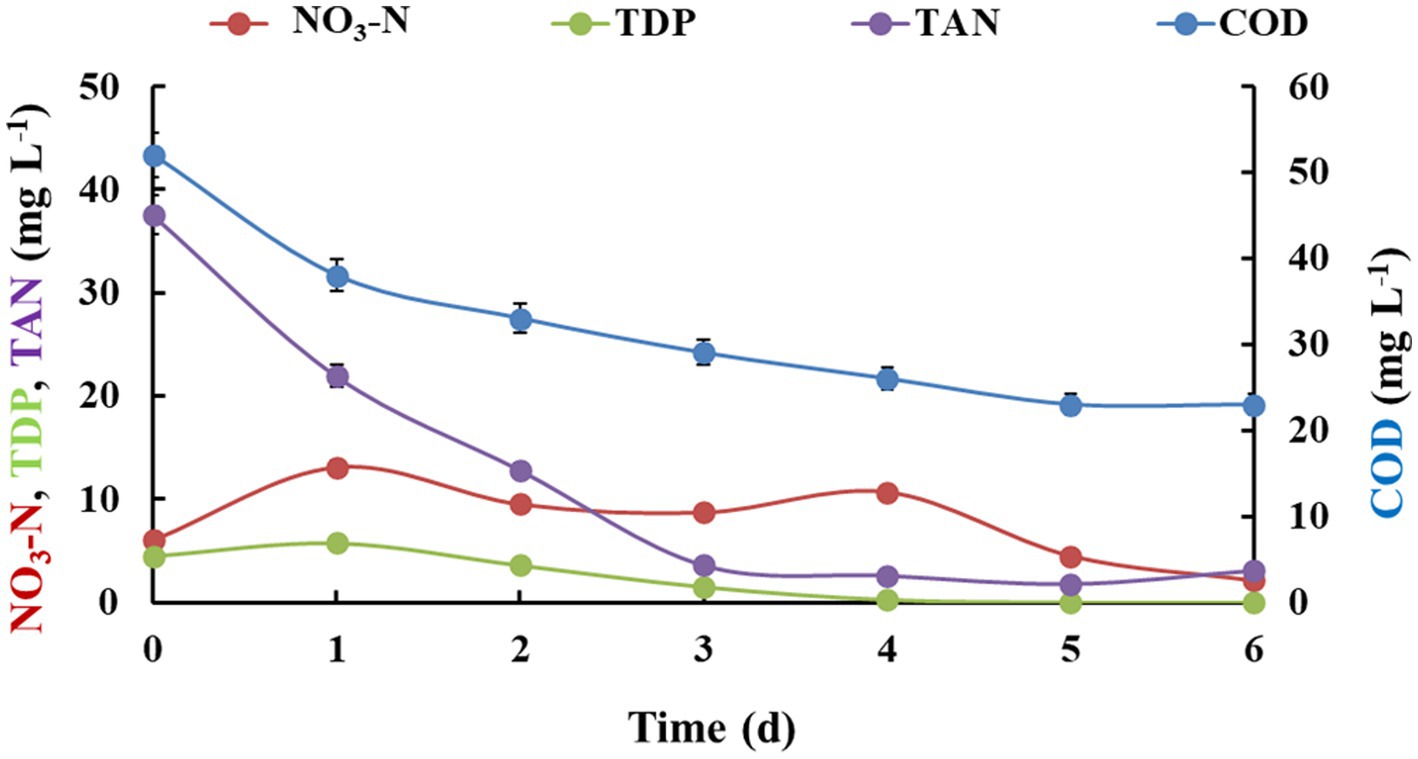
Figure 5. Nutrient removal by algal-bacterial biofilm from gray water in a batch reactor setup under outdoor conditions. The experiment was performed in triplicates and the results are expressed in Mean ± S.E.
The wastewater treatment using algal-bacterial biofilm reactor in semi-outdoor and outdoor conditions depict the importance of applicability of the process in the actual rural settings. The problems which were faced during the experimentation at lab scale will help us provide its solution to be applied in the pilot scale and field scale set-ups for the wastewater treatment. There are very limited technologies available for onsite treatment wastewater including the constructed wetlands and microalgal technology. The wetlands need a very large arable land for the set-up and also a coarse pretreatment of wastewater is also needed before feeding into wetlands. A combination of these two treatment techniques were also studied by de Souza et al. (2019), where a combined system of algal turf scrubber and down-flow vertical constructed wetland could treat sanitary wastewater with HRT of 21 days, which could be used for washing, gardening and irrigation. These techniques are still at the research level and need further investigations and scale-ups for its actual implementation.
3.3 Wastewater treatment in low-cost bamboo based algal-bacterial biofilm reactor
After successful attempts of gray water treatment using algal-bacterial biofilm reactor, bamboo as an alternative material for fabricating the biofilm support sheet in the reactor was explored (Figure 6; Table 2). Considering the easy availability in rural areas, durability, low cost and high tensile strength of bamboo, it was chosen to replace the existing polypropylene fiber sheet for biofilm support construction of algal biofilm reactor. As compared to polypropylene fiber-sheet based systems, biomass productivity was found to be 10-fold higher along with significant nutrient removal efficiency (9.83 ± 0.94 g m−2 day−1). The nutrient removal efficiencies depicted 77% of COD removal, 92.12% of TAN removal, 81.21% TDP removal and 86.4% Nitrate-nitrogen removal after 6 days of treatment. TSS also showed a sharp decrease from 78 ± 4.71 to 1 ± 0.12 mg L−1. The treated water was free from any coliform bacterial contamination (100% removal as compared to feed wastewater) and the DO levels were also significantly high. In spite of such efficient removal potential by bamboo-based system, there were some problems which occurred during the operation. The long terms operation (6 days or beyond) of the system resulted into some white and black fungal growth on the bamboo substratum. This resulted in hampering the use of this system for long term and for multiple cycles. Moreover, this also caused occurrence of yellowish tinge into the water, which could be due to the release of digested compounds from bamboo caused by fungal invasion. Moreover, the spread of fungus on the algal-bacterial biofilm may also be related to the consumption of algal-bacterial biomass by fungus as the nutrient source. Hence, this also degrades the algal biomass quality for any future application. This problem can be resolved by using the treated bamboo for the construction of biofilm reactor in future, as wood preservation is a well-known process to preserve bamboo from any fungicidal activity (Möller and Mild, 2019). The conventional ways of chemical treatment for wood protection (bamboo in this case) might lead to the increase in cost of the overall process in rural settings due to the cost of chemicals and transportation of this chemical to the rural areas. In view of this, lot of plant based natural products like essential oils, tannins and wood extracts have also been reported to show wood protection against fungal contamination (Broda, 2020). Moreover, the one of the active components of essential oil namely Euglenol has been reported to show embedment in natural cotton fabric and depicts anti-fungal activities to protect the wood (Wen et al., 2024). A bio-based wood paint derived from the pigment extracted from wood waste has also been reported to be effective in wood protect specifically counteracting the proliferation of fungus also (Calovi and Rossi, 2023). Our previous studies have reported a highly effective the formulation prepared by the use of essential oils for insecticidal (Chauhan et al., 2016) and anti-fungal activities (Tyagi and Malik, 2010). This formulation was also used to control the worms in our previous experiment in semi-outdoor conditions. Hence, the use of these formulations did not harm the growth efficiency of the algal-bacterial biofilm. These natural essential-oil based formulations/fabric/bio-based wood paints can be prepared in rural settings in a hassle-free manner, which may lead to the protection of bamboo sheets from any other microbial/insect contamination. This might result in a longer life span of the bamboo-based reactor for rural applications and might be cost-effective also. However, these formulations/essential oil based fabric/paint has not been tested in the present study and needs extensive research in future.
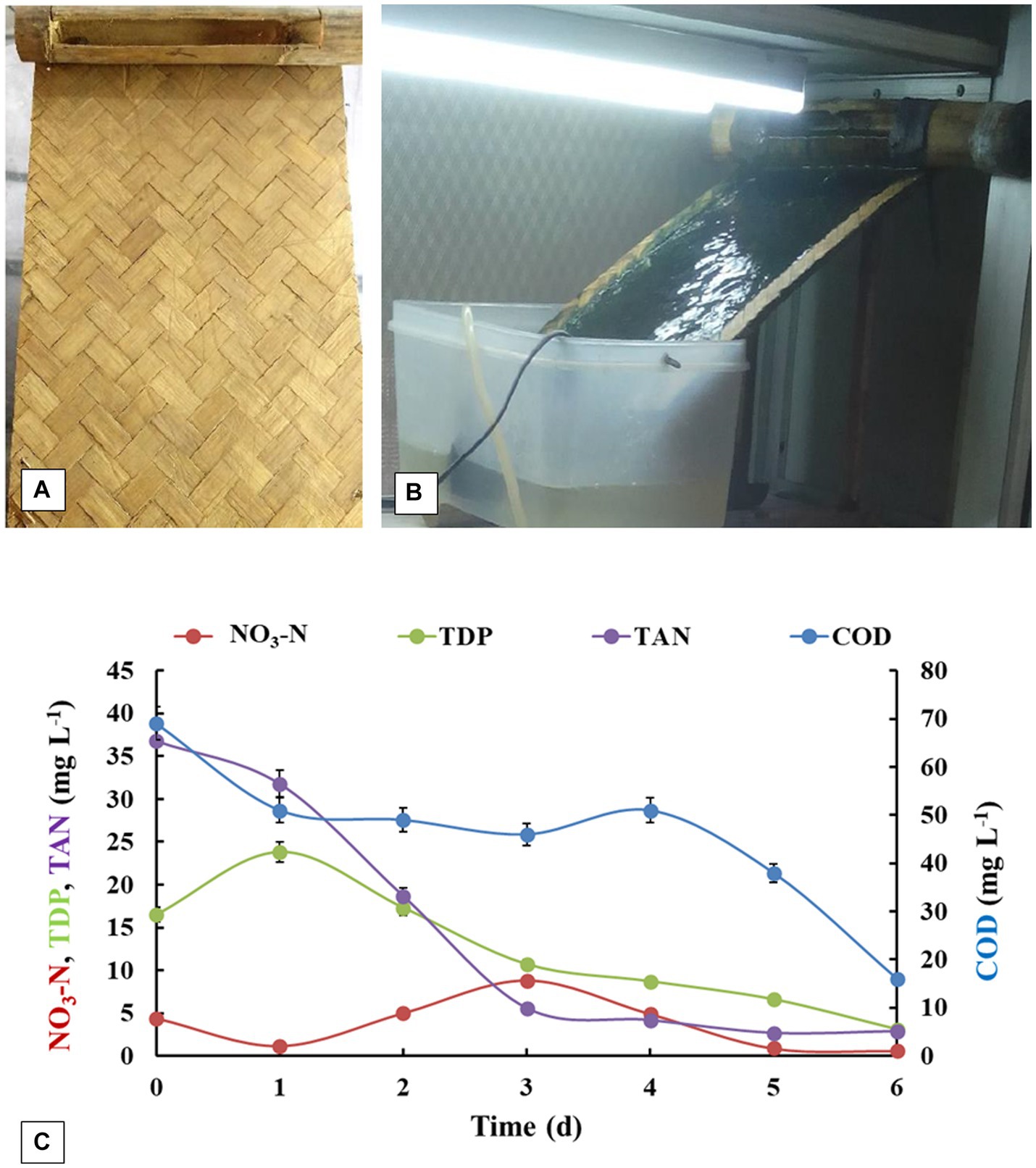
Figure 6. (A) Bamboo support material with perforated bamboo pipe. (B) Algal-bacterial biofilm grown on bamboo-based reactor. (C) Nutrient removal profiles of gray water treated in bamboo based algal-bacterial biofilm reactor. The experiment was performed in triplicates and the results are expressed in Mean ± S.E.
Researchers have used alternate support material for the microalgal biofilm growth to achieve enhanced biomass productivity and better wastewater treatment efficiency. A study depicted development of efficient biofilm on a walnut shell of Chlorella vulgaris, however this study focused on lipid accumulation in microalgal biomass instead of wastewater treatment (Zou et al., 2021). In a study, piggery wastewater was efficiently treated total phosphorus (93.90%), total nitrogen (96.5%) and ammonia (98.16%) in a rotatory porous substratum biofilm reactor (Shen et al., 2017). The reactor was initially fed with 4.5 L wastewater followed by addition of aliquot of wastewater to compensate the evaporation losses. This reactor included vertically aligned parallel plates slightly submerged into the wastewater at the bottom. These plates were made up of a rigid base and a porous medium made up of canvas on which the microalgal biomass developed the biofilm with a productivity of 52 g m−2 day−1 when initial inoculum density was 18 g m−2. The canvas medium was selected due to high hydrophobicity, water retention, and duration. The performance of different lignocellulosic biomass like pine sawdust, rice husk, oak sawdust and sugarcane bagasse was studied as biocarriers for biofilm in the flat plate attached biofilm reactor (Zhang et al., 2017). These substrates were tested to reduce the cost and durability of the biofilm support material. The results depicted pine saw dust showed the best biomass productivity of 10.92 g m−2 day−1 after 16 days of cultivation in the culture medium. This research group (Zhang et al., 2020) later studied the piggery wastewater treatment potential of attached biofilm reactor with pine sawdust as biocarrier for microalgae (Chlorella vulgaris, Hydrodictyon reticulatum, Diplosphaera sp., and Scenedesmus obliquus). This biofilm reactor system showed 68.89% removal of COD, 69.22% BOD, 95.54% Total nitrogen, 96.10% TAN, and 64.89% of total phosphorus from piggery wastewater with 5.53 g m−2 day−1 biomass productivity. The results in the present study depicted significantly higher removal efficiencies and biomass productivity in bamboo based algal-bacterial biofilm reactors.
3.4 Algae-bacterial biofilm reactors as algae farming model for integrated wastewater management and valorization in rural areas
On the basis of overall biomass and nutrient removal performance of bamboo based algal-bacterial biofilm reactor, an outdoor pilot scale production can be proposed for simultaneous wastewater treatment and mass cultivation of algal biomass. Considering the overall population and water consumption/household, a total of 96 KLD gray water is generated at selected site of Mubarakpur and transferred to agricultural fields through gravity flow. Instead of using wastewater for irrigation purposes, introducing algal biofilm-based farming requires minimum operating cost but maximum benefits. The wastewater collected in a common drain will undergo pre-filtration to remove any debris/plastic materials and can be pumped to algal-biofilm cultivation area installed near agricultural farms. With the presence of in situ harvesting system, farmers can collect wet slurry and keep for solar drying. Approximately 24 kg of dry biomass per day can be generated with nutrient rich value and can be utilized as either as feed supplement for cattle or biofertilizers in agriculture or as a feedstock for biogas generation. However, for the application as cattle feed, the wastewater grown biomass might need to undergo quality assessment in terms of presence of any toxicant. Our previous studies have indicated the potential of gray water grown biomass as biofertilizer owing to presence of minerals and high N/P ratio (Choudhary et al., 2017).
4 Conclusion
In this research, an algal-bacterial biofilm reactor with minimal resources (ecofriendly bamboo-based system) was developed which can be used as prototype bioreactors for wastewater treatment in rural areas. The PA6 consortium grown in previously developed algal bioreactor successfully accomplished treatment of wastewater collected in different seasons, with simultaneous high biomass productivity. After preliminary testing of batch scale system, algal-bacterial biofilm reactor was tested for its phycoremediation potential under fed-batch and continuous mode. Hence, it could be considered a viable technology for mass cultivation of algae-bacterial biomass using wastewater from rural areas with further applications in biogas production, cattle feed supplement, wastewater treatment and its utilization. Such interventions can trigger the local production and consumption cycles for materials and resources, thereby advancing toward sustainable development goals. Further research on algal-bacterial biofilm reactors could be targeted on long duration pilot- and demonstration-scale studies to assess the economics and sustainability of these systems for direct applications. Additionally, the quantitative and qualitative assessment of wastewater grown biomass for application in cattle feed, biofertilizer and biogas feedstock is required to make integrated process for rural areas.
Data availability statement
The original contributions presented in the study are included in the article/Supplementary material, further inquiries can be directed to the corresponding author.
Author contributions
MM: Conceptualization, Data curation, Methodology, Resources, Validation, Writing – original draft, Writing – review & editing. PC: Conceptualization, Data curation, Methodology, Validation, Writing – original draft, Writing – review & editing. SS: Conceptualization, Data curation, Methodology, Resources, Writing – review & editing. FN: Data curation, Methodology, Validation, Writing – review & editing. CT-Y: Supervision, Validation, Writing – review & editing. AM: Conceptualization, Data curation, Funding acquisition, Methodology, Resources, Supervision, Validation, Writing – review & editing.
Funding
The author(s) declare financial support was received for the research, authorship, and/or publication of this article.
Acknowledgments
MM acknowledges funding from IIT Delhi as Post-Doctoral Fellow to carry out the research (IITD/IESI/U-3/160456). The authors gratefully acknowledge Department of Biotechnology (DBT), Government of India for partial financial assistance (BT/PR46019/BCE/8/1800/2023). The authors also acknowledge the support of the Central Research Facility (CRF), IIT Delhi, India, for SEM. United States-India Educational Foundation (USIEF) and Fulbright India are gratefully acknowledged for Fulbright-Kalam (Climate) Academic & Professional Excellence Scholarship to AM at Princeton University, United States, a platform that enabled exciting discussions between AM and CT-Y.
Conflict of interest
The authors declare that the research was conducted in the absence of any commercial or financial relationships that could be construed as a potential conflict of interest.
Publisher’s note
All claims expressed in this article are solely those of the authors and do not necessarily represent those of their affiliated organizations, or those of the publisher, the editors and the reviewers. Any product that may be evaluated in this article, or claim that may be made by its manufacturer, is not guaranteed or endorsed by the publisher.
Supplementary material
The Supplementary material for this article can be found online at: https://www.frontiersin.org/articles/10.3389/frwa.2024.1430900/full#supplementary-material
SUPPLEMENTARY FIGURE S1 | (A) Satellite image (taken from Google earth) of the Mubarakpur Village, Haryana along with schematic representation of the village outlay. (B) Drain coming from village households. (C) Pond in which the gray water is flown through the drain.
SUPPLEMENTARY FIGURE S2 | Scanning electron micrograph of PA6 algal-bacterial biofilm at 10,000X.
References
Akao, P. K., Singh, B., Kaur, P., Sor, A., Avni, A., Dhir, A., et al. (2021). Coupled microalgal–bacterial biofilm for enhanced wastewater treatment without energy investment. J. Water Process Eng. 41:102029. doi: 10.1016/j.jwpe.2021.102029
Ali, N. S., Mo, K., and Kim, M. (2012). A case study on the relationship between conductivity and dissolved solids to evaluate the potential for reuse of reclaimed industrial wastewater. KSCE J. Civ. Eng. 16, 708–713. doi: 10.1007/s12205-012-1581-x
Arcila, J. S., Halloum, I., Calvo, D., Buitrón, G., and Torres, C. I. (2023). Decoupling solid and hydraulic retention times in microalgal biofilm reactors treating primary wastewater: performance and biodiesel potential. J. Chem. Technol. Biotechnol. 98, 1936–1944. doi: 10.1002/jctb.7411
Ashraf, N., Ahmad, F., and Lu, Y. (2023). Synergy between microalgae and microbiome in polluted waters. Trends Microbiol. 31, 9–21. doi: 10.1016/j.tim.2022.06.004
Beydeş, H., and Karapinar, I. (2018). Algal nutrient removal from wastewater in fed-batch operated photobioreactor. Int. J. Environ. Res. 12, 303–311. doi: 10.1007/s41742-018-0092-0
Bhattacharya, A., Mathur, M., Kumar, P., Prajapati, S. K., and Malik, A. (2017). A rapid method for fungal assisted algal flocculation: critical parameters & mechanism insights. Algal Res. 21, 42–51. doi: 10.1016/j.algal.2016.10.022
Biller, P., Ross, A. B., Skill, S. C., Lea-langton, A., Balasundaram, B., Hall, C., et al. (2012). Nutrient recycling of aqueous phase for microalgae cultivation from the hydrothermal liquefaction process. Algal Res. 1, 70–76. doi: 10.1016/j.algal.2012.02.002
Broda, M. (2020). Natural compounds for wood protection against fungi—a review. Molecules 25:3538. doi: 10.3390/molecules25153538
Calovi, M., and Rossi, S. (2023). From wood waste to wood protection: new application of black bio renewable water-based dispersions as pigment for bio-based wood paint. Prog. Org. Coat. 180:107577. doi: 10.1016/j.porgcoat.2023.107577
Chauhan, N., Kumar, P., Mishra, S., Verma, S., Malik, A., and Sharma, S. (2015). Insecticidal activity of Jatropha curcas extracts against housefly, Musca domestica. Environ. Sci. Pollut. Res. 22, 14793–14800. doi: 10.1007/s11356-015-4686-1
Chauhan, N., Malik, A., Sharma, S., and Dhiman, R. C. (2016). Larvicidal potential of essential oils against Musca domestica and Anopheles stephensi. Parasitol. Res. 115, 2223–2231. doi: 10.1007/s00436-016-4965-x
Choudhary, P., Malik, A., and Pant, K. K. (2017). Mass-scale algal biomass production using algal biofilm reactor and conversion to energy and chemical precursors by Hydropyrolysis. ACS Sustain. Chem. Eng. 5, 4234–4242. doi: 10.1021/acssuschemeng.7b00233
Choudhary, P., Prajapati, S. K., Kumar, P., Malik, A., and Pant, K. K. (2017). Development and performance evaluation of an algal biofilm reactor for treatment of multiple wastewaters and characterization of biomass for diverse applications. Bioresour. Technol. 224, 276–284. doi: 10.1016/j.biortech.2016.10.078
Choudhary, P., Prajapati, S. K., and Malik, A. (2016). Screening native microalgal consortia for biomass production and nutrient removal from rural wastewaters for bioenergy applications. Ecol. Eng. 91, 221–230. doi: 10.1016/j.ecoleng.2015.11.056
Dalvi, V., Chawla, P., and Malik, A. (2021). Year-long performance assessment of an on-site pilot scale (100 L) photobioreactor on nutrient recovery and pathogen removal from urban wastewater using native microalgal consortium. Algal Res. 55:102228. doi: 10.1016/j.algal.2021.102228
de Souza, C. G., Colares, G. S., Machado, Ê. L., and Lobo, E. A. (2019). Algae turf scrubber and vertical constructed wetlands combined system for decentralized secondary wastewater treatment. Environ. Sci. Pollut. Res. 26, 9931–9937. doi: 10.1007/s11356-019-04425-6
Delanka Pedige, H., Preethika, S., Cornelius, J., Gedara, S., Tchinda, D., Zhang, Y., et al. (2019). Pathogen reduction in an algal-based wastewater treatment system employing Galdieria sulphuraria. Algal Res. 39:101423. doi: 10.1016/j.algal.2019.101423
Delanka-Pedige, H. M. K., Abeysiriwardana-Arachchige, I. S. A., Tchinda, D., Munasinghe-Arachchige, S. P., Henkanatte-Gedera, S. M., and Nirmalakhandan, N. (2020). Algal process for single-step removal of organic carbon and nutrients: modeling and optimization of fed-batch operation. J. Water Process Eng. 38:101622. doi: 10.1016/j.jwpe.2020.101622
Fallahi, A., Rezvani, F., Asgharnejad, H., Khorshidi Nazloo, E., Hajinajaf, N., and Higgins, B. (2021). Interactions of microalgae-bacteria consortia for nutrient removal from wastewater: a review. Chem. Int. 272:129878. doi: 10.1016/j.chemosphere.2021.129878
Franchino, M., Comino, E., Bona, F., and Riggio, V. A. (2013). Chemosphere growth of three microalgae strains and nutrient removal from an agro-zootechnical digestate. Chem. Int. 92, 738–744. doi: 10.1016/j.chemosphere.2013.04.023
Gupta, S. K., Sriwastav, A., Ansari, F. A., Nasr, M., and Nema, A. K. (2017). “Phycoremediation: An eco-friendly algal technology for bioremediation and bioenergy production” in Phytoremediation Potential of Bioenergy Plants. eds. K. Bauddh, B. Singh, and J. Korstad (Singapore: Springer Singapore), 431–456.
Hasnain, M., Zainab, R., Ali, F., Abideen, Z., Yong, J. W. H., El-Keblawy, A., et al. (2023). Utilization of microalgal-bacterial energy nexus improves CO2 sequestration and remediation of wastewater pollutants for beneficial environmental services. Ecotoxicol. Environ. Saf. 267:115646. doi: 10.1016/j.ecoenv.2023.115646
Hodges, A., Fica, Z., Wanlass, J., VanDarlin, J., and Sims, R. (2017). Nutrient and suspended solids removal from petrochemical wastewater via microalgal biofilm cultivation. Chem. Int. 174, 46–48. doi: 10.1016/j.chemosphere.2017.01.107
Ji, X., Jiang, M., Zhang, J., Jiang, X., and Zheng, Z. (2018). The interactions of algae-bacteria symbiotic system and its effects on nutrients removal from synthetic wastewater. Bioresour. Technol. 247, 44–50. doi: 10.1016/j.biortech.2017.09.074
Jones, G. B., Sims, R. C., and Zhao, J. (2023). Experimental and theoretical investigations of rotating algae biofilm reactors (RABRs): areal productivity, nutrient recovery, and energy efficiency. Biotechnol. Bioeng. 120, 2865–2879. doi: 10.1002/bit.28451
Katam, K., and Bhattacharyya, D. (2021). Improving the performance of activated sludge process with integrated algal biofilm for domestic wastewater treatment: system behavior during the start-up phase. Bioresour. Technol. Rep. 13:100618. doi: 10.1016/j.biteb.2020.100618
Leonel, L. P., and Tonetti, A. L. (2021). Wastewater reuse for crop irrigation: crop yield, soil and human health implications based on giardiasis epidemiology. Sci. Total Environ. 775:145833. doi: 10.1016/j.scitotenv.2021.145833
Li, L., Wang, Y., Gao, L., Zhou, W., Chen, L., Zhang, Z., et al. (2023). Experiments and cellular automata simulation reveal light/carbon transportation and growth mechanism of Chlorella vulgaris biofilm in attached cultivation. Chem. Eng. J. 457:141177. doi: 10.1016/j.cej.2022.141177
Liu, W., Qian, J., Ding, H., Li, J., Liu, J., and Zhou, W. (2024). Synergistic interactions of light and dark biofilms in rotating algal biofilm system for enhanced aquaculture wastewater treatment. Bioresour. Technol. 400:130654. doi: 10.1016/j.biortech.2024.130654
Mohit, A., and Remya, N. (2023). Low-cost greywater treatment using polyculture microalgae—microalgal growth, organics, and nutrient removal subject to pH and temperature variations during the treatment. Appl. Biochem. Biotechnol. 196, 2728–2740. doi: 10.1007/s12010-023-04371-7
Möller, R., and Mild, G. (2019). Protection of Moso bamboo (Phyllostachys pubescens) materials against fungal decay and discolouration by treatment with wood preservatives. Eur. J. Wood Wood Products 77, 139–145. doi: 10.1007/s00107-018-1371-3
Mousavi, S. A., and Khodadoost, F. (2019). Effects of detergents on natural ecosystems and wastewater treatment processes: a review. Environ. Sci. Pollut. Res. 26, 26439–26448. doi: 10.1007/s11356-019-05802-x
Naaz, F., Bhattacharya, A., Pant, K. K., and Malik, A. (2019). Investigations on energy efficiency of biomethane/biocrude production from pilot scale wastewater grown algal biomass. Appl. Energy 254:113656. doi: 10.1016/j.apenergy.2019.113656
Nguyen, V. T., Le, V. A., Do, Q. H., Le, T. N. C., and Vo, T. D. H. (2024). Emerging revolving algae biofilm system for algal biomass production and nutrient recovery from wastewater. Sci. Total Environ. 912:168911. doi: 10.1016/j.scitotenv.2023.168911
Podola, B., Li, T., and Melkonian, M. (2017). Porous substrate bioreactors: a paradigm shift in microalgal biotechnology? Trends Biotechnol. 35, 121–132. doi: 10.1016/j.tibtech.2016.06.004
Posadas, E., García-Encina, P. A., Soltau, A., Domínguez, A., Díaz, I., and Muñoz, R. (2013). Carbon and nutrient removal from centrates and domestic wastewater using algal-bacterial biofilm bioreactors. Bioresour. Technol. 139, 50–58. doi: 10.1016/j.biortech.2013.04.008
Prajapati, S. K., Kaushik, P., Malik, A., and Vijay, V. K. (2013). Phycoremediation and biogas potential of native algal isolates from soil and wastewater. Bioresour. Technol. 135, 232–238. doi: 10.1016/j.biortech.2012.08.069
Qadir, G., Pino, V., Brambilla, A., and Alonso-Marroquin, F. (2023). Staircase wetlands for the treatment of greywater and the effect of greywater on soil microbes. Sustain. For. 15:6102. doi: 10.3390/su15076102
Qian, W., Yang, Y., Chou, S., Ge, S., Li, P., Wang, X., et al. (2024). Effect of N/P ratio on attached microalgae growth and the differentiated metabolism along the depth of biofilm. Environ. Res. 240:117428. doi: 10.1016/j.envres.2023.117428
Qv, M., Dai, D., Liu, D., Wu, Q., Tang, C., Li, S., et al. (2023). Towards advanced nutrient removal by microalgae-bacteria symbiosis system for wastewater treatment. Bioresour. Technol. 370:128574. doi: 10.1016/j.biortech.2022.128574
Renuka, N., Sood, A., Prasanna, R., and Ahluwalia, A. S. (2015). Phycoremediation of wastewaters: a synergistic approach using microalgae for bioremediation and biomass generation. Int. J. Environ. Sci. Technol. 12, 1443–1460. doi: 10.1007/s13762-014-0700-2
Rodrigues de Assis, L., Calijuri, M. L., Assemany, P. P., Silva, T. A., and Teixeira, J. S. (2020). Innovative hybrid system for wastewater treatment: high-rate algal ponds for effluent treatment and biofilm reactor for biomass production and harvesting. J. Environ. Manag. 274:111183. doi: 10.1016/j.jenvman.2020.111183
Salbitani, G., and Carfagna, S. (2021). Ammonium utilization in microalgae: A sustainable method for wastewater treatment. Sustain. For. 13, 1–17. doi: 10.3390/su13020956
Shen, Y., Yang, T., Zhu, W., and Zhao, Y. (2017). Wastewater treatment and biofuel production through attached culture of Chlorella vulgaris in a porous substratum biofilm reactor. J. Appl. Phycol. 29, 833–841. doi: 10.1007/s10811-016-0981-6
Tyagi, A. K., and Malik, A. (2010). Liquid and vapour-phase antifungal activities of selected essential oils against candida albicans: microscopic observations and chemical characterization of cymbopogon citratus. BMC Complement. Altern. Med. 10:65. doi: 10.1186/1472-6882-10-65
Vishwakarma, R., Dhaka, V., Ariyadasa, T. U., and Malik, A. (2022). Exploring algal technologies for a circular bio-based economy in rural sector. J. Clean. Prod. 354:131653. doi: 10.1016/j.jclepro.2022.131653
Wang, J., Deng, Y., Chen, W., Song, H., Zan, F., Wu, X. H., et al. (2023). Revealing the role of algae in algae enhanced bacteria consortia for municipal wastewater treatment: performance, characteristics, and microbial pathways. Journal of water. J. Water Process. Eng. 53:103640. doi: 10.1016/j.jwpe.2023.103640
Wang, J., Ying, X., Huang, Y., Chen, Y., Shen, D., Zhang, X., et al. (2021). Numerical study of hydrodynamic characteristics in a moving bed biofilm reactor. Environ. Res. 194:110614. doi: 10.1016/j.envres.2020.110614
Wardrop, N. A., Hill, A. G., Dzodzomenyo, M., Aryeetey, G., and Wright, J. A. (2018). Livestock ownership and microbial contamination of drinking-water: evidence from nationally representative household surveys in Ghana, Nepal and Bangladesh. Int. J. Hyg. Environ. Health 221, 33–40. doi: 10.1016/j.ijheh.2017.09.014
Wen, W., Zhang, Q., Jiang, Y., Zhao, R., Chen, J., Shi, Y., et al. (2024). Green modifications for rendering cotton fabric with antibacterial, anti-mite, and mosquito-repellent functions using single natural eugenol. Chem. Eng. J. 492:152194. doi: 10.1016/j.cej.2024.152194
Wu, B., Ran, T., Liu, S., Li, Q., Cui, X., and Zhou, Y. (2023). Biofilm bioactivity affects nitrogen metabolism in a push-flow microalgae-bacteria biofilm reactor during aeration-free graywater treatment. Water Res. 244:120461. doi: 10.1016/j.watres.2023.120461
Zhang, H., Li, X., An, Z., Liu, Z., Tang, C., and Zhao, X. (2021). Treatment of polyacrylamide-polluted wastewater using a revolving algae biofilm reactor: pollutant removal performance and microbial community characterization. Bioresour. Technol. 332:125132. doi: 10.1016/j.biortech.2021.125132
Zhang, Q., Liu, C., Li, Y., Yu, Z., Chen, Z., Ye, T., et al. (2017). Cultivation of algal biofilm using different lignocellulosic materials as carriers. Biotechnol. Biofuels 10, 1–16. doi: 10.1186/s13068-017-0799-8
Zhang, Q., Wang, L., Yu, Z., Zhou, T., Gu, Z., Huang, Q., et al. (2020). Pine sawdust as algal biofilm biocarrier for wastewater treatment and algae-based byproducts production. J. Clean. Prod. 256:120449. doi: 10.1016/j.jclepro.2020.120449
Zhang, L., Wang, B., Zhang, L., Lian, L., Cheng, X., Yang, Z., et al. (2023). Responses of Chlorella vulgaris to the native bacteria in real wastewater: improvement in wastewater treatment and lipid production. Environ. Pollut. 339:122737. doi: 10.1016/j.envpol.2023.122737
Keywords: algae farming, pollutant removal, rural wastewater, algae-bacterial biofilm reactor, bamboo-based reactor
Citation: Mathur M, Choudhary P, Sujathan S, Naaz F, Trenado-Yuste C and Malik A (2024) A biologically driven model for rural wastewater management: feasibility and efficiency of algal-bacterial biofilm reactors for combined treatment and algae farming. Front. Water. 6:1430900. doi: 10.3389/frwa.2024.1430900
Edited by:
Nevil Wyndham Quinn, University of the West of England, United KingdomReviewed by:
Francesco Di Capua, University of Basilicata, ItalyYonglan Tian, North China Electric Power University, China
Copyright © 2024 Mathur, Choudhary, Sujathan, Naaz, Trenado-Yuste and Malik. This is an open-access article distributed under the terms of the Creative Commons Attribution License (CC BY). The use, distribution or reproduction in other forums is permitted, provided the original author(s) and the copyright owner(s) are credited and that the original publication in this journal is cited, in accordance with accepted academic practice. No use, distribution or reproduction is permitted which does not comply with these terms.
*Correspondence: Anushree Malik, YW51c2hyZWVAcmRhdC5paXRkLmFjLmlu