- 1Water Global Practice, World Bank, Washington, DC, United States
- 2Land and Water Management Department, IHE Delft Institute for Water Education, Delft, Netherlands
Driven by increasing water demand, scarcity concerns, and climate change impacts, numerous countries prioritize solutions for enhanced water use efficiency. However, these solutions often focus primarily on managing water quantities to improve water productivity in agriculture, urban, and industrial sectors. Effective and sustainable water use, however, requires monitoring and management of both water quantity and quality. Traditionally, water quantity and water quality have been managed separately, often by different government agencies with different missions and limited interaction. Ensuring sufficient water quantity for agriculture and food production often takes precedence over managing water quality. Water accounting, as a tool for allocating and managing water quantity is now widely accepted and numerous examples of successful implementation exist worldwide. However, the concept of incorporating water quality into water accounting has not yet been widely promoted. Measuring both quantity and quality in the same water bodies is a fundamental principle of assessment of impacts on water quality through the determination of loads. The load is the amount of a given substance or pollutant for a given period of time. Using the key steps necessary for the development of a water quality monitoring and assessment programme, a framework has been developed that can be applied to water accounting projects using typical water accounting applications. Two examples of potential applications are used to consider the technical, institutional, and financial requirements. Implementing a framework for incorporating water quality monitoring and assessment into water accounting should contribute substantially to the need for more water quality data at global scale. Such data are required to facilitate achievement of Sustainable Development Goal 6 “Ensure availability and sustainable management of water and sanitation for all” through more efficient water resources management and greater awareness of water quality impacts in the agricultural water use sector.
1 Introduction
The need for sustainable use of freshwater resources has been highlighted by the United Nations Agenda 2030 (United Nations, 2015) and captured by Sustainable Development Goal (SDG) 6 “Ensure availability and sustainable management of water and sanitation for all.” SDG 6 recognizes that both the quantity and quality of water used, including “use” by the freshwater ecosystem itself, require an integrated approach to management for the benefit of local communities and society in general (United Nations-Water, 2021; United Nations, 2023a). There are many challenges to achieving integrated water resources management, some of which stem from historical institutional arrangements where different agencies are responsible for managing water quantity and water quality. The Organisation for Economic Co-operation and Development (2016) recommends joint management of water quantity and quality at all governmental levels, from local to national. Even where water quality and water quantity monitoring are implemented by government agencies, sharing of data or co-ordination of monitoring activities may not be adequate for effective water resources management to support achievement of SDG 6 (United Nations, 2023a,b). In the last SDG 6 progress update, it was reported that 107 countries worldwide are not on track to have sustainably managed water resources by 2030 (United Nations-Water, 2021). Evidence-based sustainable management of water resources requires sufficient and appropriate data at relevant temporal and spatial scales (Larsen et al., 2016; United Nations-Water, 2021; United Nations, 2023a) and yet, in many world regions, the quality of freshwater is unknown, leading to potential consequences for their socio-economic development (Damania et al., 2019).
Over the last two decades, to aid water resources management, several water accounting methods have been developed that assess and quantify water stocks, uses, and allocations across various scales from agricultural fields and irrigation schemes to river basins. Water accounting has also been applied at national level where, in addition to irrigation, water accounts extend to other sectors such drinking water and industry (Godfrey and Chalmers, 2012; Karimi et al., 2013; Batchelor et al., 2016; Vardon et al., 2023). These frameworks and methods largely acknowledge that water use can lead to water quality degradation and can be a cause for rendering water not useable for some purposes. Nevertheless, they remain short on offering ways to incorporate water quality aspects into water accounting. This leads to a considerable gap in the complete picture of how water use, and reuse, affects the suitability of water for different uses. For example, drainage water, even where it is widely reused for irrigation by farmers, ultimately becomes unsuitable for any use and is flushed to the sea in large quantities, notwithstanding the significant physical water scarcity in some regions.
Agriculture is the greatest user of freshwater on a global scale but is also one of the major sources of water pollution (Mateo-Sagasta et al., 2017). Growing populations and the demand for food to meet those populations mean that the contribution to water pollution from agriculture will continue to increase (Mateo-Sagasta et al., 2017). The need to ensure adequate freshwater for agriculture and food production often takes precedence over ensuring that good freshwater quality is maintained. Some countries focus mainly on managing water quantity, especially in water scarce regions, with poor understanding or appreciation of the causes and consequences of pollution of surface and groundwaters amongst the people responsible for water management and policy. This can be partially attributed to a lack of institutional and technical capacity to generate the necessary information for effective management by relevant water quality monitoring, especially in low-income countries (United Nations-Water, 2015; Kirschke et al., 2020; Chapman and Sullivan, 2022; United Nations, 2023a). Quantifying the contribution of agricultural activities to the state of water quality is essential for the development of appropriate and cost-effective responses to the problem at national and international scale (Mateo-Sagasta et al., 2017).
Water quality varies naturally within a catchment but adverse changes are more pronounced in catchments with a diversity of human activities, such as domestic, industrial, and agricultural activities (Peters et al., 2005). Within a water basin, some of the water that is used is subsequently returned to the hydrological cycle with or without treatment, although not necessarily to the same water body from which it was withdrawn. For example, water abstracted from groundwaters for urban use or irrigation may be returned to a river as sewage discharges or drainage water. Water that is returned almost always has a different, and often degraded, quality compared with the quality of the water that was initially withdrawn, which may have impacts on suitability for use, or even risks to users, downstream. To reduce pressure on available water resources, re-use of wastewater is encouraged to meet the demand for freshwater for human activities, particularly agriculture (Jaramillo and Restrepo, 2017). In some countries the wastewater is used untreated and, due to its high pathogen load, it can present serious health risks for agricultural workers and consumers of the irrigated crops (Kesari et al., 2021).
Water quality monitoring can indicate whether the water meets the required quality for specific uses, such as irrigation, and whether that returned water has the potential to affect the freshwater ecosystem by altering the ambient water quality (Chapman and Sullivan, 2022). Use and return of water to the hydrological cycle forms part of water quantity accounting (Molden et al., 2023; Vardon et al., 2023), but for many water use activities the water quality is only monitored if guidelines or standards require it. Nevertheless, alterations to water quantity and quality have potential implications for other users within the catchment or water basin, and for the freshwater ecosystems within that water basin. Without considering the water basin as a whole, it is difficult for individual activities to evaluate whether their actions may have consequences for other water users (Damania et al., 2019) and their relative contribution to water quality degradation within the catchment.
This paper summarizes the results and recommendations from a project of the Climate Resilient Irrigation Global Solutions Group of the World Bank that explored how incorporating water quality monitoring into water accounting projects could have the potential to improve overall management of ambient freshwaters, particularly in relation to agricultural activities (Chapman et al., 2024). If widely adopted, the recommendations could also increase the availability of water quality data to support sustainable management of water quality at national and international scales.
2 Linking water accounting and water quality monitoring
Water accounting involves collecting information on the quantity of water, measured as the volume. Water quality is determined by making measurements of specific parameters, such as nutrients and contaminants, using grab samples taken from the water body, or with in-situ or remote sensors (Murray et al., 2022; Yang et al., 2022; Adjovu et al., 2023). Measured constituents are reported as concentrations, —the amount of substance per unit volume of water (e.g., mg/L)—at the time at which the sample was taken or, in the case of in-situ or remote sensors, averaged over a selected time interval. Concentrations are used to indicate potential impacts on a water use, the aquatic ecosystem or human health by comparison with standards or guidelines that are stipulated in regulatory frameworks (United Nations-Water, 2015). However, concentrations at any location in a water body are influenced by the amount of water in the water body, which in turn is influenced by rainfall and abstraction. Rainfall run-off and the associated increase in amount of water in a water body can lead to dilution of point source discharges, but it can also lead to the addition of chemicals and substances from the land surface. Therefore, to understand the causes and potential impacts of water quality variations within a water basin, water quantity and water quality measurements should be complimentary activities. Measuring both quantity and quality at the same locations greatly enhances the value of the individual data sets, particularly for the validation of hydrology and water quality models (Harmel et al., 2023).
Water quantity and water quality data from the same monitoring location in the water body can be combined to represent the amount of each substance at that location by calculating loads. Loads are defined as the amount of substance in a given period of time, for example, kilograms per day (kg/day). In rivers, they are calculated by the product of the measured concentration of the water quality parameter and the discharge (Meals et al., 2013). In lakes, the measured concentration is converted to the total lake or reservoir volume for a given period of time and is often referred to as the loading (Thomas et al., 1996). Although measured concentrations may not change from one sampling event to another, the load may increase or decrease because of changes in the amount of water in the lake or flowing in the river. Loads are often used to indicate the amount of a pollutant discharged from a point source, or the amount of substances moving between water bodies or across boundaries. They also enable comparisons between one water body location and another and can be used for source apportionment of contaminants. In the context of agricultural activities, they could give an indication of the relative contribution to water pollution from run-off compared with drainage discharges to a water body (Jarvie et al., 2010; Bowes et al., 2014; Halliday et al., 2015; Rattan et al., 2021). Hence if quantity and quality monitoring stations can be co-located, there is considerable potential for basin-scale management, as demonstrated by Jones et al. (2017). However, the variability in estimates of pollutant loads depends on the accuracy of the measurement of flow and concentrations (Meals et al., 2013). Therefore, wherever possible, the anticipated accuracy of the load calculations should be defined and the intensity of the monitoring (spatial and temporal) established accordingly (Meals et al., 2013).
3 A framework for water quality accounting
Now that accounting for water quantity has been widely accepted and is being implemented in many water allocation projects (Vardon et al., 2023), there is an opportunity to encourage better understanding and management of water quality by incorporating water quality monitoring into water accounting activities. Wherever flow is measured, whether for a small irrigation scheme or over an entire catchment, managers and operators could be encouraged to make basic water quality measurements of key parameters that are important for the aquatic ecosystem and for the designated water use. Given that over 3.7 million km2 of land globally are irrigated (Meier et al., 2018), this would not only provide valuable data for prioritizing and managing sources of impacts on water quality worldwide, but also raise awareness of the impacts of agricultural water use and irrigation. This paper proposes a framework to assist operators with minimal knowledge or understanding of water quality in making appropriate choices of water quality parameters, essential monitoring locations and the frequency for data collection in the context of agricultural water use. More detailed guidance on each of the proposed steps is available in Chapman et al. (2024).
The framework (Figure 1) comprises six steps:
• Decide the scope and scale at which the water quality accounting will be applied. This can range from considering agricultural water use only to placing agricultural use into the wider context of all water use and impact activities within a catchment, such as urban and industrial activities. In the former case the scale would be very local, comprising for example a single irrigation scheme that may use water from a surface and/or groundwater source. In the latter, it might involve a whole catchment or a sub-basin within a catchment. Basin-scale water accounting can be complex and could involve accounting for all water sources (rainfall, surface water and groundwater), as well as returns and losses (discharges, evaporation, run-off, and percolation to groundwater). An example of a water balance in a river basin with numerous irrigation schemes is illustrated for the lower Jordan River Basin in figure 9 of Courcier et al. (2005). Implementing water quality accounting at this scale would require considerable co-operation amongst stakeholders, but it could be implemented as a number of small-scale activities.
• Gather and review existing relevant information. This may involve a desktop and field survey of all potential sources of impacts on water quality within the selected water basin, including agricultural, domestic, and industrial sources, together with the most likely locations of impacts, such as run-off areas and discharge points. Additional information that may be relevant includes existing guidelines or standards for water quality for which data may already be collected.
• Identify water quantity and quality data requirements. This involves (a) selecting the most useful and relevant water quality parameters, (b) identifying the locations from which water quantity and quality will be required, and (c) considering the frequency of measurements. These needs will vary with the scope and scale of the water quality accounting scheme. Two examples are discussed briefly in Section 3.1 below and more detail is available in Chapman et al. (2024). An indication of the most applicable water quality monitoring parameters can be obtained from the list of parameters for which guidelines exist, especially agricultural water use and discharge, and ambient or ecosystem quality guidelines such as Australian and New Zealand Environment and Conservation Council and Agriculture and Resource Management Council of Australia and New Zealand (2000). Ideally samples for water quality analysis should be taken from the same point at which flow measurements are made. Flow measurements are used to determine the volume of water abstracted over a given period of time for agricultural use and are essential for water quantity accounting. To quantify the difference between abstracted and returned water quality, which represents the impacts of the agricultural activity (see step 6), return flows and their quality should also be measured. The frequency of water quality measurements needs to be compatible with the time over which water volumes are measured and calculated. If water use is determined as an annual volume, water quality monitoring must include sampling in each season, but preferably monthly, to enable an annual average value to be calculated for each water quality parameter. Monthly or seasonal sampling should allow for variations due to rainfall and abstraction events. Wherever possible a monitoring location should be selected to represent natural ambient water quality without human influence, or without influence from the activity under consideration. These locations are usually in headwaters, or upstream of the identified study area.
• Obtain water quality data. Depending on the scale and complexity of the identified water quality data needs, data may be available from existing monitoring activities carried out at local or national level. A frequent issue with using existing water quality monitoring data is that the location at which the monitoring is carried out may not be close to a flow monitoring station. If suitable data are not already available, it may be necessary to implement some additional monitoring activities, or even to establish a dedicated monitoring network. There are many sources of detailed information on how to plan and implement water quality monitoring programmes for surface water and groundwater, such as Bartram and Ballance (1996), Chapman (1996), Chapman et al. (2005), Ravenscroft and Lytton (2022), and United Nations Environment Programme (2022, 2023a,b). A review of different monitoring approaches is available in Behmel et al. (2016). Implementing new monitoring activities may require additional field and laboratory equipment and training in new skills for personnel. This has financial implications and requires careful planning and sometimes choices that affect data quality (Harmel et al., 2023).
• Create a data management and manipulation system. Careful consideration needs to be given to all aspects of data management to ensure that they are fit for purpose and will be of value in the future and to other potential data users (Larsen et al., 2016; Wilkinson et al., 2016). Manipulation of the water quality and quantity data involves statistical treatment to ensure they are in appropriate units for the calculation of loads (Meals et al., 2013), or for input to a chosen model (such as Young and Muneer, 2019) or a water quality index (Uddin et al., 2021).
• Interpret and present water quality and quantity accounts. Depending on the complexity of the water accounting project, interpretation could vary from comparing each measured water quality parameter at a water abstraction point with the return flow (calculated as loads where applicable), to comparing loads at multiple locations within a catchment upstream and downstream of different pressures on the water resource, such as urbanization, irrigation, intensive livestock rearing, forestry, etc. The feasibility, and complexity, of accounting for different activities contributing to changes in water quality at catchment scale has been demonstrated by Jones et al. (2017). To be easily understood by non-experts, the results should be presented visually, for example, by showing per cent variation in loads between abstraction and return flows, deviation from natural water quality measured above the agricultural area, or water quality index values at different locations in the catchment. The relative magnitude of different flows can also be included with the water quality as in Figure 2. Where upstream or natural water quality data are not available, monitoring data from locations in the catchment can be compared with the applicable guidelines for ambient or ecosystem water quality (if available).
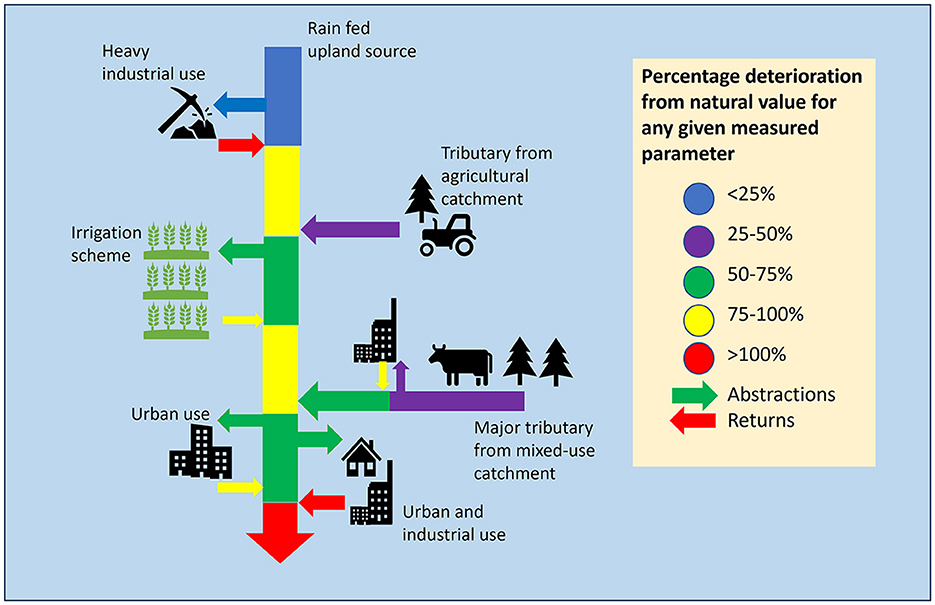
Figure 2. Example of a simple schematic presentation that illustrates water quality accounting in a catchment with diverse activities. Color can be used to indicate relative differences in water quality and the width of the arrows can indicate relative volume of water.
3.1 Examples of potential applications of the water quality accounting framework
An example of a straightforward water accounting project is a small irrigation scheme that abstracts water from a river (Figure 3). The drainage from the irrigation scheme is returned directly to the river downstream, either through collection channels or indirectly through infiltration and run-off. For the purposes of determining volume used (and the associated water charges), and to ensure river levels are maintained for the aquatic ecosystem, the abstraction and return flow volumes are monitored. The quality of the river water is important for the health and growth of the crops and should comply with the relevant guidelines for irrigation (Drechsel et al., 2023). The quality of the river downstream of the return flows (run-off and drainage discharges) demonstrates impacts of its use for irrigation, such as nutrients arising from fertilizer use or the presence of toxic organic chemicals arising from pesticide use. In such a local scenario, it would be unusual to find existing water quality monitoring stations that would provide data for the river upstream and downstream of the irrigation area. Hence, water quality monitoring would need to be introduced at the flow monitoring stations. Guidelines for irrigation water quality use (Drechsel et al., 2023) and for the aquatic ecosystem (United States Environmental Protection Agency, 2022) suggest the necessary water quality parameters for monitoring (Table 1). Most of the basic parameters included in this water quality accounting scenario are relatively cheap and easy to monitor with simple sensors or field kits that require minimal expertise or training, and could be measured monthly. If financial and technical resources allow, monitoring for specific pesticides applied to the crops could also be included in the relevant seasons.
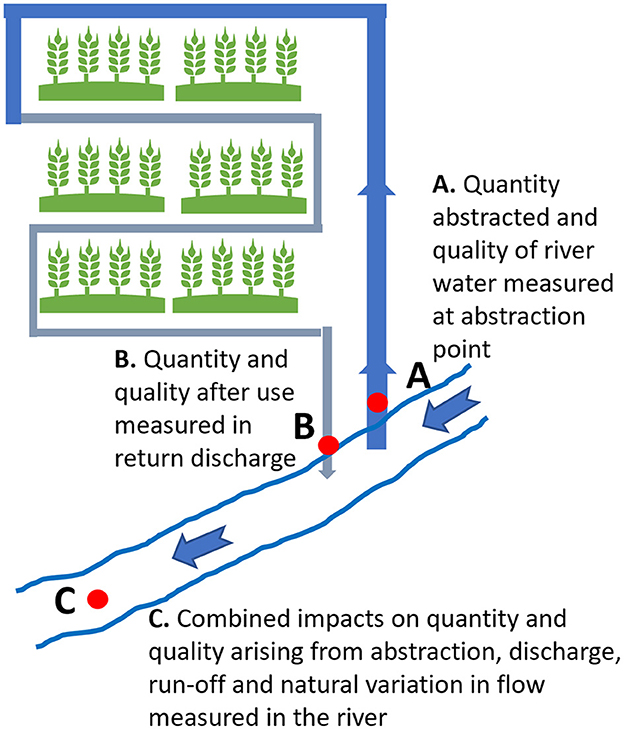
Figure 3. An example of necessary monitoring locations for water quantity and quality accounting in a simple irrigation scheme.
In catchments with multiple water uses, there will be several water abstraction points and return flow discharges. There will also be diffuse run-off and point source discharges potentially adding a very wide range of contaminants to the overall water resource. To account for agricultural use and impacts on water quality, in relation to other uses, monitoring would be required at numerous locations (Figure 4). Implementing a monitoring programme to account for all users and impacts at this scale would require substantial resources (Harmel et al., 2023). However, it is likely that existing compliance monitoring would be carried out by major water users at abstraction and discharge points, although the data may not be freely available. The local water or environment agency may also conduct regular ambient water quality monitoring, such as that recommended for SDG indicator 6.3.2 for ambient water quality (United Nations-Water, 2018). In this scenario, full stakeholder consultation and engagement would be needed to enable data sharing from existing monitoring and to identify where additional monitoring may be required. With diverse activities within the water basin, the range of water quality parameters needed for full accounting could be large, including parameters relevant for ecosystem and human health, including pathogens and toxic compounds. Such complex monitoring needs require specialized analytical facilities and trained personnel; therefore, the objectives and anticipated outcomes of the water quality monitoring need to be carefully defined to ensure monitoring activities will provide the necessary data within financial limitations (Chapman and Sullivan, 2022; Harmel et al., 2023).
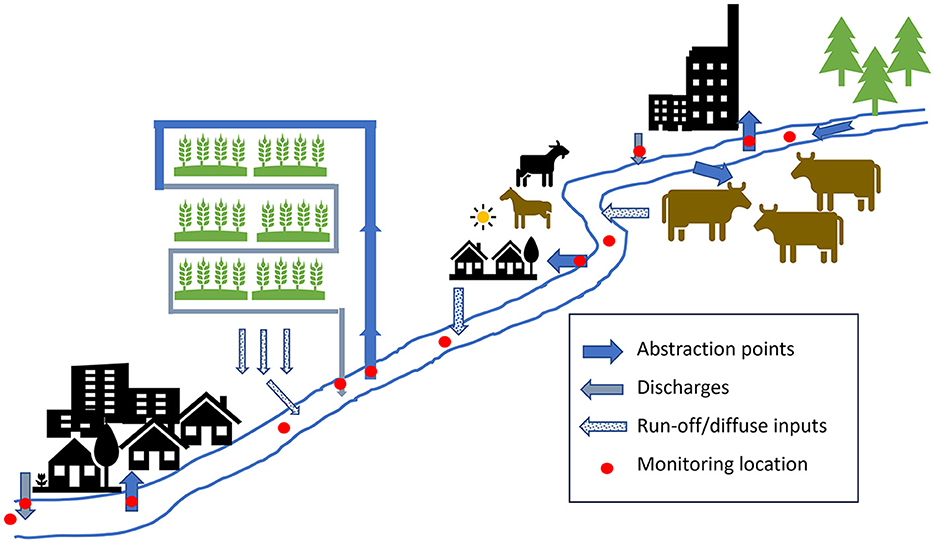
Figure 4. Possible monitoring locations from which to obtain data for water quantity and quality accounting in a catchment with multiple uses, including agriculture.
4 Discussion
The need for more water quality data to support evidence-based sustainable management of water resources at all scales has been brought into focus recently by the United Nations and its assessment of progress toward achieving SDG 6 (United Nations-Water, 2021; United Nations, 2023a). Worldwide there are significant gaps in water quality data resulting in the level and severity of pollution being unrecorded, with associated risks to human health and the ecosystem (United Nations-Water, 2021). In addition, the continued pollution of freshwaters can lead to reduced economic growth by 0.8 to 2.0 per cent (Desbureaux et al., 2019). Although efforts to increase the spatial and temporal scale of water quality data through initiatives, such as satellite remote sensing (Murray et al., 2022; Yang et al., 2022; Adjovu et al., 2023), greater use of technology (Behmel et al., 2016), modeling (Veljkovic et al., 2013; Young and Muneer, 2019) and citizen science (Thornhill et al., 2019; Hegarty et al., 2021; Yevenes et al., 2022) are ongoing, there are other potential mechanisms for increasing water quality data availability that could still be exploited. These include, increasing willingness from public and private organizations to share water quality data and encouraging the simultaneous collection of water quality data with existing or new water quantity monitoring activities, such as highlighted here for agricultural water use. Even the inclusion of very basic water quality parameters, such as those required by SDG Indicator 6.3.2 for ambient water quality (United Nations Environment Programme, 2023c), collected with simple and inexpensive monitoring equipment such as that used by citizen monitoring programmes (Quinlivan et al., 2020a,b) has the potential to greatly increase the spatial and temporal coverage of water quality monitoring and contribute to better water resource management (Capdevila et al., 2020). Such monitoring could be implemented by local irrigation scheme operators and farmers, with the added benefit of creating greater awareness of their relative contribution to water quality deterioration. If implemented by all major agricultural activities within a water basin, water quality accounting has the potential to increase significantly the amount of water quality data available for water resources management, especially if all water users, e.g., municipal, industrial, within the basin also monitor water quality and share their data. A critical aspect of developing any new water quality monitoring activities, at any scale, for water quality accounting is that they are designed to share the water quality data through accessible data platforms that are interoperable (Larsen et al., 2016). However, there can be issues with collecting and combining quantity and quality data at different scales and from different sources, as has been highlighted by Larson et al. (2021). Nevertheless, encouraging monitoring and data management activities to adopt the FAIR principles (findability, accessibility, interoperability, and reusability) (Wilkinson et al., 2016) will help to address some of the issues.
Wherever possible, data gathered for checking compliance with guidelines should be used for water quality accounting to avoid the necessity for additional monitoring activities. Any additional water quality monitoring by agricultural water users will incur technical and financial resources for implementation and is likely to require capacity development (Capdevila et al., 2020; Kirschke et al., 2020). If samples are collected by the project operator and sent to an accredited laboratory for analysis, the costs can be quite minimal, i.e., principally the cost per individual analysis, e.g., UDS25 per analysis (Harmel et al., 2023). Costs increase with the number of sampling events, the number of sampling locations and the number of parameters to be measured. A variety of automated samplers and continuous in situ sensors can be used (Lin et al., 2020) but these incur capital costs as well as maintenance costs (O'Flynn et al., 2010). A detailed breakdown of the capital and recurring costs associated with different options for sampling, analysis and data acquisition is given by Harmel et al. (2023), including the option of collecting data that enables the calculation of loads.
5 Conclusions
Worldwide, a lack of adequate data on water quantity and quality is reducing the efficiency of integrated water resources management, resulting in deterioration of the ambient water sources on which the environment and human society depend. Agriculture is a major user of ambient freshwater but the emphasis, from national to local scales, is on managing quantity despite being a major contributor to water quality deterioration. Water quality accounting can play an integral role in helping interventions and investments in improved water resources management by encouraging the use and implementation of a simple framework for water quality monitoring and the sharing of both quantity and quality data. This will ultimately bring about benefits for both anthropogenic and ecosystems-related use of water. This is at the heart of the SDGs and essential for keeping our planet habitable while pursuing national and global water and food security strategies to meet the demands of the growing population.
Author contributions
PK: Conceptualization, Writing – original draft. DC: Conceptualization, Writing – original draft. SV: Writing – review & editing. RL: Writing – review & editing. AT: Writing – review & editing.
Funding
The author(s) declare that no financial support was received for the research, authorship, and/or publication of this article.
Conflict of interest
The authors declare that the research was conducted in the absence of any commercial or financial relationships that could be construed as a potential conflict of interest.
Publisher's note
All claims expressed in this article are solely those of the authors and do not necessarily represent those of their affiliated organizations, or those of the publisher, the editors and the reviewers. Any product that may be evaluated in this article, or claim that may be made by its manufacturer, is not guaranteed or endorsed by the publisher.
Author disclaimer
This work is a product of the staff of The World Bank with external contributions. The findings, interpretations, and conclusions expressed in this work do not necessarily reflect the views of The World Bank, its Board of Executive Directors, or the governments they represent. The World Bank does not guarantee the accuracy, completeness, or currency of the data included in this work and does not assume responsibility for any errors, omissions, or discrepancies in the information, or liability with respect to the use of or failure to use the information, methods, processes, or conclusions set forth. The boundaries, colors, denominations, and other information shown on any map in this work do not imply any judgment on the part of The World Bank concerning the legal status of any territory or the endorsement or acceptance of such boundaries.
References
Adjovu, G. E., Stephen, H., James, D., and Ahmad, S. (2023). Overview of the application of remote sensing in effective monitoring of water quality parameters. Remote Sens. 15:1938. doi: 10.3390/rs15071938
Australian and New Zealand Environment and Conservation Council and Agriculture and Resource Management Council of Australia and New Zealand (2000). Australian and New Zealand Guidelines for Fresh and Marine Water Quality. Available online at: https://www.waterquality.gov.au/sites/default/files/documents/anzecc-armcanz-2000-guidelines-vol1.pdf (accessed August 08, 2023).
Bartram, J., and Ballance, R., (eds) (1996). Water Quality Monitoring: A Practical Guide to the Design and Implementation of Freshwater Quality Studies and Monitoring Programmes. London: E and FN Spon on behalf of United Nations Environment Programme and the World Health Organization. Available online at: https://apps.who.int/iris/handle/10665/41851 (accessed March 25, 2024).
Batchelor, C., Hoogeveen, J., Faurès, J. M., and Peiser, L. (2016). Water Accounting and Auditing Guidelines: A Sourcebook, Vol. 43. Rome: Food and Agriculture Organization.
Behmel, S., Damour, M., Ludwig, R., and Rodriguez, M. J. (2016). Water quality monitoring strategies — a review and future perspectives. Sci. Total Environ. 571, 1312–1329. doi: 10.1016/j.scitotenv.2016.06.235
Bowes, M. J., Jarvie, H. P., Naden, P. S., Old, G. H., Scarlett, P. M., Roberts, C., et al. (2014). Identifying priorities for nutrient mitigation using river concentration–flow relationships: the Thames basin, UK. J. Hydrol. 517, 1–12. doi: 10.1016/j.jhydrol.2014.03.063
Capdevila, A. S. L., Kokimova, A., Ray, S. S., Avellán, T., Kim, J., Kirschke, S., et al. (2020). Success factors for citizen science projects in water quality monitoring. Sci. Total Environ. 728:137843. doi: 10.1016/j.scitotenv.2020.137843
Chapman, D. V., (ed.). (1996). Water Quality Assessments: A Guide to Use of Biota, Sediments and Water in Environmental Monitoring, 2nd ed. London: E and FN Spon. Available online at: https://apps.who.int/iris/handle/10665/41850 (accessed March 25, 2024).
Chapman, D. V., Karimi, P., Valieva, S., and Li, R. (2024). Accounting for Water Quality: Insights for Operational Task Teams. Washington DC: World Bank Group, In Press
Chapman, D. V., Meybeck, M., and Peters, N. E. (2005). “Water quality monitoring” in Encyclopedia of Hydrological Sciences, ed. M. G. Anderson (Chichester: Wiley and Sons), 1387–1408. doi: 10.1002/0470848944.hsa094
Chapman, D. V., and Sullivan, T. (2022). The role of water quality monitoring in the sustainable use of ambient waters. One Earth 5, 132–137. doi: 10.1016/j.oneear.2022.01.008
Courcier, R., Venot, J.-P., and Molle, F. (2005). Historical Transformations of the Lower Jordan River Basin in Jordan: Changes in Water Use and Projections (1950-2025). Comprehensive Assessment of Water Management in Agriculture Research Report 009. Colombo: International Water Management Institute (IWMI).
Damania, R., Desbureaux, S., Rodella, A.-S., Russ, J., and Zaveri, E. (2019). Quality Unknown: The Invisible Water Crisis. Washington, DC: World Bank. Available online at: http://hdl.handle.net/10986/32245 (accessed March 25, 2024).
Desbureaux, S. G., Damania, R., Rodella, A.-S., Russ, J., Daniel, Z., Esha, D., et al. (2019). The Impact of Water Quality on GDP Growth: Evidence from Around the World (English). Washington, DC: World Bank Group. Available online at: http://documents.worldbank.org/curated/en/656191576065317601/The-Impact-of-Water-Quality-on-GDP-Growth-Evidence-from-Around-the-World (accessed March 25, 2024).
Drechsel, P., Marjani Zadeh, S., and Pedrero, F., (eds) (2023). Water Quality in Agriculture: Risks and Risk Mitigation. Rome: Food and Agriculture Organization and International Water Management Institute. doi: 10.4060/cc7340en
Godfrey, J. M., and Chalmers, K., (eds). (2012). Water Accounting: International Approaches to Policy and Decision-Making. Cheltenham: Edward Elgar Publishing. doi: 10.4337/9781849807500
Halliday, S. J., Skeffington, R. A., Wade, A. J., Bowes, M. J., Gozzard, E., Newman, J. R., et al. (2015). High-frequency water quality monitoring in an urban catchment: hydrochemical dynamics, primary production and implications for the Water Framework Directive. Hydrol. Process. 29, 3388–3407. doi: 10.1002/hyp.10453
Harmel, R. D., Preisendanz, H. E., King, K. W., Busch, D., Birgand, F., and Sahoo, D. A. (2023). Review of data quality and cost considerations for water quality monitoring at the field scale and in small watersheds. Water 15:3110. doi: 10.3390/w15173110
Hegarty, S., Hayes, A., Regan, F., Bishop, I., and Clinton, R. (2021). Using citizen science to understand river water quality while filling data gaps to meet United Nations Sustainable Development Goal 6 objectives. Sci. Total Environ. 783:146953 doi: 10.1016/j.scitotenv.2021.146953
Jaramillo, M. F., and Restrepo, I. (2017). Wastewater reuse in agriculture: a review about its limitations and benefits. Sustainability 9:1734. doi: 10.3390/su9101734
Jarvie, H. P., Withers, P. J. A., Bowes, M. J., Palmer-Felgate, E. J., Harper, D. M., Wasiak, K., et al. (2010). Streamwater phosphorus and nitrogen across a gradient in rural–agricultural land use intensity. Agric. Ecosyst. Environ. 135, 238–252. doi: 10.1016/j.agee.2009.10.002
Jones, A. S., Aanderud, Z. T., Horsburgh, J. S., Eiriksson, D. P., Dastrup, D., Cox, C., et al. (2017). Designing and implementing a network for sensing water quality and hydrology across mountain to urban transitions. J. Am. Water Resour. Assoc. 53, 1095–1120. doi: 10.1111/1752-1688.12557
Karimi, P., Bastiaanssen, W. G., and Molden, D. (2013). Water Accounting Plus (WA+)–a water accounting procedure for complex river basins based on satellite measurements. Hydrol. Earth Syst. Sci. 17, 2459–2472. doi: 10.5194/hess-17-2459-2013
Kesari, K. K., Soni, S., Jamal, Q. M., Tripathi, P., Lal, J. A., Jha, N. K., et al. (2021). Wastewater treatment and reuse: a review of its applications and health implications. Water Air Soil Pollut. 232:208. doi: 10.1007/s11270-021-05154-8
Kirschke, S., Avellán, T., Bärlund, I., Bogardi, J. J., Carvalho, L., Chapman, D., et al. (2020). Capacity challenges in water quality monitoring: understanding the role of human development. Environ. Monit. Assess. 192:298. doi: 10.1007/s10661-020-8224-3
Larsen, S., Hamilton, S., Lucido, J., Garner, B., and Young, D. (2016). Supporting Diverse Data Providers in the Open Water Data Initiative: Communicating Water Data Quality and Fitness of Use. J Am Water Resour Assoc 52, 859–872. doi: 10.1111/1752-1688.12406
Larson, K. B., Wong, M. A. Y. L., Mitchel, K. C., Tagestad, J. D., Saulsbury, J. W., Bellgraph, B. J., et al. (2021). Improving Discovery, Sharing, and Use of Water Data: Initial Findings and Suggested Future Work. Richland, WA: Pacific Northwest National Laboratory. Available online at: https://www.pnnl.gov/main/publications/external/technical_reports/PNNL-29737.pdf
Lin, Y. P., Mukhtar, H., Huang, K. T., Petway, J. R., Lin, C. M., Chou, C. F., et al. (2020). Real-time identification of irrigation water pollution sources and pathways with a wireless sensor network and blockchain framework. Sensors 20:3634. doi: 10.3390/s20133634
Mateo-Sagasta, J., Marjani Zadeh, S., and Turral, H. (2017). Water Pollution from Agriculture: A Global Review. Executive Summary. Rome: Food and Agriculture Organization of the United Nations Rome (FAO) and the International Water Management Institute (IWMI) on behalf of the Water Land and Ecosystems research program Colombo. Available online at: https://www.fao.org/3/i7754e/i7754e.pdf (accessed March 25, 2024).
Meals, D. W., Richards, R. P., and Dressing, S. A. (2013). Pollutant Load Estimation for Water Quality Monitoring Projects. Tech Notes 8, April 2013. Developed for U.S. Environmental Protection Agency by Tetra Tech, Inc. Fairfax, VA, 21. Available online at https://www.epa.gov/sites/default/files/2016-05/documents/tech_notes_8_dec_2013_load.pdf (accessed March 25, 2024).
Meier, J., Zabel, F., and Mauser, W. (2018). A global approach to estimate irrigated areas – a comparison between different data and statistics. Hydrol. Earth Syst. Sci. 22, 1119–1133. doi: 10.5194/hess-22-1119-2018
Molden, D., Karimi, P., Cestti, R., Valieva, S., and Li, R. (2023). Why and When Water Accounting Matters: A Guidance Note for Operational Task Teams. Washington DC: World Bank Group.
Murray, C., Larson, A., Goodwill, J., Wang, Y., Cardace, D., Akanda, A. S., et al. (2022). Water quality observations from space: a review of critical issues and challenges. Environments 9:125. doi: 10.3390/environments9100125
O'Flynn, B., Regan, F., Lawlor, A., Wallace, J., Torres, J., O'Mathuna, C., et al. (2010). Experiences and recommendations in deploying a real-time, water quality monitoring system. Meas. Sci. Technol. 21:124004. doi: 10.1088/0957-0233/21/12/124004
Organisation for Economic Co-operation and Development (2016). OECD Council Recommendation on Water. Paris. Available online at: https://www.oecd.org/environment/resources/Council-Recommendation-on-water.pdf (accessed March 25, 2024).
Peters, N. E., Meybeck, M., and Chapman, D. V. (2005). “Effects of human activities on water quality,” in Encyclopedia of Hydrological Sciences, ed M. G. Anderson (Chichester: Wiley and Sons), 1409–1426. doi: 10.1002/0470848944.hsa096
Quinlivan, L., Chapman, D., and Sullivan, T. (2020a). Validating citizen science monitoring of ambient water quality monitoring for the United Nations sustainable development goals. Sci. Total Environ. 699:134255. doi: 10.1016/j.scitotenv.2019.134255
Quinlivan, L., Chapman, D. V., and Sullivan, T. (2020b). Applying citizen science to monitor for the Sustainable Development Goal Indicator 6.3.2: a review. Environ. Monit. Assess. 192:218. doi: 10.1007/s10661-020-8193-6
Rattan, K. J., Bowes, M. J., Yates, A. G., Culp, J. M., and Chambers, P. A. (2021). Evaluating diffuse and point source phosphorus inputs to streams in a cold climate region using a load apportionment model. J. Great Lakes Res. 47, 761–772. doi: 10.1016/j.jglr.2020.05.003
Ravenscroft, P., and Lytton, L. (2022). Practical Manual on Groundwater Quality Monitoring. Washington, DC: World Bank. doi: 10.1596/37196
Thomas, R., Meybeck, M., and Beim, A. (1996). “Chapter 7 Lakes” in Water Quality Assessments. A Guide to the Use of Biota, Sediments and Water in Environmental Monitoring, 2nd ed., ed. D. Chapman (London: E and FN Spon). Available online at: https://apps.who.int/iris/handle/10665/41850 (accessed March 25, 2024).
Thornhill, I., Loiselle, S., Clymans, W., and van Noordwijk, C. G. E. (2019). How citizen scientists can enrich freshwater science as contributors, collaborators, and co-creators. Freshw. Sci. 38, 231–235. doi: 10.1086/703378
Uddin, M. G., Nash, S., and Olbert, A. I. (2021). A review of water quality index models and their use for assessing surface water quality. Ecol. Indic. 122:107218. doi: 10.1016/j.ecolind.2020.107218
United Nations (2015). Transforming Our World: The 2030 Agenda for Sustainable Development. Resolution adopted by the General Assembly on 25 September 2015. A/RES/70/1. New York, NY. Available online at: https://sdgs.un.org/2030agenda (accessed March 25, 2024).
United Nations (2023a). The United Nations World Water Development Report 2023: Partnerships and Cooperation for Water. Paris: United Nations Educational Scientific and Cultural Organization (UNESCO). Available online at: https://www.unesco.org/reports/wwdr/2023/en/download (accessed March 25, 2024).
United Nations (2023b). Blueprint for Acceleration: Sustainable Development Goal 6 Synthesis Report on Water and Sanitation. New York, NY. Available online at: https://www.unwater.org/sites/default/files/2023-08/UN-Water_SDG6_SynthesisReport_2023.pdf (accessed March 25, 2024).
United Nations Environment Programme (2022). Water Quality Monitoring and Assessment of Groundwater – Technical Guidance Document. Nairobi. Available online at: https://wedocs.unep.org/handle/20.500.11822/40414 (accessed March 25, 2024).
United Nations Environment Programme (2023a). Quality Assurance for Freshwater Quality Monitoring – Technical Guidance Document. Nairobi. Available online at: https://wedocs.unep.org/handle/20.500.11822/42666 (accessed March 25, 2024).
United Nations Environment Programme (2023b). Water Quality Monitoring and Assessment in Rivers, Lakes and Reservoirs – Technical Guidance Document. Nairobi. Available online at: https://wedocs.unep.org/handle/20.500.11822/43007 (accessed March 25, 2024).
United Nations Environment Programme (2023c). An Introduction to SDG Indicator 6.3.2: Proportion of Bodies of Water with Good Ambient Water Quality. Nairobi. Available online at: https://communities.unep.org/display/sdg632/Documents+and+Materials (accessed November 10, 2023).
United Nations-Water (2015). Compendium of Water Quality Regulatory Frameworks: Which Water for Which Use? Geneva. Available online at: https://www.unwater.org/sites/default/files/app/uploads/2017/05/Compendium-of-Water-Quality-Main-Report_4.pdf (accessed August 08, 2023).
United Nations-Water (2018). Step-by-Step Methodology for Monitoring Water Quality (6.3.2). Available online at: https://www.unwater.org/publications/step-step-methodology-monitoring-water-quality-632 (accessed November 16, 2018).
United Nations-Water (2021). Summary Progress Update 2021 – SDG 6 – Water and Sanitation for All. Version: July 2021. Geneva. Available online at: https://www.unwater.org/publications/summary-progress-update-2021-sdg-6-water-and-sanitation-all (accessed March 25, 2024).
United States Environmental Protection Agency (2022). National Recommended Water Quality Criteria - Aquatic Life Criteria Table. Available online at: https://www.epa.gov/wqc/national-recommended-water-quality-criteria-aquatic-life-criteria-table (accessed August 08, 2023).
Vardon, M. J., Ha, T., Le, L., Martinez-Lagunes, R., Pule, O. B., Schenau, S., et al. (2023). Water Accounts and Water Accounting. Paris: Global Commission on the Economics of Water.
Veljkovic, N., Dopudja-Glisic, T., and Jovicic, M. (2013). Modeling and Assessment of Diffuse Water Pollution Load Principles and application. London: Lambert Academic Publishing.
Wilkinson, M., Dumontier, M., Aalbersberg, I., Appleton, G., Axton, M., Baak, A., et al. (2016). The FAIR guiding principles for scientific data management and stewardship. Sci. Data 3:160018. doi: 10.1038/sdata.2016.18
Yang, H., Kong, J., Hu, H., Du, Y., Gao, M., Chen, F., et al. (2022). A review of remote sensing for water quality retrieval: progress and challenges. Remote Sens. 14:1770. doi: 10.3390/rs14081770
Yevenes, M. A., Pereira, H., and Bermudez, R. (2022). Citizen science as a co-creative measure to water quality: chemical data and local participation in a rural territory. Front. Environ. Sci. 10:940778. doi: 10.3389/fenvs.2022.940778
Young, D. F., and Muneer, A. (2019). Point Source Calculator: A Model for Estimating Chemical Concentration in Water Bodies. Washington, DC: Office of Chemical Safety and Pollution Prevention, U.S. Environmental Protection Agency. Available online at: https://www.epa.gov/sites/default/files/2019-06/documents/point_source_calculator_13_june_2019.ver_.2_clean.pdf (accessed March 25, 2024).
Keywords: water accounting, irrigation, IWRM, monitoring, water basin, water quality
Citation: Karimi P, Chapman DV, Valieva S, Li R and Talbi A (2024) Accounting for water quality—A framework for agricultural water use. Front. Water 6:1375791. doi: 10.3389/frwa.2024.1375791
Received: 24 January 2024; Accepted: 19 March 2024;
Published: 11 April 2024.
Edited by:
Wen-Cheng Liu, National United University, TaiwanReviewed by:
Ahmed S. Elshall, Florida Gulf Coast University, United StatesIvan Portoghese, Consiglio Nazionale delle Ricerche, Italy
Anja Du Plessis, University of South Africa, South Africa
Copyright © 2024 Karimi, Chapman, Valieva, Li and Talbi. This is an open-access article distributed under the terms of the Creative Commons Attribution License (CC BY). The use, distribution or reproduction in other forums is permitted, provided the original author(s) and the copyright owner(s) are credited and that the original publication in this journal is cited, in accordance with accepted academic practice. No use, distribution or reproduction is permitted which does not comply with these terms.
*Correspondence: Deborah V. Chapman, d.chapman@ucc.ie
†These authors have contributed equally to this work and share first authorship