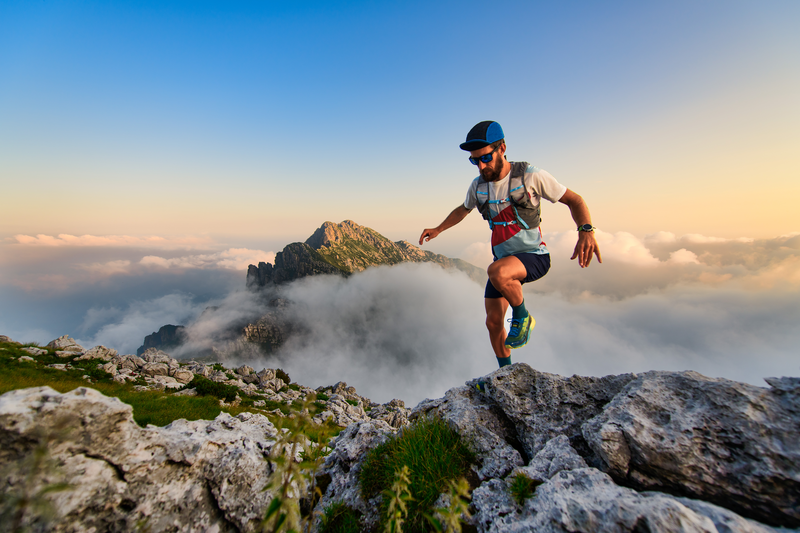
94% of researchers rate our articles as excellent or good
Learn more about the work of our research integrity team to safeguard the quality of each article we publish.
Find out more
ORIGINAL RESEARCH article
Front. Water , 01 July 2024
Sec. Water and Human Systems
Volume 6 - 2024 | https://doi.org/10.3389/frwa.2024.1408856
This article is part of the Research Topic Advances in Biomonitoring of African Aquatic Ecosystems, Volume II View all 5 articles
Introduction: Water quality assessment is a fundamental requirement for comprehensive water management. Access to water is not exclusively a quantitative issue, as human activities often lead to negative impacts on water quality due to pollution. This results in a need for the systematic monitoring of water bodies to surveil ongoing pollution dynamics and, if needed, facilitate the implementation of suitable solutions for water quality management.
Methods: To that effect, in March 2022 and July 2023, we conducted two sampling campaigns in four headwater streams in QwaQwa, South Africa, to assess the water quality under high (summer) and low (winter) flow conditions. The overarching aim was to support local decision-makers with relevant information on water quality in a semirural and less studied area. Beside the hydrological characterization of the catchment, which drains the mountainous areas around Phuthaditjhaba (the main town of the region), physicochemical and biological monitoring were conducted, including in vitro tests detecting endocrine disruption, dioxin-like impacts, and genotoxic potentials in the water.
Results: The elemental analysis showed that at several sampling sites, Al, Cu, Mn, Fe, and Zn were above the Target-Water-Quality-Range (TWQR) for aquatic ecosystems and Fe, Al, and Mn were additionally above the TWQR for domestic use. Interestingly, the organic micropollutants (OMPs) revealed a region with a high incidence of HIV infections, given the occurrence of the drugs efavirenz, its transformation product 8-OH-efavirenz and nevirapine, among other micropollutants. Possibly, the signals from the in vitro tests for endocrine disruption are also induced by the detected micropollutants. Our results ultimately show that even though anthropogenic impacts increase from upstream to downstream, none of the sampling sites are without concern. This indicates the urgent need for measures to increase the water quality of these headwater streams in this region.
QwaQwa, a former homeland area for Sesotho speaking people at the foot of the Maloti-Drakensberg Mountain range in the northeastern corner of the Free State Province, South Africa, has been plagued by water insecurity for more than two decades. Phuthaditjhaba, QwaQwa's urban center, falls under the jurisdiction of the Maluti-a-Phofung Local Municipality (MAP LM) that is responsible for providing basic services to communities, including water and sanitation. The municipality, however, has a poor record of service delivery. Service delivery protests by communities in the MAP LM have been occurring since 2004, with limited or no access to safe drinking water being one of the main grievances (Mail and Guardian, 2004; Gouws et al., 2010; Mdlalane, 2020). Nearly 80% of houses in the urban center are connected to the municipal water supply (Statistics South Africa, 2012), but this percentage is expected to be considerably lower for informal settlements on the outskirts of town and rural villages higher up in the mountains. However, access to the municipal water network does not necessarily guarantee access to clean water, since prolonged interruptions in the water supply have become a common occurrence in QwaQwa (Sekhele and Voua Otomo, 2023). Water insecurity problems came to a head during a severe drought in 2015 and 2016 that caused a drastic decline in the water levels of the Fika Patso and Metsi-Matso reservoirs, the main water supply in QwaQwa, necessitating the LM to erect communal water tanks and deliver water with hired water trucks (Macupe, 2020; Mocwagae, 2020; Mukwada and Mutana, 2023). Despite these efforts, many poor households that were unable to afford buying drinking water, were forced to rely directly on potentially polluted local rivers and streams for access to water (Mocwagae, 2020). Alarmingly, the frequency and intensity of severe droughts are expected to increase in the catchment in the immediate future (Abiodun et al., 2019; Mukwada and Mutana, 2023). Together with other risk factors such as an increasing trend in minimum and maximum temperatures (Mohamed and Mukwada, 2019), reduced streamflow in the headwater streams (Mukwada and Mutana, 2023), continued influx of people into the area (Statistics South Africa, 2012), widespread environmental degradation in the catchment (Avenant et al., 2023), high levels of poverty and unemployment (Noble and Wright, 2013; Melore and Nel, 2020), poor governance and gross mismanagement of resources (Sekhele and Voua Otomo, 2023), insufficient water infrastructure and maintenance, underperforming wastewater treatment plants and improper sewage sludge disposal (Sekhele and Voua Otomo, 2023), communities in QwaQwa remain highly vulnerable to water insecurity—even during years with average or above average rainfall.
QwaQwa may well be seen as a microcosm of the larger South Africa. The National Water and Sanitation Master Plan acknowledges that “South Africa is facing a water crisis caused by insufficient water infrastructure maintenance and investment, recurrent droughts driven by climate variation, inequities in access to water and sanitation, deteriorating water quality and a lack of skilled engineers” (Department of Water and Sanitation, 2018). The problem is especially acute at the local level where not only water scarcity, but also water quality is a source of concern. The 2022 Blue and Green Drop reports, published by the Department of Water and Sanitation (2022a,b), confirmed the poor state of drinking water services and high rate of non-compliance from municipal water treatment (WTWs) and wastewater treatment works (WWTWs) in South Africa. According to the Blue Drop report, 77% of WTWs do not comply with the required chemical standards, while 60% do not comply with microbial standards (Department of Water and Sanitation, 2022a). The Green Drop report showed that there was an overall decline in the functionality and performance of WWTWs since the previous report was published in 2013, with 39% of WWTWs being in a critical condition as compared to 29% in 2013 (Department of Water and Sanitation, 2022b). The poor quality of wastewater effluents poses a serious risk not only to public health, but also to the ecological integrity of natural water resources (Edokpayi et al., 2017; Steffen et al., 2022). This is especially concerning since surface water provides 85% of South Africa's usable water and is the main source of potable water (Colvin et al., 2016). Also, wastewater effluents are not the only source of pollution, since effluents from mining, domestic, agricultural, and industrial sources also contribute to the poor state of water quality of surface water in South Africa (e.g., Adeyinka et al., 2018, 2019; Verlicchi and Grillini, 2020). In a recent review, Verlicchi and Grillini (2020) found that the release of untreated effluents from these sources have led to the presence of a wide range of inorganic chemicals (including heavy metals), micropollutants (e.g., analgesics, antiarrythmic agents, antibiotics, antidiabetics, antihistamines, and antiseptics), macro-pollutants (e.g., nitrate, bicarbonate, and chloride), and microorganisms (e.g., pathogenic bacteria and fungi) in South Africa's surface water. Disturbingly, the concentrations of many of these micropollutants are 2–185 times higher in South African water bodies than in Europe (Verlicchi and Grillini, 2020). This state of affair makes it necessary to implement widespread water quality monitoring programmes in South Africa, especially in areas such as QwaQwa where water insecurity is more pronounced. Moreover, chemical monitoring, on its own, is deemed inadequate to reflect the cumulative and synergistic effects of water pollution from diffuse sources, which is why surface water monitoring programmes generally include response monitoring (Karr and Dudley, 1981; Karr and Chu, 1997; Roux, 2001). In South Africa, the River Eco-Status Monitoring Programme (REMP) includes measuring the present ecological state of biological responses or communities (riparian vegetation, aquatic macroinvertebrates, and freshwater fish), in addition to physical and chemical water monitoring. Regarding anthropogenic organic micropollutants (OMPs), polar and persistent substances are particularly relevant, as they can be dispersed relatively quickly, are constantly replenished due to their enormous use, and can also reach more distant ecosystems or even drinking water treatment plants due to their stability (Wilkinson et al., 2017). This also applies to their transformation products, which are usually even more hydrophilic. These environmentally problematic substances are often referred to as emerging pollutants. Their characteristics are, among others, that there are no regulations/threshold values for them so far and that they enter the water cycle mainly from domestic wastewater via wastewater treatment plants, where they are not or only partially removed. In addition, there is a high potential for these substances to cause a range of negative effects in humans and organisms, as they are often substances that are specifically intended to cause certain effects (such as pharmacological, biocidal).
Globally the number of persistent and mobile toxic substances is increasing in surface water bodies, as well as in drinking water, making it necessary to develop new analytical methods that could identify and quantify these critical chemicals in water resources (Rüdel et al., 2020). This is also true for South Africa where traditional monitoring approaches are insufficient for detecting the growing list of toxic substances in our surface waters (Kruger et al., 2022). The Global Water Research Coalition recently advocated for the integration of effect-based monitoring techniques in existing surface water monitoring programmes (Neale et al., 2022). Effect-based monitoring techniques, such as in vitro and in vivo assays, allow the investigation and evaluation of chemical substance mixtures whose composition is unknown, and to detect potential additive, synergistic or antagonistic effects of different substances in a given water sample. In vitro bioassays can also detect the effects of substances that are difficult to detect by conventional chemical analyses, or where unknown transformation products may arise from processes. The aim of this study was to investigate the suitability of in vitro bioassays for effect-based monitoring, in addition to the chemical monitoring of surface waters in the QwaQwa region, South Africa, and how this may contribute to decision-making and resource management at a local level.
QwaQwa is situated in the northeastern Free State province, bordering on the KwaZulu-Natal Province to the southeast and Lesotho on the southwest. The study area, which falls within MAP LM (Figure 1), is in a relatively narrow valley in the northern parts of the Drakensberg-Maloti Mountain range, at an elevation of between 1,675 m to more than 2,200 m above sea level. The QwaQwa area falls within the summer-rainfall region of South Africa with most of the rain occurring between October and March (Department of Water Affairs and Forestry, 2004). Rainfall is the highest in the high-altitude headwaters of the streams (mean annual precipitation >950 mm), decreasing gradually toward Phuthaditjhaba (MAP < 750 mm). Long term meteorological data (1986–2016) show that rainfall is declining, with Phuthaditjhaba receiving a mean annual rainfall of 629 mm over the last three decades (Agricultural Research Council Institute for Soil Climate and Water South Africa, 2016; Melore, 2017).
Figure 1. The Maluti-A-Phofung Local Municipality is situated in the northeastern Free State Province, South Africa.
Nine sampling sites were selected for the study on the Metsi-Matso, Mphukojwane, Namahadi, and Elands Rivers, which drain the mountainous areas around Phuthaditjhaba and the surrounding rural villages (Figure 2). The sites were chosen to represent sparsely and densely inhabited areas, relatively undisturbed and disturbed areas, upstream and downstream from potential pollution sources, and higher-lying and lower-lying areas. Site-selection criteria also included accessibility, sampling suitability and data availability.
The Metsi-Matso River is largely natural from its origin to upstream of its confluence with the Namahadi River, despite the presence of a relatively large dam in its upper reaches. Water from this stream provides 20% of the potable water for the QwaQwa area through the Metsi-Matso Dam Scheme (Free State Department of Cooperative Governance and Traditional Affairs and Chell Engineering, 2018). Two sites were sampled on the stream: Metsi-Matso Upper (MU) immediately downstream of the Metsi-Matso Dam, and Metsi-Matso Middle (MM) in the sparsely populated middle reaches where subsistence agriculture is practiced (Figure 2).
The Mphukojwane stream originates on an escarpment (at an elevation of more than 1,900 m) southwest of Phuthadithjaba. After dropping over the escarpment, it flows northwards for ~13 km through very densely populated areas to join the Metsi-Matso River from the south. Water was sampled from the upper sampling point, Mphukojwane Upper (MPU) in the relatively pristine mountain stream zone, and Mphukojwane Middle (MPM) in a densely populated area (2,792.1 people per km2; Statistics South Africa, 2012; Figure 2).
The Namahadi River is one of the main sources of freshwater in QwaQwa, supplying 85% of the domestic water required for the region (Mocwagae, 2020). Three sites were sampled on this river, Namahadi Upper (NU) in the sparsely populated upper reaches of the river, Namahadi Middle (NM) in a densely populated area (1,515.2 people per km2; Statistics South Africa, 2012), and Namahadi Lower (NL) in the very densely populated (2,495.9 people per km2; Statistics South Africa, 2012) lower reaches of the river (Figure 2).
The Elands River, which receives water from several streams originating in the mountains above QwaQwa, is situated at the outflow of the catchment. Two sites were sampled: Elands Upper (ELU) situated 2.3 km downstream of where the Mpukojwane meets the Elands River, and the most downstream sampling point, Elands Lower (ELL) situated downstream of Phuthaditjhaba (Figure 2).
Two sampling campaigns were conducted in the study area: a summer campaign between the 7th and 10th of March 2022 to reflect high flow conditions and a winter campaign between the 17th and 20th of July 2023 to reflect low flow conditions. The respective rivers were visited between 10 am and 4 pm. Samples were afterwards transported in cooler boxes (4°C) to the chemistry laboratories at the QwaQwa campus of the University of the Free State (South Africa) where they were further processed. Detailed information on the specific procedures and techniques followed for each aspect of the study are provided below.
Physico-chemical parameters were measured during field sampling conducted at the nine sampling locations. A portable multi parameter reader (SensoDirect 150—Lovibond) was used for onsite measurement of pH, dissolved oxygen, electrical conductivity, total dissolved solids, redox potential, and temperature. Samples for chemical oxygen demand and other water quality parameters that could not be measured onsite, were collected in 1L amber bottles to avoid photodegradation and transported to the chemistry laboratories of the UFS in cooler boxes.
For interpretation, the measured values were compared to the South African Water Quality Guidelines for domestic use (Department of Water Affairs and Forestry, 1996a) and the In-stream Water Quality Guidelines for the Elands River as set by the Upper Vaal Catchment Management Forum (2022). The In-stream Water Quality Guidelines determined ranges for selected parameters (including Electrical conductivity, pH, Chemical oxygen demand, Chloride, Fluoride, Nitrate, Phosphate, and Sulfate) for four categories: Ideal, Acceptable, Tolerable, and Unacceptable.
A Pearson correlation matrix was used to calculate the correlations between selected physico-chemical parameters for the respective years (significance p < 0.05).
Solid phase extraction (SPE) of surface water samples was performed to reduce matrix effects and to concentrate micropollutants in the diverse water samples. For the SPE, an aliquot of 1,000 mL surface water was filtered through glass fiber filters (< 0.7 μm, 50 mm, Sartorius, Germany) using a vacuum manifolder and at a low underpressure (−200 mbar). Hydrophilic lipophilic balanced cartridges (HLB 200 mg 6 cc, Waters Oasis) were conditioned with 5 mL acetone (analytical grade, purity > 99.8%, Honeywell/Riedel-de Haën, US), 5 mL methanol (analytical grade, purity > 99.9%, Honeywell/Riedel-de Haën, US), and 5 mL ultrapure water (CHROMASOLV™ Plus for HPLC, Honeywell/Riedel-de Haën, US). After conditioning, filtered water samples were loaded onto the HLB cartridges with a flow rate of 4.0–5.5 mL/min and subsequently washed with 5 mL ultrapure water. Loaded cartridges were gently dried under a nitrogen stream (purity 99.5%, Afrox, South Africa) and afterwards stored and cooled until elution.
After transport to the laboratories at the Technical University of Dresden (Germany), the elution of the cartridges was performed in the following way: eluted with 2 mL acetone (ROTISOLV® HPLC, analytical grade, purity > 99.9%, Carl Roth GmbH, Germany) and 2 mL methanol (ROTISOLV® HPLC Ultra Gradient Grade, purity > 99.95%, Carl Roth GmbH, Germany), further, completely dried under a gentle nitrogen stream, diluted using 100 μL dimethyl sulphoxide (DMSO; ROTIPURAN® ≥99.8%, p.a., Carl Roth GmbH, Germany). The diluted eluates were stored in 1.5 mL glass vials at −20°C until testing in the enrichment factor of 10,000.
Yeast-based reporter-gene assays for endocrine activities are described in detail by Carstensen et al. (2023) based on the ISO 19040-1 (2018). The measured fluorescence values were normalized by the corrected optical density. The equivalence concentrations (EQ, e.g., 17ß-Estradiol equivalence E2-EQ in ng/L) were derived from concentration-effect curves of the reference compounds. The concentration-effect curves of the reference compound were fitted by using a four-parametric logistic function. The biological equivalent concentrations of each technical replicate of the respective sample were calculated by inserting the associated corrected fluorescence value and the derived parameters (curve point of inflection (EC50), Hillslope, bottom, and top curve plateau) in the inverse of the four-parametric logistic function. The results were divided through the final enrichment factor of 20.834. The limit of quantification (LOQ) was calculated based on the variability of the corrected fluorescence values of all negative controls. The LOQ was derived by inserting the mean response of all negative control values plus (YES, YAS, YDS) respectively minus (YAES, YAAS) 3-fold the standard deviation (SD) of the mean response of all negative control values in the inverse of the four-parametric logistic function of the respective reference compound. The derived LOQ value was also divided by the final enrichment factor of 20.834. All calculations were performed with the statistical software R (version 4.2.1, 2022-06-23) and drc-package (Ritz et al., 2015). For a better overview of the results a green-yellow-red color scheme was applied to the results. EQ values below LOQ were assessed as low (green), EQ values above LOQ, but below three times the LOQ were assessed as moderate (yellow) and EQ values above three times the LOQ were assessed as high (red).
The mutagenicity of native and extracted wastewater samples was assessed using the Ames fluctuation test (ISO 11350, 2012; Organization for Economic Cooperation and Development, 2020) with the Salmonella typhimurium strains TA98 (detection of frameshift mutation) and T100 (detection of base pair substitution). The research group of J. Oehlmann (Department Aquatic Ecotoxicology, Goethe University Frankfurt am Main, Germany) kindly provided the strains used. Ames fluctuation tests were conducted as described by Magdeburg et al. (2014). For testing extracted samples, overnight cultures were diluted to 180 (TA98) and 45 (TA100) formazine attenuation units in the assay medium. The 10,000-fold enriched surface water samples were diluted 1:250 in the Ames test, which resulted in a final enrichment factor of 40. The positive control was carried out using 4-Nitro-Phenylenediamine (4-NOPD, CAS 99-56-9, Sigma-Aldrich, Germany) for TA98 and nitrofurantoin (NF, CAS 67-20-9, Sigma-Aldrich, Germany) for TA100. DMSO was used for solvent control of the extracted samples.
For the statistical analysis of differences in the number of wells with revertant growth per 48-well area of the samples compared to the negative control, Pearson's Chi-squared test with Yates' continuity correction (Yates' chi-square test) were used.
For testing the genotoxicity of wastewater, the in vitro mammalian cell micronucleus test MNvit (Reifferscheid et al., 2007; DIN EN ISO 21427-2, 2009; Organization for Economic Cooperation and Development, 2016) was conducted with human hepatoma cells (HepG2; Sommaggio et al., 2018). HepG2 cells were obtained from the Leibnitz institute DSMZ (German collection of Microorganism and cell culture, cell no. ACC-180, Braunschweig, Germany) and cultured in RPMI1640 medium (with l-glutamine; ROTI®Cell RPMI-1640 CELLPURE®, Carl Roth GmbH, Germany) with 10% newborn calf serum (heat activated; Sigma-Aldrich, Germany) and 1% penicillin/streptomycin (WFI solution; Biochrom, Germany) at 37°C and 5% CO2. For MNvit test, HepG2 cells were used between passages 6–15 and seeded (6 × 104 cells/mL) onto adhesive microscope slides (Superfrost® UltraPlus by Menzel, Carl Roth GmbH, Germany) in four-well microtiter plates for an incubation period of 24 h. Cells were then treated with three different dilution levels of the 10,000 fold enriched surface water samples: DS.1: dilution 1:1000, DS.2: dilution 1:2000 and DS.3 dilution 1:4000. This dilution with medium and DMSO diluted the 10,000-fold enriched water samples to subsamples with final enrichment factors of 10, 5, and 2.5, respectively. For the negative solvent controls 4.95 mL medium and 50 μl DMSO were used. For the positive control 3.95 mL medium, 1 mL ethyl methanesulfonate (EMS, CAS 62-50-0, Sigma-Aldrich, Germany), 1.75 mg EMS/mL in medium, and 50 μL DMSO were used. All treatments were tested in duplicates. After 24 h of exposure, the cells were fixed and stained for microscopy as described in the DIN EN ISO 21427-2 (2009) guidelines. The fixing solution consisted of ethanol (ROTIPURAN® ≥ 99.8%, p.a, Carl Roth GmbH, Germany), acetic acid glacial (99.8–100.5%, AnalaR® NORMAPUR® ACS, Reag. Ph. Eur. analytical reagent, VWR Chemical) and formaldehyde (37% solution, VWR chemicals). For staining a 5% Giemsa solution (Giemsa stock solution, Carl Roth GmbH, Germany) was prepared using buffer tablets (pH 7.2; Merck KGaA).
The analysis was performed microscopically and mono-, di-, tri- to tetra- and multi-nucleated cells as well as micronucleus per dinucleated cell were counted. Approximately 500 cells per slide, two slides per sample and control as well as three different concentrations per sample were counted, thus, ~1,000 cells per control and 3,000 cells per sample were analyzed.
For statistical analysis, the results of the two slides per treatment were summed and standardized to micronuclei per 1,000 cells. For the statistical analysis of differences in the number of micronuclei per 1,000 cells, the Pearson's Chi-squared test with Yates' continuity correction (Yates' chi-square test) was used. Additionally, the results were compared to the historical negative control range of the Institute of Hydrobiology at the Technical University of Dresden (Germany).
All the chemicals used in the analyses were of analytical grade. Hydrochloric acid and nitric acid were obtained from VWR chemicals. Water (Fisher Scientific GmbH), acetonitrile (Th. Geyer GmbH and Co. KG) and acetic acid (LiChropur, Merck KGaA), used for LC-MS/MS measurements and the corresponding sample preparation, were of LC/MS grade. The selection of the organic micropollutants (OMPs) investigated by means of target analysis was based on a frequent and global occurrence in the water cycle, with typical representatives of pharmaceuticals, personal care products, industrial chemicals, sweeteners, pesticides, as well as biocides. While only 35 OMPs were included in the analysis for the first sampling campaign March 2022, the range of substances was significantly expanded to include 114 substances for the second campaign in July 2023. Analytical standards of these compounds and internal isotopically labeled standards were of analytical purity (manufacturers and suppliers are listed in Supplementary Tables 1, 2).
Immediately after sampling, filtration was performed at the sampling sites according to the procedures given below.
A 6 mL syringe with attached syringe filter (CHROMAFIL Xtra, regenerated cellulose, 25 mm, 0.2 μm) was pre-rinsed five times with sample water. Then, 6 mL of sample water were filtered in a 40 mL glass vessel (rinsed five times in advance with sample water) for further use. Exactly 5 mL of the filtered sample water were transferred into a 12 mL glass vessel (pre-flushed with sample water). After addition of 100 μL of internal standard mixture (concentration of each reference compound was 5 μg/L, list of all internal standards provided in Supplementary Table 2) and intensive shaking, 5 mL of the water sample was filtered through the pre-loaded filter. The first 4 mL were put into the waste, the last 1 mL was collected into a designated 2 mL HPLC vial which were stored in a cool (4°C) and dark place until analyzed in the laboratory.
A 20 mL syringe was filled with sample water and attached to a syringe filter (1.0/0.45 μm, glass fiber/PET, Macherey-Nagel). After flushing the syringe filter with 2 mL of sample water, at least 10 mL of water sample were filtered into a 15 mL plastic vessel. The samples were not acidified or treated with biocides.
A 20 mL syringe was filled with sample water and attached to a syringe filter (1.0/0.45 μm, glass fiber/PET, Macherey-Nagel). After flushing the syringe filter with 2 mL of sample water, 40 mL of water sample were filtered into a 50 mL PE vessel. The filtered sample was acidified with HCl to reach a pH of ~2 (controlled with pH indicator test trips).
A 20 mL syringe was filled with sample water and attached to a syringe filter (1.0/0.45 μm, glass fiber/PET, Macherey-Nagel). After flushing the syringe filter with 2 mL of sample water, 10 mL of water sample were filtered into a 15 mL PE vessel. The filtered sample was acidified with HNO3 to a pH lower than 2 (controlled with pH indicator test trips). All sample vials were stored in the dark and at 4°C until laboratory analysis.
The main anions fluoride, chloride, nitrite, bromide, nitrate, sulfate, and phosphate were analyzed by ion chromatography (INTEGRION HPIC, Dionex/Thermo Fisher Scientific) following the unit method EN ISO 10304-1 (2007). The determination of 21 elements, dissolved in water, was carried out by means of Inductively Coupled Plasma Optical Emission Spectrometry (ICP-OES; AVIO 200, Perkin Elmer) according to EN ISO 11885:2007 (2007). The dissolved organic carbon (DOC) was determined as non-purgeable organic carbon with a TOC-5,000 (Shimadzu) according to the standard DIN EN 1484:2019-04 guidelines. The measurement of the spectral absorption coefficient at 254 nm (SAC254, determination of absorption in the UV radiation range) in accordance with DIN 38404-3:2005-2007 was used as a further summary method for the determination of dissolved organic substances, in which molecules with multiple bonds or aromatic compounds, in particular, are detected.
The analytical determination of 35 and 114 trace organic pollutants (from the 2022 and 2023 sampling campaign, respectively) was carried out via UHPLC system (Nexera X2, Shimadzu) with the chromatographic column Waters AQUITY Premier HSS T3; 1.8 μm VanGuard FIT (100 × 2.1 mm) and the corresponding precolumns in combination with a tandem mass spectrometer (Triple Quadrupol-MS QTRAP 6,500+ detector, Sciex) according to a method developed at the Institute of Water Chemistry, Technical University of Dresden (Germany), for more details see Supplementary Table 3. Due to the high sensitivity of the mass detector used, OMPs analysis could be performed directly without enrichment. The injection volume was 50 μl. The compound separation was performed at a constant flow rate of 0.3 ml/min using a water/acetonitrile gradient (Supplementary Table 3). Each eluent was supplemented with acetic acid (0.04% v/v) and with and without buffering (acetic acid) depending on the type of OMP. Analytical standard mix of the OMPs (10 μg/L) was prepared from stock solutions in water/methanol (50% v/v) and diluted for quality control standards (QC, 0.1 μg/L) for measurement. Internal standard mix (IS) of isotopically labeled compounds was prepared equally and all solutions were stored at −18°C. The calibration was carried out for all substances in the range from 10 to 2,500 ng/L. Detection of micropollutants was performed in ESI(-) or ESI(+) mode according to optimized ionization conditions for each analyte. The quantitative determination of masses was carried out in MRM measurements. For more details on the mass transitions of the analytes, and their limits of quantification, compare with Supplementary Table 1.
Analogous to the yeast-based reporter-gene assays, a green-yellow-red color scale was used for a general assessment of the contamination of the streams. In the absence of drinking water or environmental limits for the selected OMPs, the critical threshold was considered to be an exceedance of the Health-oriented Guidance Value (HGV). The approach for these HGVs was developed by the German Federal Environment Agency (Umweltbundesamt, 2003) for an initial assessment of the trace substance levels detected. The basis of this concept is the general precautionary value of 100 ng/l for previously unevaluated or only partially evaluated substances in drinking waters, which is derived from the studies by Dieter (2014). For some substances for which more extensive toxicological data are available, the HGV values have since been corrected; in the case of some of investigated active pharmaceutical ingredients, e.g., carbamazepine (0.3 μg/l), diclofenac (0.3 μg/l), and ibuprofen (1 μg/l), an upward adjustment was made. The following classification was made for the green-yellow-red color scheme: Concentrations exceeding the critical HGV threshold were marked in red, values below the HGV threshold were colored in yellow, and instances where no substances were detected, or the measured values were below the working range of the calibration, were marked in green.
For elemental analysis, water samples were collected using 500 mL plastic bottles, pre-treated with 10% nitric acid. They were then kept on ice during transportation to the laboratory and stored at 4°C before they were sent for analysis. Analysis was conducted by an accredited commercial laboratory (ISO/IEC 17025:2005), WaterLab (Pty) LTD, based in Pretoria, South Africa. Following standard techniques, the water samples were analyzed to determine the concentrations of 66 elements, including heavy metals, and micro and macro nutrients using an Inductively Coupled Plasma Optical Emission Spectrometer (ICP-OES; Perkin Elmer, Optima 2100 DV). Analytical accuracy was determined using certified standards (De Bruyn Spectroscopic Solutions 500MUL20-50 STD2), and recoveries were within 10% of certified values. The Target Water Quality Ranges (TWQRs) suggested by the South African Department of Water Affairs and Forestry (DWAF) for selected elements (Department of Water Affairs and Forestry, 1996a,b) were used to evaluate and interpret the quality of the water samples. Department of Water Affairs and Forestry (1996a,b) defines the TWQRs as a “management objective which has been derived from quantitative and qualitative criteria.” If a concentration is below the TWQR value for a respective chemical parameter, it indicates that the element would rarely affect aquatic life. However, at a concentration equal to TWQR but below the Acute Effect Value (AEV), the aquatic organisms could occasionally be affected by the pollutants. TWQR values for aquatic ecosystems (AE), and domestic use (DU) were compared with measured concentrations following the two sets of DWAF guidelines.
The values for most of the measured parameters increased from upstream to downstream (Table 1). Whereas, electrical conductivity, fluoride, chloride, and nitrite measurements recorded in the upper and middle reaches in the Namahadi and Metsi-Matso Rivers were Ideal according to the In-stream Water Quality Guidelines (Upper Vaal Catchment Management Forum, 2022), measurements in the Mphukojwane and the Elands River varied between Acceptable and Unacceptable. Chloride and nitrate values were Unacceptable at MPM, ELU, and ELL, while COD values were Unacceptable in summer (2022) and Tolerable in winter (2023). Compared to the upper reaches of the streams, parameters such as DOC, electrical conductivity, and total nitrogen (TNb) increased by factors of 7, 50, and 64, respectively, in the lower-lying, urbanized areas (Supplementary Figures 1A–C).
Table 1. Physico-chemical characteristics of water samples collected at the sampling sites during the summer (March 2022; S) and winter (July 2023; W) campaigns.
Mphukojwane stream was heavily impacted by poor water quality, with the highest electrical conductivity, chloride, nitrate, and sulfate values being recorded at MPM (Table 1). Here (at MPM) electrical conductivity, chloride, nitrate, and sulfate values were Unacceptable in both summer and winter (except for electrical conductivity, which was Acceptable in winter). Water quality at the two most downstream sites, ELU and ELL, located in the Elands River below the confluence with the Mphukojwane, was also heavily impacted compared to the upstream sites.
Differences were also evident between the summer and the winter samples (see Table 1). As expected, water temperatures were higher in summer than in winter, and higher at downstream than at upstream sites—but only in summer. In winter, water temperatures were higher at the upper sites compared to the downstream sites. pH, electrical conductivity, fluoride, SAC254, and COD were higher in summer (2022), but a clear seasonal trend was not observed for the other parameters. For example, nitrate values were higher for the summer samples collected from the Metsi-Matso and Elands Rivers, but lower for the samples from the Namahadi and Mphukojwane Rivers. Nitrite values were below the limit of detection for all the sites, except at the middle Mphukojwane site where it was detected in summer.
Correlations between the important water quality parameters are shown for the summer (Figure 3) and winter (Figure 4) samples. Significant relationships (p > 0.05) were observed between water temperature, pH and total phosphorous in summer (Figure 3), and between temperature, electrical conductivity, chloride, nitrate, and sulfate in winter (Figure 4). As expected, the parameters that are typically known as indicators of wastewater show a significant relationship with each other. This applies, for example, to chloride, electrical conductivity, the total organic content (measured as COD, TOC, or DOC), and the SAC254 value (which stands for the aromaticity of the organics, as well as nitrogen and phosphorus values).
Figure 3. Significant correlations identified between the measured physico-chemical parameters for the summer samples collected in 2022. Illustrated circles are significant within a significance level of p < 0.05. Filled circles: dark color indicates a negative correlation; light color indicates a positive correlation; circle area corresponds to the strength of the correlation. Not significant correlations are not depicted.
Figure 4. Significant correlations identified between the measured physico-chemical parameters for the winter samples collected in 2023. Illustrated circles are significant within a significance level of p < 0.05. Filled circles: dark color indicates a negative correlation; light color indicates a positive correlation; circle area corresponds to the strength of the correlation. Not significant correlations are not depicted.
The results of yeast-based estrogenic screen (YES) show for 2022 an increased estrogenic activity for the samples of site ELU (2.0 ± 0.91 E2-EQ/L) and MPM (2.0 ± 0.26 ng E2-EQ/L; Table 2). This was also true for 2023, with values of 8.8 ± 2.5 E2-EQ/l and 3.4 ± 0.3 ng E2-EQ/l, respectively. Additionally, the site ELL with 12.0 ± 3.0 ng E2-EQ/l as well as NL (1.5 ± 0.3 ng E2-EQ/l) and NM (1.1 ± 0.1 ng E2-EQ/l) were of concern (Table 3). These values are three times higher compared to the calculated LOQ (0.4 ng E2-EQ/L); thus, the estrogenic potential was categorized as high for these sites.
Table 2. Overview of the results of the in vitro-tests to determine mutagenic (Ames), genotoxic (Micronucleus), and endocrine potential (yeast-based tests), for summer samples (2022).
Table 3. Overview of the results of the in vitro-tests to determine mutagenic (Ames), genotoxic (Micronucleus), and endocrine potential (yeast-based tests), for winter samples (2023).
In 2022, during the rainy summer, for none of the sampling sites anti-estrogenic (YAES), androgenic (YAS) or anti-androgenic (YAAS) activities above the LOQs could be detected (Table 2). In 2023, during the dry winter, the sampling site ELL showed androgen potential in a concentration of 27.5 ± 3.4 ng/L T-EQ and ELU with 31.7 ± 3.6 ng/L T-EQ, which were above the LOQ (5.4 ng T-EQ/L; Table 3).
In 2022, the yeast dioxin-like screen revealed a highly increased activity for the sample of site ELU with 727.9 ± 96.5 ng β-NF/L (Table 2). This value significantly exceeded the value of 3 times LOQ. The dioxin-like potential of the sample was, therefore, considered high. The samples of the sites MPM, NL, NM and MU showed mean values β-NF-equivalent concentrations above but close to the LOQ of 110.4 ng β-NF/L. The dioxin-like potential of these samples was, therefore, rated as moderate. The mean values of β-NF-equivalent concentrations of the samples from site MPU, NU and MM were below the LOQ. The samples of site NU and MM are rated as cytotoxic.
In the campaign 2023, again the site ELU and additionally the site ELL revealed highly increased cytotoxic activity with concentrations of 402.7 ± 71.7 ng β-NF/L and 205.1 ± 23.9 ng β-NF/L, respectively (Table 3). All other sites were below the LOQ with 17.8 ng β-NF/L.
In the Ames-test none of the samples showed significant increase of the number of wells with revertant growth compared to negative control with both test strains TA98 and TA100 (Yates' chi-square test, p > 0.05). Thus, there was no evidence of mutagenic potential in any of the samples from both 2022 and 2023.
Furthermore, none of the samples from 2022 show a significant increase in micronuclei/1,000 cells compared to the negative control (p > 0.05) at any of the dilution levels except for the highest enrichment level of the sample of site NL (NL-DS.1, Yates' chi-square test, p-value = 0.03; Table 2). The higher dilution levels of this site which are closer to the real sample, however, show no increased number of micronuclei/1,000 cells compared to the negative control (NL-DS.2: p = 0.5 and NL-DS.3: p = 0.3). Overall, none of the samples clearly showed genotoxic potential. Similarly, none of the samples from 2023 show a significantly increased number of micronuclei/1,000 cells compared to the negative control (p > 0.05) at any of the dilutions (Table 3).
In general, a comparison of the concentrations of the respective equivalents showed that at low water levels (i.e., in the winter of 2023), there were more sampling sites of concern than in high water levels (i.e., in the summer of 2022).
As expected, the rivers Metsi-Matso, Namahadi, and Mphukojwane showed hardly any metal pollution in the upper reaches (especially at the MU, NU, and MPU sampling sites) during the summer (2022) campaign. However, with increasing urban settlement, a significant input of some organic trace substances such as sulfamethoxazole, BP-4, ibuprofen, carbamazepine, atenolol, nevirapine, efavirenz or its metabolite 8-OH-efavirenz, and the classical indicator of human influence, caffeine, were observed during the summer campaign (2022). The MPM, ELU, and ELL sampling sites were particularly strongly affected (Figure 5). Up to the last sampling point in the direction of flow (ELL, situated downstream of the confluence with the above-mentioned streams), no reduction in substance concentrations was detected, which is certainly related to the comparatively short flow distances and high population density. It is also clear from Table 4, that contamination with OMPs was concentrated at the downstream sampling locations, all of which were within, or downstream of, the human settlements.
Table 4. General overview of detected OMPs for summer samples (2022), green: below 10 ng/L (mostly analytical limit of quantification LOQ), yellow: higher LOQ, lower Health-Oriented Guidance Value (HGV), and red: exceeding the HGV.
A total of 14 of the 35 selected OMPs were not detected at all, and the concentrations of 6 other substances (including atrazine, BP-3) were below the limits of quantification or below 10 ng/L. The highest concentrations were determined for BP-4, caffeine and 8-OH-efavirenz with maximum values of 262, 217, and 195 ng/L, respectively.
There were some sporadic, in part relatively high concentrations of acetochlor (404 ng/L only at the ELL sampling point), fipronil (45 ng/L at ELU), and bisphenol A (68 ng/L at ELL). For diclofenac, DEET, propinconazole, and BP, there were no occurrence at any sites, with all concentrations below detection limit.
As with the first sampling in the summer of 2022, a significant increase in the concentrations of OMP downstream of all streams was recorded in the winter of 2023. The highest levels were measured for the antidiabetic agent metformin (up to 9,000 ng/L), the sweeteners saccharin (4,500 ng/L), acesulfame (3,300 ng/L) and sucralose (1,500 ng/L), caffeine (1,300 ng/L), transformation product of the antiretroviral agent efavirenz), and the analgesic ibuprofen (1,000 ng/L) at the ELL, ELU, and MPM sites. The concentrations of several other trace substances, such as efavirenz (antiretroviral agent), the antibiotics erythromycin, and sulfamethoxazole, as well as BP-4 (UV blocker), were in the upper ng/L range at these sites. Of the total of 114 substances considered in this second campaign, Figure 6 shows the concentration curves of the detected OMPs with the highest concentrations for all sites (the concentration curves of all detected OMPs are shown in Supplementary Figures 2A–D). The concentrations of 60 substances were above the limit of quantification, which corresponds to 63% of the total. Accordingly, in addition to the absolute concentrations of OMPs, the relative proportion of positive findings also increased compared to the 2022 summer campaign. Both aspects can also be compared well in the general schematic representation in Tables 4, 5.
Table 5. General overview of detected OMPs for winter samples (2023), green: below 10 ng/L (mostly analytical limit of quantification LOQ), yellow: higher LOQ, lower Health-Oriented Guidance Value (HGV), and red: exceeding the HGV.
As pesticides are rarely used intensively in agriculture in the QwaQwa region, their occurrence in the analyzed streams is of minor importance compared to other OMPs, especially pharmaceuticals. However, individual pesticides may be of seasonal importance in the lower reaches of the streams (such as pre-emergent herbicide acetochlor, herbicide bromacil). In contrast to the concentration trends of all other OMPs along the course of the rivers, an unusually high concentration of bromacil (~700 ng/L) was determined at MPM site. It is possible that this herbicide, which is used to control annual and perennial grasses and broad-leaved weeds, was used locally in considerable quantities, and might not be detected in follow-up monitoring (Supplementary Figure 2B).
During the 2022 sampling, aluminum (Al) concentrations were higher than the permissible DWAF TWQR limits for aquatic ecosystems, except for the two sampling sites, NU and MM (Table 6). However, in 2023, all sampled sites had Al concentrations above the TWQRs (Table 7). The concentration of Al ranged from below the detection limit to 0.194 mg/L in 2022 and from 0.173 to 0.540 mg/L in 2023. In 2022, low arsenic (As) concentrations of 0.001 mg/L were only quantified in two sampling sites (ELU and NL), while as concentrations were below detection limits in all the other samples. In 2023, As was below detection limits in all samples. Calcium (Ca) concentrations range between 7 and 23 mg/L in 2022, with the highest value recorded at ELU. All Ca concentrations were below the thresholds of 32 mg/L suggested in DWAF guidelines. A similar trend was seen in 2023 where Ca levels ranged from 4 to 24 mg/L. Copper (Cu) concentrations were above the TWQR for aquatic ecosystems in most sampling sites except for NM and the ELU. All recorded Cu concentrations were lower than the TWQR value for domestic use, with values ranging from 0.001 to 0.006 mg/L. However, in 2023, Cu levels were all below the detection limit, making them less than all the TWQRs. Iron (Fe) levels below the recommended TWQR value for domestic use were only recorded at one site, NU, during both seasons. The NU sampling point had the lowest Fe concentration of 0.063 and 0.051 mg/L in 2022 and 2023, respectively. While the ELU sampling point had the highest recorded Fe value of 2.140 mg/L in 2022, in 2023 it was MPM with 0.765 mg/L. A sample from the MPU had the highest magnesium (Mg) concentration of 10 mg/L. Mg was detected in all samples; all recorded concentrations were less than the TWQR values for domestic use. A single manganese (Mn) concentration higher than the TWQR for aquatic ecosystems and the TWQR for domestic use was recorded in the water sample collected from the ELU sampling site during 2022 sampling. However, in 2023, the MPM point had a Mn value exceeding the TWQR for aquatic ecosystems. Table 7 also shows that zinc (Zn) concentrations were above the recommended range when compared to the TWQR values for the aquatic ecosystem (i.e., < 0.002 mg/L) in all the samples in 2022, but below the TWQR value for domestic use. However, in 2023, Zn levels were below the detection limit at all sampled sites.
Table 6. Metal content for summer samples (2022) compared with Target Water Quality Range (TWQR) values for aquatic ecosystems and domestic use.
Table 7. Metal content for winter samples (2023) compared with Target Water Quality Range (TWQR) values for aquatic ecosystems and domestic use.
QwaQwa is situated in one of the major strategic source areas of the Vaal River (Nel et al., 2017). The Vaal River supplies Gauteng, South Africa's economic powerhouse contributing more than 33% to the national Gross Domestic Product, with water and is, therefore, very important for job creation and economic development (Fourie, 2023). The ecological integrity and water quality of headwater streams, such as the Namahadi, Metsi-Matso, and Mphukojwane that feeds into the Elands River and then into the Wilge River, a major tributary of the Vaal River, is therefore of the utmost importance (Department of Water and Sanitation, 2014). The water quality of these streams is affected by both natural (e.g., seasonal trends, underlying geology) and anthropogenic (e.g., urbanization, agriculture) factors (Du Plessis, 2017).
The results showed clearly that water quality deteriorated from upstream to downstream in the catchment. Although the sampling sites in the upper reaches of the Metsi-Matso and Namahadi Rivers were not pristine (e.g., presence of alien trees, sedimentation, and trampling by small stock; see Avenant et al., 2023), the water quality was good during both summer and winter. The negative impacts of anthropogenic activities on the streams were, however, clearly noticeable. Electrical conductivity, chloride, nitrate, and sulfate values increased progressively along the river courses as the surrounding areas became more urbanized and densely populated, resulting in unacceptable high chloride and nitrate levels at the most downstream sites, ELU and ELL. The chemical oxygen demand at these sites was also unacceptably high during summer when high river flows generally occur. The poor water quality is most probably related to the accumulated effect of uncontrolled waste disposal (e.g., disposable diapers) and industrial and sewage effluent discharges along the rivers. Wastewater treatment plants in QwaQwa are in a poor condition, leading to improper sewage sludge disposal and raw sewage being released into the rivers (Department of Water and Sanitation, 2022a; Sekhele and Voua Otomo, 2023).
The Mphukojwane stream was heavily impacted by poor water quality. Electrical conductivity, chloride, nitrate, sulfate, and COD values were unacceptably high in the middle section of the stream (at sampling site MPM). The stream, which flows through some of the most densely populated areas in Phuthaditjhaba (see Section 2.1.2), is highly impacted by urban runoff and pollution (Motholo, 2014). The high electrical conductivity values recorded in the summer of 2022 (more than double the values recorded during the winter of 2023) points toward urban pollutants being washed into the river by the heavy rainfall experienced before and during summer sampling campaign. River habitat assessment also showed that the riparian habitats of the Mphukojwane are heavily impacted by uncontrolled solid waste disposal, bank erosion, the removal of riparian vegetation, and sand mining (Avenant et al., 2023), all of which could contribute to poor water quality.
The measured values for some general and summary parameters recorded in summer 2022 are in a similar order of magnitude (e.g., DOC, chloride; Supplementary Figures 3A, B) or even in higher ranges (SAC254, EC; Supplementary Figures 3C, D) compared to winter 2023. This can be explained by the considerable leaching of Natural Organic Matter (NOM), or ions, from soils and sediments because of heavy precipitation in summer. Increased degradation of natural organic water constituents is also possible at higher temperatures but can only be recorded via the summary parameters DOC and SAC254 in the event of complete degradation (mineralization). Pedogenic humic substances with higher aromaticity, which are reflected by SAC254 (Albrektiene et al., 2012), are considered to be relatively stable (Gerke, 2018). The input of NOM through precipitation thus dominates over its (complete) degradation.
A number of significant correlations were observed between selected water quality parameters (Figures 3, 4). The importance of these correlations should, however, not be overestimated. For example, the correlations between temperature and parameters such as pH, conductivity, or total phosphorus, require careful interpretation considering the limited nature of the sampled data and the complex influence of temperature on some processes (e.g., water solubility of substances, microbial degradation, and photosynthesis). It may, however, be useful to inform further monitoring efforts in the catchment.
The in vitro biotests conducted as part of this study identified two sampling sites of concern in 2022, namely the Elands River, upper site (ELU) and the Mphukojwane River middle site (MPM). In 2023, five sampling sites were identified as being of concern, namely ELU, MPM, Namahadi Lower (NL), Namahadi Middle (ML), and Elands Lower (ELL)—all of which are located in urbanized parts of the catchments. The results showed that during the dry season (winter 2023) there were more sites of concern and that the respective values were higher than during the rainy season (summer 2022) when the rivers experienced high flow volumes.
Samples with a high estrogenic potential in the YES-Test indicate pollution with estrogenic substances. Beside the natural endogenous estrogens, such as 17β-Estradiol, estrone and estriol, further synthetic estrogens used in human medicine, such as 17α-Ethynylestradiol (EE2) and Mestrone which are present in oral contraceptives, should also be considered in this context. However, estrogen-active substances are not only found in pharmaceuticals, but also in some industrial chemicals, like bisphenol A and Nonylphenol, as well as in certain agrochemicals such as DDT, aldrin, cyhalothrin, cypermethrin, deltamethrin, fenvalerate, glyphosate, permethirndiazinon, prothiofos pyriproxyfen, thiabendazole, and tolclofos-methyl. These agrochemicals are known to act as estrogenic active substances (Robison and Stancel, 1982; Kojima et al., 2004; Kiyama and Wada-Kiyama, 2015) and may have contributed to the estrogenic activity of the water samples. Although estrogenic potential has been detected in South African wastewater (Mahomed et al., 2008; Archer et al., 2020), groundwater (Aneck-Hahn et al., 2009), and surface water (Aneck-Hahn et al., 2008; Archer et al., 2020) samples, the substances causing the estrogenic potential, as well as the sources of these substances, can only be assumed at this stage.
The presence of endocrine disrupting compounds in drinking water is concerning because they can disrupt the delicate balance of the endocrine system of both, humans, and animals, which regulates homeostasis in the body. This disruption can lead to various health issues, including obesity, diabetes, and thyroid disorders (Mendes, 2002). Exposure to high levels of estrogenic compounds can lead to reproductive health problems in both men and women. In men, it might decrease sperm count and increase the incidence of testicular cancer. In women, it can cause irregular menstrual cycles and contribute to reproductive disorders (Cravedi et al., 2007). For fetuses, infants, and children, exposure to estrogenic compounds can affect normal growth and development. This might include early onset of puberty and developmental issues related to the reproductive system (Wang and Zhao, 2019). These concerns are relevant for communities in QwaQwa, since poor households who were unable to afford buying bottled water during the last drought (2015–2016), relied on water from the local rivers for domestic use (Mocwagae, 2020).
A high dioxine-like potential was detected in the samples from site ELU in 2022, and ELL and ELU in 2023. Samples from the other sampling sites, except for MPU, showed a dioxine-like potential above the LOQ (MPM, NL, NM, and MU), or were classified as cytotoxic (NU, MM). The aryl hydrocarbon receptor (ah-receptor) is a rather unspecific biosensor, whose activation occurs through numerous structurally diverse substances (Denison and Nagy, 2003). Among the best studied ligands of the ah-receptor are halogenated aromatic hydrocarbons (HAH), biphenyls, polycyclic aromatic hydrocarbons (PAHs) and benzoflavones (Poland and Knutson, 1982; Denison and Nagy, 2003). As numerous as these substances are, so are their formation pathways, use, and entry into the environment. While some of them are unwanted by-products of incineration processes, industrial exhaust gases, or contaminants in commercial products, others are deliberately produced for use in plastic products, such as flame retardants, disinfectants, biocides, and plant protection products, to name a few. Due to the numerous sources of these substances, the input into the environment can occur via point sources (industrial wastewater, leakages) and diffuse sources (atmospheric deposition due to incineration processes; exhaust gases from industry and traffic; agricultural and urban runoff). There are, however, numerous other substances with a binding affinity to the ah-receptor (in addition to the widely known ligands of the ah-receptor), including many naturally occurring substances such as indoles and indole metabolites, tetrapyrroles (e.g., chlorophylls) and carotenoids (Denison and Nagy, 2003). Knowing this, further monitoring should focus primarily on the sampling site ELU, whose sample showed a very strong activation of the ah-receptor, indicating an anthropogenic impact on the water quality.
The in vitro assessment of the mutagenic and genotoxic potential showed that none of the samples collected in 2022 and 2023 had a mutagenic or genotoxic potential. However, it should be noted that the present study focused predominantly on water-soluble substances. These substances, which bind to suspended particulate matter and sediment, are removed by filtration, reducing the mutagenic/genotoxic potential of the samples. Different authors showed that there can be higher mutagenic/genotoxic activity of the particulate matter compared to the dissolved phase of water samples (Grifoll et al., 1992; White et al., 1996; Reifferscheid and Oepen, 2002; Morales et al., 2022). In future studies, therefore, suspended matter in the water samples and sediments should also be tested for their mutagenic and genotoxic potential, especially at the sites where other bioassays in this study indicate the presence of pollution.
Since this study was the first to investigate the presence of OMPs in the rivers above Phuthaditjhaba, a relatively small number of substances (35) were included in the first sampling campaign (summer 2022) based on substances previously recorded in surface waters impacted by urban and agricultural pollution (Fekadu et al., 2019; Shehu et al., 2022). This number was increased to 114 substances during the second sampling campaign (winter 2023). Due to the relatively long storage period between sampling and analysis in Germany (>10 d), OMPs with known instability, such as antibiotics from the groups of macrolides, fluoroquinolones, or estrogens, were not included in the study. However, the antiretroviral HIV drugs efavirenz (its main metabolite 8-OH-efavirenz) and nevirapine were included, considering the prevalence of nevirapine, efavirenz, and other antiretroviral drugs in South African freshwater bodies (Wood et al., 2015; Madikizela et al., 2017; Schoeman et al., 2017; Rimayi et al., 2018; Nibamureke et al., 2019; Mlunguza et al., 2020; Gani et al., 2021; Adeola and Forbes, 2022). The contribution of these compounds to the overall endocrine disrupting potential of OMPs in the hydrologic cycle remains to be adequately clarified. Indeed, antiretrovirals used for HIV therapy, are suspected of having an estrogenic effect. Sikora et al. (2010) demonstrated that the antiretroviral Efavirenz directly binds the estrogen receptor alpha. Despite declining HIV incidences in South Africa, there is still a relatively high number of HIV infections and with 75% of those infected receiving HIV therapy (World Health Organization, 2022), the likelihood of antiretrovirals entering various environmental compartments after human excretion is high. The poor performance of the WWTWs in QwaQwa (Department of Water and Sanitation, 2022b, 2023) may, therefore, contribute to the fact that Efavirenz concentrations in the lower Elands River (at the downstream end of the catchment) were exceeding the Health-Oriented Guidance Value (Tables 4, 5). Efavirenz, its metabolite 8-OH-efavirenz, and nevirapine most probably contributed to the estrogenic effect of the water samples in the present study.
The concentrations of most OMPs, analyzed during the first campaign in summer 2022, were generally in the low to mid ng/L range, even at sites where they were clearly detected (MPM, ELU, and ELL). Concentrations were, however, significantly lower compared to moderately polluted rivers in Central Europe or North America. For example, up to 1,980 ng/L BP-4 (Wickersbach, tributary of the Main River; Wick et al., 2010), 1,500 ng/L bisphenol A (Elbe River; Huang et al., 2021), or 640 ng/L carbamazepine (Rhine River; Sacher et al., 2008) were determined analytically in German rivers. However, heavy precipitation that occurred during sampling may have resulted in dilution. In contrast, the concentrations measured in winter 2023 were significantly higher and correspond to the above-mentioned ranges of contaminated rivers in other regions.
For substances that were only measured sporadically but in significant concentrations, such as the herbicide acetochlor or the insecticide fipronil, the findings must be verified by further measurements and the results analyzed. In the event of confirmation, the polluters must be identified since their presence cannot necessarily be attributed to domestic wastewater.
The pronounced differences in concentration for the four OMPs ibuprofen, sulfamethoxazole, efavirenz, and atenolol between summer 2022 and winter 2023 (Figure 7) could be due to the following two reasons: (1) the lower flow volumes and water levels during the dry winter season, resulting in higher OMP concentrations being recorded in winter (July 2023) than in summer (March 2022); (2) reduced biological activity due to significantly lower water temperatures in winter (7–11°C in July 2023 vs. 17–22°C in March 2022), slowing down the degradation of OMPs in the rivers.
Figure 7. Comparison of the concentrations of the OMPs ibuprofen, sulfamethoxazole, efavirenz, and atenolol between 2022 (summer) and 2023 (winter).
Contamination of surface water with selected metal elements occurs worldwide, and besides geomorphology, human activities act as a source of pollution (Merian, 1991). This is a concern in aquatic environments because heavy metals, in particular, have negative impacts on aquatic organisms, such as fish (Aziz et al., 2022), snails (Lefcort et al., 2008), and frogs (Burger and Snodgrass, 2001). That can consequently affect humans at the end of the food chain. The DWAF guidelines released in 1996 (Department of Water Affairs and Forestry, 1996a) are a specification of the quality of water for different uses, hence showing limits of the pollution level, and the TWQR values enable the risk assessment of surface water for aquatic ecosystems and domestic use. For the metal elements, As, Ca, and Mg, the measured concentrations at all sampling sites were below the TWQR for aquatic environments and domestic use, respectively (Tables 6, 7). Hence, these elements are not of concern. However, Cu and Zn exceeded the recommended concentrations in almost all sites in the TWQR for aquatic environments and are thus of concern (Tables 6, 7). The highest risk levels for the environment and domestic use come from the heavy metals Fe, Al, and Mn as they exceeded the TWQR values at most sampling sites (Tables 6, 7).
There are several possible sources for such high metal concentrations, including natural sources, agriculture, industrial waste, and wastewater treatment plant effluent. Abundant elements like Fe and Cu could occur naturally (Department of Water Affairs and Forestry, 1996a; Galvin, 1996). In QwaQwa, it is not uncommon to see housing with small vegetable gardens or farming fields near human settlements. This could also be a source of metal pollution (Fei et al., 2020). Metal pollution could also emanate from the effluents released from improperly treated sewage and local industries. For example, Moloi et al. (2020) reported that the effluent from the Phuthaditjhaba wastewater treatment plant contained unacceptable concentrations of metals such as Mg, Fe, Mn, Zn, As, Co, and Cu. Concerning toxicologically relevant heavy metals such as Pb, As, Cd, or Hg, no concentrations of concern were detected in the filtered water samples. Nevertheless, our findings show that water quality in these river systems is being increasingly degraded from the upper to the lower reaches. Most of this adverse effect emanates from anthropogenic activities, which release heavy metals into these rivers. In future, monitoring suspended solids and sediments must be included in the investigations to make a more comprehensive assessment.
Surface water quality in the rivers and streams of QwaQwa has been steadily deteriorating over the past three decades mainly due to urbanization, industrialization, poor waste management, agriculture, and soil erosion in the catchment (River Health Programme, 2003; Motholo, 2014; Mocwagae, 2020; Moloi et al., 2020; Avenant et al., 2023; Sekhele and Voua Otomo, 2023; Kamdem et al., 2024). People have been moving into the area since the 1970's (Slater, 2002), resulting in densely populated urban and semi-urban conditions and a bustling local economy (Statistics South Africa, 2012; Mukwada and Mutana, 2023). Local industries produce a wide range of products such as furniture, textile, leather works, dairy products, agricultural, aluminum, and glass products (Liedtke, 2018). These industries are potential hotspots of industrial pollutants, including heavy metals (Kamdem et al., 2024). Natural occurring sandstone, which is common in QwaQwa, contains Al, Ca, Fe, Mg, and Mn, and could explain the occurrence of some elements from the upper reaches of the rivers (Mubiayi, 2014). The prevalence of small-scale sand mining and brick manufacturing along the riverbanks may also contribute to water pollution. Moloi et al. (2020) have found that the Phuthaditjhaba wastewater treatment plant to be a significant source of metals such as Mg, Mn, Fe, Cu, Zn, and As.
Effluent from the critically poor performing WWTWs in the catchment is arguably an important source of pollutants such as pharmaceuticals and personal care products (Department of Water and Sanitation, 2022b, 2023). Different authors have identified domestic wastewater as the main source of pharmaceuticals in freshwater ecosystems (Shehu et al., 2022; Sekhele and Voua Otomo, 2023). The chemical analysis confirms the presence of pharmaceuticals and their degradation products in the sampled streams. The results of the in vitro tests indicate that pollution by these pharmaceuticals could be exerting various effects on the aquatic organisms found in the headwater streams and rivers in QwaQwa. Further studies in the QwaQwa area should include investigative monitoring for detecting hot spots of contamination (WWTW effluent and other possible sewage outlets).
In areas without connection to wastewater treatment plants diffuse input of pharmaceuticals via direct human excretion, especially during rainfall events should be considered as a main source of the increased estrogenic potential and increased concentrations of measured pharmaceuticals at the respective sampling sites as well. For example, in the City of Ekurhuleni Metropolitan area (Gauteng Province, South Africa), Archer et al. (2020) found that for 70% of the WWTWs investigated, the estrogenic potential was lower downstream of the WWTWs compared to upstream. Archer et al. (2020) concluded that the estrogenic potential in the river water samples downstream of the WWTW “may not necessarily be associated with WWTW effluent alone” but with other sources like direct sewage outlets via stormwater drainage systems, as well as input from diffuse sources like informal settlements and industrial areas.
Our findings show that the water quality in these streams is being increasingly degraded from the upper to the lower reaches. Most of this adverse effect emanates from anthropogenic activities, which release heavy metals, pharmaceuticals and substances found in personal care products into these rivers. The lack of regular monitoring of the rivers in the region raises severe concerns since it is not known how much of an impact local point sources (such as the Phuthaditjhaba WWTW and local factories) and non-point sources of pollution are having on these aquatic systems. The level of concern is even more significant when one considers that the local communities sometimes use these rivers as water sources for domestic purposes without any treatment. Department of Water Affairs and Forestry (1996b) suggested prioritizing adequate maintenance and protection of local aquatic ecosystems over mitigating adverse effects when they happen. However, protecting aquatic ecosystems can only be achieved through better compliance with, and enforcement of, governmental guidelines at local levels.
As already mentioned in this contribution, the QwaQwa region is not peculiar within the South African context. Countrywide, a lack of proper wastewater treatment infrastructure (Department of Water and Sanitation, 2018), the poor performance of the existing infrastructure (Department of Water and Sanitation, 2022a,b, 2023), and effluent from domestic, agricultural, and industrial sources all contribute to the degradation of surface water quality (Adeyinka et al., 2018, 2019; Verlicchi and Grillini, 2020). Research reveals similar pressures on surface water quality in Nigeria (Ighalo and Adeniyi, 2020), Tanzania (Shen et al., 2022), Cameroon (Mvongo and Defo, 2021), and across sub-Saharan Africa in general (Onu et al., 2023). As such, the present contribution could provide insight into the increased pressures posed by anthropogenic activities on surface water challenges in Africa.
This study underscores the alarming state of pollution and degradation in the rivers and streams of QwaQwa, with implications for both ecological integrity and human wellbeing. The reliance of communities on the local streams as domestic water sources during water interruptions and droughts is particularly concerning. The detection of high levels of pollutants, such as heavy metals and organic micropollutants, highlights the multifaceted nature of the challenges faced by these aquatic ecosystems in the context of sustainable integrated water quality management.
In anticipation of future developments, the study provides valuable insights and recommendations for addressing the identified challenges. Enhancing monitoring efforts through the integration of a range of complimentary techniques, from measuring simple water quality parameters to complex biological and chemical analyses, are essential for gaining a more comprehensive understanding of pollutant sources, distribution pathways, and potential impacts. By expanding the substance spectrum for target analyses and conducting spatial analyses to locate the origins of contamination, mitigatory efforts can be efficiently prioritized and managed.
Community engagement and compliance with governmental guidelines are critical aspects of successful remediation strategies. By fostering awareness and promoting proactive measures for the protection of local aquatic ecosystems, stakeholders can work toward sustainable solutions to safeguard water quality for present and future generations.
In summary, addressing the complex challenges of water pollution in QwaQwa requires a concerted effort from all stakeholders, guided by scientific evidence and proactive measures. By implementing the recommendations outlined in this study, the critical water resources can be protected to secure water supply and other ecosystem services in future.
The following recommendations are given for future monitoring in the QwaQwa area and other comparable river basins for which information is lacking:
• Develop a stepwise monitoring approach: step 1—do an initial screening of the catchment by using general and affordable water quality measurements to identify river sections impacted by pollution; step 2—verify the river sections and sites identified during step 1 by using applying more sophisticated sampling methods such as in vitro bioassays; step 3—use more complex chemical analyses of single compounds by means of chromatography-mass spectrometry for further investigation at sites where traces of pollution were identified in step 2.
• Use GIS-based land-use analysis to identify potential sources of pollution in catchments, and to identify potential sampling sites to ensure effective monitoring of pollution coming from these sources. Such analyses could also reveal illegal activities and river degradation in the catchment.
• Identify direct industrial and commercial dischargers in urban areas and record the treatment of wastewater, for example are they disposing wastewater directly into a water body or via a wastewater treatment plant.
• Monitor selected sampling sites by applying standard methods at regular intervals to allow for establishing long-term trends and comparison of the data between seasons and years.
• Involve local communities in the biomonitoring of rivers through Citizen science programmes. Community members can be trained to collect basic water quality information by using simple, low-cost, and easy-to-use methods (e.g., Mini-SASS: a simple river assessment and scoring system using aquatic macroinvertebrates; Graham et al., 2004) and instruments (e.g., clarity tube: clear acrylic tube used to visually assess water clarity; Graham et al., 2024). The collected data can be submitted to a central database using cell phones.
• Restore highly impacted and degraded river habitats and riparian zones through the removal of solid waste and invasive vegetation from rivers, protecting riparian vegetation and riverbanks against trampling by cattle and small stock, controlling illegal sand mining, etc.
• Encourage traditional leaders and ward committees to become actively involved in the management of local water resources. Mobilize community leaders to hold local government departments accountable for mismanagement or failure to address poor water quality and pollution.
• Inform riverbank communities about the negative impacts of inappropriate waste disposal methods such as burning or disposing solid waste onto riverbanks and into waterbodies. Implement solid waste management systems or strategies to ensure regular waste removal or providing dedicated waste disposal sites to communities.
• Develop dedicated South African monitoring protocols and legislative guidelines for emerging contaminants in freshwater bodies.
The raw data supporting the conclusions of this article will be made available by the authors, without undue reservation.
MA: Conceptualization, Funding acquisition, Investigation, Project administration, Resources, Writing – original draft, Writing – review & editing, Supervision, Methodology. HB: Conceptualization, Formal analysis, Investigation, Methodology, Resources, Visualization, Writing – original draft, Writing – review & editing. SG: Formal analysis, Investigation, Methodology, Writing – original draft, Visualization. NN: Formal analysis, Investigation, Visualization, Writing – original draft. BO: Investigation, Writing – original draft, Methodology, Supervision. PV: Investigation, Methodology, Resources, Supervision, Writing – original draft, Writing – review & editing. AS: Formal analysis, Visualization, Writing – original draft. SS: Formal analysis, Writing – original draft. TV: Investigation, Resources, Writing – original draft. DJ: Conceptualization, Funding acquisition, Investigation, Methodology, Project administration, Resources, Supervision, Visualization, Writing – original draft, Writing – review & editing.
The author(s) declare financial support was received for the research, authorship, and/or publication of this article. This work was financially supported by the Water Research Commission of South Africa (project number: C2019/2020-00239), the Volkswagen Foundation (project number: 9A 926, Germany), and the International Office of the Technical University of Dresden (Germany). The Q6500+ analyzer used in some of the analyses was funded by the European Regional Development Fund (ERDF) and the Free State of Saxony.
SG was employed by GWT-TUD GmbH.
The remaining authors declare that the research was conducted in the absence of any commercial or financial relationships that could be construed as a potential conflict of interest.
All claims expressed in this article are solely those of the authors and do not necessarily represent those of their affiliated organizations, or those of the publisher, the editors and the reviewers. Any product that may be evaluated in this article, or claim that may be made by its manufacturer, is not guaranteed or endorsed by the publisher.
The Supplementary Material for this article can be found online at: https://www.frontiersin.org/articles/10.3389/frwa.2024.1408856/full#supplementary-material
Abiodun, B. J., Makhanya, N., Petja, B., Abatan, A. A., and Oguntunde, P. G. (2019). Future projection of droughts over major river basins in Southern Africa at specific global warming levels. Theor. Appl. Climatol. 137, 1785–1799. doi: 10.1007/s00704-018-2693-0
Adeola, A. O., and Forbes, P. B. C. (2022). Antiretroviral drugs in African surface waters: prevalence, analysis, and potential remediation. Environ. Toxicol. Chem. 41, 247–262. doi: 10.1002/etc.5127
Adeyinka, G. C., Moodley, B., Birungi, G., and Ndungu, P. (2018). Quantitative analyses of selected polychlorinated biphenyl (PCB) congeners in water, soil, and sediment during winter and spring seasons from Msunduzi River, South Africa. Environ. Monit. Assess. 190, 1–13. doi: 10.1007/s10661-018-6993-8
Adeyinka, G. C., Moodley, B., Birungi, G., and Ndungu, P. (2019). Evaluation of organochlorinated pesticide (OCP) residues in soil, sediment and water from the Msunduzi River in South Africa. Environ. Earth Sci. 78, 1–13. doi: 10.1007/s12665-019-8227-y
Agricultural Research Council Institute for Soil Climate and Water South Africa (2016). Meteorological Data - Weather Stations. Agricultural Research Council of South Africa.
Albrektiene, R., Rimeika, M., Zalieckiene, E., Šaulys, V., and Zagorskis, A. (2012). Determination of organic matter by UV absorption in the ground water. J. Environ. Eng. Landsc. Manag. 20, 163–167. doi: 10.3846/16486897.2012.674039
Aneck-Hahn, N. H., Bornman, M., and de Jager, C. (2008). Preliminary assessment of oestrogenic activity in water sources in Rietvlei Nature Reserve, Gauteng, South Africa. Afr. J. Aquat. Sci. 33, 249–254. doi: 10.2989/AJAS.2008.33.3.7.619
Aneck-Hahn, N. H., Bornman, M., and de Jager, C. (2009). Oestrogenic activity in drinking waters from a rural area in the Waterberg District, Limpopo Province, South Africa. Water SA 35, 245–251. doi: 10.4314/wsa.v35i3.76760
Archer, E., Wolfaardt, G. M., van Wyk, J. H., and van Blerk, N. (2020). Investigating (anti)estrogenic activities within South African wastewater and receiving surface waters: implication for reliable monitoring. Environ. Pollut. 263:114424. doi: 10.1016/j.envpol.2020.114424
Avenant, M. F., Belle, J. A., Boernick, H., Jungmann, D., Opeolu, B., Voua Otomo, P., et al. (2023). Threats of Extreme Weather Events: Improving the Resilience of QwaQwa to the Multiple Risks of Climate Change. WRC Report No. 3091/1/23. Pretoria: Water Research Commission. Available online at: https://www.wrc.org.za/wp-content/uploads/mdocs/3091%20Vol%201%20final.pdf (accessed March 17, 2024).
Aziz, S., Abdullah, S., Anwar, H., and Latif, F. (2022). DNA damage and oxidative stress in economically important fish, bighead carp (Hypophthalmichthys nobilis) exposed to engineered copper oxide nanoparticles. Pak. Vet. J. 42, 1–8. doi: 10.29261/pakvetj/2022.002
Burger, J., and Snodgrass, J. (2001). Metal levels in southern leopard frogs from the Savannah River Site: location and body compartment effects. Environ. Res. 86, 157–166. doi: 10.1006/enrs.2001.4245
Carstensen, L., Zippel, R., Fiskal, R., Börnick, H., Schmalz, V., Schubert, S., et al. (2023). Trace analysis of benzophenone-type UV filters in water and their effects on human estrogen and androgen receptors. J. Hazard. Mater. 456:131617. doi: 10.1016/j.jhazmat.2023.131617
Colvin, C., Lindley, D., Gordon, H., and Schachtschneider, K. (2016). Water: Facts and Futures: Rethinking South Africa's Future. Cape Town: World Wildlife Fund South Africa (WWF-SA). Available online at: http://awsassets.wwf.org.za/downloads/wwf009_waterfactsandfutures_report_web__lowres_.pdf (accessed March 22, 2024).
Cravedi, J. P., Zalko, D., Savouret, J. F., Menuet, A., and Jegou, B. (2007). The concept of endocrine disruption and human health. M. S Med. Sci. 23, 198–204. doi: 10.1051/medsci/2007232198
Denison, M. S., and Nagy, S. R. (2003). Activation of the aryl hydrocarbon receptor by structurally diverse exogenous and endogenous chemicals. Annu. Rev. Pharmacol. Toxicol. 43, 309–334. doi: 10.1146/annurev.pharmtox.43.100901.135828
Department of Water Affairs and Forestry (1996a). South African Water Quality Guidelines, Volume 1: Domestic Use, 2nd Edn. Available online at: https://www.dws.gov.za/Groundwater/documents/Pol_saWQguideFRESHDomesticusevol1.pdf (accessed March 22, 2024).
Department of Water Affairs and Forestry (1996b). South African Water Quality Guidelines, Volume 7: Aquatic Ecosystems, 2nd Edn. Available online at: https://www.dws.gov.za/iwqs/wq_guide/edited/Pol_saWQguideFRESH_vol7_Aquaticecosystems.pdf (accessed March 22, 2024).
Department of Water Affairs and Forestry (2004). Upper Vaal Water Management Area: Internal Strategic Perspective. Report Prepared by PDNA, WRP Consulting Engineers (Pty) Ltd., WMB and Kwezi-V3 for the DWAF. DWAF Report no. P WMA 08/000/00/0304. Pretoria: DWAF. Available online at: https://www.dws.gov.za/Documents/Other/WMA/Upper_Vaal_ISP.pdf (accessed March 22, 2024).
Department of Water and Sanitation (2014). Determination of Resource Quality Objectives in the Upper Vaal Water Management Area (WMA8): Resource Quality Objectives and numerical limits report. Report No.: RDM/WMA08/00/CON/RQO/0214. Pretoria: DWS. Available online at: https://www.dws.gov.za/RDM/WRCS/doc/Upper%20Vaal%20_WMA8_%20RQO%20Report%206%20-%20RQOs%20and%20Numerical%20Limits%20Report_FINAL.pdf (accessed March 22, 2024).
Department of Water and Sanitation (2018). National Water and Sanitation Master Plan. Volume 1: Call to Action (Version 10.1). Available online at: https://www.gov.za/sites/default/files/gcis_document/201911/national-water-and-sanitation-master-plandf.pdf (accessed March 22, 2024).
Department of Water and Sanitation (2022a). Blue Drop 2022: Progress Report. Available online at: https://ws.dws.gov.za/IRIS/releases/2021_BD_PAT_report_final-28Mar22_MN_web.pdf (accessed March 22, 2024).
Department of Water and Sanitation (2022b). Green Drop 2022: National Report. Available online at: https://ws.dws.gov.za/iris/releases/Report_DPW_Rev02_29Mar22_MN%20web.pdf (accessed March 22, 2024).
Department of Water and Sanitation (2023). Green Drop: Watch Report 2023. Available online at: https://ws.dws.gov.za/iris/releases/GDWR.pdf (accessed May 14, 2024).
Dieter, H. H. (2014). Health related guide values for drinking-water since 1993 as guidance to assess presence of new analytes in drinking-water. Int. J. Hyg. Environ. Health 217, 117–132. doi: 10.1016/j.ijheh.2013.05.001
DIN EN ISO 21427-2 (2009). Wasserbeschaffenheit-Bestimmung der Gentoxizität mit dem In-vitro-Mikrokerntest -Teil 2: Verwendung einer nicht-synchronisierten V79-Zellkulturlinie (ISO 21427-2:2006); Deutsche Fassung EN ISO 21427-2:2009. Deutsches Institut für Normung (German Institute for Standardization).
Du Plessis, A. (2017). Freshwater Challenges of South Africa and Its Upper Vaal River: Current State and Outlook (Cham: Springer Water), 77–98.
Edokpayi, J. N., Odiyo, J. O., and Durowoju, O. S. (2017). “Impact of wastewater on surface water quality in developing countries: a case study of South Africa,” in Water Quality, ed. H. Tutu (IntechOpen), 66561. Available online at: https://www.intechopen.com/chapters/53194 (accessed March 22, 2024).
EN ISO 10304-1 (2007). Water Quality—Determination of Dissolved Anions By Liquid Chromatography of Ions. Part 1: Determination of Bromide, Chloride, Fluoride, Nitrate, Nitrite, Phosphate and Sulfate. German Version EN ISO 10304-1:2009. Berlin: Beuth.
EN ISO 11885:2007 (2007). Determination of Selected Elements By Inductively Coupled Plasma Optical Emission Spectrometry (ICP-OES); German Version EN ISO 11885:2009. Berlin: Beuth.
Fei, X., Lou, Z., Xiao, R., Ren, Z., and Lv, X. (2020). Contamination assessment and source apportionment of heavy metals in agricultural soil through the synthesis of PMF and GeogDetector models. Sci. Total Environ. 747:141293. doi: 10.1016/j.scitotenv.2020.141293
Fekadu, S., Alemayehu, E., Dewil, R., and van der Bruggen, B. (2019). Pharmaceuticals in freshwater aquatic environments: a comparison of the African and European challenge. Sci. Total Environ. 654, 324–337. doi: 10.1016/j.scitotenv.2018.11.072
Fourie, B. (2023). Economic Powerhouse Gauteng Makes More Than a Third of South Africa's Money. IOL Property, September 27, 2023. Available online at: https://www.iol.co.za/news/economic-powerhouse-gauteng-makes-more-than-a-third-of-sas-money-75210f70-8dd0-41fe-a415-6619e6b799d3#:~:text=Economic%20powerhouse%20Gauteng%20makes%20more%20than%20a%20third%20of%20SA's%20money,-The%20manufacturing%20industry&text=Gauteng%20may%20be%20the%20smallest,made%20by%20the%20country's%20economy (accessed March 27, 2024).
Free State Department of Cooperative Governance and Traditional Affairs and Chell Engineering (2018). Updating of the Water Master Plan for Maluti-a-Phofung-Local Municipality. Bloemfontein: FS COGTA.
Gani, K. M., Hlongwa, N., Abunama, T., Kumari, S., and Bux, F. (2021). Emerging contaminants in the South African water environment: a critical review of their occurrence, sources and ecotoxicological risks. Chemosphere 269:128737. doi: 10.1016/j.chemosphere.2020.128737
Gerke, J. (2018). Concepts and misconceptions of humic substances as the stable part of soil organic matter: a review. Agronomy 8, 76. doi: 10.3390/agronomy8050076
Gouws, C. M., Mbambo, B., Moeketsi, I., Morotolo, M., Motloung, S., and Tempelhoff, J. W. N. (2010). What About the Votes? Water, Sanitation and Civil Disorientation: the Case of Maluti-a-Phofung Local Municipality (Harrismith). Research Report by the Research Group for the Cultural Dynamics of Water. Vanderbijlpark: Northwest University.
Graham, P. M., Dickens, C. W., and Taylor, R. J. (2004). miniSASS-A novel technique for community participation in river health monitoring and management. Afr. J. Aquat. Sci. 29, 25–35. doi: 10.2989/16085910409503789
Graham, P. M., Pattinson, N. B., Lepheana, A. T., and Taylor, R. J. (2024). Clarity tubes as effective citizen science tools for monitoring wastewater treatment works and rivers. Integr. Environ. Assess. Manag. 2024, 1–10. doi: 10.2139/ssrn.4662221
Grifoll, M., Solanas, A. M., and Bayona, J. M. (1992). Bioassay-directed chemical characterization of genotoxic agents in the dissolved and particulate water phases of the Besos and Llobregat rivers (Barcelona, Spain). Arch. Environ. Contam. Toxicol. 23, 19–25. doi: 10.1007/BF00225991
Huang, Z., Hua, P., Wang, Z., Li, R., Dong, L., Hu, B. X., et al. (2021). Environmental behavior and potential driving force of bisphenol A in the Elbe River: a long-term trend study. Sci. Total Environ. 761:143251. doi: 10.1016/j.scitotenv.2020.143251
Ighalo, J. O., and Adeniyi, A. G. (2020). A comprehensive review of water quality monitoring and assessment in Nigeria. Chemosphere 260:127569. doi: 10.1016/j.chemosphere.2020.127569
ISO 11350 (2012). Water Quality—Determination of the Genotoxicity of Water and Waste Water—Salmonella/Microsome Fluctuation Test (Ames Fluctuation Test). Geneva: International Organization for Standardization (ISO).
ISO 19040-1 (2018). Water Quality—Determination of the Estrogenic Potential of Water and Waste Water—Part 1: Yeast Estrogen Screen (Saccharomyces cerevisiae). Geneva: International Organization for Standardization (ISO).
Kamdem, M. M., Kubheka, N., Nyoka, N. K., and Otomo, P. V. (2024). Using Folsomia candida (Collembola) for the ecological assessment of sediment samples from three rivers from the QwaQwa region, South Africa. Int. J. Energ. Water Res. 2024, 1–11. doi: 10.1007/s42108-024-00282-3
Karr, J. R., and Chu, E. W. (1997). Biological monitoring: essential foundation for ecological risk assessment. Hum. Ecol. Risk Assess. 3, 993–1004. doi: 10.1080/10807039709383742
Karr, J. R., and Dudley, D. R. (1981). Ecological perspective on water quality goals. Environ. Manag. 5, 55–68. doi: 10.1007/BF01866609
Kiyama, R., and Wada-Kiyama, Y. (2015). Estrogenic endocrine disruptors: molecular mechanisms of action. Environ. Int. 83, 11–40. doi: 10.1016/j.envint.2015.05.012
Kojima, H., Katsura, E., Takeuchi, S, Niiyama, K., and Kobayashi, K. (2004). Screening for estrogen and androgen receptor activities in 200 pesticides by in vitro reporter gene assays using Chinese hamster ovary cells. Environ. Health Perspect. 112, 524–531. doi: 10.1289/ehp.6649
Kruger, A., Pieters, R., Horn, S., van Zijl, C., and Aneck-Hahn, N. (2022). The role of effect-based methods to address water quality monitoring in South Africa: a developing country's struggle. Environ. Sci. Pollut. Res. Int. 29, 84049–84055. doi: 10.1007/s11356-022-23534-3
Lefcort, H., Freedman, Z., House, S., and Pendleton, M. (2008). Hormetic effects of heavy metals in aquatic snails: is a little bit of pollution good? EcoHealth 5, 10–17. doi: 10.1007/s10393-008-0158-0
Liedtke, S. (2018). DTI Completes R50m Phase 1 Upgrade of Phuthaditjhaba Industrial Park. Engineering News, May 3, 2018. Available online at: https://www.engineeringnews.co.za/article/dti-completes-r50m-phase-1-upgrade-of-phuthaditjhaba-industrial-park-2018-05-03 (accessed February 22, 2023).
Macupe, B. (2020). Impossible Choices in QwaQwa: What it Takes to Get Water. Mail and Guardian, February 7, 2020. Available online at: https://mg.co.za/article/2020-02-07-impossible-choices-in-QwaQwa-what-it-takes-to-get-water/ (accessed February 22, 2023).
Madikizela, L. M., Tavengwa, N. T., and Chimuka, L. (2017). Status of pharmaceuticals in African water bodies: occurrence, removal and analytical methods. J. Environ. Manage. 193, 211–220. doi: 10.1016/j.jenvman.2017.02.022
Magdeburg, A., Stalter, D., Schlüsener, M., Ternes, T., and Oehlmann, J. (2014). Evaluating the efficiency of advanced wastewater treatment: target analysis of organic contaminants and (geno-) toxicity assessment tell a different story. Water Res. 50, 35–47. doi: 10.1016/j.watres.2013.11.041
Mahomed, S. I., Voyi, K., Aneck-Hahn, N. H., and de Jager, C. (2008). Oestrogenicity and chemical target analysis of water from small-sized industries in Pretoria, South Africa. Water SA 34, 357–363. doi: 10.4314/wsa.v34i3.180629
Mail and Guardian (2004). Teenager Dies After Harrismith Protest. Mail and Guardian, August 31, 2004. Available online at: https://mg.co.za/article/2004-08-31-teenager-dies-after-harrismith-protest/ (accessed February 22, 2023).
Mdlalane, A. (2020). QwaQwa Water Crises Blamed on Infrastructure. SABC News, February 1, 2020. Available online at: https://www.sabcnews.com/sabcnews/QwaQwa-water-crises-blamed-on-poor-infrastructure/ (accessed February 22, 2023).
Melore, T. W. (2017). Improving the Climate Change Resilience of Informal Settlements in Mountainous Regions of Africa: Comparative Case Studies of QwaQwa in South Africa and Konso in Ethiopia (Doctoral dissertation). University of the Free State, Bloemfontein, South Africa.
Melore, T. W., and Nel, V. (2020). Resilience of informal settlements to climate change in the mountainous areas of Konso, Ethiopia and QwaQwa, South Africa. Jàmbá 12, 1–9. doi: 10.4102/jamba.v12i1.778
Mendes, J. A. (2002). The endocrine disrupters: a major medical challenge. Food Chem. Toxicol. 40, 781–788. doi: 10.1016/S0278-6915(02)00018-2
Merian, E. (1991). Metals and their Compounds in the Environment. Occurrence, Analysis and Biological Relevance. Hoboken, NJ: VCH.
Mlunguza, N. Y., Somandla Ncube, S., Mahlamb, P. N., Chimuka, L., and Madikizel, L. M. (2020). Determination of selected antiretroviral drugs in wastewater, surface water and aquatic plants using hollow fibre liquid phase microextraction and liquid chromatography - tandem mass spectrometry. J. Hazard. Mater. 382:121067. doi: 10.1016/j.jhazmat.2019.121067
Mocwagae, K. (2020). Exploring the QwaQwa Water Crisis for Effective Planning in Post-apartheid South Africa (Dissertation). University of the Free State, Bloemfontein, South Africa.
Mohamed, A. A., and Mukwada, G. (2019). Temperature changes in the Maloti-Drakensberg region: an analysis of trends for the 1960-2016 period. Atmosphere 10:471. doi: 10.3390/atmos10080471
Moloi, M., Ogbeide, O., and Otomo, P. V. (2020). Probabilistic health risk assessment of heavy metals at wastewater discharge points within the Vaal River Basin, South Africa. Int. J. Hyg. Environ. Health 224:113421. doi: 10.1016/j.ijheh.2019.113421
Morales, D. A., Massei, R., Schulze, T., Krauss, M., Brack, W., and de Aragão Umbuzeiro, G. (2022). Mutagenicity of the Danube River: the contribution of liquid phase and particulate suspended matter. Environ. Mol. Mutagen. 63, 162–168. doi: 10.1002/em.22478
Motholo, L. F. (2014). Characterization of Macro-and Micro-Invertebrates and Assessment of Water Quality in Dams and Rivers of QwaQwa (Doctoral dissertation). University of the Free State, Bloemfontein, South Africa.
Mubiayi, M. P. (2014). “Mineralogical and physical characterisation of QwaQwa sandstones,” in Transactions on Engineering Technologies: Special Volume of the World Congress on Engineering 2013, eds. G. C. Yang, S. I. Ao, and L. Gelman (Dordrecht: Springer), 213–225.
Mukwada, G., and Mutana, S. (2023). “Surviving the limits imposed by a changing climate: the case of urban drought and water supply sustainability in Phuthaditjhaba,” in Sustainable Futures in Southern Africa's Mountains: Multiple Perspectives on an Emerging City, eds. A. Membretti, S. J. Taylor, and J. L. Delves (Cham: Springer Nature), 75–89.
Mvongo, V. D., and Defo, C. (2021). Assessing water service performances in rural sub-Saharan Africa environment: the case studies of two councils of the southern and eastern regions of the Republic of Cameroon (Central Africa). J. Water Sanit. Hyg. Dev. 11, 37–50. doi: 10.2166/washdev.2020.164
Neale, P., Escher, B., and Leusch, F. (2022). Effect Based Monitoring in Water Safety Planning. Global Water Research Coalition. Available online at: https://globalwaterresearchcoalition.net/wp-content/uploads/2023/05/20200614_GWRC-WP5.3-and-5.4-Report-1.pdf (accessed March 22, 2023).
Nel, J. L., Le Maitre, D. C., Roux, D. J., Colvin, C., Smith, J. S., Smith-Adao, L. B., et al. (2017). Strategic water source areas for urban water security: making the connection between protecting ecosystems and benefiting from their services. Ecosyst. Serv. 28, 251–259. doi: 10.1016/j.ecoser.2017.07.013
Nibamureke, M. C. U., Barnhoorn, I. E., and Wagenaar, G. M. (2019). Assessing the potential effects of nevirapine in South African surface water on fish growth: a chronic exposure of Oreochromis mossambicus. S. Afr. J. Sci. 115, 1–6. doi: 10.17159/sajs.2019/5516
Noble, M., and Wright, G. (2013). Using indicators of multiple deprivation to demonstrate the spatial legacy of apartheid in South Africa. Soc. Indic. Res. 112, 187–201. doi: 10.1007/s11205-012-0047-3
Onu, M. A., Ayeleru, O. O., Oboirien, B., and Olubambi, P. A. (2023). Challenges of wastewater generation and management in sub-Saharan Africa: a review. Environ. Chall. 11:100686. doi: 10.1016/j.envc.2023.100686
Organization for Economic Cooperation and Development (2016). Test No. 487: in vitro Mammalian Cell Micronucleus Test, OECD Guidelines for the Testing of Chemicals, Section 4. Paris: OECD Publishing.
Organization for Economic Cooperation and Development (2020). Test No. 471: Bacterial Reverse Mutation Test, OECD Guidelines for the Testing of Chemicals, Section 4. Paris: OECD Publishing.
Poland, A., and Knutson, J. C. (1982). 2, 3, 7, 8-tetrachlorodibenzo-p-dioxin and related halogenated aromatic hydrocarbons: examination of the mechanism of toxicity. Annu. Rev. Pharmacol. 22, 517–554. doi: 10.1146/annurev.pa.22.040182.002505
Reifferscheid, G., Dill, F., Fieblinger, D., Gminski, R., Grummt, H. J., Hafner, C., et al. (2007). Untersuchung von Abwasserproben auf Gentoxizität (Measurement of genotoxicity in waste water samples-results of a collaborative study on the in vitro micronucleus test in the context of standardisation according to ISO). Umweltwissenschaften und Schadstoff-Forschung 19, 7–16. doi: 10.1065/uwsf2006.12.155
Reifferscheid, G., and Oepen, B. V. (2002). Genotoxicity and mutagenicity of suspended particulate matter of river water and waste water samples. Sci. World J. 2, 1036–1039. doi: 10.1100/tsw.2002.206
Rimayi, C., Odusanya, D., Weiss, J. M., de Boer, J., and Chimuka, L. (2018). Contaminants of emerging concern in the Hartbeespoort Dam catchment and the uMngeni River estuary 2016 pollution incident, South Africa. Sci. Tot. Environ. 627, 1008–1017. doi: 10.1016/j.scitotenv.2018.01.263
Ritz, C., Baty, F., Streibig, J. C., and Gerhard, D. (2015). Dose-response analysis using R. PLoS ONE 10:e0146021. doi: 10.1371/journal.pone.0146021
River Health Programme (2003). State-of-the-Rivers Report: Free State Region River Systems. Pretoria: Department of Water Affairs and Forestry.
Robison, A. K., and Stancel, G. M. (1982). The estrogenic activity of DDT: correlation of estrogenic effect with nuclear level of estrogen receptor. Life Sci. 31, 2479–2484. doi: 10.1016/0024-3205(82)90753-6
Roux, D. J. (2001). Development of Procedures for the Implementation of the National River Health Program. Water Research Commission Report 850/1/01. Pretoria: Water Research Commission.
Rüdel, H., Körner, W., Letzel, T., Neumann, M., Nödler, K., and Reemtsma, T. (2020). Persistent, mobile and toxic substances in the environment: a spotlight on current research and regulatory activities. Environ. Sci. Eur. 32, 1–11. doi: 10.1186/s12302-019-0286-x
Sacher, F., Ehmann, M., Gabriel, S., Graf, C., and Brauch, H. J. (2008). Pharmaceutical residues in the river Rhine-results of a one-decade monitoring programme. J. Environ. Monit. 10, 664–670. doi: 10.1039/b800701b
Schoeman, C., Dlamini, M., and Okonkwo, O. J. (2017). The impact of a Wastewater Treatment Works in Southern Gauteng, South Africa on efavirenz and nevirapine discharges into the aquatic environment. Emerg. Contam. 3, 95–106. doi: 10.1016/j.emcon.2017.09.001
Sekhele, N., and Voua Otomo, P. (2023). “Afromontane community's dependence on the water and climate change nexus of the Maloti-Drakensberg Mountain range: the case of Phuthaditjhaba,” in Sustainable Futures in Southern Africa's Mountains: Multiple Perspectives on an Emerging City, eds. A. Membretti, S. J. Taylor, and J. L. Delves (Cham: Springer Nature), 91–104.
Shehu, Z., Nyakairu, G. W. A., Tebandeke, E., and Odume, O. N. (2022). Overview of African water resources contamination by contaminants of emerging concern. Sci. Total Environ. 852:158303. doi: 10.1016/j.scitotenv.2022.158303
Shen, Q., Friese, K., Gao, Q., Yu, C., Kimirei, I. A., Kishe-Machumu, M. A., et al. (2022). Status and changes of water quality in typical near-city zones of three East African Great Lakes in Tanzania. Environ. Sci. Pollut. Res. 29, 1–14. doi: 10.1007/s11356-021-18079-w
Sikora, M., Rae, J., Johnson, M., and Desta, Z. (2010). Efavirenz directly modulates the oestrogen receptor and induces breast cancer cell growth. HIV Med. 11, 603–607. doi: 10.1111/j.1468-1293.2010.00831.x
Slater, R. (2002). Differentiation and diversification: changing livelihoods in Qwaqwa, South Africa, 1970-2000. J. South. Afr. Stud. 28, 599–614. doi: 10.1080/0305707022000006530
Sommaggio, L. R. D., Mazzeo, D. E. C., Pamplona-Silva, M. T., and Marin-Morales, M. A. (2018). Evaluation of the potential agricultural use of biostimulated sewage sludge using mammalian cell culture assays. Chemosphere 199, 10–15. doi: 10.1016/j.chemosphere.2018.01.144
Statistics South Africa (2012). 2011 Census. Available online at: http://www.statssa.gov.za/publications/P03014/P030142011.pdf (accessed February 22, 2023).
Steffen, H., Bosch, C., Wolfaardt, G., and Botha, A. (2022). Rising environmental temperatures and polluted surface waters: the prelude to the rise of mycoses in South Africa. Water SA 48, 199–216. doi: 10.17159/wsa/2022.v48.i2.3918
Umweltbundesamt (2003). Bundesgesundheitsbl - Gesundheitsforsch - Gesundheitsschutz 2003, 46, 249–251. Available online at: http://www.umweltbundesamt.de/sites/default/files/medien/374/dokumente/gow-empfehlung_2003_46.pdf (accessed April 4, 2023).
Upper Vaal Catchment Management Forum (2022). Water Quality Guidelines: in-Stream Water Quality Guidelines for the Wilge River Catchment—Elands River. Available online at: https://drive.google.com/file/d/1YVVED3izJ3EK-3myjKOc6690HNKDIU7e/view?pli=1 (accessed March 26, 2024).
Verlicchi, P., and Grillini, V. (2020). Surface water and groundwater quality in South Africa and Mozambique: analysis of the most critical pollutants for drinking purposes and challenges in water treatment selection. Water 12:305. doi: 10.3390/w12010305
Wang, P., and Zhao, Y. (2019). “Plasticizer exposure and reproductive health: phthalates and bisphenol A,” in Emerging Chemicals and Human Health ed. Y. Zhang (Singapore: Springer), 49–67.
White, P. A., Rasmussen, J. B., and Blaise, C. (1996). Sorption of organic genotoxins to particulate matter in industrial effluents. Environ. Mol. Mutagen. 27, 140–151. doi: 10.1002/(SICI)1098-2280(1996)27:2<140::AID-EM8>3.0.CO;2-K
Wick, A., Fink, G., and Ternes, T. A. (2010). Comparison of electrospray ionization and atmospheric pressure chemical ionization for multi-residue analysis of biocides, UV-filters and benzothiazoles in aqueous matrices and activated sludge by liquid chromatography-tandem mass spectrometry. J. Chromatogr. A 1217, 2088–2103. doi: 10.1016/j.chroma.2010.01.079
Wilkinson, J., Hooda, P. S., Barker, J., Barton, S., and Swinden, J. (2017). Occurrence, fate and transformation of emerging contaminants in water: an overarching review of the field. Environ. Pollut. 231, 954–970. doi: 10.1016/j.envpol.2017.08.032
Wood, T. P., Duvenage, C. S., and Rohwer, E. (2015). The occurrence of anti-retroviral compounds used for HIV treatment in South African surface water. Environ. Pollut. 99, 235–243. doi: 10.1016/j.envpol.2015.01.030
World Health Organization (2022). South Africa HIV Country Profile 2022. Available online at: https://cfs.hivci.org/ (accessed January 19, 2023).
Keywords: in vitro biotests, organic micropollutants, metal and aquatic pollution, effect-based methods, Afromontane region, Maloti-Drakensberg, northeastern Free State, Namahadi River
Citation: Avenant M, Börnick H, Graumnitz S, Nyoka N, Opeolu B, Voua Otomo P, Schubert A, Schubert S, Vos T and Jungmann D (2024) Investigating a surface water quality monitoring approach for QwaQwa, South Africa, by combining biological in vitro tests and chemical analyses. Front. Water 6:1408856. doi: 10.3389/frwa.2024.1408856
Received: 28 March 2024; Accepted: 27 May 2024;
Published: 01 July 2024.
Edited by:
Frank Onderi Masese, University of Eldoret, KenyaReviewed by:
Venkatramanan Senapathi, National College, Tiruchirappalli, IndiaCopyright © 2024 Avenant, Börnick, Graumnitz, Nyoka, Opeolu, Voua Otomo, Schubert, Schubert, Vos and Jungmann. This is an open-access article distributed under the terms of the Creative Commons Attribution License (CC BY). The use, distribution or reproduction in other forums is permitted, provided the original author(s) and the copyright owner(s) are credited and that the original publication in this journal is cited, in accordance with accepted academic practice. No use, distribution or reproduction is permitted which does not comply with these terms.
*Correspondence: Marinda Avenant, YXZlbmFudG1mQHVmcy5hYy56YQ==
Disclaimer: All claims expressed in this article are solely those of the authors and do not necessarily represent those of their affiliated organizations, or those of the publisher, the editors and the reviewers. Any product that may be evaluated in this article or claim that may be made by its manufacturer is not guaranteed or endorsed by the publisher.
Research integrity at Frontiers
Learn more about the work of our research integrity team to safeguard the quality of each article we publish.