- 1Mamiraua Institute for Sustainable Development, Tefé, Brazil
- 2Department of Civil and Environmental Engineering, Federal University of Minas Gerais, Belo Horizonte, Brazil
- 3Institute of Geosciences, University of São Paulo, São Paulo, Brazil
- 4State University of Amazonas, Tefé, Brazil
Residents of remote areas in the Amazon often lack access to a water supply system and thus need to produce their potable water at home. This study examined the efficacy of household water treatments traditionally used by these communities to treat rainwater and river water, their predominant water sources. Samples of untreated, treated, and stored drinking water were collected from 18 households in three communities in Central Amazon, Amazonas State, Brazil. We describe the materials and practices involved and traditionally used in each treatment technique – cloth filtration (water straining), chlorination, and sedimentation, and their efficiency. In the samples we evaluate water quality analyses, as free chlorine, color, coliforms, and turbidity. The treatment steps for the separation of solids in river water were effective only for removing turbidity and apparent color. Straining river water after sedimentation had no relevant effect on water quality. Chlorination of rainwater was efficient in inactivating Escherichia coli; however, all samples showed some level of contamination by E. coli. We found a significant difference (p < 0.05) between untreated and treated river water turbidity, reduced by up to 22%. Untreated rain and river waters showed similar levels of microbiological contamination, close to 3.5 log CFU/100 mL of E. coli. Chlorine effectively removed microbiological contaminants in rainwater (median removal of 100, 44.5% of samples with <1 CFU/100 mL). Yet, this treatment was less effective for river water (94% median removal, with 11% of samples with <100 CFU/100 mL and only 5.5% with <1 CFU/100 mL found in treated water), showing a significant reduction in both cases when the Wilcoxon test was applied. Sodium hypochlorite treatment showed the best results among the techniques evaluated in this study. It can be used in remote areas where rainwater is available for consumption. Microorganism concentration increased after water underwent water straining and sedimentation processes. These results suggest that the improper handling of water containers and materials used during treatment processes leads to contamination of water. Thus, more robust outreach and educational efforts are recommended to improve remote communities’ water collection, treatment, and storage practices.
1 Introduction
Universal and equitable access to safe drinking water across the globe is an international priority and is part of Sustainable Development Goal number 6, as established by the United Nations (UNICEF, 2019). In different regions of the world, urban and rural populations lack access to drinking water. In Northern Brazil, home to the Amazon biome, 25% of households are not connected to a water supply network; this is well above the national average of 8% (IBGE, 2019). Rainwater and river waters are rural populations’ main drinking water sources (Cardoso, 2021; Gomes et al., 2022). The Amazon hosts high levels of biodiversity and provides ecosystem services globally, including carbon sequestration (Strand et al., 2018; Joly et al., 2019). Sustainable development is the most viable strategy for biodiversity conservation and maintaining ecosystem services. This development model concurrently seeks to improve the quality of life of local populations (Campos-silva et al., 2018; Franco et al., 2021).
The riverine people, recognized as traditional communities in Brazil, exist in a challenging environment for human development due to the annual flood pulses, waterway-only transport, long distances from urban centers, and a lack of infrastructure (de Andrade et al., 2021). Most of them live in wood-made stilt houses along the main rivers, with gain income from extractivism, fishing, agriculture, and services. Access to safe drinking water is fundamental to quality of life and is urgent for Amazonian populations.
Since the beginning of COVID-19, actions to prevent emerging diseases and new global pandemics have been urgent. Households in remote areas are in contact with wild animals, and populations are subject to zoonoses from the crossover of pathogenic organisms from wild and domestic non-human and human species (Ellwanger et al., 2020). In this context, high-quality water is a barrier to emerging diseases, as the pollution of waters, soils, and other objects also promotes widespread exposure to pathogenic microorganisms (O’Brien and Xagoraraki, 2019).
Household or point-of-use (POU) water treatment is encouraged in the absence of safe water supply systems to reduce diarrheal diseases (WHO, 2017). Nevertheless, positive effects depend on correct, effective, consistent, and continued use of these methods (Bivins et al., 2019). POU treatments are only effective when more than 90% of water consumed at home is being treated (Brown and Clasen, 2012) – or, in other words, great adherence to these practices is sustained (UNICEF, 2019). The quality of treated water is used to classify the risk level for health diseases (WHO, 2012). In the Central Amazon, many families use traditional POU practices, such as sedimentation, cloth filtrations, and hypochlorite. Sodium hypochlorite is employed by 43% of all households as the only treatment technique, and it is combined with other techniques at 25% of households (Gomes et al., 2022). Despite the widespread use of POU, its efficiency in improving water quality is unknown. Thus, this study aimed to evaluate the effectiveness of removing solids and microbial contamination of household water treatments in riverine communities from Central Amazon.
In this study we sampled water untreated and treated (through traditional household water treatment), from river and rainwater. Thus, we have identified cases where treatment techniques, and handling practices have not been effectively utilized to make the water potable and propose specific efforts that the government and institutions should undertake to enhance water quality.
2 Materials and methods
We collected POU-treated water from 18 residences belonging to three communities (Caburini, Sítio Fortaleza, and Várzea Alegre) located in a floodplain area in the middle Solimões region, Amazonas State, Central Amazon, Brazil (Figure 1). Here, we also conducted observations to learn more about household treatments.
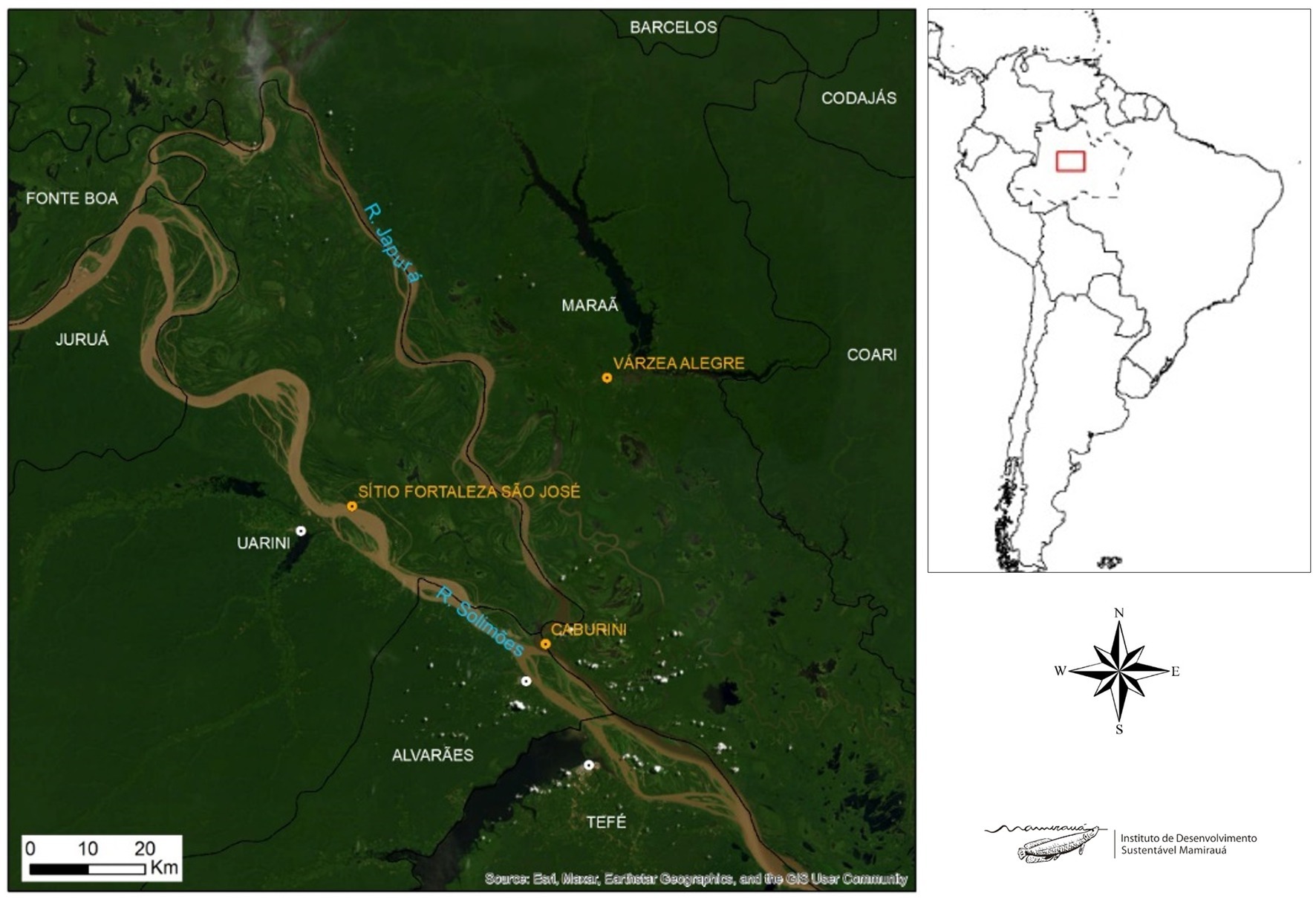
Figure 1. Location map of study area in the Central Amazon with yellow points at the communities where samples were collected.
Rainwater samples were collected during the rainy season (Dec/2021 to Feb/2022). River water samples were collected during the dry season (July to October 2022) – when families rely more heavily on this water source.
For rainwater, we evaluated three treatment techniques: cloth filtration (C), chlorine disinfection (Cl), and cloth filtration + chlorine disinfection (C + Cl). For river water, five-technique combinations were tested (all of which included at least one sedimentation step): sedimentation (S); sedimentation + cloth filtration (S + C); sedimentation + cloth filtration + chlorine disinfection (S + C + Cl); sedimentation + chlorine disinfection (S + Cl); flocculant + chlorine disinfection (F + Cl). The commercial flocculant Water Purifier P&G® was used as a reference, as some families in the region have had access to this treatment type since 2017 (FAS, 2019). Sodium hypochlorite, distributed by the Brazilian government, has a concentration around 2.38% of active chlorine (Ministério da Saúde, 2018).
Residents were asked to carry out daily water treatment techniques as usual. Water samples were collected at each step of the treatment process, and details about the practice were described and recorded. Drinking water samples were collected from household containers readily available for drinking, and residents described the origin and type of water treatment conducted.
Samples were placed inside sterile flasks and bags containing sodium thiosulphate when containing chlorine, transported at 4°C to the laboratory at the Mamirauá Institute (Tefé/AM) in thermal boxes, and packed and processed within 48 h after collection.
2.1 Water quality analyses
The free chlorine concentration was measured (photometer Hanna® HI 97710) between 25 and 30 min after adding sodium hypochlorite and between 20 and 30 min after mixing P&G® flocculant, as recommended by the manufacturers.
In the laboratory, we evaluated the following: true and apparent color (Hach® DR3900 spectrophotometer, 465 nm); total coliform and Escherichia coli [Method 9222 membrane filtration (APHA, 2005)]; turbidity (turbidimeters Policontrol® AP2000 for rainwater and Hanna® HI93793 for river water).
2.2 Data analysis and statistics
The concentration of E. coli in treated water was classified according to health risk levels, from “No Risk” to “Very High Risk” (WHO, 2012).
Data distribution was evaluated using Normal Probability charts and Shapiro–Wilk adherence test. The Wilcoxon test for paired data comparison was used to analyze the differences in quality between untreated and treated water pairs, with each type of treatment evaluated separately for river water and rainwater. A 95% confidence interval was considered (significant p < 0.05). Statistica10 software was used in the analyses.
2.3 Ethical aspects
The study was approved on ethical grounds and presented no risk to participant groups (Certificate of Presentation of Ethical Appreciation No. 42236920.7.0000.8117). Participants signed a Free and Informed Consent Form to participate in the research.
3 Results
The initial results comprise a description of the household water treatments based on the observations, followed by an assessment of their effectiveness in enhancing the quality of river and rainwater.
3.1 Description of traditionally used water treatment techniques
Cloth filter (straining): Rainwater is filtered at its source or POU (Figure 2A). Residents used the following fabrics: old T-shirts, cotton kitchen towels, bed linen fabrics, non-woven fabric, and cloth coffee filters; most used a single layer (no folds) of these materials. Some fabrics are used exclusively for filtering water, while others are used to dry kitchen utensils and surfaces. Families use buckets, plastic bottles, and pans as water containers. Buckets and pans are generally used for water collection and other household uses.
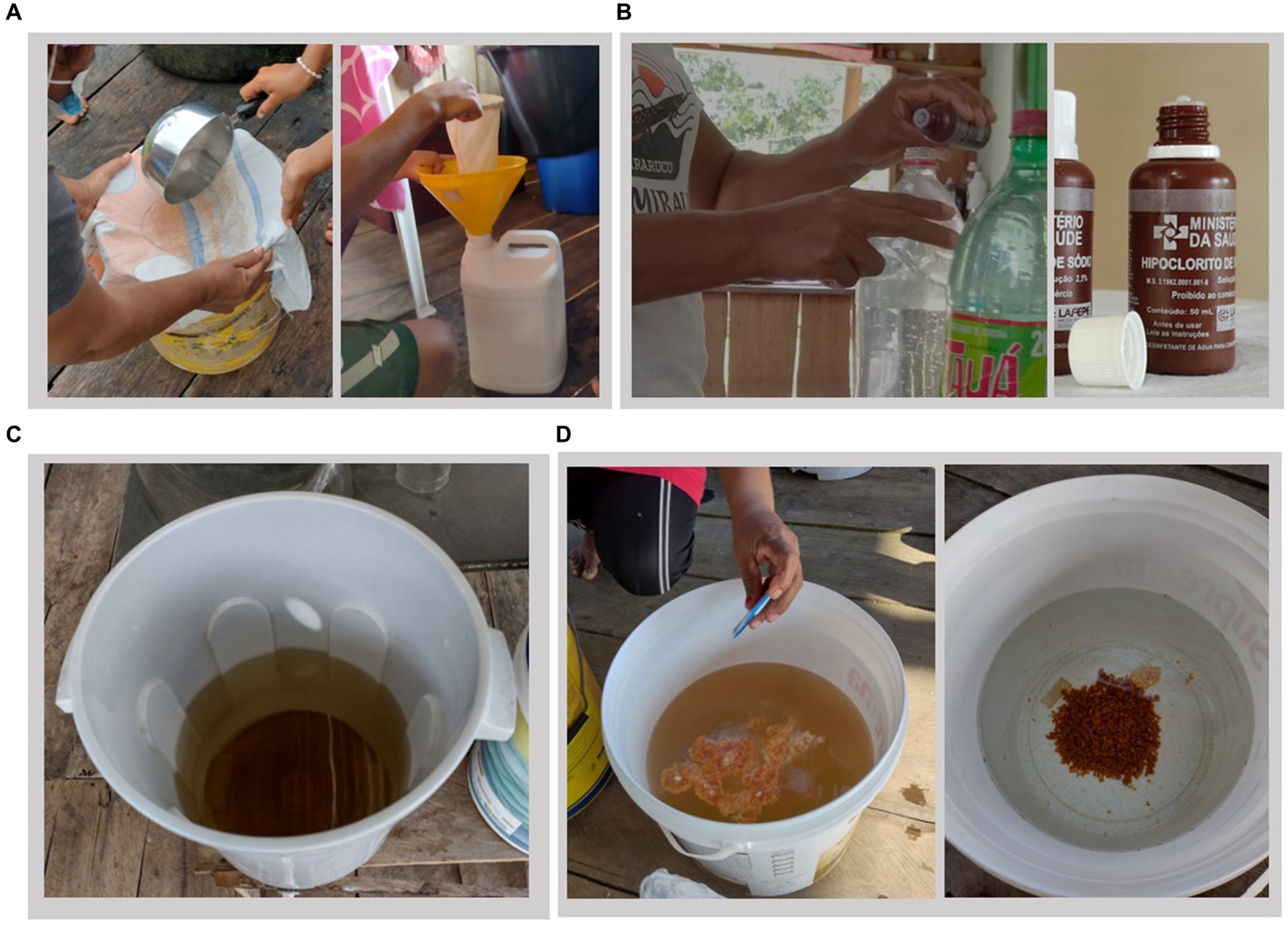
Figure 2. Household water treatments: (A) straining water with cloth filter C, (B) addition of chlorine Cl, (C) sedimentation process S, (D) P&G Water Purifier F + Cl.
Chlorination: Water is treated with sodium hypochlorite solution, usually added to drinking water receptacles (buckets, bottles, etc.) or sometimes directly to the tank used to collect rainwater (Figure 2B). The chlorinated water is left to stand for at least 30 min before consumption. Doses of 0.25–1.5 drops per liter in rainwater and 1.0–2.5 drops per liter in river water were used. Some participants reported that adding more chlorine would result in a bad taste in the water.
Sedimentation: In this process, river water is collected in buckets or plastic gallons of up to 20 L in the afternoon. The water then sits at rest for 15–17 h (most commonly) up to 36 h. Water is then poured into a large storage container and is strained with a cloth filter. Water collection and sedimentation containers are not used exclusively for these purposes and may be used for other domestic activities. The recipient has a lid or is covered with cloth (Figure 2C).
Water Purifying Flocculant (P&G)®: The contents of the sachet (5 g) are added to a bucket of 10 L of water and mixed with a spoon. After mixing, residents wait for the water to settle until it becomes clear – being left for up to one night. Water is then strained into the water storage. No particular attention was paid to the recommended mixing and rest time of 20 min after straining (Figure 2D).
3.2 How effective are the household water treatment methods?
In riverine communities in Central Amazonia, river and rainwater collected by families have a high concentration of total coliform and E. coli (Table 1) and need to be treated before consumption. Raw rainwater and river water showed similar patterns concerning E. coli concentration, being close to 3.5 log CFU/100 mL (Table 1).
Table 2 summarizes the efficiency of the methods for each contamination. The treatment steps for the separation of solids in river water were partially effective only for removing turbidity (12–22% of turbidity) and apparent color (18–26% of apparent color). Pouring rainwater through cloth filters (water straining) (C) did not reduce any of the evaluated parameters (Table 1).
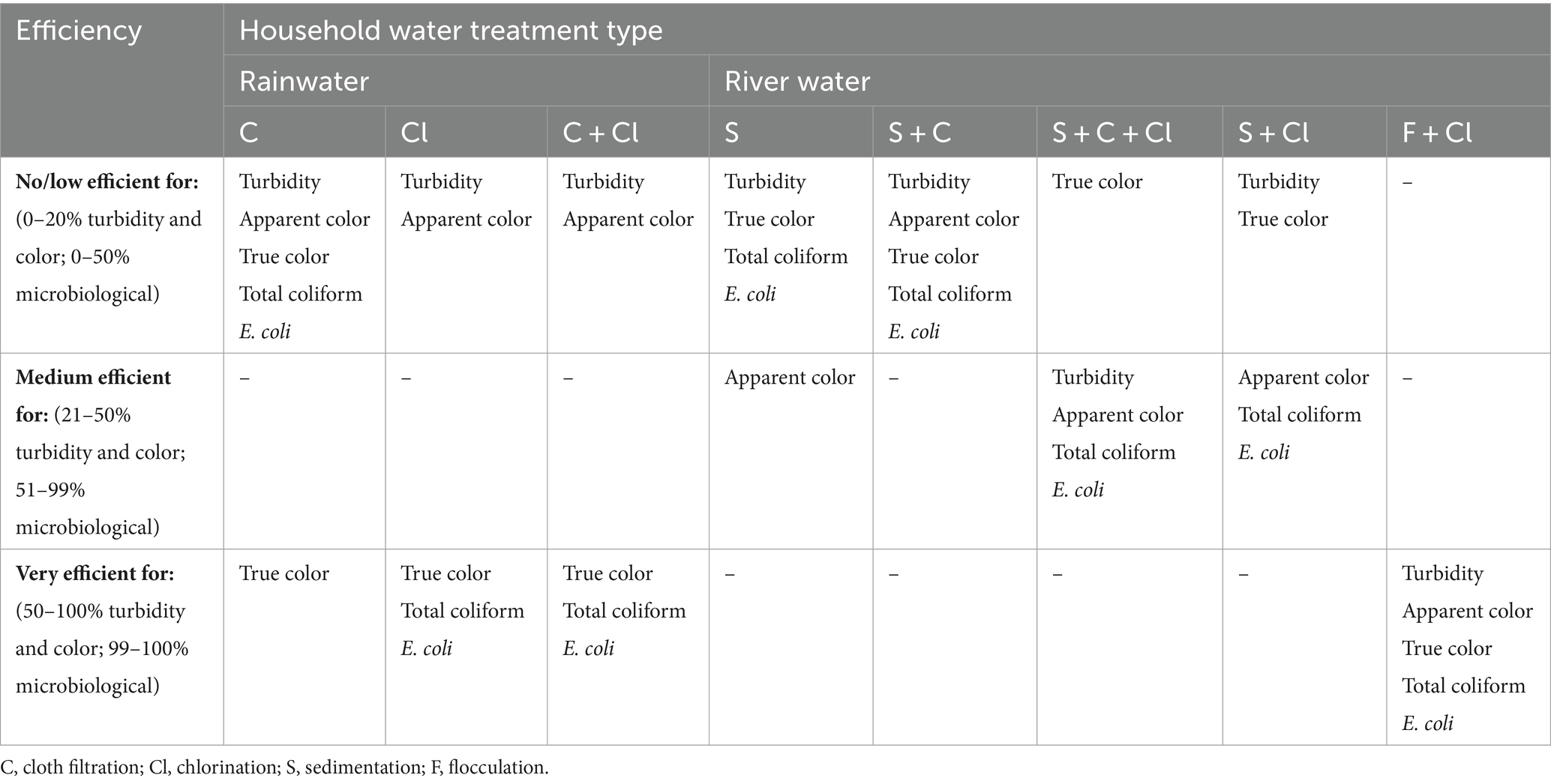
Table 2. Summary of the efficiency of traditional rainwater and river water treatments in study area.
The combined sedimentation and cloth filtration method (S + C) of river water had a median removal of 12% of the initial turbidity, with a minimum value of 33 NTU for treated water (Table 1). Comparison between the S and S + C methods indicates that straining river water after sedimentation had no relevant effect on water quality; in fact, it increased turbidity concentration (from 36 to 39 NTU). Also, an increase in microorganisms was observed after sedimentation processes.
Chlorination of rainwater (Cl) was efficient in changing the water quality (p < 0.05) by inactivating E. coli, with a median of 3.46 log CFU/100 mL in untreated water and 0 log CFU/100 mL in chlorinated water. Eighty-nine percent of the chlorinated rainwater samples obtained No Risk and Low Health Risk levels (Figure 3). The median dose of Cl applied to river water was 1.2 mg/L. Chlorine was effective for rainwater disinfection (median 100%) but not for river water (median 94%).
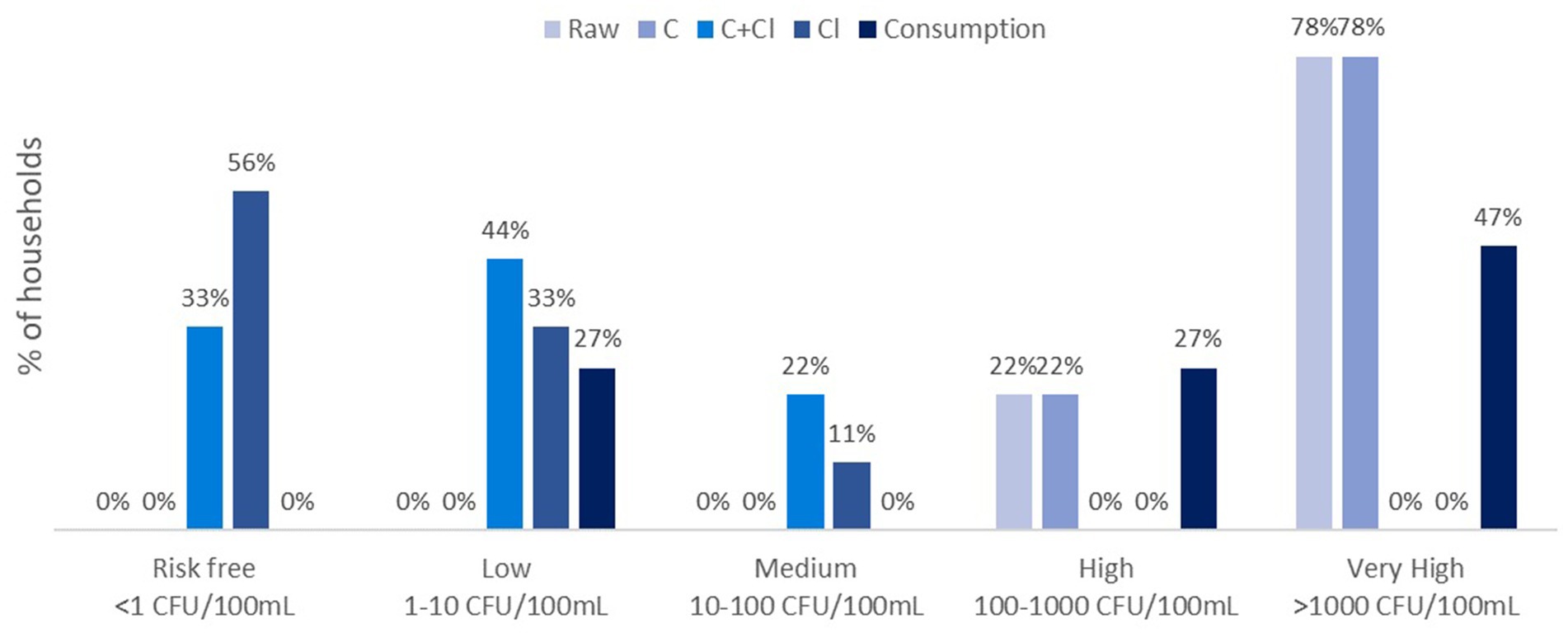
Figure 3. The concentration of E. coli in rainwater collected in the study area, grouped by WHO (2012) health risk levels.
Regarding health risks for local water consumption (Figure 3), rainwater samples treated with chlorine were classified as No Risk, Low Risk, and Medium Risk. In contrast, the other samples were distributed in the highest risk levels. Importantly, C + Cl treated rainwater samples also significantly reduced E. coli (p < 0.05).
The application of sodium hypochlorite to decanted river water (S + Cl) reduced total coliforms by 95% (Table 1) and did not result in disinfection, with 11% of the samples having <100 CFU/100 mL and 5.5% having <1 CFU/100 mL (Figure 4). The addition of the cloth filtration step (S + C + Cl) did not change the turbidity, color, or coliforms (Table 1) compared to its absence (S + Cl).
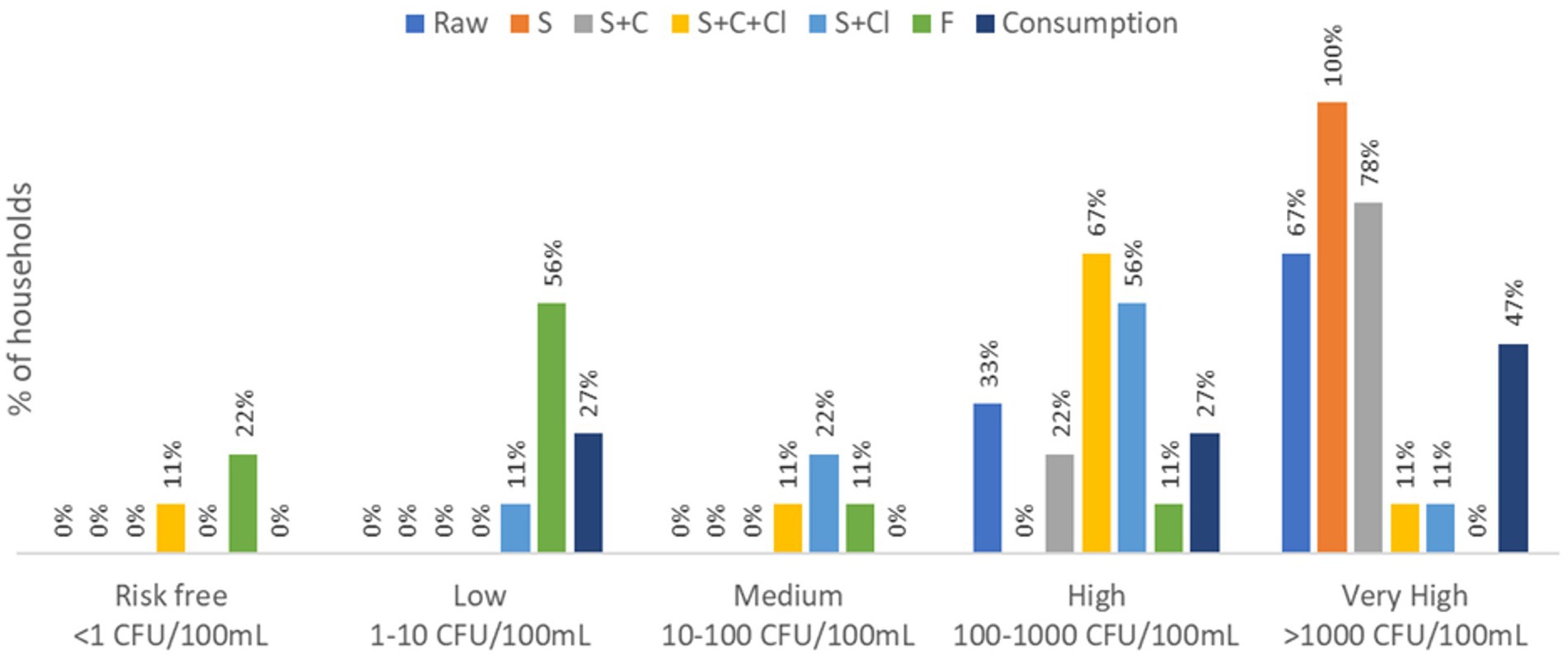
Figure 4. Concentration of E. coli in river water samples collected in the study area, grouped by WHO (2012) health risk levels.
The Water Purifier® (F + Cl)—used as a comparative reference in this study—was the most effective water treatment technique. It removed 99% of turbidity (0.3 NTU in treated water), 94% of true color, and 2.39 log CFU/100 mL of E. coli (Table 1).
Despite the predominant preference for rainwater for drinking purposes (Table 3), with only one resident in a study household using a mixture of river and rainwater, the quality of this drinking water was unproper. Drinking water (n = 15) showed a level of contamination similar to that of untreated rainwater (2.9 and 3.5 log E. coli/100 mL). Notably, all samples, including samples treated with chlorine, showed some level of contamination by E. coli (Figure 3).
4 Discussion
Fecal contamination of untreated rainwater (median 3.46 log CFU/100 mL E. coli) can occur during water collection and storage. Rainwater can become contaminated when running off roofs and into gutters (or uncovered reservoirs) by animals (bats, rats, possums, vultures) or through atmospheric pollution (Novaes and Cintra, 2013; Ahmed et al., 2014; Hamilton et al., 2019). E. coli bacteria deposited on rooftops in animal feces only remain active for 2 h on sunny days; however, they survive from 9 to 53 h in cloudy conditions (Ahmed et al., 2014) and can be carried to household water reserves when it rains. In water reservoirs (tanks), 90% of bacteria will become inactive (Ahmed et al., 2014) after 38–72 h, and recontamination may also occur during this period.
Water straining (C), a common POU, was ineffective and eventually damaging (Table 1) for microbiological water quality. The absence of larger suspended solids in rainwater, such as plankton (Colwell et al., 2003) for adhesion of microorganisms (Ali et al., 2011) potentially retained in the straining process, may have negatively affected the effectiveness of this method. Kotlarz et al. (2009) also reported that straining water with a single layer of fabric showed low effectiveness in reducing the turbidity of slightly turbid water. Enterobacteria are typically 2–3 μm in length and 0.6 μm in thickness (Rogers et al., 2016) – and are not removed during cloth filtration.
Positive results in straining efficacy could be obtained in the field for surface waters (rivers and lakes), with a 50% removal of turbidity using 16 layers of fabric or four folds (Ali et al., 2011). Old fabrics, which have been washed several times, are more effective in treating water due to the reduction in pore size (Huq et al., 2010). In this study, since fabric wear does not negatively impact the straining process of river water, the use of a single layer of fabric is likely to explain the results found. Nonetheless, as total coliform and E. coli quality indicators could be abundant on surfaces, objects, and fabrics under inadequate hygienic conditions, the water handling potentially altered the results of water straining (Zimmer and Dorea, 2023). For example, better fabric hygiene could be achieved by rinsing with chlorinated water or heating.
Natural sedimentation (S), for 16–18 h (overnight), reduced the apparent color (11%) and turbidity of the river water (p < 0.05) but had only 16% efficacy and a final value of 36 NTU above the 0.5 NTU portability limit (Ministério da Saúde, 2021). Longer settling times could change the results. With controlled values of 10–300 NTU for untreated water, sedimentation for 24 h had a 79–87% efficacy rate in reducing turbidity (Kotlarz et al., 2009).
The increased number of microorganisms after the sedimentation processes was also observed in previous studies about water storage for domestic use (Mintz et al., 1995; Yi et al., 2019). Despite the coverage of storage containers, contact with unhygienic hands and objects can lead to contamination of treated and untreated water. Microorganisms in biofilm covering the surfaces of containers, such as buckets and bottles, can survive for more than 48 h. They can also detach, becoming a continuous source of water contamination (Momba and Kaleni, 2002). Other microorganisms such as E. coli, Salmonella sp. and coliphages persist during water storage and display little growth (Momba and Kaleni, 2002). E. coli is a commensal bacterium that lives in the intestinal tract of humans and vertebrate animals (Berthe et al., 2013). Researchers are studying the possibility of the multiplication of these bacteria outside the intestinal tract in the case of phylogenetic groups that contain strains adapted to the conditions of the aquatic environment (Nowicki et al., 2021).
Water turbidity provides suitable conditions for bacterial multiplication through the association of nutrients with organic carbon (Momba and Kaleni, 2002; Yi et al., 2019). It is likely that, after the proliferation of bacteria immediately following water straining (with contaminated cloth), a decay process would begin due to nutrient and oxygen reduction, in addition to changes in temperature, and other factors (Allwood et al., 2003; Blaustein et al., 2013).
Removing turbidity from water before disinfection influences its efficacy, as it reduces the demand for chlorine by reducing oxidizable components in the water (Kotlarz et al., 2009). Removing turbidity through sedimentation processes and other simplified techniques can guarantee the maintenance of residual free chlorine for up to 24 h after chlorination (Kotlarz et al., 2009). Free chlorine, in turn, prevents recontamination of water during storage (WHO, 2017).
Adding sodium hypochlorite did not effectively disinfect river water due to its high turbidity (more than 31 NTU). In decanted river water (S + Cl treatment), chlorination substantially reduced E. coli by 95% (Table 1), but only 5.5% of the samples measured <1 CFU/100 mL (Figure 4). However, the correct chlorination dose for this turbidity level is 3.75 mg/L of chlorine (Lantagne, 2008), representing 4 drops/L addition. The Brazilian Ministry of Health recommends a standard dose of 2 drops/L – and in practice, smaller doses are used (median 1.2 mg/L). Considering that the local population rejects the taste of chlorine, it is likely that residents would disregard the recommendation to use even higher doses of chlorine.
The household treatment of river water with the best result of the risk of water consumption was sedimentation, followed by sodium hypochlorite (S + Cl) (Figure 4). This technique had the highest percentage of samples (33%) in the No risk to Medium risk classes (WHO, 2012).
The Water Purifier (F + Cl) method combines coagulation of sediments and water disinfection by the simultaneous use of ferrous sulfate and calcium hypochlorite (Souter et al., 2003), thus having advantages over the simple and natural techniques used in this study. Chemical coagulants and combination products are challenging to purchase compared to sodium hypochlorite, which is distributed free of charge by the Brazilian government. Its relative inaccessibility makes it less sustainable to use in the long term. Studies have reported a 44% reduction in per capita consumption of treated water 2 months after the delivery of flocculant sachets despite intensive follow-up (Shaheed et al., 2018). Using devices like filters results in greater adherence and prolonged use (Sobsey et al., 2008). Promoting a technique that depends on the continuous distribution of the product is not a viable medium/long-term solution, being more suitable for emergencies (Ariel Branz et al., 2017).
While in the region, 67% of families had reported using chlorine for water treatment (Gomes et al., 2022), this study found that only 18% of families reported using chlorine for this purpose; we detected the presence of residual chlorine in a mere 8% of samples. Brown and Sobsey (2012) also found a difference between families who stated that they boiled water (>90%) and those who boiled it (31%). In rural Peru, only 23% of the families who had reported POU water treatment consistently did so, which may indicate a failure to collect “good” data (Rosa et al., 2014), but also poor adherence to treatment or its inappropriate use.
The sodium hypochlorite distribution program (around 2.5% active chlorine solution) in Brazil is widespread and involves Community Health Agents (CHAs). Considering its wide reach and impact, we recommend further training of CHAs to raise public awareness on the proper use of chlorine. This would enhance the effectiveness of the investment and promote public health by reducing diseases and health consequences like malnutrition and child mortality. Improving and monitoring the quality of drinking water in riverine communities in Amazonia is urgent to promote the well-being of the local population. Additionally, it would improve preparedness for water access during emergencies, such as extreme droughts and heavy rainfall events, which are increasingly common in the Amazon (Flores et al., 2024).
5 Conclusion
The riverine population lives in scarcity of drinking water despite individual efforts to treat water at home. Considering the analyzed parameters, the water available could not be classified as safe for human consumption even after treatment. Therefore, emergency investment in the topic is needed, thus contributing to quality of life and health barriers for unknown emerging pathogens.
Improving the techniques used, particularly the use of hypochlorite and the straining of water (cloth filter), could potentially promote greater water treatment efficacy. Treating rainwater with sodium hypochlorite had the best results despite observing that residents were using doses below the recommended level. This thus represents the most recommended treatment of all those evaluated. No treatment effectively removed turbidity or disinfected river water. Therefore, investments are needed to improve the efficacy of removing solids from water; improvements to filters or coagulants may show promise.
Investing in education and outreach initiatives to teach proper cleaning of utensils and containers used in water treatment and storage is imperative. These measures are recommended to enhance treatment efficacy for local families, along with investments in adequate water supply infrastructure delivering potable water.
Data availability statement
The raw data supporting the conclusions of this article will be made available by the authors, without undue reservation.
Ethics statement
The studies involving humans were approved by Comitê de Ética em Pesquisa Instituto de Desenvolvimento Sustentável Mamirauá Plataforma Brasil/CONEP. The studies were conducted in accordance with the local legislation and institutional requirements. The participants provided their written informed consent to participate in this study.
Author contributions
MG: Writing – original draft, Writing – review & editing. LA: Writing – review & editing. MB: Writing – review & editing. BL: Writing – review & editing. CM: Supervision, Writing – review & editing.
Funding
The author(s) declare financial support was received for the research, authorship, and/or publication of this article. This research was funded by the National Council for Scientific and Technological Development (CNPq/PCI, Process No. 301152/2019-5) and Banco do Brasil Foundation (Project Agreement No. 20415).
Acknowledgments
The authors thank the Brazilian Ministry of Science, Technology and Innovation (MCTI), the National Council for Scientific and Technological Development, the Bank of Brasil Foundation, the National Institute of Science and Technology for Sustainable Wastewater Treatment (INCT/ETES Sustentáveis), and the Research Support Foundation of the State of Minas Gerais (FAPEMIG).
Conflict of interest
The authors declare that the research was conducted in the absence of any commercial or financial relationships that could be construed as a potential conflict of interest.
Publisher’s note
All claims expressed in this article are solely those of the authors and do not necessarily represent those of their affiliated organizations, or those of the publisher, the editors and the reviewers. Any product that may be evaluated in this article, or claim that may be made by its manufacturer, is not guaranteed or endorsed by the publisher.
References
Ahmed, W., Richardson, K., Sidhu, J. P. S., Jagals, P., and Toze, S. (2014). Inactivation of faecal indicator bacteria in a roof-captured rainwater system under ambient meteorological conditions. J. Appl. Microbiol. 116, 199–207. doi: 10.1111/jam.12342
Ali, S. I., MacDonald, M., Jincy, J., Sampath, K. A., Vinothini, G., Philip, L., et al. (2011). Efficacy of an appropriate point-of-use water treatment intervention for low-income communities in India utilizing Moringa oleifera, sari-cloth filtration and solar UV disinfection. J. Water Sanit. Hygiene Dev. 1, 112–123. doi: 10.2166/washdev.2011.043
Allwood, P. B., Malik, Y. S., Hedberg, C. W., and Goyal, S. M. (2003). Survival of F-specific RNA Coliphage, feline Calicivirus, and Escherichia coli in water: a comparative study 69, 5707–5710. doi: 10.1128/AEM.69.9.5707-5710.2003
APHA (2005). Standard methods for the examination of water and wastewater. 15th Edn. Whashington, DC: American Public Health Association.
Ariel Branz, A. B., Matthew Levine, M. L., Lilian Lehmann, L. L., Andy Bastable, A. B., Syed Imran Ali, S. I. A., Khalid Kadir, K. K., et al. (2017). Chlorination of drinking water in emergencies: a review of knowledge to develop recommendations for implementation and research needed. Waterlines 36, 4–39. doi: 10.3362/1756-3488.2017.002
Berthe, T., Ratajczak, M., Clermont, O., Denamur, E., and Petit, F. (2013). Evidence for coexistence of distinct Escherichia coli populations in various aquatic environments and their survival in estuary water. Appl. Environ. Microbiol. 79, 4684–4693. doi: 10.1128/AEM.00698-13
Bivins, A., Beetsch, N., Majuru, B., Montgomery, M., Sumner, T., and Brown, J. (2019). Selecting household water treatment options on the basis of World Health Organization performance testing protocols 53, 5043–5051. doi: 10.1021/acs.est.8b05682
Blaustein, R. A., Pachepsky, Y., Hill, R. L., Shelton, D. R., and Whelan, G. (2013). Escherichia coli survival in waters: temperature dependence. Water Res. 47, 569–578. doi: 10.1016/j.watres.2012.10.027
Brown, J., and Clasen, T. (2012). High adherence is necessary to realize health gains from water quality interventions. PLoS One 7:e36735. doi: 10.1371/journal.pone.0036735
Brown, J., and Sobsey, M. D. (2012). Boiling as household water treatment in Cambodia: a longitudinal study of boiling practice and microbiological effectiveness. Am. J. Trop. Med. Hyg. 87, 394–398. doi: 10.4269/ajtmh.2012.11-0715
Campos-Silva, J. V., Hawes, J. E., Andrade, P. C. M., and Peres, C. A. (2018). Unintended multispecies co-benefits of an Amazonian community-based conservation programme. Nat. Sustain. 1, 650–656. doi: 10.1038/s41893-018-0170-5
Cardoso, J. D. S. (2021). Narrativa sobre água e os saberes ambientais Baniwa: uma contribuição para o ensino de ciências na escola indígena de educação básica. [dissertation]. São Gabriel da Cachoeira (Brasil): Federal University of Amazonas.
Colwell, R. R., Huq, A., Islam, M. S., Aziz, K. M. A., Yunus, M., Khan, N. H., et al. (2003). Reduction of cholera in Bangladeshi villages by simple filtration. Proc. Natl. Acad. Sci. U. S. A. 100, 1051–1055. doi: 10.1073/pnas.0237386100
de Andrade, L. C., Borges-Pedro, J. P., Gomes, M. C. R. L., Tregidgo, D. J., do Nascimento, A. C. S., Paim, F. P., et al. (2021). Discover sustainability the sustainable development goals in two sustainable development reserves in central amazon: achievements and challenges. Discov. Sustain. 2:54. doi: 10.1007/s43621-021-00065-4
Ellwanger, J. H., Kulmann-Leal, B., Kaminski, V. L., Valverde-Villegas, J. M., DA Veiga, A. B. G., Spilki, F. R., et al. (2020). Beyond diversity loss and climate change: impacts of Amazon deforestation on infectious diseases and public health. An. Acad. Bras. Cienc. 92:e20191375. doi: 10.1590/0001-3765202020191375
FAS (2019). Soluções para água potável em áreas remotas da Amazônia, 25. Available at: https://fas-amazonia.org/wp-content/uploads/2022/12/infraestrutura-solucoes-aguaacesso_compressed.pdf
Flores, B. M., Montoya, E., Sakschewski, B., Nascimento, N., Staal, A., Betts, R. A., et al. (2024). Critical transitions in the Amazon forest system. Nature 626, 555–564. doi: 10.1038/s41586-023-06970-0
Franco, C. L. B., el Bizri, H. R., Souza, P. R., Fa, J. E., Valsecchi, J., Sousa, I. S., et al. (2021). Community-based environmental protection in the Brazilian Amazon: recent history, legal landmarks and expansion across protected areas. J. Environ. Manag. 287:112314. doi: 10.1016/j.jenvman.2021.112314
Gomes, M. C. R. L., Andrade, L. C., Nascimento, A. C. S., Pedro, J. P. B., and Filho, C. R. M. (2022). Conditions of use and levels of household access to water in rural communities in the Amazon. Ambiente Soc. 25:e01782. doi: 10.1590/1809-4422asoc20210178r12vu2022l4oa
Hamilton, K., Reyneke, B., Waso, M., Clements, T., Ndlovu, T., Khan, W., et al. (2019). A global review of the microbiological quality and potential health risks associated with roof-harvested rainwater tanks. npj Clean Water 2:7. doi: 10.1038/s41545-019-0030-5
Huq, A., Yunus, M., Sohel, S. S., Bhuiya, A., Emch, M., Luby, S. P., et al. (2010). Simple sari cloth filtration of water protect villagers from cholera in Matlab, Bangladesh. Knowl. Global Lifesaving Solut. 1, 1–5. doi: 10.1128/mBio.00034-10.Invited
IBGE (2019). Pesquisa Nacional por Amostragem de Domicílios (PNAD) Contínua. Available at: www.ibge.gov.br/estatisticas/sociais
Joly, C. A., Scarano, F. R., Seixas, C. R., Metzger, J. P., Ometto, J. P., Bustamante, M. M. C., et al. (2019). 1o Diagnóstico Brasileiro de Biodiversidade & Serviços Ecossistêmicos. São Carlos (Brasil): BPBES.
Kotlarz, N., Lantagne, D., Preston, K., and Jellison, K. (2009). Turbidity and chlorine demand reduction using locally available physical water clarification mechanisms before household chlorination in developing countries. J. Water Health 7, 497–506. doi: 10.2166/wh.2009.071
Lantagne, D. (2008). Sodium hypochlorite dosage for household and emergency water treatment. J. AWWA 100, 106–119. doi: 10.1002/j.1551-8833.2008.tb09704.x
Ministério da Saúde (2018). Quality of water for human consumption: Booklet for promotion and protection of health, Ministério da Saúde. Brasília: Ministério da Saúde Available at: http://bvsms.saude.gov.br/bvs/publicacoes/qualidade_agua_pro-mocao_protecao_saude.pdf.
Ministério da Saúde (2021). Portaria GM/n. 888, de 4 de maio de 2021. Brasília (Brasil): Gabinete do Ministro.
Mintz, E., Reiff, F., and Tauxe, R. (1995). Safe water treatment and storage in the home: a practical new strategy to prevent waterborne disease. JAMA 273, 948–953. doi: 10.1001/jama.1995.03520360062040
Momba, M. N. B., and Kaleni, P. (2002). Regrowth and survival of indicator microorganisms on the surfaces of household containers used for the storage of drinking water in rural communities of South Africa. Water Res. 36, 3023–3028. doi: 10.1016/S0043-1354(02)00011-8
Novaes, W. G., and Cintra, R. (2013). Factors influencing the selection of communal roost sites by the black vulture Coragyps atratus (Aves: Cathartidae) in an urban area in Central Amazon. Fortschr. Zool. 30, 607–614. doi: 10.1590/S1984-46702013005000014
Nowicki, S., deLaurent, Z., de Villiers, E. P., Githinji, G., and Charles, K. J. (2021). The utility of Escherichia coli as a contamination indicator for rural drinking water: evidence from whole genome sequencing. PLoS One 16, 1–23. doi: 10.1371/journal.pone.0245910
O’Brien, E., and Xagoraraki, I. (2019). A water-focused one-health approach for early detection and prevention of viral outbreaks. One Health 7:100094. doi: 10.1016/j.onehlt.2019.100094
Rogers, L., Power, K., Gaora, P. Ó., and Fanning, S. (2016). Escherichia coli and other Enterobacteriaceae: occurrence and detection. Encyclopedia of food and health. 1st Edn: Elsevier Ltd.
Rosa, G., Huaylinos, M. L., Gil, A., Lanata, C., and Clasen, T. (2014). Assessing the consistency and microbiological effectiveness of household water treatment practices by urban and rural populations claiming to treat their water at home: a case study in Peru. PLoS One 9:e114997. doi: 10.1371/journal.pone.0114997
Shaheed, A., Rathore, S., Bastable, A., Bruce, J., Cairncross, S., and Brown, J. (2018). Adherence to point-of-use water treatment over short-term implementation: parallel crossover trials of flocculation-disinfection sachets in Pakistan and Zambia. Environ. Sci. Technol. 52, 6601–6609. doi: 10.1021/acs.est.8b00167
Sobsey, M. D., Stauber, C. E., Casanova, L. M., Brown, J. M., and Elliott, M. A. (2008). Point of use household drinking water filtration: a practical, effective solution for providing sustained access to safe drinking water in the developing world. Environ. Sci. Technol. 42, 4261–4267. doi: 10.1021/es702746n
Souter, P. F., Cruickshank, G. D., Tankerville, M. Z., Keswick, B. H., Ellis, B. D., Langworthy, D. E., et al. (2003). Evaluation of a new water treatment for point-of-use household applications to remove microorganisms and arsenic from drinking water. Available at: https://www.pg.com/pghsi/safewater/pdf/souterdoc.pdf (Accessed April 19, 2019).
Strand, J., Soares-Filho, B., Costa, M. H., Oliveira, U., Ribeiro, S. C., Pires, G. F., et al. (2018). Spatially explicit valuation of the Brazilian Amazon forest’s ecosystem services. Nat. Sustain. 1, 657–664. doi: 10.1038/s41893-018-0175-0
UNICEF (2019). Progress on household drinking water, sanitation and hygiene 2000–2017. Special focus on inequalities. New York: United Nations Children’s Fund (UNICEF) and World Health Organization. Available at: https://www.unicef.org/reports/progress-on-drinking-water-sanitation-and-hygiene-2019
WHO (2012). A toolkit for monitoring and evaluating household water treatment and safe storage programmes. 1st Edn. Geneva: World Health Organization.
WHO (2017) in Guidelines for drinking-water quality: fourth edition incorporating the first addendum. ed. World Health Organization. 1st ed (Geneva: World Health Organization).
Yi, J., Lee, J., Jung, H., Park, P. K., and Noh, S. H. (2019). Reduction of bacterial regrowth in treated water by minimizing water stagnation in the filtrate line of a gravity-driven membrane system. Environ. Eng. Res. 24, 17–23. doi: 10.4491/eer.2018.048
Keywords: waterborne diseases, SDG6, rainwater, river, water safety, Amazonia, riverine people
Citation: Gomes MCRL, de Andrade LC, Barbosa MP, Lopes BC and Mota Filho CR (2024) Performance of traditional household drinking water treatment methods used in rural Amazon. Front. Water. 6:1392800. doi: 10.3389/frwa.2024.1392800
Edited by:
Priscila Neves Silva, Oswaldo Cruz Foundation (Fiocruz), BrazilReviewed by:
Gagan Matta, Gurukul Kangri University, IndiaDavi Victral, Oswaldo Cruz Foundation (Fiocruz), Brazil
Copyright © 2024 Gomes, de Andrade, Barbosa, Lopes and Mota Filho. This is an open-access article distributed under the terms of the Creative Commons Attribution License (CC BY). The use, distribution or reproduction in other forums is permitted, provided the original author(s) and the copyright owner(s) are credited and that the original publication in this journal is cited, in accordance with accepted academic practice. No use, distribution or reproduction is permitted which does not comply with these terms.
*Correspondence: Maria Cecilia Rosinski Lima Gomes, engceciliagomes@gmail.com