Erratum: The Three-Points Sponge Policy approach; toward an enhanced multi-level resilience strategy
- 1Deltares, Delft, Netherlands
- 2Delft University of Technology, Delft, Netherlands
- 3IHE Delft Institute for Water Education, Delft, Netherlands
- 4China Academy of Urban Planning and Design, Beijing, China
Urban water management is confronted with more frequent, more extreme weather events. This paper introduces the Three-Points Approach (3PA) into Sponge City concept, to create a novel framework aimed at guiding water management interventions across local, urban, regional, and river basin scales. The 3PA integrates three domains—Day-to-day, Design, and Extreme —offering a nuanced strategy for flood and drought risk mitigation, also for extreme events. Notably, the 3PA emphasizes the fusion of blue-green infrastructure (BGI) with conventional gray approaches to enhance both multifunctionality and resilience in extreme weather conditions. The study identifies critical issues of implementing this Three-Points Sponge Policy (3PSP) approach, including spatial and temporal scales, transitioning from gray to blue-green infrastructure, asset management, data handling, and effective communication. Interventions spanning various spatial scales and addressing flood protection, drought resilience, and water quality are explored in a first and indicative application in Zhengzhou. The case study distills key design principles, highlighting the imperative of never shifting problems, embracing ecosystem-based adaptation, seeking synergy between interventions, and incorporating adaptability into designs. The 3PSP approach emerges as a holistic framework that considers both risks and benefits, contributing valuable insights to the discourse on integrating urban and river basin water management, improving our ways of dealing with extreme weather events while maximizing the day-to-day benefits of our interventions.
1 Introduction
Both the Netherlands and China suffer from extreme weather events. Cloudbursts cause destructive waterlogging, periods of extreme rainfall cause pluvial, fluvial and groundwater flooding (Handmer et al., 2012; O’Donnell and Thorne, 2020) as well as water quality issues, while periods of drought cause water shortages, depletion of water resources, water quality issues and economic and ecological losses (Liu et al., 2018; Machairas et al., 2022; Montanari et al., 2023). Since 2008, an average of more than 20 million people has been internally displaced each year by extreme weather-related events, with storms and floods being the most common (IPCC, 2022). In Europe, floods are the most common and costly natural disaster (World Health Organization, 2002). Managing flood risks is one of the biggest challenges cities are currently facing, not only due to their density of people and valuable assets but also because flooding is likely to increase substantially due to climate change (Tkachenko et al., 2016). In the summer of 2021, many villages and cities in Europe were also hit by an unprecedented extreme rainstorm (Tradowsky et al., 2023). In China, most of the large and rapidly expanding cities are at risk of pluvial flooding, as demonstrated by the catastrophic events which recently hit Beijing region (2023), Ningbo (2013), Wuhan (2016), Shenzhen (2019), Guangzhou (2020) and Zhengzhou (2021), all of them resulted in serious economic impacts and casualties (Chan et al., 2021).
Extreme droughts are equally destructive to society, economy, and nature, the more because they trigger water quality deterioration, land subsidence, foundation problems and so on (Hoogvliet et al., 2012). Water shortages, amongst others, reduce agricultural and industrial production, threaten public water supply and public health, and have catastrophic consequences for biodiversity. Our concern about flooding has led us to implement ‘enhanced’ drainage systems with faster discharge rates to prevent waterlogging, inadvertently elevating the risk of drought.
In various studies, the Three-Points Approach (3PA), first developed by Geldof and Kluck (2008) and elaborately described by Fratini et al. (2012), has received significant attention as a comprehensive framework for enhancing the resilience of stormwater systems (Fratini et al., 2012). For instance, under the umbrella of the Chinese European Water Partnership (CEWP), a recently launched Policy Brief has advocated for the integration of this approach alongside the Sponge City methodology in the planning and design of urban water infrastructure (CEWP, 2022). This combination allows for the accommodation of diverse rainfall intensities, thereby enhancing the adaptability and effectiveness of stormwater management strategies. The approach also involves integrating blue-green infrastructure with conventional gray infrastructure, resulting in what is referred to as green-gray infrastructure (GGI).
The objective of this paper is to review the Chinese Sponge City approach to integrated urban water management (IUWM), including flood and drought, to discuss potential improvements by integrating the Three-Points approach, and to explore its consequences for spatial planning, data collection, engineering resilience and land and water governance. Furthermore, it will address the challenge of achieving systemic integration between hydrological engineering and urban design at the scale of the city and its related river basin. A first start was made with applying this new approach in a case study in Zhengzhou and the Huai River / Yellow River basin (Avellar Montezuma, 2023; Ding, 2023). This process led to the identification of key design principles and provided an initial overview of potential interventions essential for developing a robust resiliency plan.
2 Different approaches to (urban) water management
Integrated approaches to urban water management have been in existence for several decades, but their popularity has surged since the early 1990s. Integrated urban water management (IUWM) aims to effectively manage various sub-systems of the whole urban water cycle, including stormwater, wastewater, water supply, groundwater, water quantity and water quality (Fletcher et al., 2015). In Japan, IUWM originated from efforts to address peak runoffs using infiltration technologies (Fujita, 1997). In the United States, the focus was largely on managing stormwater quality (Feng et al., 2022), while in the Netherlands and other Western European countries, the emphasis was on limiting stormwater peaks entering combined-sewer systems to avoid polluting combined sewer overflows and street flooding. For example, the city of Copenhagen is retrofitting part of its road system to convey floods to parks and canals (City of Copenhagen, 2012) after a cloudburst in 2011. And the city of Amsterdam is rolling out its Amsterdam Rainproof program (Amsterdam Rainproof, 2021), including numerous large- and small-scale interventions in the streetscape to this end. Consequently, the spatial consequences of the interventions and their effect beyond the urban boundaries became part of IUWM. Similarly, the emergence of Sponge City Concept (SCC) in China was a response to the increasing flood damages caused by rainstorms (MoHURD, 2014).
2.1 Different ‘western’ approaches to IUWM
IUWM forms the basis for Water Sensitive Urban Design (WSUD), which aims to transform the impact of urban development on the natural water cycle. By managing the entire urban water cycle, WSUD seeks to enhance resource efficiency, generate economic benefits, and improve social and environmental outcomes (Fletcher et al., 2015). WSUD integrates “water sensitivity” and “urban design,” where “water sensitivity” entails recognizing water as a finite and vulnerable resource critical to livability, influencing behaviors of individuals, organizations, and governmental bodies. “Urban design” involves shaping neighborhoods, districts, and cities to create functional, attractive, and sustainable urban areas through planning and architectural design. WSUD ideally leads to the development of Water Sensitive Cities (WSC), as the ultimate goal (Wong, 2007). According to the Australian National Water Initiative, WSUD is defined as the integration of urban planning with the management, protection, and conservation of the urban water cycle, ensuring alignment with natural hydrological and ecological processes (Commonwealth of Australia [COAG], 2004). Therefore, WSUD and SCC promote water sustainability and environmental protection, closely tied to urban design, with a focus on optimizing opportunities within the water cycle.
Sustainable Urban Drainage Systems (SUDS) are technologies designed to counteract the excessive runoff resulting from urbanization by encouraging infiltration, detention, retention, and to treat stormwater pollution as close to the source as possible. In the UK, they are referred to as SuDS (Sustainable Drainage Systems), while in the USA, they are commonly known as BMPs (Best Management Practices) (CIRIA, 2007). Although SUDS primarily focus on stormwater as a component of the urban water cycle, the ideal approach is multifunctional. SUDS rely on natural processes like evapotranspiration, infiltration, and natural treatment, often complementing traditional “gray” infrastructure. Hence, they are also referred to as Nature-Based Solutions (NBS) or as green or blue-green infrastructure (BGI) – we prefer the latter term. Effective implementation of combinations of SUDS is in the USA referred to as Low Impact Development (LID) (Fletcher et al., 2015).
Green or blue-green infrastructure (BGI) entails a planned approach to incorporating nature and water into urban areas, to provide benefits to residents. BGI utilizes natural or semi-natural systems to manage water resources effectively while delivering critical ecosystem services such as pollution filtration, water treatment, flood risk reduction, and mitigation of high urban temperatures. Maximizing the benefits and value of BGI investments is an area of increasing attention within the scientific community, project development and the finance sector. It is becoming more evident that the broader value of BGI should be considered when addressing any aspect of water systems (Browder et al., 2019; European Commission, 2020; Water Europe, 2021). Like gray water infrastructure, BGI is designed to handle storm events up to the design storm (s). Storms exceeding this will make the system fail and damage will occur. Smart, hybrid combinations of these blue-green solutions with gray solution, the so-called green-gray infrastructure (GGI) will be more resilient, reliable and helps minimizing damage of extreme weather conditions. Examples of GGI are already available; developing more is an innovation challenge (Water Europe, 2021).
The International Water Association introduced the Principles for Water Wise Cities in 2016, aiming to promote sustainable and resilient urban water management. These principles serve as a framework for urban leaders and professionals to develop and implement their vision for sustainable urban water systems and resilient planning and design. By embracing these principles, urban leaders, water managers, and stakeholders are encouraged to collaboratively address water management challenges and implement water-wise strategies, fostering the creation of flexible and adaptable cities.
2.2 Sponge City approach
In line with these developments, China launched its Sponge City approach and program. As illustrated by Figure 1, this development builds on earlier steps in urban drainage research and practice in China.
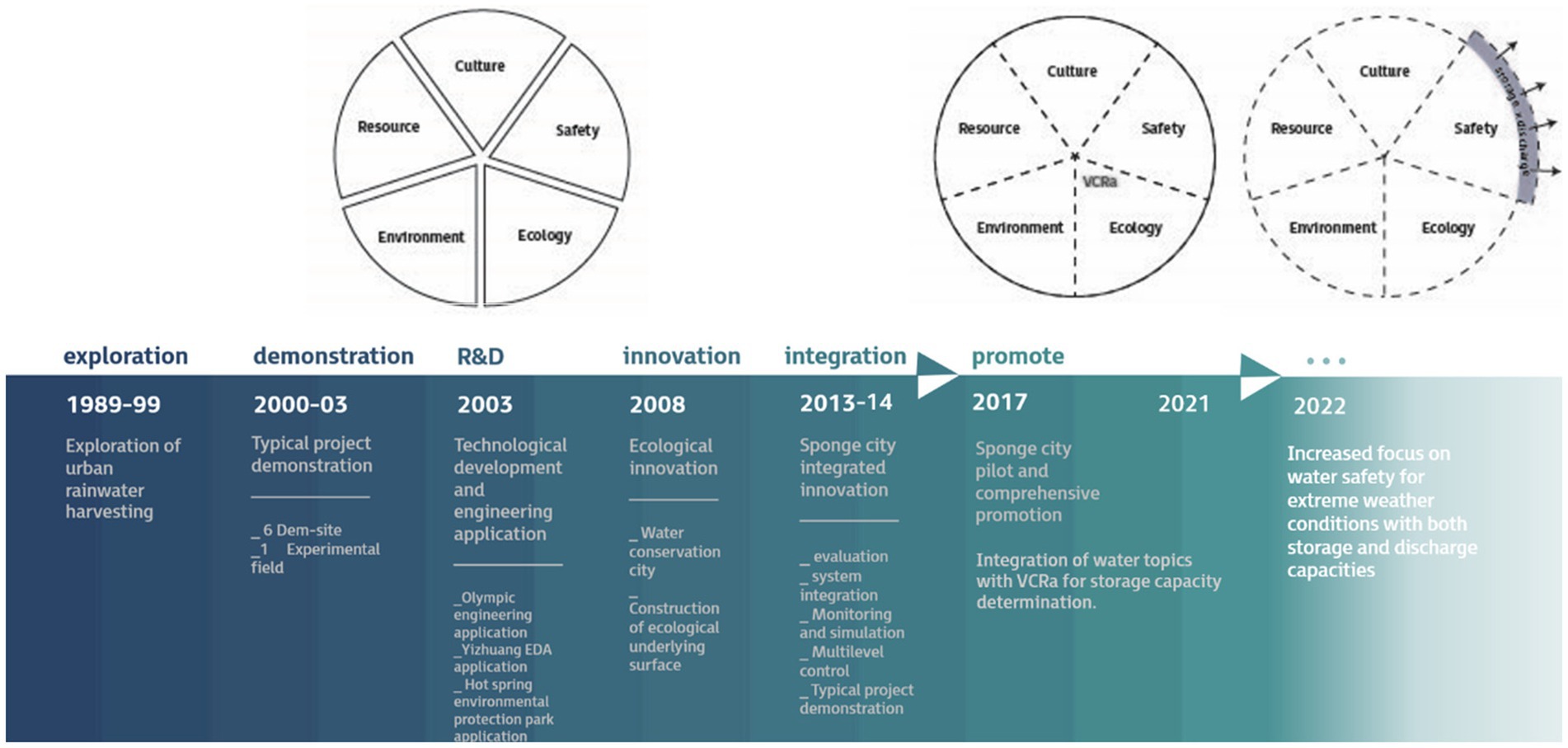
Figure 1. Sponge city research and practice, a result of earlier research and innovation. Important changes in the guidelines occurred in 2017 and 2022 (Chen et al., 2021; Avellar Montezuma, 2023).
After the first official debut at the Central Urbanization Conference in 2013, the Sponge City concept was formulated and in 2014 the first technical guideline was published, outlining the technical framework of Sponge City construction (MoHURD, 2014). Performance indicators on six aspects were formulated, i.e., water ecology, water environment, water resources, water safety, institutional system building & implementation, demonstration effects (MoHURD, 2015). The call for local Sponge City project proposals sets general principles of downscaling Sponge City indicators to city level and more sectoral integration (Ministry of Finance, The Ministry of Housing and Urban-Rural Development, The Ministry of Water Resources, 2016). In 2018 the official national standard was released for evaluating sponge effects (MoHURD, 2018). Since 2022 more attention is paid to flood safety and waterlogging (MoHURD, 2022).
Driven by the central government’s policy, the Sponge City concept and the planning approach cascaded down to local levels, with more detailed local interpretations and planning procedures. Stormwater is to be managed by at-source measures, en route conveyance, and end-of-pipe measures. Water retention is required as part of the ecological indicators. The capacity to retain water of this ‘sponge’ is determined by an indicator called VCRa (Volume Capture Ratio of annual rainfall). For areas with significant water-related historical or social values, water culture is also considered in their sponge planning (Chen et al., 2021). For example, in Nanjing the Sponge City approach covers the topics flood safety, water ecology, water environment, water resources and culture.
Throughout the years, the focus of the Sponge City Program has evolved, expanding beyond the enhancement of water environments to encompass the critical aspects of water security and pluvial flood protection. The task of “focusing on solving the problem of urban flood control” was added for the first time in Notice on launching the Second Batch of Comprehensive Sponge City Construction Demonstration Program across the Entire Region under “14th Five-Year Plan” (Ministry of Finance, The Ministry of Housing and Urban-Rural Development, The Ministry of Water Resources, 2022) in 2022, compared to the previous rounds of Sponge City selections (Ministry of Finance, The Ministry of Housing and Urban-Rural Development, The Ministry of Water Resources, 2014, 2016, 2021). The catastrophic floods of Zhengzhou on July 20, 2021, a pilot city of Sponge City Program, played a crucial role in propelling a switch of focus in Sponge City construction. The Investigation report on this so-called “720” Cloudburst in Zhengzhou, Henan (State Council, 2022) uncovered that approximately 56% of Sponge City investments were used in landscaping and greenery without sponge function, which raised a wide discussion and reflection on Sponge City’s tasks.
Recently, the approach of stormwater management has evolved beyond a focus on discharge capacity alone. Instead, there’s a growing emphasis on the importance of combining storage and discharge capabilities. This shift toward a dual strategy of both storage and discharge is highlighted in Xinhua (2021). By 2025, the central government of China has mandated that each city must establish an urban drainage and pluvial flood prevention engineering system that adheres to the principles of “on-source reduction, pipe network discharge, a balanced combination of storage and discharge, and emergency response to excess storm runoffs.” These systems should be designed in accordance with local conditions in each city (State Council, 2021). Technical standards and national research & development projects are aligned with this new policy. For example, the Code for Urban and Rural Drainage Engineering Projects requires the layout of urban stormwater systems should adhere to the principle of “combination of green, blue and gray” and “combination of storage and discharge” (MoHURD, 2022); Research and Application of Key Techniques for Urban Pluvial Flood Risk Prevention and System Control includes the study on synergistic pluvial flood prevention methods of storage and discharge balance of blue-green space and construction land (Ministry of Science and Technology, 2021).
2.3 The three-points approach to (urban) water management
The Three-Points Approach (3PA) has been developed with the ultimate goal to identify and select interventions which jointly minimize the risks of floods and droughts and at the same time provide multiple functions to maximize the benefits and co-benefits of these measures. Hence, this framework serves as a guidance to facilitate integrative planning and design for water management interventions (Geldof and Kluck, 2008; Fratini et al., 2012). The 3PA (see Figure 2) divides storm events in three groups. The first group comprises significant rain events, which are taken into account when designing the service level of the drainage system. These occurrences are less frequent, typically happening once every 10 years. They encompass a range of storms, varying in duration, such as short-lived bursts and longer-lasting downpours—commonly referred to as 2-h and 24-h design storms. The second group consists of extreme storms that surpass the magnitude of these design storms. Given their magnitude exceeding the design criteria of the drainage system, instances of waterlogging, flooding, and associated damages are likely. The third category is the group of little to normal rain events, happening day-to-day. While the boundaries between these categories lack precise mathematical definition, urban water management strategies can be delineated into three distinct domains: (1) Design domain; (2) Extreme domain; (3) Everyday domain. Each domain necessitates a tailored approach to the planning and design process, with the ultimate goal of integrating the outcomes into a cohesive final plan.
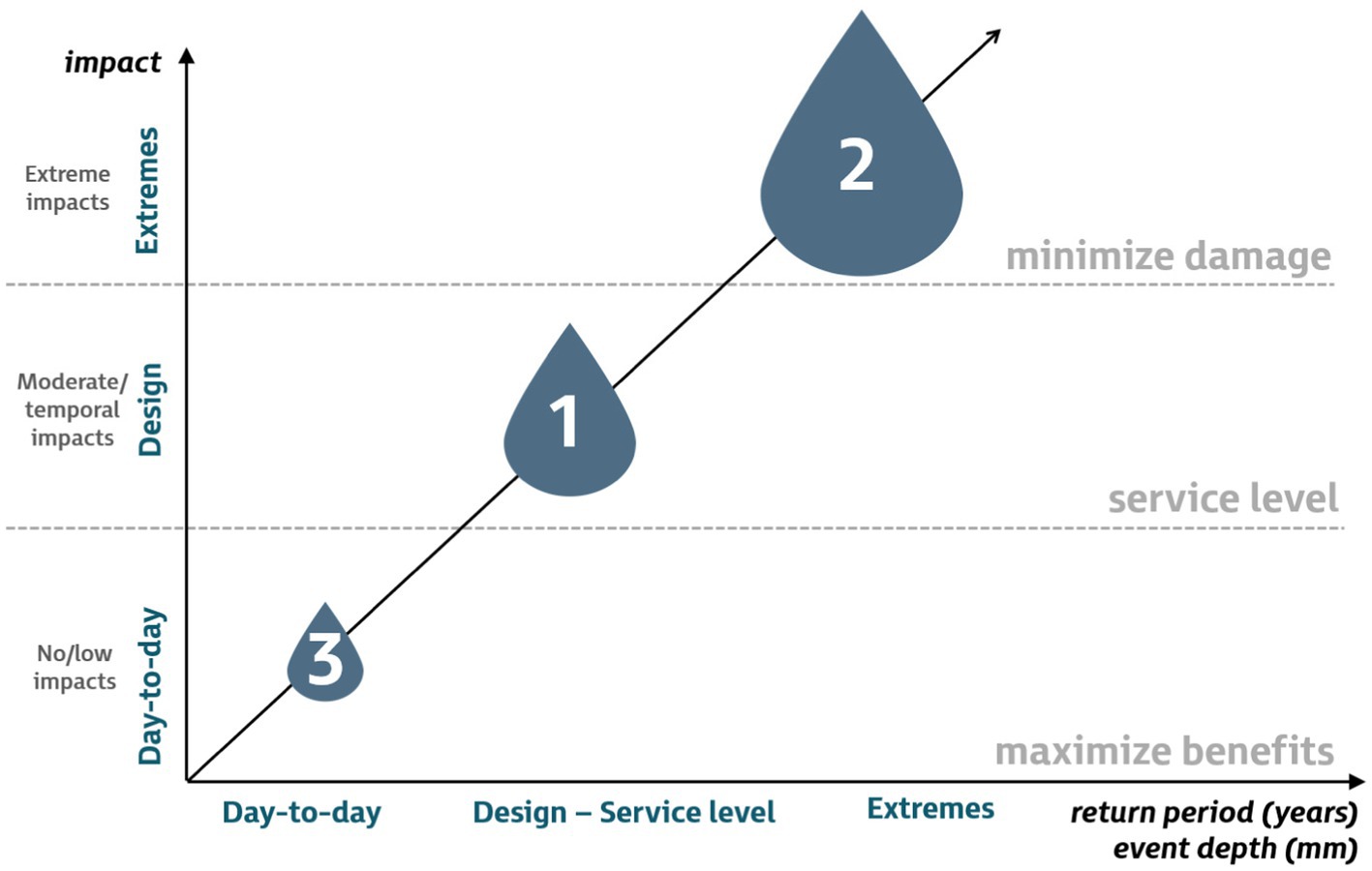
Figure 2. The Three-Points Approach (adapted from Fratini et al., 2012). The return period of rainfall events relates to the size of storm events.
This 3PA was developed for rainfall and drainage, but can also be constructed for drought. We could make a distinction between a normal, regular drought, a drought that we have prepared (designed) the water management system for, and an extreme drought that is more severe than we have prepared for.
The service level of our protection system is defined in the Design domain, by the criteria for the design of facilities that will protect us from damage of flooding and drought. These facilities can, if planned and designed carefully, become a valuable, multifunctional asset for society day-by-day, strengthening physical, ecological, economic and ecological values and services. Maximizing the benefits of these facilities in the day-to day situation is the goal of the Day-to-day domain of the 3PA. On the other hand, minimization of the physical, economic, social and ecological damage – while maintaining the benefits and services in the Day-to-day and Design domain – is the central objective for the water management interventions in this Extreme domain. Substantial damage and casualties can often be avoided with smart zoning, design and simple facilities.
The Three- Points approach has proven useful for urban flood risk management under climate change (Fratini et al., 2012; Sørup et al., 2016; Lerer et al., 2017). All three domains are to be considered in the design of interventions, aiming to maximizing the threshold, coping, recovery, and adaptive capacity that is needed to reduce the vulnerability of our environment now and on the long run (De Graaf et al., 2007).
Water management facilities for flooding and drought, with high value for society and ecology, are the so-called blue-green infrastructure (BGI) solutions. These solutions make use natural processes to control water quantity and quality. By landscaping, soil improvement, selected vegetation and specific surface and subsurface constructions the natural processes are mimicked and/or boosted, not only to improve water management but also to provide a wide range of ecosystem services. Rather than the traditional mono-functional gray drainage solutions, often hidden underground, invisible for the public, N like rain gardens, bioswales, green roofs, wet detentions ponds, wetlands – up to porous pavement and rain tanks – help create an attractive blue-green environment for humans and biodiversity. Facilities can be located on public land, but also on private properties.
Minimizing the damage in extreme, rare weather conditions requires careful spatial planning, building design and contingency planning. Priority is to provide extra protection for vital infrastructure and vulnerable people. Damage to public services and economic activities can often be prevented, or at least reduced, by adapting the landscape (Roosjen et al., 2022), the streetscape, land and building levels. Blue-green infrastructure like emergency detention reservoirs for flooding, and tanks, reservoirs and strategic groundwater stocks for water supply during extreme droughts can mitigate the damage. Development of innovative green-gray infrastructure will result in more effective solutions (Water Europe, 2021). Vertical and horizontal evacuation routes as well as emergency supplies and the availability of equipment for evacuation and recovery after the flood help minimizing the damage too. And, last but not least, awareness and preparedness of the population is crucial to minimize damage.
Figure 3 presents a conceptual depiction of the benefits and costs associated with infrastructure relative to the magnitude of a rainfall event. Failure of the conventional, gray approach during extreme events that exceed the size of the design load leads to substantial damage; costs rapidly increase with storm size, as essential functions and services are failing en recovery is costly. The introduction of the blue-green approach brought an increase in the benefits in the day-to-day and design domain, due to the co-benefits and ecosystem services provided by these facilities, up to storm events these facilities are designed for. Overloading them causes a rapid increase in damage, equal to the conventional gray approach. Consequently, the benefit/cost ratio line lies above the line of the conventional approach but drops to about the same level for very extreme events. Employing a Three-Points Approach with green-gray infrastructure (GGI) not only maximizes everyday benefits; the GGI is designed to also mitigate the damage in the event of system overload from heavy rainfall. Direct damage, indirect damage due to the failing of functions and services and recovery costs are to be minimized as an essential part of the comprehensive planning and design process. The benefits/cost ratio is now maximized in the day-to-day and design domain and drops much more slowly in the extreme domain, as direct, indirect and recovery costs are minimized with the application of green-gray infrastructure.
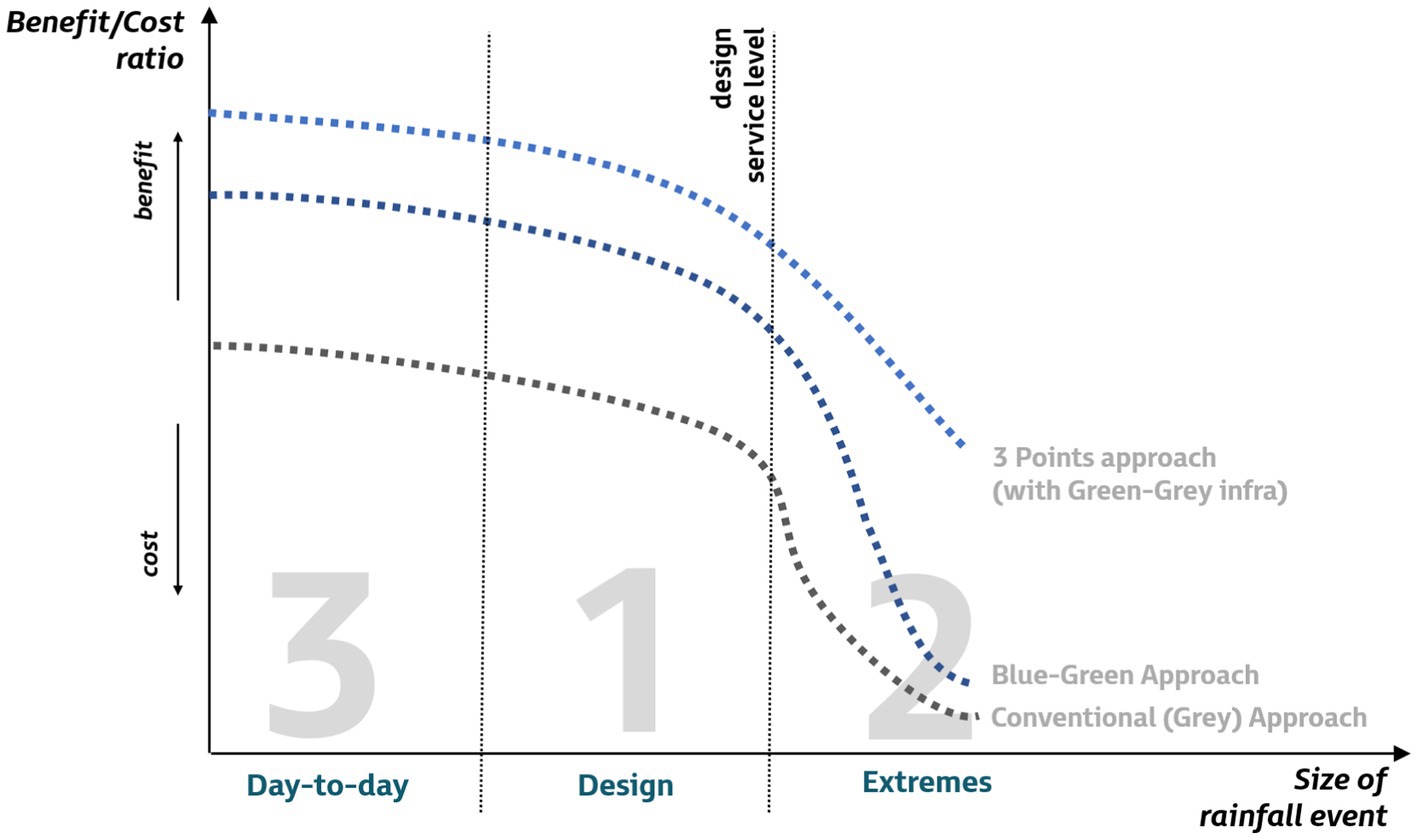
Figure 3. Conceptual image of costs and benefits of the conventional gray approach to flood risk management, the blue-green approach and the Three-Points approach using green-gray infrastructure as they develop over the three domains of rainfall event sizes.
The representation for drought is analogous, although the absolute values for benefits and costs will be different.
The Sponge City approach lacks to address events in the Extreme domain and therefore cannot deal with low probability and high-damage events (Fu et al., 2023). This equally holds for the other approaches to integrated urban water management. Implementation of the 3PA with green-gray infrastructure (GGI) and damage minimization solutions requires a well-directed, coherent transition process to change planning, policies, regulations, organization, and financing of the water management at local, regional and river basin scale. A number of issues to be addressed in this transition process is elaborated on the next section. Further to the results of CEWP’s CECoSC-project and inspired by the issues brought up by Fratini et al. (2012) and Ministerie van Infrastructuur en Waterstaat (2022) we explored the impact on water and spatial planning at various spatial scales, timescales, the development of green-gray infrastructure, the consequences for asset management and monitoring, the interdisciplinary communication and consequences for the governance of safety and resilience.
3 Issues in the novel approach to urban, regional and river basin water management
3.1 Spatial scales
Floods and droughts are not confined to a single spatial scale; hence a multiscale approach is crucial, (Zevenbergen et al., 2008). The integration of multiscale design follows from the application of the Three-Points Approach at each spatial scale, as a broad spectrum of interventions to address flood and drought challenges across a range of spatial scales is to be considered. At the local level, the design predominantly focuses on stand-alone solutions that provide immediate flood and water shortage mitigation, thus enhancing climate resilience. However, local-level solutions alone can hardly ever fully address the broader flood and drought risks. Therefore, it is necessary to extend its application to the scale of the city and the entire river basin and find a coherent set of interventions (Sui and Van de Ven, 2023). We ought to speak no longer of a Sponge City approach, but rather of a Sponge Policy approach, with interventions at building, street, district, city, region and river basin scale.
Guiding for the planning of interventions are the local water system, soil, and subsurface (Ministerie van Infrastructuur en Waterstaat, 2022). Planning through spatial scales therefor requires thorough knowledge of the local, regional, and supra-regional water, soil and subsurface system to avoid less-effective interventions. We also need to understand how the spatial scales and the interventions are interconnected; they depend on each other to work under all conditions, technically, but also economically and socially, hence requiring effective coordination and alignment among stakeholders across the urban and rural landscape. Networks of interconnected solutions tend to perform better than stand-alone interventions.
The Dutch policy brief on water soil and subsurface (Ministerie van Infrastructuur en Waterstaat, 2022) builds on the principle that problems should never be shifted, neither in space, nor in time and not from private to public land; solving problems as close to the source as possible is essential. Figure 4 shows a combination of the 3PA with the spatial scales and some examples of interventions. As can be seen, solutions to maximize the benefits and to achieve the required service level are often blue-green and local in nature, while measures to minimize damage at regional and basin scale are gray, green or a combination of the two, depending on the availability of space.
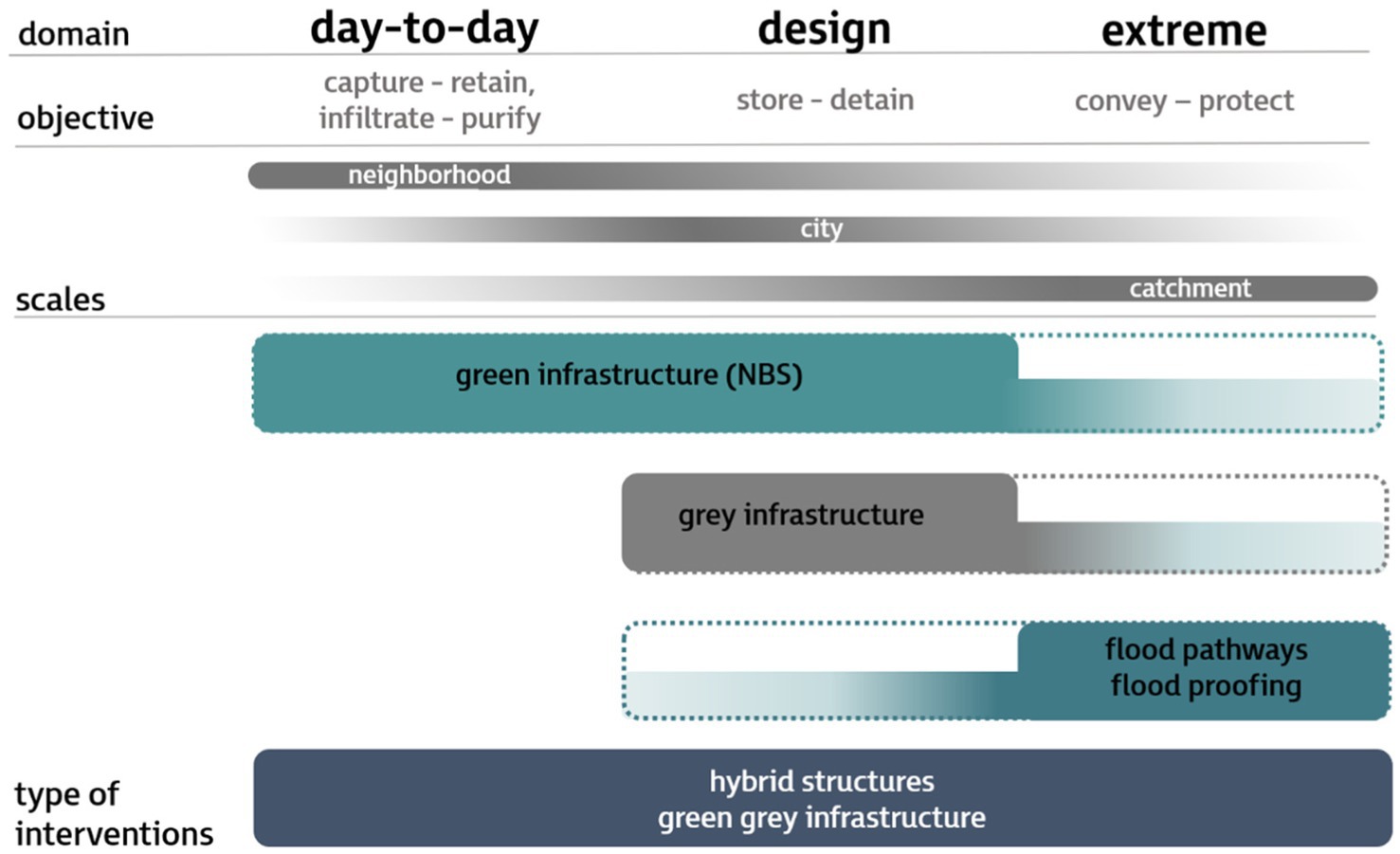
Figure 4. Three Points approach with related potential interventions and their spatial scales (adapted from Zhang et al., 2022). Blue-green infrastructure, contributing benefits in the day-to day and design domain, is found mostly at the local, neighborhood scale, or at district or city scale, while gray interventions to provide a specific service level for drainage are mostly at district to city scale. Interventions to deal with extreme event often take place at the catchment scale and can be gray as well as green. Green-gray infrastructure is meant to contribute to all three domains and to cover all scales.
3.2 Timescales
Operationalisation of the 3PA also requires an integration of temporal scales in which the short-term time window (forecasting of extreme weather events; early warning), and the long-term time window (predictions/foresight of gradual changes and trends which impacts the hydrological cycle such as urbanization and climate change) are jointly considered and synergies between selected interventions are maximized at the local, city and basin scale (Van de Ven et al., 2005).
With respect to the short-term time window, this includes decisions on how best to prepare the water system for the incoming rainfall (e.g., pre-empty detention reservoirs through anticipatory measures), and decisions to be made during extreme rainfall event. Such operational decisions can be identified in a number of ways, ranging from basing on experience to using some pre-defined set of control rules or a sophisticated optimisation methodology. Short- and medium-term forecasts, aimed at supporting anticipatory water management, involves temporal/reversible interventions, emergency plans and early-warning systems.
With respect to the long-term time window interventions range from single, localized measures to water-sensitive urban transformation at the scale of a neighborhood and city scale to the catchment scale. The time window to be considered normally expands when the spatial scale increases as interventions at the whole catchment scale generally have longer response times than those at local scale. Most measures are constructed for decades to come. To minimize the risk of lock-in, the interventions considered should allow adjustments in their construction and operation over time (Haasnoot et al., 2019). A certain degree of flexibility enables to adapt to changing conditions such as changing rainfall intensities and infiltration rates of the catchment areas.
3.3 Gray to blue-green to green-gray infrastructure
Water management in rural and urban areas is traditionally aimed at rapidly draining any surplus of water, to avoid water logging and damage. Drains with minimal land use – hence, subsurface pipes in urban areas - and maximum conveyance capacity, if necessary combined with strong pumps, are used to this end. Consequently, flooding problems were shifted downstream. Moreover, the drained area became sensitive to damage during extreme droughts; additional water is then to be supplied from outside or from local groundwater resources. Precious drinking water is sometimes used for low-end purposes, and groundwater resources became overexploited. It is now realized that more local water detention capacity is needed to reduce runoff and make local water available during periods of drought.
Blue green infrastructure (BGI) solutions help to create detention capacity, and many provide groundwater recharge; moreover, many BGI facilities clean the water through natural purification processes. A wide variety of solutions exist. BGI is known for its co-benefits. Many ecosystem services are provided in addition to the water management services, such as biodiversity, recreation, supply of food and/or building materials. See, e.g., Urban Green Blue Grids or Climate ADAPT. To get a glimpse of the diversity (Figure 5) provides a brief selection of BG solutions.
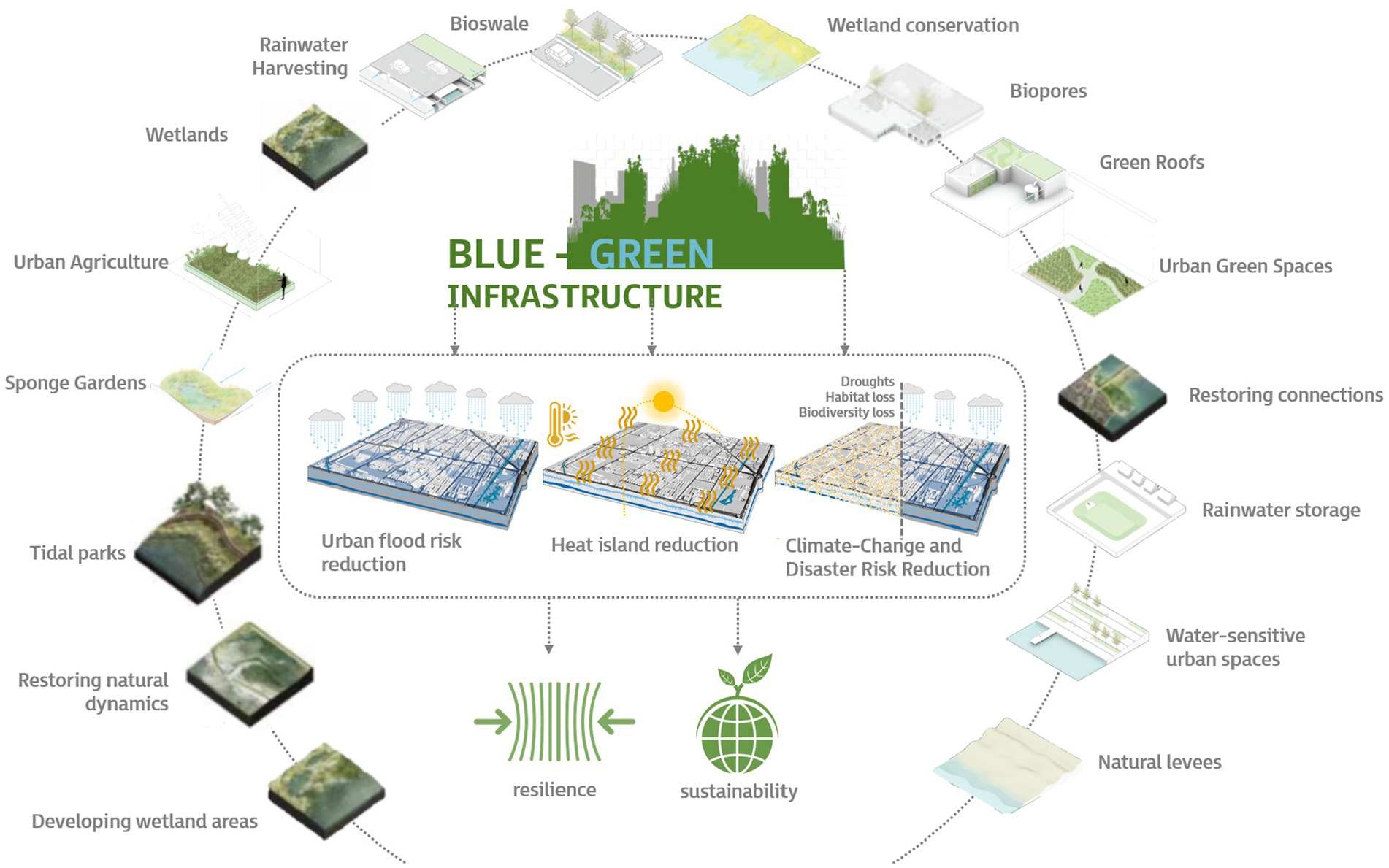
Figure 5. Examples of Blue-Green Infrastructure solutions for a resilient and sustainable urban environment. Along rivers, additional wetlands and flood plains are created to reduce flood levels and peak flows and to capture sediment. In urban areas water is detained and infiltrated, also for drought resilience and water quality improvement (Bouw and Van Eekelen, 2020; Newman and Qiao, 2022; Avellar Montezuma, 2023).
Planning, design, construction, maintenance and operation of BGI are multi-disciplinary challenges and require expertise and skills not (yet) widely available in drainage engineering and construction practice (Vollaers et al., 2021). Think of spatial planning, landscape design, horticulture, forestry, health and pest control, maintenance, governance, financing and so on. As some of these facilities are on private land, privately owned and operated, involvement of the citizens is a prerequisite for successful implementation. They too have to learn how to manage the facilities and to make use of its ecosystem services, in order to maximize its benefits and minimize the damage in case the facility becomes overloaded. This makes implementation of BGI a learning process for all stakeholders, creating new relations with other disciplines, departments and sectors.
The reliability of BGI’s water management services is different from the gray drainage practices and not guaranteed in extreme weather conditions. Examples of poor performance are detention basins that are still partially filled by earlier rainfall when that heavy storm comes, the limited water treatment performance of a BG facility in winter cold, or the harmful algae blooms in wetlands during periods of drought. BG facilities can be overloaded, by hydraulic loading or with pollution. To better deal with and to minimize damage of extreme events new combinations of BGI with gray and smart technologies are needed. Such hybrid green-gray infrastructure (GGI) solutions for water management, water supply, stormwater and wastewater treatment are the innovation challenge of the near future (Water Europe, 2021).
3.4 Asset management, data and monitoring
The introduction of BGI and GGI facilities brings new requirements for asset management, monitoring and data handling, compared to the asset management of our gray water infrastructure. BGI/GGI facilities are essential elements of the drainage and water management system, like sewer pipes, culverts, pumps and weirs. Monitoring their performance and timely interpreting the collected data is an essential part of managing these assets (Geberemariam, 2017). This starts with registering the BGI and GGI facilities in a standardized way in a formal asset register, just like sewer pipes and other gray infrastructure. In the Netherlands this follows national guidelines. Standardizing the registration system is ongoing.
More and more data is being collected remotely, through remote sensing and telemetry of water levels, flow and quality. Online information is available and often (partly) publicly accessible. Warning systems are in place in case certain levels are exceeded. Real time control systems are coming up, often combined with forecasting systems, to issue warnings and take action when there is a risk of exceeding any alarm level. The rise of artificial intelligence will boost this technological development, contributing to a water-smart society in which the value of water is permanently optimized (Water Europe, 2023).
These technological developments will inevitably have consequences for (the debate on) data protection / data availability and on the responsibility of organizations and persons regarding the monitoring of facilities, the data that is produced, their interpretation and analysis and the actions taken – or not – by individuals in specific observed or forecasted situations (Water Europe, 2023).
3.5 Communicating risk
The implementation of blue-green and green-gray solutions necessitates the involvement of additional stakeholders compared to those involved in traditional gray solutions. These new stakeholder groups encompass the parks and green departments, traffic, housing, and health departments, emergency services, other contractors for installation and maintenance, additional suppliers, consultants, landscape architects, local businesses, residents, property owners, schools, and more. All stakeholders must familiarize themselves with the characteristics of the new solutions, including their construction, properties, performance in both extreme and normal conditions, maintenance requirements, monitoring protocols, as well as understanding their strengths and weaknesses. New skills and capacities are to be developed, new rules, new language, regulations and contracts. Communication is the key to collaborative learning, needed fora successful implementation.
Communicating risk as a hard job; communicating opportunities for maximizing the benefits and for minimizing damage is easier. Introduction of the 3PA and introduction of BGI and GGI requires new communication lines in a new network of stakeholders, often aimed at collaborative learning. Excursions to see demonstration projects, meetings with front-runners and evaluations of field practices help to build confidence in the new approach and related solutions.
The transition toward this new approach to water management at the local, urban to catchment scale is a complex quest, making it inherently time-consuming. Time is a scarce resource in this process, yet it remains a prerequisite for the successful adoption of the new approach.
3.6 Governance of safety and resilience
Governance plays a crucial role in enhancing safety and resilience in water management. Effective governance entails management approaches that consider the interconnectedness of various aspects of water management, such as flood control, drought management, water quality, and ecological protection. It requires active public participation, adequate financial resources, novel business and operation models, proactive planning for future scenarios, robust enforcement mechanisms, and continuous knowledge sharing for capacity building, embedded in a robust legal and regulatory framework and an adequate organization; this all at the local urban, regional, and river basin scale (Ministerie van Verkeer en Waterstaat, 1997; IPCC, 2023). To operationalize the 3PA, local actors require enhanced capacity and an empowered role for planners and residents, including access to more effective planning support tools to be able to deal with and evaluate the technical, financial, organizational, and regulatory aspects of alternative plans.
Each of the 3 domains of the Three Points Approach are to be covered by a solid, coherent governance structure. Good governance enables the coordination, cooperation, and integration of efforts across these different domains, scales and issues, leading to a coherent management strategy. The complexity of this planning and implementation process can however lead to significant delays and stagnation. Parallel and collaborative planning, design and implementation are essential to keep the speed and to create wide support and acceptance of the final plan. Such complex collaborative actions can best be coordinated by a process manager (Van de Ven et al., 2005), responsible for smoothening and accelerating decision making by each of the involved stakeholder parties.
4 Application of the Three-Points Sponge Policy approach: a proof-of-concept in Zhengzhou, China
A first and conceptual trial of the applicability of the Three-Points Sponge Policy approach for water management planning and design was started for the city of Zhengzhou. Zhengzhou as the capital of Henan Province is situated in the Huai River Basin, on the southern bank of the Yellow River. The city has a population of over 12 million people. Historically, Zhengzhou has experienced a number of devastating floods, the most notable in 1931. This flood was caused by extreme rainfall and the failure of several dams upstream. The disaster resulted in the deaths of over 150,000 people and left millions homeless. More recently, in July 2021, Zhengzhou experienced another catastrophic flood. The daily rainfall of 624 mm observed at Zhengzhou National Meteorological Station on July 20 is 3.4 times the maximum since the station was built (189 mm, on July 2, 1978). This extreme rainfall caused flooding throughout the city, resulting in the deaths of over 300 people and a direct economic loss of 40.9 billion RMB. In response, Chinese government launched a large-scale rescue and recovery effort.
Employing the Three-Points Sponge Policy approach to identify and select interventions for the planning and design of a cohesive, resilient water management system across all spatial scales presents a complex challenge. Therefore, a ‘research by design’ approach was employed to combine potential interventions, system characteristics, and design principles in an iterative process, resulting in a comprehensive design (referred to as ‘alternative’). Two pilot areas of Zhengzhou, both seriously flood-prone and affected by the 2021-flood, were selected for analysis and design at the local scale:
• (B1) Longzi Lake Smart Island, situated in a 15 ha artificial ecological lake in the New District. Contemporary urban development; Medium density with high ground space index.
• (B2) Shangdu Historical and Cultural District in the old city of Zhengzhou. Older area; High density with low ground space index and limited space for interventions.
Figure 6 provides a photo impression of the streetscape in the two areas.
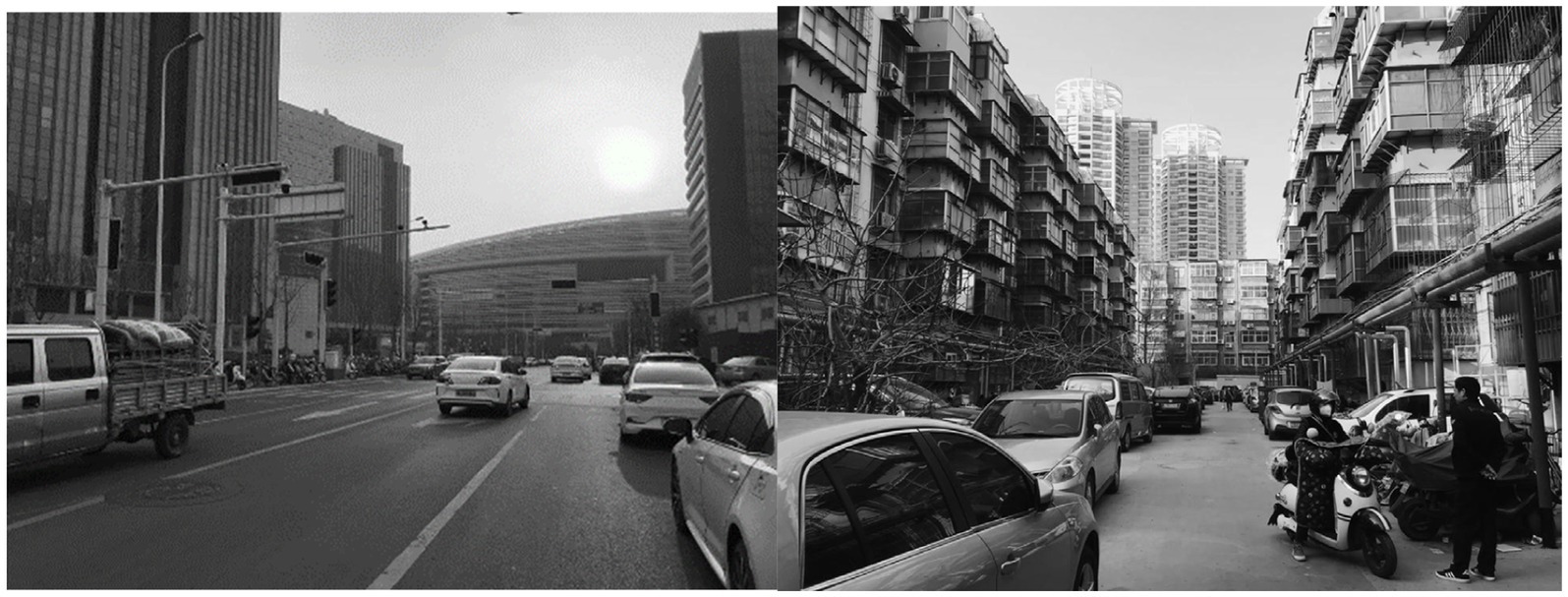
Figure 6. Representative streetscape of Longzi Lake Smart Island (left) and Shangdu Historical and Cultural district (right) (Avellar Montezuma, 2023).
Redesigns were studied at the street, neighborhood, city and catchment scale, as shown in Figure 7.
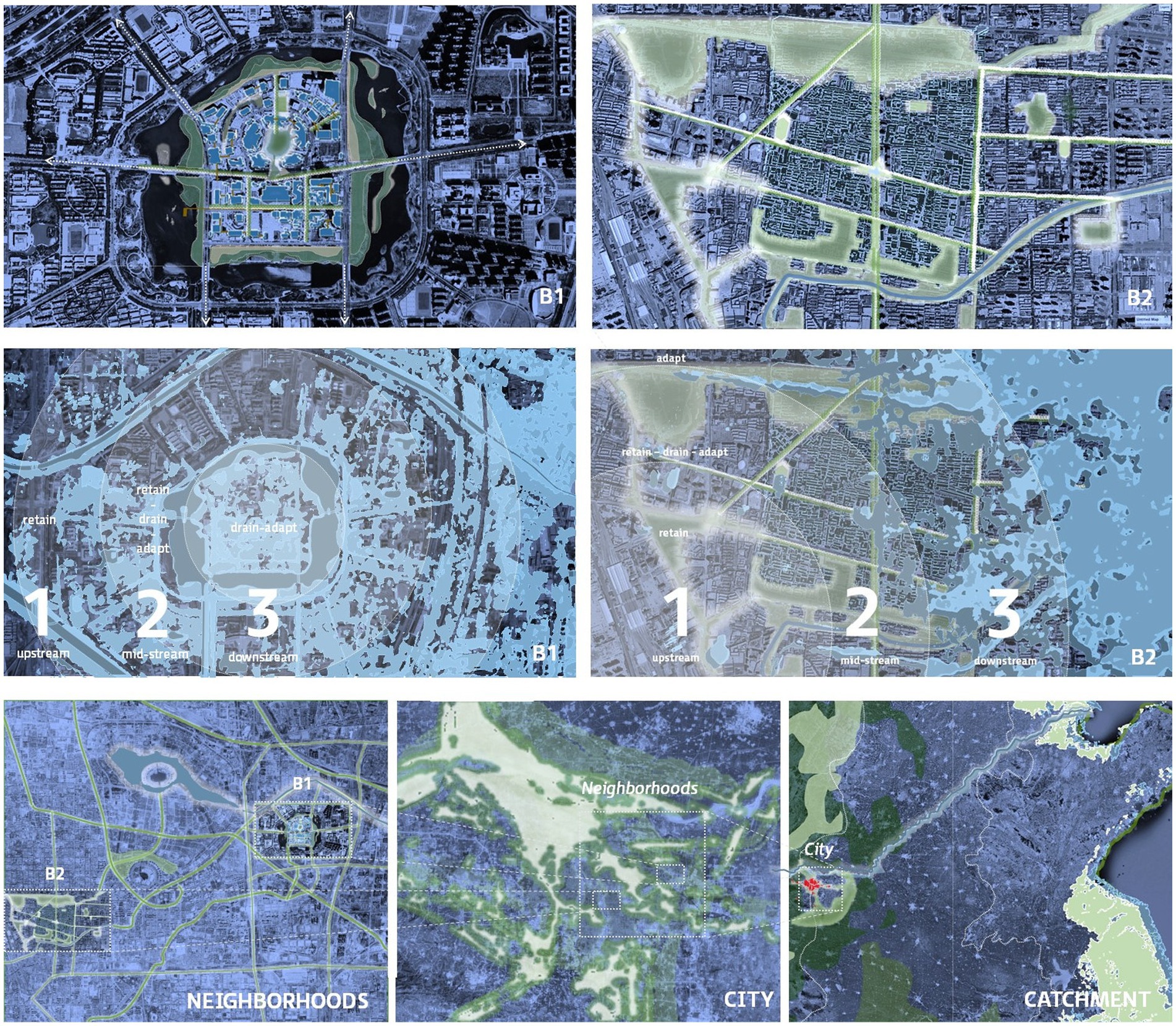
Figure 7. Top line: The two pilot areas in Zhengzhou; B1 is Longzi Lake Smart Island and B2 is Shangdu Historical and Cultural district. White areas indicate a prolongation of existing green spaces into green connections such as ecological corridors, bioswales, etc. Middle line: Flood hazard map of the two areas; substantial parts are at risk. Lower line: Neighborhoods as part of the city, as part of the catchment (Avellar Montezuma, 2023).
As Zhengzhou is located in the very upstream part of the Huai River basis the concern of the water manager should be to avoid shifting problems downstream. Stormwater detention and water quality protection are the highest priorities to create enabling conditions for water management and ecological restoration downstream. Actions further downstream in and along the Huai River and in the delta are of limited importance for the water management of Zhengzhou city.
Design efforts were consequently focused on the two pilot areas and how these could be reconstructed to minimize the damage of extreme weather events while maximizing the benefits in the day-to-day situation. This is illustrated in Figure 8 with a redesign of an existing street in Shangdu Historical and Cultural district.
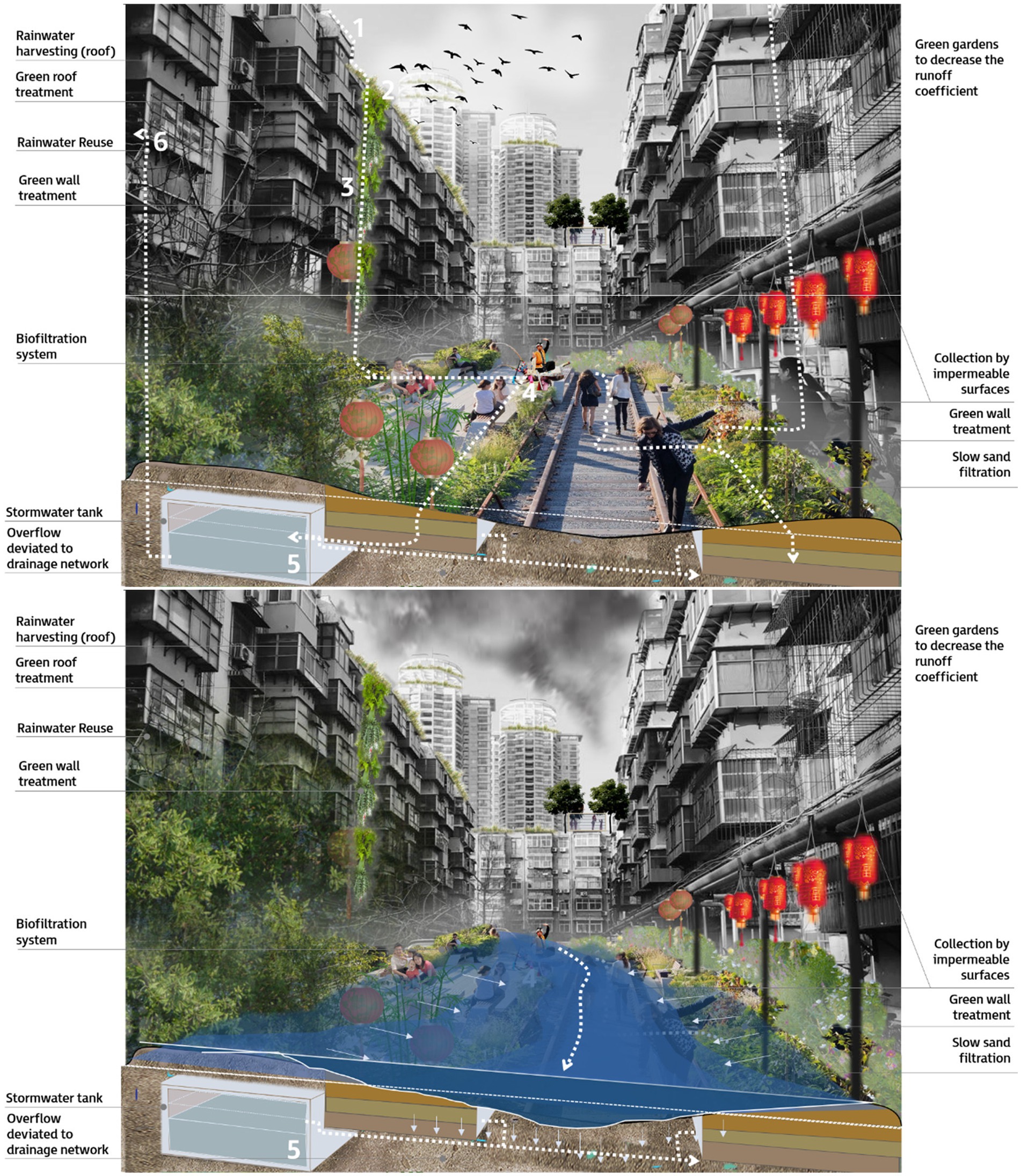
Figure 8. Potential interventions at street scale in Shangdu Historical and Cultural district to strengthen the extreme weather resilience of Zhengzhou, while maximizing ecosystem services and other benefits in area B2 (Avellar Montezuma, 2023). Day-to-day and design rainfall events are handled by the green, infiltration and subsurface facilities, while extreme rainfall events are handled by the detention and conveyance capacity of the street, without flooding the buildings.
At street, city and basin level we have selected a set of interventions of blue-green and green-gray infrastructure to be implemented across the various spatial scales. The next step will be to assess their effectiveness, taking into account the interactions and synergies between these interventions at the spatial scale of the urban district, city, and catchment area. Which will lead to system-integration, i.e., the formulation of a coherent and efficient set of interventions, possibly in several alternative plans.
As demonstrated in this case study, applying the 3PSP approach enables to go beyond the limitations of conventional approaches targeting isolated, often monodisciplinary solutions. The process of selecting an integrated and effective set of BGI/GGI interventions (alternatives) across all scales demands a co-creative, multi-disciplinary planning approach. This complex and iterative process requires active participation of numerous stakeholders and the use of interactive planning tools such as the Adaptation Support Tool (Van de Ven et al., 2016; McEvoy, 2019) or the Climate Resilient City Tool.1 The responsibility for evaluating the alternatives and for making a final choice rest with local and regional authorities. By working in this way, the comprehensive 3PSP approach ensures a well-informed decision-making process that considers the nuanced interactions between different interventions across the spatial scales.
5 Discussion and outlook
Cities in both Europe and China are increasingly suffering from flooding by extreme rainfall, drought and related heat stress and water quality problems. Climate change, ongoing urbanization and aging/decay of existing water management infrastructure together are the major drivers. New approaches to integrated urban water management (IUWM) are continuously being tested and revised to address these challenges in an effective way, such as Low Impact Development, Water Sensitive Uran Design and the Sponge City approach. These approaches all foster the widespread deployment of multi-beneficial blue-green infra solutions for creating protection up to a certain design or service level, while providing ecosystem services and other benefits. Minimizing the damage in case the water system becomes overloaded – by extreme rainfall, river flooding, extreme drought and or related issues – is not explicitly considered in these planning and design approaches. This equally holds for the systemic integration of interventions at different spatial scales, from local to catchment scale.
While analyzing the applicability of the Three-Points Approach as a potential way to strengthen the Sponge City approach to IUWM in the Zhengzhou case study, a number of design principles have been highlighted as essential terms of reference for planning multi-level resilient interventions. These principles are from different sources, but equally important for the quality of the final plan:
• Never Shift Problems, neither in place nor in time: This principle underscores the importance of not to shift issues from an area to downstream, from the present to the future, or from private to public land (Ministerie van Infrastructuur en Waterstaat, 2022).
• Use blue-green infrastructure and natural processes to ‘build with nature’ and manage water quantity and water quality, where- and whenever possible. Their adaptability to changing conditions is high and their ecosystem services are attractive (European Commission, 2020). Integration with gray solutions can make them perform better in extreme conditions (Water Europe, 2021).
• Seek synergy between flood and drought mitigating interventions. Detention (sponge) capacity is needed for both flood and drought risk reduction (Ministry of Finance, The Ministry of Housing and Urban-Rural Development, The Ministry of Water Resources, 2014).
• Embrace a water and soil systems perspective, where local soil and subsurface conditions take precedence, while simultaneously comprehending the dynamics of surface and groundwater systems at both the local and river basin scales. A deep understanding of these systems is crucial for developing robust solutions (Ministerie van Infrastructuur en Waterstaat, 2022).
• Incorporate adaptability into the design: Given the uncertainty of the future and the inevitable changes of requirements, interventions should possess inherent flexibility to accommodate changing conditions, emerging ideas, and evolving preferences (De Graaf et al., 2007).
• Embrace open access to data: Multifunctional solutions have many stakeholders involved in the planning, implementation, management, and maintenance of all the water management systems’ elements. Sharing all available data on quantity and quality is essential for balancing their interests (European Union, 2021).
• Good governance: The existing regulations, policies, organization, financing mechanisms and operational capacity to implement and maintain the facilities should match with the proposed interventions. If not, this governance context needs adaptation for a successful implementation (Ministerie van Verkeer en Waterstaat, 1997).
This list, likely incomplete, was used and found useful to discuss and evaluate alternative ideas in a qualitative way in the case study.
In conclusion, it is essential to plan and design water systems at al spatial scales for, with and by extremes beyond the design load, to minimize the damage they can do. While designing, assume it will flood – and the flood will be extreme. And do the same for droughts. The current approaches to IUWM are missing this component, not only the Sponge City approach (Fu et al., 2023). Different types of floods and droughts require different management strategies, including structural and non-structural interventions. Evident is that vulnerable people, critical infrastructure, and the local fauna need extra attention and protection under such extreme weather conditions.
At the same time the benefits and services provided by these planned interventions can be maximized. By combining blue-green and gray solutions for water management at the urban and catchment scale the benefits of nature-based solutions are combined with the robustness of gray solutions and smart control technologies, to perform better under extreme conditions (Water Europe, 2021).
By adopting the Three-Points Approach (3PA) as an overarching framework to guide existing planning practices like the Sponge City approach, both the risks as well as the benefits of planned interventions are being considered in a holistic way. To achieve integration into a Three-Points Sponge Policy approach, it is essential to apply a portfolio of interventions which covers the three domains of the 3PA. These interventions should be implemented across a range of spatial scales, from local to catchment scale, creating interconnections and coherence between different components of the system. This however requires communication, cooperation and co-creation of departments, authorities and stakeholders that so far have separated roles and responsibilities. Considering all aspects and issues of the Three-Points Sponge Policy (3PSP) approach, including the spatial scales, relevant time scales as well as the governance issues related to the implementation – legislation, communication, stakeholder participation, organization, financing, et cetera – and the consequences for performance monitoring and asset management in planning and design is a learning process for all participants. As demonstrated in a first conceptual pilot application, this 3PSP approach structures and supports this process.
Data availability statement
The original contributions presented in the study are included in the article/supplementary material, further inquiries can be directed to the corresponding author.
Author contributions
FV: Conceptualization, Methodology, Writing – original draft, Writing – review & editing. CZ: Conceptualization, Methodology, Writing – original draft, Writing – review & editing. MA: Methodology, Visualization, Writing – original draft, Writing – review & editing. ZD: Writing – review & editing. WV: Validation, Writing – review & editing. SC: Methodology, Writing – original draft.
Funding
The author(s) declare financial support was received for the research, authorship, and/or publication of this article. This research was funded by the China Europe Cooperation on Sponge Cities (CECoSC) project, part of the China Europe Water Platform (CEWP), and by the Netherlands’ Ministry of Infrastructure and Water Management.
Conflict of interest
The authors declare that the research was conducted in the absence of any commercial or financial relationships that could be construed as a potential conflict of interest.
Publisher’s note
All claims expressed in this article are solely those of the authors and do not necessarily represent those of their affiliated organizations, or those of the publisher, the editors and the reviewers. Any product that may be evaluated in this article, or claim that may be made by its manufacturer, is not guaranteed or endorsed by the publisher.
Footnotes
References
Amsterdam Rainproof (2021). Available at: https://www.rainproof.nl/english
Avellar Montezuma, M. (2023). Minimizing risks and maximizing benefits; 3PA and design integration for the day-to-day, design, exceedance and extreme flood events. MSc thesis. Delft: IHE Delft Institute for Water Education.
Bouw, M, and Van Eekelen, E (2020). Building with nature: creating, implementing and upscaling nature-based solutions.
Browder, G., Ozment, S., Bescos, I. R., Gartner, T., and Lange, G.-M. (2019). Integrating green and grey: creating next generation infrastructure : Washington, DC: World Bank Group & World Resources Institute.
CEWP (2022). Policy guidance on China-Europe cooperation on sponge cities. China Europe Water Platform. Available at: https://cewp.eu/sites/default/files/2023-05/Policy%20Guidances%20of%20Partnership%20Instrument%20Project%20-EN.pdf
Chan, F. K. S., Yang, L. E., Scheffran, J., Mitchell, G., Adekola, O., Griffiths, J., et al. (2021). Urban flood risks and emerging challenges in a Chinese delta: the case of the Pearl River Delta. Environ. Sci. Pol. 122, 101–115. doi: 10.1016/j.envsci.2021.04.009
Chen, S., van de Ven, F. H. M., Zevenbergen, C., Verbeeck, S., Ye, Q., Zhang, W., et al. (2021). Revisiting China’s Sponge City planning approach: lessons from a case study on Qinhuai District, Nanjing. Front. Environ. Sci. 9:748231. doi: 10.3389/fenvs.2021.748231
City of Copenhagen (2012). Cloudburst management plan 2012. City of Copenhagen. Available at: https://international.kk.dk/sites/default/files/2021-09/Cloudburst%20Management%20plan%202010.pdf
Commonwealth of Australia [COAG] (2004). Intergovernmental agreement on a National Water Initiative between the commonwealth of Australia and the governments of New South Wales, Victoria, Queensland, South Australia, the Australian Capital Territory and the Northern Territory. Available at: https://www.informea.org/en/legislation/intergovernmental-agreement-national-water-initiative.
De Graaf, R., Van de Giesen, N., and Van de Ven, F. H. M. (2007). Alternative water management options to reduce vulnerability for climate change in the Netherlands. Nat. Hazards 51, 407–422. doi: 10.1007/s11069-007-9184-4
Ding, Z. (2023). Application of the 3PA approach in urban China: case study Zhengzhou. MSc thesis. Delft: IHE Delft Institute for Water Education.
European Commission (2020). Directorate-General for Research and Innovation, Biodiversity and nature-based solutions – Analysis of EU-funded projects, Publications Office of the European Union.
European Union (2021). European data. Available at: https://data.europa.eu/en/publications/datastories/how-open-data-supports-water-management
Feng, W., Liu, Y., and Gao, L. (2022). Stormwater treatment for reuse: current practice and future development – a review. J. Environ. Manag. 301, 113830–114797. doi: 10.1016/j.jenvman.2021.113830
Fletcher, T. D., Shuster, W., Hunt, W. F., Ashley, R., Butler, D., Arthur, S., et al. (2015). SUDS, LID, BMPs, WSUD and more – the evolution and application of terminology surrounding urban drainage. Urban Water J. 12, 525–542. doi: 10.1080/1573062X.2014.916314
Fratini, C. F., Geldof, G. D., Kluck, J., and Mikkelsen, P. S. (2012). Three-points approach (3PA) for urban flood risk management: a tool to support climate change adaptation through transdisciplinarity and multifunctionality. Urban Water J. 9, 317–331. doi: 10.1080/1573062X.2012.668913
Fu, G., Zhang, C., Hall, J. W., and Butler, D. (2023). Are sponge cities the solution to China's growing urban flooding problems? WIREs Water 10:e1613. doi: 10.1002/wat2.1613
Fujita, S. (1997). Measures to promote stormwater infiltration. Water Sci. Technol. 36, 289–293. doi: 10.2166/wst.1997.0681
Geberemariam, T. K. (2017). Post construction green infrastructure performance monitoring parameters and their functional components. Environments 4:2. doi: 10.3390/environments4010002
Geldof, DG, and Kluck, J (2008). The three-points approach. In: Proceedings of 11th ICUD - international conference on urban drainage, 31 August–5 September 2008. Edinburgh.
Haasnoot, M., Warren, A., and Kwakkel, J. H. (2019). “Dynamic adaptive policy pathways (DAPP)” in Decision making under deep uncertainty. eds. V. Marchau, W. Walker, P. Bloemen, and S. Popper (Cham: Springer).
Handmer, J., Hongda, Y., Kundzewicz, Z. W., Arnell, N., Benito, G., Hatfield, J., et al. (2012). “Changes in climate extremes and their impacts on the natural physical environment” in Managing the risks of extreme events and disasters to advance climate change adaptation. eds. C. B. Field, V. Barros, T. F. Stocker, and D. Qin (IPCC New York, NY: Cambridge University Press), 231–290.
Hoogvliet, VF, Buma, J, Van Oostrom, N, Brolsma, R, Filatova, T, Verheijen, J, et al. (2012). Schades door watertekorten en -overschotten in stedelijk gebied; Quick scan van beschikbaarheid schadegetallen en mogelijkheden om schades te bepalen. (in Dutch) Deltares report 1205463-000-BGS-0003
IPCC (2022). “Contribution of working group II to the sixth assessment report of the intergovernmental panel on climate change” in Climate change 2022: impacts, adaptation, and vulnerability. eds. H.-O. Pörtner, D. C. Roberts, M. Tignor, E. S. Poloczanska, K. Mintenbeck, and A. Alegría, et al. (Cambridge University Press), 3056.
IPCC (2023) in Climate change 2023: synthesis report. Contribution of working groups I, II and III to the sixth assessment report of the intergovernmental panel on climate change [Core Writing Team. eds. H. Lee and J. Romero (Geneva, Switzerland: IPCC), 184.
Lerer, S. M., Righetti, F., Rozario, T., and Mikkelsen, P. S. (2017). Integrated hydrological model-based assessment of stormwater management scenarios in Copenhagen's first climate resilient neighbourhood using the three point approach. Water 9:883. doi: 10.3390/w9110883
Liu, W., Sun, F., Ho Lim, W., Zhang, J., Wang, H., Shiogama, H., et al. (2018). Global drought and severe drought-affected populations in 15 and 2 °C warmer worlds. Earth Syst. Dynam. 9, 267–283. doi: 10.5194/esd-9-267-2018
Machairas, I., and Van de Ven, F. (2022). An urban drought categorization framework and the vulnerability of a lowland city to groundwater urban droughts. Nat. Hazards. 116, 1403–1431. doi: 10.1007/s11069-022-05767-0
McEvoy, S. (2019). Planning support tools in urban adaptation practice. PhD thesis: Delft, The Netherlands: Delft University of Technology.
Ministerie van Infrastructuur en Waterstaat (2022). Water en Bodem sturend (in Dutch) letter of the minister of infrastructure and water management to the chair of Netherlands’ parliament, 25 November 2022. Available at: https://open.overheid.nl/documenten/ronl-c35e65eba0903d738ae26dab222462337b0d8de7/pdf
Ministerie van Verkeer en Waterstaat (1997). Resultaatgericht Beleid. Advies bij Beleidsontwikkeling, Communicatie en Samenwerking. Adviesunit Resultaatgericht Beleid, Den Haag: Drukkerij Deltadruk.
Ministry of Finance, The Ministry of Housing and Urban-Rural Development, The Ministry of Water Resources (2014). Notice on launching the central financial support for Sponge City construction pilot Programme. Available at: https://www.mohurd.gov.cn/gongkai/zhengce/zhengcefilelib/201501/20150115_220129.html
Ministry of Finance, The Ministry of Housing and Urban-Rural Development, The Ministry of Water Resources (2016). Notice on launching the 2016 central financial support for Sponge City construction pilot Programme. Available at: https://www.mohurd.gov.cn/gongkai/zhengce/zhengcefilelib/201603/20160302_226802.html
Ministry of Finance, The Ministry of Housing and Urban-Rural Development, The Ministry of Water Resources (2021). Notice on launching the comprehensive Sponge City construction demonstration programme across the entire region. Available at: https://www.gov.cn/zhengce/zhengceku/2021-04/26/content_5602408.htm
Ministry of Finance, The Ministry of Housing and Urban-Rural Development, The Ministry of Water Resources (2022). Notice on launching the second batch of comprehensive Sponge City construction demonstration Programme across the entire region under "14th five-year plan". Available at: https://www.gov.cn/zhengce/zhengceku/2022-04/15/content_5685362.htm
Ministry of Science and Technology (2021). "14th five-year" National key R&D Plan "major natural disaster prevention and public safety" key project application guidelines 2021. Available at: http://www.research.pku.edu.cn/docs/20210408171414435476.pdf
MoHURD (2014). Technical guide to Sponge City [海绵城市建设技术指南]. Beijing: Ministry of Housing and Urban-Rural Development.
MoHURD (2015). Sponge City construction performance evaluation and evaluation index (trial). Ministry of Housing and Urban-Rural Development. Available at: http://www.mohurd.gov.cn/wjfb/201507/W020150715042911.doc
MoHURD (2018). Evaluation standards for Sponge City construction. Beijing: China Architecture & Building Press.
MoHURD (2022). Announcement of the Ministry of Housing and Urban-Rural Development on issuing the National Standard Code for urban and rural drainage engineering project : Beijing, China: The Ministry of Housing and Urban-Rural Development.
Montanari, A., Nguyen, H., Rubinetti, S., Ceola, S., Galelli, S., Rubino, A., et al. (2023). Why the 2022 Po River drought is the worst in the past two centuries. Science. Advances 9, 1–8. doi: 10.1126/sciadv.adg8304
Newman, GD, and Qiao, Z. (2022) Landscape architecture for sea level rise: Innovative global solutions. 1st ed. Routledge.
O’Donnell, E. C., and Thorne, C. R. (2020). Drivers of future urban flood risk. Philos. Transact. A Math. Phys. Eng. Sci. 378:20190216. doi: 10.1098/rsta.2019.0216
Roosjen, R, Niesten, M, and De Leeuw, A (2022). Systeemrandvoorwaarden 2100; onderdeel van Raamwerk Waterveiligheidslandschappen (system boundary conditions 2100 for the flood safety landscapes framework, in Dutch). Available at: https://publications.deltares.nl/11208010_019_0001.pdf
Sørup, H. J. D., Lerer, S. M., Arnbjerg-Nielsen, K., Mikkelsen, P. S., and Rygaard, M. (2016). Efficiency of stormwater control measures for combined sewer retrofitting under varying rain conditions: quantifying the three-points approach (3PA). Environ. Sci. Pol. 63, 19–26. doi: 10.1016/j.envsci.2016.05.010
State Council (2021). Implementation opinions of the general Office of the State Council on strengthening urban pluvial flood control. The general of the state council. Available at: https://www.gov.cn/zhengce/content/2021-04/25/content_5601954.htm
State Council (2022). The investigation report on "720" cloudburst in Zhengzhou, Henan. Disaster Investigation Team of the State Council. Available at: https://www.mem.gov.cn/gk/sgcc/tbzdsgdcbg/202201/P020220121639049697767.pdf
Sui, X., and Van de Ven, F. H. M. (2023). The influence of low impact development (LID) on basin runoff in a half-urbanized catchment: a case study in San Antonio, Texas. J. Hydrol. 616:128793:128793. doi: 10.1016/j.jhydrol.2022.128793
Tkachenko, N., Procter, R., and Jarvis, S. (2016). Predicting the impact of urban flooding using open data. R. Soc. Open Sci. 3:160013. doi: 10.1098/rsos.160013
Tradowsky, J. S., Philip, S. Y., Kreienkamp, F., Kew, S. F., Lorenz, P., Arrighi, J., et al. (2023). Attribution of the heavy rainfall events leading to severe flooding in Western Europe during July 2021. Clim. Chang. 176:90. doi: 10.1007/s10584-023-03502-7
Van de Ven, F. H. M., Snep, R. P. H., Koole, S., Brolsma, R., Van der Brugge, R., Spijker, J., et al. (2016). Adaptation planning support toolbox: measurable performance information based tools for co-creation of resilient, ecosystem-based urban plans with urban designers, decision-makers and stakeholders. Environ. Sci. Pol. 66, 427–436. doi: 10.1016/j.envsci.2016.06.010
Van de Ven, F. H. M., Tjallingii, S. P., Baan, P., Van Eijk, P., and Rijsberman, M. (2005). Water in Drievoud; benaderingen voor stedelijke waterplannen : (in Dutch) Eburon Delft.
Vollaers, V., Nieuwenhuis, E., Van de Ven, F., and Langeveld, J. (2021). Root causes of failures in sustainable urban drainage systems (SUDS): an exploratory study in 11 municipalities in the Netherlands. Blue Green Syst. 3, 31–48. doi: 10.2166/bgs.2021.002
Water Europe (2021). Opportunities for hybrid grey and green infrastructure in water management: challenges and ways forward. Available at: https://watereurope.eu/wp-content/uploads/HGGI-Publication_online.pdf
Water Europe (2023). Water vision, the value of water – towards a water-smart society. Available at: https://watereurope.eu/wp-content/uploads/WE-Water-Vision-2023_online.pdf
Wong, T. H. F. (2007). Water sensitive urban design; the journey thus far. Aust. J. Water Resour. 10, 213–222. doi: 10.1080/13241583.2006.11465296
World Health Organization (2002). Floods: climate change and adaptation strategies for human health: report on a WHO meeting: London, United Kingdom, 30 June– 2 July 2002. Geneva, Switzerland: World Health Organization Regional Office for Europe.
Xinhua (2021). The fourteenth five-year plan for the National Economic and social development of the People's Republic of China and outline of long-term goals for 2035, vol. 2021. Beijing: Xinhua News Agency.
Zevenbergen, C., Veerbeek, W., Gersonius, B., and Herk, S. (2008). Challenges in urban flood management: travelling across spatial and temporal scales. J. Flood Risk Manag. 1, 81–88. doi: 10.1111/j.1753-318X.2008.00010.x
Keywords: Sponge City, Three-Points Approach, green-gray infrastructure, extreme events, spatial scales, principles, resilience, planning
Citation: van de Ven FHM, Zevenbergen C, Avellar Montezuma M, Ding Z, Veerbeek W and Chen S (2024) The Three-Points Sponge Policy approach; toward an enhanced multi-level resilience strategy. Front. Water. 6:1361058. doi: 10.3389/frwa.2024.1361058
Edited by:
Faith Ka Shun Chan, The University of Nottingham Ningbo, ChinaReviewed by:
Jeroen Kluck, Amsterdam University of Applied Sciences, NetherlandsJan Friesen, Helmholtz Association of German Research Centres (HZ), Germany
Rui Manuel Vitor Cortes, University of Trás-os-Montes and Alto Douro, Portugal
Copyright © 2024 van de Ven, Zevenbergen, Avellar Montezuma, Ding, Veerbeek and Chen. This is an open-access article distributed under the terms of the Creative Commons Attribution License (CC BY). The use, distribution or reproduction in other forums is permitted, provided the original author(s) and the copyright owner(s) are credited and that the original publication in this journal is cited, in accordance with accepted academic practice. No use, distribution or reproduction is permitted which does not comply with these terms.
*Correspondence: Frans H. M. van de Ven, ZnJhbnMudmFuZGV2ZW5AZGVsdGFyZXMubmw=; Zi5oLm0udmFuZGV2ZW5AdHVkZWxmdC5ubA==
†ORCID: Frans H. M. van de Ven, https://orcid.org/0000-0003-4268-8918
Chris Zevenbergen, https://orcid.org/0000-0003-0807-5253
Mila Avellar Montezuma, https://orcid.org/0000-0003-4435-1569
William Veerbeek, https://orcid.org/0000-0002-8636-6630
Shiyang Chen, https://orcid.org/0009-0005-2609-0096