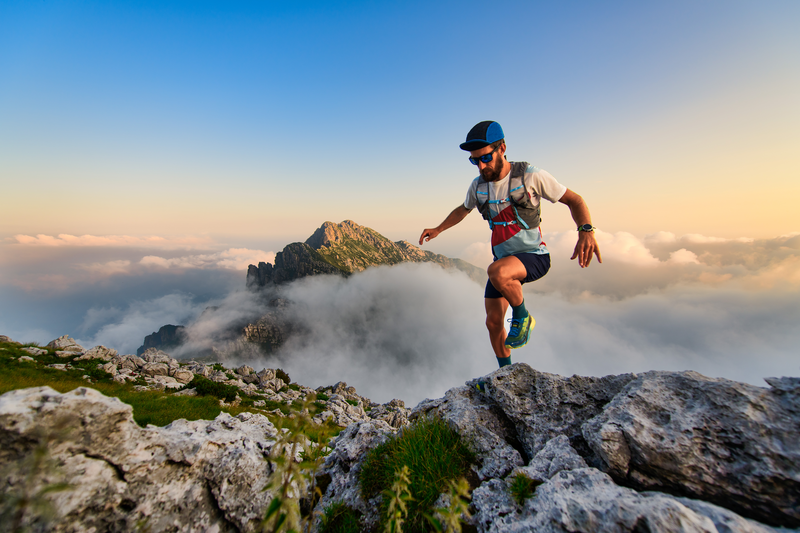
94% of researchers rate our articles as excellent or good
Learn more about the work of our research integrity team to safeguard the quality of each article we publish.
Find out more
REVIEW article
Front. Water , 17 July 2024
Sec. Water and Human Systems
Volume 6 - 2024 | https://doi.org/10.3389/frwa.2024.1360941
This article is part of the Research Topic Advances in Biomonitoring of African Aquatic Ecosystems, Volume II View all 5 articles
The contribution of citizen scientists to environmental monitoring is notably increasing significantly. Governments worldwide establish Water Users Associations (WUAs) as a good practice model to implement Integrated Water Resource Management (IWRM) at local levels. These associations target different stakeholders depending on the expected outcome. However, their effectiveness, especially in East Africa, can only be determined case by case. In Kenya, Water Resources Users Associations are registered, voluntary community groups whose members are water users and land owners. Similarly, in Uganda, community-based initiatives exist, especially along degraded rivers, involving cooperation between local communities and regional water authorities. On the contrary, it has been reported that in Tanzania, many community initiatives created become non-functional within 2–3 years of induction. In general, the main responsibility of Water Users Associations or its equivalent is to manage and conserve water resources for sustainable uses. In most cases, water quality monitoring by communities through citizen science is limited to the visual appearance of water in the river channel. While this can indicate the water’s esthetic value, it is insufficient for assessing the ecological status of rivers, which is influenced by a variety of physical, chemical, biological, and socioeconomic factors. The use of bioindicators has been advocated as a feasible method for community-based water quality river monitoring. Therefore, this review explores the commonly used bio-indicators and bio-assessment tools for river health assessment and their complexities when using a biomonitoring community-based approach through citizen science. Tolerance and intolerance macroinvertebrate and fish metric protocols that utilize identification keys have been recommended. These protocols are designed to be user-friendly and require minimal taxonomic expertise, making them easy for community volunteers to use with minimal basic training. The sustainability of these citizen science initiatives relies on the motivation of volunteers, the frequency of monitoring activities, and collaboration with researchers and government agencies. These initiatives not only facilitate environmental monitoring but also foster community engagement and awareness regarding the ecological status of rivers, thereby addressing knowledge and data gaps necessary for effective policy-making. This approach provides a practical model for environmental stewardship and participatory resource management in East Africa.
Globally, rivers are the most vulnerable aquatic systems due to increased exposure to intertwined stressors (Mamun and An, 2020). These stressors, particularly human settlements and other anthropogenic activities, have an immense impact on river systems’ health. Social stimulation models have been used to assess the health status of several river systems, including identifying the Drivers, Pressure, State, Impact, and Response (DPSIR). However, the DPSIR framework, which elaborates the cause–effect relationships between interacting components of social, economic, and environmental systems, had barely been used in East Africa before. However, several drivers affecting rivers in Uganda and Kenya have been reported by Andreas Melcher (Personal communication, 22nd February 2023, during an online training on Community-Based Water Quality Monitoring in River Health). Agriculture, industry, and urban development have been identified as the key drivers, with pollution and physical alteration of channel characteristics being the main pressures. However, DIPSIR requires the availability of water quality data and information, indicating the necessity of a structured health monitoring system for the river basins. This has been a big challenge for many rivers, especially in East Africa, posing a gap toward the development of science-based management regulations and policies (Kagalou et al., 2012; Sendzimir and Schmutz, 2018; Georgiou et al., 2024). In addition, factors such as the timing and duration of the sampling period may cause differences in the estimates of targeted organisms and benthic biodiversity reported for some West African streams (Kaboré et al., 2016).
Furthermore, the development of environmental monitoring tools strongly relies on several principles: technical co-evolution of data processing techniques, the use of scientific principles in the design of monitoring systems at the local, regional, and global scales, and the use of data from humankind risk assessment and the environment (Feld et al., 2011; Dahm et al., 2013). To put in place a monitoring system, information systems are key for effective long-term management strategies of water systems, of which many are facing complex socioecological challenges (Naigaga et al., 2011). Therefore, information leading to an understanding of the effects of some of the key environmental stressors (land use change, urbanization, and industrialization) on the status of river resources is essential for formulating effective basin management plans (Urbanič et al., 2020) for wise use. This infers that there is a need for a concerted effort to enhance river systems’ health monitoring due to their close proximity to the people and their livelihoods, hence more exposed to human-impacted stressors. Exploring other options for data acquisition to gather more information on the ecological integrity of rivers is crucial. The option of citizen science through community-based action for empowering citizens to monitor the status of their immediate rivers, identify issues and causes, and lobby for action from relevant water authorities cannot be ignored in the 21st century, where the world is facing major environmental challenges such as climate change and its extreme hydrological impacts, particularly to rivers.
Citizen science, also sometimes referred to as community science, has drawn a lot of attention since the mid-1990s. There are several definitions of citizen science in literature (Hecker et al., 2019) derived from either policy or practice impacts. Haklay et al. (2021) demonstrated the complexities and challenges of defining citizen science based on different contexts and objectives of those defining it: policymakers, funding agencies, scientific communities, and practitioners. However, when adopting citizen science initiatives, a common understanding among the relevant stakeholders would be enough to identify the influencing factors and preconditions to develop citizen science practices in the required context. This study adopts a broad definition of citizen science to refer to the active engagement of the general public (of varying education levels) to produce new knowledge for science and society in collaboration with scientists. The general public is then viewed as citizen scientists whose motivation is the desire for awareness to increase their scientific literacy, initiate policy and decisions, or fill gaps in societal academia-led monitoring of ecosystem health (Whitelaw et al., 2003; Conrad and Hilchey, 2011). Their motivation may be influenced by age, level of education, gender, and socioeconomic status. Community-based monitoring is a concept of citizen science defined as “a process where concerned citizens, government agencies, industry, academia, community groups, and local institutions collaborate to monitor, track and respond to issues of common community (environmental) concern” (Whitelaw et al., 2003; Conrad and Hilchey, 2011) and/or co-management of natural resources and catchments, where stakeholders are all involved (Keough and Blahna, 2006).
Citizen scientists play a fundamental role in the conservation of river ecosystems. The Kenyan Water Act, 2002, section 15 (3e), led to the formation of Water Resource Users Associations (WRUAs) in Kenya, similar to Water Users Associations (WUAs) in Tanzania (Allouche, 2016; Richards, 2019). Water associations are registered and voluntary community groups comprising men and women of varying ages and education levels living within river catchments. It recognizes the role of communities in the stewardship and management of water resources, which was included in the Citizen Index of Ecological Integrity (CIEI) (Aura et al., 2021). Their role is to monitor water quality, resolve water conflicts arising from water use, share information and ideas about rivers, and protect and conserve them. In most cases, community-based water quality monitoring is mainly through visual observations of water, which does not reveal the ecological status. Despite East African countries transitioning initiatives to use biomonitoring for water quality, the development of citizen science is still at a rudimentary budding state (Requier et al., 2020). Therefore, this study gives novel insights into possible biomonitoring tools, such as indices and models, and identifies suitable indicators that are easy to adopt, affordable, and reliable for assessing river health. However, for successful citizen science monitoring, the volunteers need awareness of the tools available and those that they can easily use through training and retooling.
Citizen science creates awareness of the necessity of continuous monitoring of rivers and the need for data quality assurance in the communities. Bioassessment has been identified as one of the best strategies to monitor aquatic systems’ health and response to disturbance and environmental characteristics shifts. Therefore, the need for bioassessment studies of these systems has led to the development and adoption of various biomonitoring tools (Table 1). In recent times, initiatives for the development of different bioassessment tools for African river systems based on different responses of organisms to environmental variations are on the increase. Bioassessment tools are mostly preferred because they are sensitive, highly robust, cost-effective, and easy to interpret (Dalu et al., 2016). However, no single tool can be used across all the river systems because of their diverse and sometimes dynamic characteristics, especially in tropical systems.
Table 1. Characteristics of different biomonitoring tools adopted or developed for assessment of water quality and system health in African rivers.
Rapid Bioassessment Protocols (RBPs) are some of the tools that have been proven to be reliable and quick in evaluating the water quality of riverine ecosystems (Lowe et al., 2013). South African Scoring System (SASS) was first developed in Africa using riverine macroinvertebrates to evaluate the state of South African rivers (Dickens and Graham, 2002) and in Zimbabwe (Mwedzi et al., 2016). SASS has been further modified, standardized, and adopted by other countries, such as the Zambia Invertebrates Scoring System (ZMSS), Okavango Assessment System (OKAS) in Botswana, and Namibia Scoring System (NASS) (Lowe et al., 2013). SASS has also been successfully adopted in other sub-Saharan countries, including Ethiopia, where a laboratory-based macroinvertebrate biotic system (ETHbios) was developed (Aschalew and Moog, 2015). In Nigeria, for instance, the Niger Delta Urban Multimetric Index (MINDU) has been developed and applied for evaluating the water quality of urban rivers (Edegbene et al., 2022) in addition to various biometric indices, including Hilsenhoff’s Biotic Index previously adopted for water quality monitoring in the country (Ogbeibu et al., 2013).
In East Africa, SASS has been applied with modifications successfully in Uganda and Tanzania, for example, Rwenzori Score (RS) and Tanzania River Scoring System (TARISS), respectively. Furthermore, TARISS has been applied successfully in the Rwanda and Uganda river systems (Dusabe et al., 2019; Tumusiime et al., 2019). In Kenya, SASS version 5 has been used in many rivers, including the Mara River (Oigara and Masese, 2017) and the Mount Kenya Rivers (M’Erimba et al., 2014). However, one of the limitations of RBPs is the adoption in non-wadeable rivers more than 20 m wide, which tend to adjust their sensitivity to different pollution types, hence affecting the interpretation of outputs due to high community structure variation coupled with patchy distribution (Dallas et al., 2018).
Other indices used in bioassessment tools in East Africa include multimetric indices such as the Benthic Index of Biological Integrity (B-IBI), which has been used for assessing macroinvertebrates in Tanzania (Elias et al., 2014) and Kenyan rivers (Masese et al., 2009). Elsewhere, bioindicators have been integrated into monitoring aquatic ecosystems. Although a wide spectrum of aquatic organisms can be used as bioindicators, regional indices extensively focus on macroinvertebrates, diatoms, ciliates, and fungi. However, they are hardly identified to the lowest taxonomic level, resulting in some uncertainty and sometimes inaccuracy of the system health categorization. Therefore, this study provides the framework for future studies on the use of biomonitoring tools to support decision-making for better management and conservation of rivers, particularly in East Africa, and integrating the approach of including citizen science in ecosystem health monitoring (Chandler et al., 2017; Aura et al., 2021).
A thorough literature review was conducted to identify the advancements of biomonitoring and to establish a community-based bioassessment protocol using selected bioindicators for East African rivers. This study adopted a comprehensive literature search using various search engines, including Google Scholar, Science Direct, and Web of Science. The search was narrowed down to the use of biomonitoring, bioindicators, community-based collaboration, environmental changes, citizen science, river ecosystems management, and water quality as keywords. The inclusion criteria for peer-reviewed publications were based on relevance to the topic and publications between 2000 and 2023, although some aspects required older publications (history and evolution of biomonitoring). Publications that were not available in English were excluded. The study further employed in-depth review and analysis of information from different peer-research reports and reviewed publications. Published research articles, reports, theses, and conference proceedings were reviewed.
Biomonitoring began 350 years Before Christ (BC) when Aristotle (A Greek philosopher) found “black mud” and “red tubes” growing out of “white slime” in brooks contaminated with sewage (Moog et al., 2018). Aristotle concluded the brooks had low oxygen, evidenced by black decaying mud (caused by the sewage), a community of Beggiatoa sulfur bacteria (white slime), oligochaete sludge worms, and chironomids (red tubes). After 1800 years, Friedrich Kolenati discovered that caddis larvae were absent in a stream that had factories on the upper section. The concept of biological indicators of pollution, generally called the saprobic system, was developed around 1900 by two German scientists, namely R. Kolkwitz and M. Marsson (Persoone and De Pauw, 1979). They used benthic algae and invertebrates as bioindicators. They called organisms in clean water `Kathrobien` and those in polluted water as `Saprobien.`
After World War 11, Liebmann introduced visualization of the river’s ecological status in the saprobic system using color bands. Politicians, decision-makers, and water managers became interested in the new saprobic system. Later, in Pantle and Buck (1955) developed the saprobic system to quantify pollution (Moog et al., 2018). This index was easy for the users to interpret. It ranged from 1 (very good quality) to 4 (extremely poor quality). Around the same time, a biotic index was developed in the United States (Beck, 1954). The index was developed to quantify the extent of stream pollution and its effects on stream biota. In 1961, the concept of saprobic valences was introduced in saprobic system by Zelinka (1961). This concept reflected a 100% occurrence of taxon among four water quality classes. In 1973, Sládecek wrote a book titled “System of Water Quality from the Biological Point of View.” This book was used in Central and Eastern Europe until the saprobic system was modified again by the European Water Framework Directive 2000/60/EC (WFD) to include more water quality elements, type-specific reference conditions, and protocol to classify ecological status (Moog et al., 2018).
Several methods have been developed since the concept of biomonitoring began. From the mid to late 1980s, Rapid Bioassessment Protocols (RBPs) for macroinvertebrates, fish, and periphyton were developed. The macroinvertebrate protocol was the most commonly adopted and used due to its sedentary nature and sensitivity to a wide range of anthropogenic pressures and stressors (Wen et al., 2017; Moog et al., 2018; Ruaro et al., 2020). In South Africa, the first biotic index was developed in 1972, but it required intense labor (Chutter, 1998). The index was based on subjective scoring considering the presumed sensitivity or resistance of each taxon to impairment. South Africa Scoring System (SASS) was developed later (Dickens and Graham, 2002). Since then, other countries in Africa have developed their own biotic indices mainly by modifying SASS (Dallas, 2021). To date, there are several initiatives by countries in Africa targeting the development or modifying existing indices suitable for their river monitoring programs.
Most rivers are subjected to multiple stressors, and therefore, determination of the present ecological status by the use of bioindicators is of vital importance in guiding management and conservation decisions (Masese et al., 2023). Consequently, the development and application of bioassessment and biomonitoring techniques is an essential component of Integrated Water Resource Management (Masese et al., 2021). Integrated Water Resources Management (IWRM), as defined by the Global Water Partnership (GWP), is the process that promotes the coordinated development and management of water, land, and related resources to maximize economic and social welfare in an equitable manner without compromising the sustainability of vital ecosystems (Agarwal et al., 2000). Biomonitoring serves as a vital component of IWRM due to its provision of real-time data on aquatic ecosystem health, early detection of pollution, cost-effectiveness, and understanding of complex interactions between environmental factors and biological communities, and thus guides decision-making processes in water management (Pandey et al., 2018; Sumudumali and Jayawardana, 2021).
Maintaining or restoring aquatic ecosystem integrity guarantees that ecosystem services remain preserved, as well as maintaining the biological, physical, and chemical components of ecological integrity (Barbour et al., 2000). Most water laws and regulations, such as the Clean Water Act (CWA) of the United States of America (USA) and the Water Framework Directive (WFD) of the European Union (EU), acknowledge the interdependence of these three components and emphasize the need to combine evaluations to assess ecosystem health (Barbour and Paul, 2010). The EU’s Water Framework Directive (WFD) prioritizes the use of biotic factors, including fish, macroinvertebrates, and aquatic plants, for assessing freshwater quality in streams (Gritzalis, 2006). Many countries with aquatic ecosystem assessment programs recognize the value of biomonitoring as a tool for IWRM and have adopted a strategy that uses biological indicators as barometers for ecological conditions and trends (Jungwirth et al., 2012), as well as a scientific foundation for decision-making on aquatic resource management (Moog et al., 2018).
The CWA, USA, and WFD, EU, are the initial IWRM frameworks directly implementing extensive biomonitoring and bioassessment systems. Similar legislations do exist in other countries, for instance, South Korea (National Stream Health Monitoring Act), Australia (Sustainable Rivers Audit), New Zealand (Resource Management Act), South Africa (National Water Act), and Canada (Canada Water Act) (Masese et al., 2013). Within East Africa, Kenya’s current legislative framework for IWRM includes the Environmental Management and Coordination (Water Quality) Regulations of 2006 (Government of Kenya, 2006), which aims at the protection of water sources such as lakes, rivers, streams, springs, and wells. The Water Act of 2002 (Government of Kenya, 2002), which focuses on the management of water resources, regulates water supply and sewerage services. The Environmental Management and Coordination Act (EMCA) of 1999 (EMCA, 1999), which focuses on protecting rivers, lakes, and wetlands, mandates the National Environmental Management Authority (NEMA) regulatory role in the conservation of biological diversity. In Uganda, the Water Act of 1997, the National Water Policy of 1999, and the Uganda Law Reform Commission (2000) have been designed to “provide for use, protection and management of water resources and supply; to provide and facilitate water supply and sewerage undertakings, and manage water resources in a sustainable manner for the benefit of the people of Uganda” (Zizinga et al., 2015). Tanzania implemented a River Basin Management Approach for water resource management in the 1980s, where the nation was sub-divided into nine management regions after the amendment of the Principal Act No. 42 of 1974 (Sokile et al., 2005). The country’s first National Water Policy was adopted in 1991 as a supplement to reforms in the water sector. Currently, the Water Resources Management Act of 2022 (Government of Tanzania, 2009) provides the institutional and legal framework for the development and sustainable management of water resources to generate principles for water resources management. Although the above EA legislative frameworks do not explicitly mention biomonitoring as one of the tools to be used, they do acknowledge the importance of habitat resource quality management, which includes the physical, chemical, and biological characteristics of the water systems. Although bioassessment and biomonitoring are still in their early stages in many countries, especially in Africa, they are expanding fast as a scientific foundation for decision-making on aquatic resource management. A good example is the adoption of TARISS in the national water monitoring program in Tanzania.
Bioindicators are a group of organisms whose quantity, existence, and nature project the quality of ecological conditions (Parmar et al., 2016; Moog et al., 2018). They are fundamental mirrors of ecological impacts and are, hence, instrumental in the development of strategies for the conservation and management of ecosystems. They further noted that bioindicators have the ability to assimilate both long- and short-term fluxes in an array of ecological variables.
Many indicators, including physical, chemical, and biological indicators (bioindicators), have been used to evaluate living or non-living elements in the environment and to monitor environmental change (Bowler and Bohning-Gaese, 2017; Gustavsson et al., 2017; Areco et al., 2021; Dias et al., 2021). However, water quality approaches are useful in identifying different sources of contaminants and checking compliance with set limits. However, these methods are less effective in comparing sites impacted by different water quality (Debels et al., 2005). Furthermore, Abdelkarim (2020) reported that bioindicator species can respond to environmental changes and biological effects in a comprehensive and dynamic way, providing a basis for accurate assessment of ecological changes. Therefore, biological monitoring using the response of bioindicators provides a much easier way to measure ecological integrity due to environmental change (Dolédec and Statzner, 2010).
In the last few decades, several biological metrics and indices have been developed to assess the ecological status of riverine ecosystems using bioindicators (Table 1). Most metrics and indices have been developed based on five biological quality elements: macroinvertebrates, fishes, phytoplanktons, benthic diatoms, and macrophytes (Birk et al., 2012). These key elements are efficient in monitoring anthropogenic impairment on the lotic ecosystem because they respond to different stressors affecting water quality (Gabriel et al., 2017). Furthermore, there has been increasing use of other bioindicators in river monitoring, including fungi (Samson et al., 2020), ciliates (Kulas et al., 2021), and bacteria (Chen et al., 2022). However, the type and characteristics of the river system being investigated and the pollution source determine the choice of the key bioindicator element to be chosen for monitoring. Furthermore, other environmental factors such as temperature, light transmission, and suspended solids affect the distribution of bioindicators in ecosystems (Khatri and Tyagi, 2015) and must be taken into consideration when choosing bioindicators. Some bioindicators for assessing the ecological quality of African rivers are discussed below, and key factors are summarized in Table 2.
Macroinvertebrates, especially benthos, are the most commonly used biomonitors in freshwater ecosystems (Chadwick et al., 2012). They are significant components of aquatic food webs linking organic matter and nutrient sources (detritus, algae, and leaf litter) with higher trophic levels (Carter et al., 2017). They are mostly preferred due to their wide range of sensitivity toward various stressors, wide distribution, long lifespan with subtle life stages, and sedentary behavior, thus providing good spatial resolution (Wen et al., 2017). Previous studies have documented the use of macroinvertebrates in monitoring riverine ecosystems (Table 2). Macroinvertebrates have been used successfully to assess land-induced changes to water quality in Zimbabwe (Mwedzi et al., 2016; Chikodzi et al., 2017) stream ecosystem response to land use (agricultural and urbanization) in Burkina Faso (Kaboré et al., 2016) and Benin (Gouissi et al., 2019), among many other case studies in the literature. Within East Africa, bioindicator species (Ephemeroptera, Plecoptera, and Trichoptera) EPT index, Functional and Feeding Guild (FFG), and biodiversity have been used to classify water quality in Mount Kenya streams (Chamia and Kutuny, 2022; Benjamin et al., 2023) and western Kenya (Mzungu et al., 2022). Similar studies have been undertaken in Tanzania (Kaaya, 2014), Uganda (Turibamwe and Wangalwa, 2020), and Rwanda (Dusabe et al., 2019). In the Afrotropical biogeographical realm, land use has been identified as one of the factors that can be used to predict longitudinal changes in water quality and characteristics of macroinvertebrate assemblages in rivers (Gichana et al., 2015), together with stream order and elevation gradients (Yegon et al., 2021; Wanderi et al., 2022). Attempts by some countries to have macroinvertebrates incorporated in technical and national standards of water quality monitoring have been initiated: TARISS in Tanzania (Kaaya Lulu, personal communication, May 2023 during online teaching).
In East Africa, biotic indices have advanced by incorporating macroinvertebrates in the bioassessment of various riverine ecosystems. For example, the Tanzania River Scoring System (TARISS) (Kaaya et al., 2015) and Rwenzori Score (Musonge et al., 2020) in Tanzania and Uganda, respectively. Other indices include the Macroinvertebrates Index of Biotic Integrity (M-IBI) and the Benthic Index of Biological Integrity used to assess rivers in Kenya (Masese et al., 2023) and Tanzania (Elias et al., 2014), respectively. Nonetheless, it is important to note that the aforementioned biotic indices have gained momentum without testing or validation of their effectiveness. For instance, TARISS has been applied in Uganda (Tumusiime et al., 2019; Turibamwe and Wangalwa, 2020; Ochieng et al., 2021), Kenya (Masese et al., 2023), and Rwanda (Dusabe et al., 2019) without validation or modifications. Similarly, ETHbios and SASS5 have been used in Kenya without standardization (Masese et al., 2023). Failure to validate these tools and protocols before use would complicate universal use in novel areas where such methods do not exist or have not been tested before (Watson and Dallas, 2013). Therefore, it is important that biotic indices be tested, validated, and, if possible, modified before rolling out for ecological assessment outside its geographical and ecological origin.
However, resource limitations, including taxonomic expertise, funding, and data availability, remain critical challenges to biomonitoring advancement in the whole of Africa. Nevertheless, considerable success has been made in advancing biomonitoring approaches in the region. A good example is the development of SAAS5 in South Africa, which other countries are now increasingly adopting or modifying to develop country-based indices. Therefore, there is a need for in-depth assessment and evaluation of the existing macroinvertebrates-based indices to trigger research for the modification and development of new ones where applicable to enhance wider adoption of these novel tools.
Fish are sensitive to environmental stressors and are commonly used, especially in large rivers, to reflect human pressures and impacts (Melcher et al., 2020). Past studies have focused on fish as bioindicators due to their special biological characteristics (López-López and Sedeño-Díaz, 2015), where individual (morphological alterations; condition factor and fecundity) parameters are used to identify damage in sentinel fishes or population-level metrics (sex-ratio, size frequency distribution as mortality, and reproductive indicators) as indicators of the fish population structure (Table 2). Furthermore, they reported that several ecologically relevant responses at the community level have been used to assess fish community health. For example, environmental degradation or stress results in changes in the number of species (richness), their identity (similarity), and the relative abundance of the species within the community. Similarly, diversity indices are useful for comparing fish communities.
Within East Africa, Achieng et al. (2021), documented changes in fish species richness and composition as indicators of water quality parameters in rivers, Nzoia, Mara, Sondu-Miriu, and Nyando, and other tributaries along Lake Victoria, Kenya. Similar attempts were made in Tanzania, in Shirati Bay (Waya et al., 2014) and Nyumba ya Mungu Reservoir (Mangi et al., 2023), as well as in selected wetlands impacted by anthropogenic activities along the shores of Lake Victoria in Uganda (Naigaga et al., 2011). Fish has also been used in the bioassessment of heavy metals in various rivers in the region, including the Nairobi River, Kenya (Budambula and Mwachiro, 2006); Thigithe River, Tanzania (Mataba et al., 2016); Sogwe River, Tanzania (Mapenzi et al., 2020); and River Rwizi, Uganda (Basooma et al., 2021).
Based on fish species sensitivity, various Multimetric Indexes (MMIs) of Biotic Integrity have been developed to assess riverine health in various regions, including East Africa (Achieng et al., 2021). MMIs are effective as they can ascertain and distinguish the impacts of different stressors on ecological conditions (Lunde and Resh, 2012). Generally, species sensitivity or tolerance of fish has been used as an indicator of the health of ecosystems (Zeni et al., 2017; Brejao et al., 2018). In the past decades, species sensitivity was based on qualitative personal judgment with minimal ecological, empirical, or physiological information support (Wang et al., 2018). However, the Niche Breadth concept using multivariate approaches, including Principal Component Analysis (PCA), has enabled the evaluation of fish taxa response and ecological variability along multiple stressors gradient (Achieng et al., 2017). However, this approach is not applicable to endangered species or species that have a narrow geographical range (Segurado et al., 2011).
Knowledge of fish species’ tolerance to various environmental alarms is crucial for developing ecological indices for monitoring the state of riverine ecosystems. For example, Labeo victorianus, Amphilus jacksonii, Clarius gariepinus, Enteromius nyanzae, Enteromius apleurogramma, Enteromius cercops, Enteromius kerstenii, and Pseudocrenilabrus multicolor are some of the tolerant fish species reported in Kenyan rivers, while the intolerant species includes Mormyrus kannume, Gnathonesmus longibarbis, Barbus neumayeri, Barbus altinialis, Oreochromis variabilis, Schilbe mystus, and Bagrus docmak (Raburu and Masese, 2012; Achieng et al., 2021). Similarly, a study by Naigaga et al. (2011) in Uganda revealed that the abundance and diversity of haplochromine cichlids were greatly influenced by wetlands’ habitat structure. This infers that haplochromines could be suitable biological indicators for pollution assessment in water systems.
The Fish Index of Biotic Integrity (FIBI) has five categories with 12 metrics that give the ecological status of an ecosystem. The five categories include species richness and composition, trophic function, indicator species or guild, abundance and condition, and reproductive function. FIBI score is calculated from the sum of all the metric ratings. A site with a FIBI score of 58–60 is given an excellent status, 48–52 being good, 40–44 fair, 28–34 poor, and 12–22 very poor (Raburu and Masese, 2012). However, recent studies have found fish to be unsuitable for biomonitoring in Afrotropical high-elevation rivers due to their low diversity (Osure et al., 2022; Raburu et al., 2022). Furthermore, Masese et al. (2020) reported that fish indexes do not reflect the overall state of ecosystem health and fish structure. Despite a substantial decline in fish communities, fish indices have hardly been adopted in many countries in the world as biosystem health indicators (Kleynhans, 2007). Furthermore, there is a need to standardize most fish biotic and multimetric indices beyond regions of origin.
Diatoms are primary producers playing a significant role in biochemical cycles and aquatic food webs (Stevenson et al., 2010). Diatoms have been widely used as bio-indicators due to their short life cycles and diverse life strategies (Table 2), which respond quickly to various intensities of ecological impairments (Potapova and Charles, 2003; Debenest et al., 2013; Mbao et al., 2020). Changes in diatom community structure and biomass have been shown to reflect ecological and water quality fluctuations, consequently used to assess the chemical, physical, and biological states of ecosystem integrity (Namwaya et al., 2013; Aura et al., 2020; Mbao et al., 2022) in Kenya (Mills and Ryves, 2012; Ochieng et al., 2022), Uganda, (Oberg et al., 2012; Sekadende et al., 2021), and Tanzania. Diatom assemblages have been identified as a key indicator characteristic for inferences of ecological conditions in paleolimnological applications and monitoring programs (Juggins and Birks, 2012). Furthermore, diatoms are among the significant groups recognized by European Water Framework Directives (WFD) for the identification of rivers’ ecological integrity (European Union WFD, 2000).
Recently, there have been significant developments regarding diatom identification approaches (John, 2015; Lange-Bertalot et al., 2017; Mbao et al., 2023). Initially, morphological studies of diatoms were preferred in monitoring systems health, but today, the new technique of diatom DNA meta-barcoding is gaining preference. Advances in technology have made the taxonomy of diatoms easier through an updated global database: Diatoms of the United States 2016 (Mann, 2017), AlgaeBase (Guiry and Guiry, 2015), OMNIDIA 2017, and EDDI 2012 (Celekli et al., 2021). Furthermore, recent studies have developed genetic barcoding of epilithic diatom species (Ballesteros et al., 2020). Although DNA meta-barcoding is rapidly gaining popularity in water biomonitoring, Mbao et al. (2023) reported biases on differences between morphological and molecular-based diatom indices due to the incompleteness of the reference diatom library databases. For universal use of DNA meta-barcoding, they have recommended the use of environmental high-throughput sequencing (HTS) runs, which can easily be related to morphological observations and reference libraries. However, currently, there is no existing reference database or genetic barcoding for East African rivers. Hence, diatom taxonomy knowledge, identification experts, and appropriate high-precision laboratory equipment for identifying diatoms remain the key challenges in adopting the new technique of barcoding in the region.
Globally, many indices have been developed with reference to diatoms indicator values and trophic weights to assess the ecological status of different water bodies (Kelly et al., 2008; Lobo et al., 2015; Benito et al., 2018; Celekli et al., 2019). These indices include the Trophic Diatom Index (TDI) in England (Kelly et al., 2008), the Trophic Index of Turkey (TIT) (Celekli et al., 2019), the Eutrophication/pollution Index (EPI-D) in Italy (Dell'Uomo and Torrisi, 2011), the Diatom Ecological Quality Index (DEQI) in Mexico (Salinas-Camarillo et al., 2020), the Trophic Water Quality Index (TWQI) in Brazil (Lobo et al., 2015), and South Africa Diatom Index (Harding and Taylor, 2011).
Diatoms-based indices are regularly applied in ecological monitoring in Uganda (Mills and Ryves, 2012), Kenya (Triest et al., 2012; Aura et al., 2020), and Tanzania (Sekadende et al., 2021). However, there is no documentation of indices developed specifically for monitoring African rivers, including East African. Tomas et al. (2017) and Riato et al. (2018) cautioned against direct application of indices developed in different ecological zones. They noted these indices could produce erroneous results with regard to the state of water quality attributed to differences in ecological variation and land use change (Charles et al., 2021). Therefore, there is a need to develop an easy-to-use diatom-based index for system health monitoring in East African rivers, which can be adopted in citizen science.
Ciliates form a pivotal portion of the benthic community and microbial food web by transmitting flux of energy and carbon to lower trophic levels (Xu et al., 2016). The functional and taxonomic composition of ciliate communities is driven by ecological variables, such as water quality, hydrology, and grazing pressure (Vermaat, 2005; Kathol et al., 2011). Past studies indicated that up to 50 species of ciliates are used as bioindicators (Mandal, 2023). Their close relationship with the sediments (epibenthic) makes them ideal candidates for monitoring saprobic water quality, especially organic pollution in lotic ecosystems (Dias et al., 2021). Furthermore, they are widely distributed, have short lifespans, are very sensitive to ecological pollution, and possess a variety of trophic niches (Cabral et al., 2017). Past studies noted that ciliates should be used along with macrozoobenthos in biomonitoring (Table 2). This is because they play a pivotal role in the decomposition process via bacterial consumption and its prompt reaction to a variety of changing factors, including an increase in organic load (Tirjakova and Vdacny, 2013).
Ciliates have been used for years as bioindicators in African regions, including South Africa (Ukomadu, 2012), Egypt (Mohammed-Geba et al., 2019), and Cameroon (Acha et al., 2022). However, there is scarce information on their bioindicator use in East African rivers. Recently, there has been a global advancement in ciliates taxonomy through molecular genetic approaches (Syberg-Olsen et al., 2016; Zhao et al., 2018; Cordier et al., 2021), enhancing their use as bioindicators. Furthermore, novel approaches propose a combination of data on ciliate evolution lineages with data on their behavior, biology, ecology, and phenotypes (Becker et al., 2018; Dwyer et al., 2018). Previous studies documented possible correlations between some taxa evolution and their respective tolerance to the saprobic system (Carew et al., 2011; Gulin et al., 2022). A phylogenetic signal is a useful tool for analyzing evolutionary changes of organisms, including ciliates, whose evolution has been associated with environmental variations (Dias et al., 2021). The necessity of these advanced approaches is unquestionable for the effective use of ciliates as bioindicators, enhancing their comprehensive identification. Freshwater ciliates are also used in environmental bioremediation of heavy metals (Abraham et al., 2017) as they form an important component in water purification systems (Radhakrishnan and Jayaprakas, 2015; Debastiani et al., 2016). Although they act as powerful tools in monitoring and assessment, they are hardly integrated into water quality assessment in East African rivers (Pawlowski et al., 2018) due to a lack of expertise in their identification, similar to other bioindicators discussed.
Fungi are a group of eukaryotic organisms that include yeasts, molds, and fleshy fungi (Singara Charya, 2015). All fungi are heterotrophic, indicating that they get their energy and carbon from organic matter. The majority of fungal nutrition is saprophytic or based on the degradation of organic matter. Fungi play a significant part in the biological processes of many aquatic ecosystems, especially in wetlands and rivers, where they improve water quality. Duarte et al. (2013) noted that anthropogenic activities greatly affect fungal community structure and function in river ecosystems. This shows clearly that fungi are essential bioindicators (Table 2) due to their biological diversity, universal distribution, and high sensitivity to environmental modifications with diverse ecological roles (Warnasuriya et al., 2023). Fungi bioaccumulate various contaminants, including pesticides, heavy metals, and hydrocarbons, thus able to reflect real-time concentration in a given ecosystem (Maurya and Pachauri, 2022).
Contemporary innovations of polymerase chain reaction (PCR) and its variants, next-generation DNA sequencing transcriptome, and metagenomics technologies have opened up opportunities to detect the change in fungal communities and populations more precisely than before (Warnasuriya et al., 2023). They can be used at various scales to assess environmental health as they are powerful tools that mirror any negative or positive ecological fluctuations, their levels, and subsequent impacts. However, East African rivers are rarely used in biomonitoring (Aylagas et al., 2021) despite having a high growth rate, metabolic activity, and being very sensitive to environmental stressors (Xie et al., 2016; Li et al., 2018). However, there is a lack of sufficient reference systems and studies with regard to the use of fungi for routine biomonitoring of East African rivers.
Fecal Indicator Bacteria (FIB) is the most reliable bioassessment tool for health risks (Table 2) posed by water pathogens (Byamukama et al., 2000). They are found in the gastrointestinal tract of both warm- and cold-blooded animals and are shed in feces together with pathogens. FIB in rivers indicates the possible presence of other enteric pathogens such as Entamoeba histolytica, Vibrio cholera, Salmonella typhi, Cryptosporidium parvum, and Giardia Lamblia, among others (Hysko et al., 2010). Clostridium perfringens are the best indicators of remote or old fecal pollution. Furthermore, they indicate health risks, such as antibiotic resistance associated with the consumption of contaminated water (Omondi, 2021). The easiest way to evaluate the level of microbial water contamination is by counting colonies (Yillia et al., 2008; Gichana et al., 2014; Sila, 2019; Omondi, 2021).
The most commonly used FIB are Escherichia coli and Enterococci, which have detected recent pollution. High concentrations exceeding the international WHO recommended levels indicate that water quality is compromised (World Health Organisation, 2006). Since total coliforms represent a sum of fecal and non-fecal coliforms, their presence in water is necessarily not an indication of pollution from fecal origin. For example, in river Njoro, the concentrations of Escherichia coli and total coliforms were highest downstream with abundant informal settlements close to the river, livestock defecating in the river during watering, and effluents from nearby Nakuru City (Omondi, 2021). In Nyakach lower division and Nyogores Rivers, Kenya, high and low concentrations of coliform counts were recorded in the rainy and dry seasons, respectively (Gichana et al., 2014; Olal et al., 2014). This could be explained as a result of increased runoff and flows from catchment areas during the wet season. High concentrations of coliforms exceeding WHO standards were also reported in shallow wells in Kisumu and Mtwapa Creek, Kenya (Mutai, 2013); Nakivubo wetland, Uganda (Katukiza et al., 2014; Fuhrimann et al., 2015); and Ruvu and Pangani Estuary in Tanzania (Mwakalobo et al., 2013).
Membrane filtration (MF) and most probable number (MPN) are methods used for testing coliforms in water (APHA, 2005). MPN method is normally conducted in three steps: presumptive test, confirmed test, and completed test, and coliform numbers are estimated as MPN per 100 mL from McCrady’s statistical tables. MPN is suitable for highly turbid waters, but serial dilutions must be undertaken for better results and precision. MF method, whose analysis is based on the use of a suitable culture medium, followed by counting of colonies, is more sensitive, reliable, and quantitative than MPN. However, the time-consuming enumeration process coupled with expensive and not easily available materials (medium, sterile gridded membrane filters, filtration unit and pump, and colony counter) makes its usability in general water quality monitoring limited, particularly in East Africa.
Globally, water policy reforms have been enacted in many countries. East African countries, including Kenya, instituted major reforms in the water sector, enabling the enactment of The Water Act (2002), which resulted in the establishment of various WRUAs. Thereafter, the Integrated Water Resource Management (IWRM) framework advocates for water resource management from the highest to the lowest level of governance (Baldwin et al., 2015). Furthermore, the enactment of the Water Act of 2002, the Kenyan Constitution of 2010, and the revised The Water Act, 2016 acknowledges the role of WRUAs in resource management at the grass root level (The Water Act, 2016). In Tanzania, the Water Management Resource Act of 2009 permits WUA operations on the basis of fulfilling IWRM pillars. WRUAs were established in all catchment areas within Kenya (The Water Act, 2016) and Tanzania (Richards, 2019). However, Kenya WRA’s (2015) performance report indicated that only 56% of anticipated WRUAs had established their operational plans. This calls for relevant stakeholders to support Water Resource User’s activities through funding and technical advice.
Over time, WRUAs have transitioned toward the realization of the association’s status through registration under the Societies Act, with the support of World Wildlife Funds (WWF), for example, Mara WRUAs covering the entire Mara basin. However, both WRUAs and WUAs have faced a myriad of interlinked challenges, including the inability to bring members together and diverse ecological characteristics between upper and lower catchments, which have made it difficult to achieve their set objectives. In Kenya, this resulted in creating partnerships and collaboration with different allies, including WWF and Water Resource Authority (WRA), which has led to the recognition of many WRUAs along major tributaries: transboundary River Mara in Kenya (upstream) and Tanzania (downstream). In Tanzania, WUAs have collaborated with the Tanzania Forest Service Agency (TFSA), the Africa Wildlife Foundation (AWF), and NGOs toward mitigation and restoration strategies for water resources (Ngonyani and Mourad, 2019). In Kenya, the amendment of The Water Act (2002) provides a clear guideline on coverage area, resulting in the disintegration of six existing WRUAs into 25 smaller units to conform to the water quality regulation (Richards, 2018). Furthermore, the WWF led the communities in the implementation of activities that generated alternative livelihood and better land use practices toward better management of water resources.
In Uganda, the Water Act (1999), which is the principal law regulating the water sector, adopted decentralization of decision-making, responsibilities, and implementation of the function, leading to the adoption of demand-driven approaches in the water sector (Naiga and Penker, 2014). Local Government Act (1997) empowers local government to provide safe water using mobilized and grant resources GoU, (1999). Furthermore, the Water User Committee (WUC) is the executive organ of the water user group responsible for maintaining, operating, and managing water resources (Water Aid, 2013). However, WUC faces a number of challenges, including a lack of participation from the community, inadequate resources, and poor management (Terry et al., 2015). As a result, the National Association of Professional Environmentalists (NAPEs) conducted a series of workshops to evaluate, monitor, and improve WUC functions. User-friendly handbooks were developed to permit community users to learn about their rights and responsibilities toward the proper functioning of WUCs (Terry et al., 2015). Thus, more requisite institutional structures and collaboration with other various agencies are critical for the effective management and conservation of water resources by communities in East Africa; a key focus of this study to advise on easier ways to strengthen impactful community water quality monitoring.
A variety of factors influence the selection of an ecosystem assessment and monitoring program, including research costs, human resources, and data requirements (Norris and Hawkins, 2000). Biomonitoring of aquatic ecosystems in African systems lags behind other regions due to a lack of financial commitment, technical capacity, and biomonitoring guides. Despite these challenges, there has been a development of several regional or country-specific indices (e.g., Dickens and Graham, 2002; Aschalew and Moog, 2015; Kaaya et al., 2015). The majority of these indices and programs are based on physical and chemical parameters and biological communities (Aura et al., 2020), which have been adopted especially in monitoring East African rivers by scientists. Nevertheless, these programs are limited in the quantity of data collected across large spatial and temporal scales (Achieng et al., 2020). Due to the limitations of these indices, researchers have come up with new approaches to river health monitoring, and citizen science has emerged as one of the methods (Chandler et al., 2017). At the same time, citizen science offers numerous advantages; in most cases, it is highly content-specific, which could come with limitations, especially on the legibility and reliability of the data collected. Therefore, citizen science should not replace traditional biomonitoring approaches to rivers but complement the scientific monitoring systems.
Citizen science involves community members voluntarily participating in the provision of information or data collection in accordance with a protocol provided, developed, and validated by experts (Aura et al., 2020). This is consistent with co-management approaches, which provide stakeholders with a platform for long-term management practices of aquatic resources (Obiero et al., 2015). The citizen science monitoring approach has greatly enhanced the inclusion of citizens in natural resources stewardship monitoring (Mochizuki and Yarime, 2016). Nevertheless, the development of citizen science as a natural resource assessment and monitoring approach is quite limited in Africa, and in most cases, it is in its nascent state (Requier et al., 2020). Citizen science has the potential to directly connect scientists to the public and share the significance of their research (Crocker et al., 2019), enhancing public participation in scientific research.
There is a paucity of information on the application of citizen science in environmental monitoring, especially in many African countries (Requier et al., 2020). Nevertheless, Aura et al. (2020) tried to address the need for its application where they developed a “Citizen Index of Ecological Integrity (CIEI)” for assessing and monitoring the ecological status of African riverine ecosystems in Lake Victoria. Furthermore, Njue et al. (2021) used a contributory citizen science approach to determine the spatiotemporal turbidity and suspended sediment dynamics in the Sondu-Miriu river basin, western Kenya. This implies that citizen science could be strategically the voice of the future to produce pertinent management-oriented knowledge and create collaborations among the stakeholders to fill the data and information gaps at a reasonable cost.
Citizen science in many African countries is a new phenomenon and is worse off in East Africa despite having been well-established in other countries. The following review discusses successful citizen monitoring initiatives both in Africa and other countries. One of the best examples of citizen science monitoring in Africa is Burkina Faso (West Africa), where fishermen were involved in fish sampling under the Sustainable Management of Water and Fish Resources (SUSFISH) project. The goal of the project was to strengthen capacities for science, policy, and practice and establish a basis for water quality and sustainable fisheries in Burkina Faso. Fishermen took an active role in the project by informing scientists where to sample and how to perform traditional cast net fishing. Fish sorting and identification (up to species level) were done in the field. Fish that could not be identified at the species level were preserved in 70% alcohol and taken to the laboratory for identification by professionals (Melcher et al., 2020).
Mini Stream Assessment Scoring System (miniSASS) was developed in South Africa for citizen scientists to assess river health in South Africa (Dickens and Graham, 2002; Graham et al., 2004). The miniSASS was developed from SASS, which required users to have the ability to identify at least 90 macroinvertebrate families. A statistical evaluation proved that miniSASS and SASS gave similar results (Graham et al., 2004). It involved sampling macroinvertebrates in a river, identifying them in the field in broad categories, and then calculating the river health index. However, the volunteers use a dichotomous key, which is available only on the miniSASS website, and record results obtained on an online portal. Although a large amount of data can be generated from citizen scientists, the validity of this data may be a concern depending on the level of training the volunteers have been exposed to. MiniSASS has been applied in monitoring aquatic resources in Kenya, especially the ‘Adopt-a-River Initiative’ project, which exemplifies how key curricula components can be used to solve real challenges (Waswala et al., 2019). In this project, the public, especially the youth, are mobilized to manage the aquatic resources around them through the identification of polluted sites in the Nairobi River Catchment, with the aim of enhancing environmental regulations to ensure ecosystem health.
Elsewhere, several examples of successful citizen science monitoring do exist. The Anglers` Riverfly Monitoring Initiative (ARMI) is a citizen science project for water quality assessment in the United Kingdom (UK) (Brooks et al., 2019). Volunteers sample macroinvertebrates at each site once a month by kicking (for 3 min) habitats present at a site using a sampling net (250-mm frame and 500-mm deep net bag with 1-mm mesh). Large stones from the river bed are brushed in the mouth of the net to dislodge any organisms that have not been collected in the sampling net. Contents of the sampling net are emptied in a sorting tray, and macroinvertebrate taxa are identified and estimated to log10 scale abundance. The macroinvertebrate taxa used as bioindicators the following: cased Trichoptera, caseless Trichoptera (caddisfy), Ephemeridae (mayfly), Ephemerellidae (blue-winged olive), Baetidae (olives), Heptageniidae (fat-bodied mayfly), Plecoptera (stonefly), and Gammaridae (shrimp). The choice of the taxa is based on ease of identification, range sensitivity to pollution, national applicability, present year-round (with the exception of Ephemerellidae), and is familiar to most anglers. A score is allocated to the log10 abundance category for each taxon, and the scores of all the target groups are added. Volunteers are trained at a 1-day workshop before participating in the ARMI monitoring program. River coordinators for each catchment are in charge of organizing volunteers and uploading data onto the national database held at the Freshwater Biological Association, although some choose not to upload their data.
In Spain, RiuNet1 was initiated by the Ecology Department of the University of Barcelona. Volunteers assess river water quality with simplified protocols available in the RiuNet handbook2. Macroinvertebrates are identified at the family level using pictures or dichotomous keys available on the RiuNet app. Volunteers identify insects (Diptera, Coleoptera, Odonata, Heteroptera, Trichoptera, Ephemeroptera, and Plecoptera) and taxa from the Mollusca, Oligochaeta, Hirudinea, and Crustacea. Volunteers sample each site twice every year, in spring and autumn. The RiuNet app automatically calculates a simplified biological quality index. The RiuNet project does not have a training program for volunteers. However, citizen scientists learn from the RiuNet app, which guides them on how to assess the ecological status of a river.
Save-our-Streams (SOS) program is a citizen science monitoring program in the USA. The SOS program mainly focused on observations of water odor, color, and the presence of trash in streams. Macroinvertebrates were added to the monitoring program later on with the aim of assessing stream degradation (Engel, 2000). Virginia SOS sampling protocol has three kick net samples. A kick net of 1,500 μm mesh size is held in a rifle by a volunteer, and an area of 1m2 is kicked in front of the net. The net is taken to the stream shore, where sorting and identification of macroinvertebrates are done based on previous training and simple pictures. Identification is mostly at the order level and, in some cases, at the family level. Data from each kick net sample is recorded in an assessment sheet, and then the SOS water quality rating score is calculated (each kick net sample is scored individually). Volunteers must undergo training and certification processes to participate in the monitoring program. The Department of Environmental Quality in Virginia signed a memorandum of agreement with the Virginia SOS program to use their data but with a quality control plan.
In river catchment management, local communities are key stakeholders, and their participation has been agreed upon as an important element in delivering water-related decisions (Rolston et al., 2017). However, sometimes bottom-up, community-led, catchment-based approaches to land and water management tend to provide new challenges to policymakers (Gurney et al., 2016) since community priorities may vary according to local values and pressures (Rolston et al., 2017). To advance citizen science in river water quality monitoring as a system health indicator, cheap, simple, easy-to-use approaches using the most common bioindicators are required and proposed in the following section.
From the review above, macroinvertebrates have the potential to be used as bioindicators by local riparian communities. Their use requires simple training and easy identification equipment coupled with simple sampling procedures, which can use a wide range of easily available materials: normal household sieves, sweep nets, mosquito nets, toothbrushes for scrubbing stones, buckets, and trays, among others. Therefore, based on this review, an intolerance/tolerance macroinvertebrate metric that includes numbers of pollution tolerant and intolerant groups (taxa) (Table 3) is recommended as an applicable tool for citizen science monitoring of water quality in rivers. With basic training and the provision of simple photographic chats, communities can easily identify key macroinvertebrates, either the intolerant, mayflies, stoneflies, and caddisflies whose scientific names are Ephemeroptera, Plecoptera, and Trichoptera (EPT), respectively, or the tolerant families comprising true flies and worms, scientifically referred to as Diptera and Oligochaeta, respectively. The communities using the pictorial chart (Plate 1) can easily relate these organisms to their local names. Nearly all families in the EPT group are sensitive to pollution. The numbers of each group of macroinvertebrates indicate the water quality and the system’s ecological quality. For example, the EPT group is higher than Diptera and Oligochaeta at pristine or minimally disturbed sites and vice versa.
Table 3. River health assessment sheet with selected macroinvertebrate taxa for easy use in citizen science.
PLATE 1. A user-friendly pictorial macroinvertebrate identification key in relation to water quality, suitable for citizen scientists (Photos by Priscilla Wangari and others adopted from Gerber and Gabriel, 2002).
The community should be able to select a sampling site within the river. The site should have different habitats, including areas that are shallow with fast-flowing water (riffle habitat) and a deep, slow-flowing water section (pool habitat). Macroinvertebrates are collected from the selected habitat outlined above using a kick net of approximately 500 μm mesh size. Stones, bedrock, or underlying layer (benthic substrate) in the habitats are sampled by kicking for approximately 30 s to dislodge the invertebrates, which are collected in the net (kick net). The contents of the net are then transferred in a white tray or basin by inverting the net, such that the mouth is placed on the white tray and flushed with water to ensure that macroinvertebrates do not remain in it. In case a kick net is not available, stones or the benthic substrate are picked randomly at the chosen habitat for 1 min. The stones and any other substrate collected are placed on a white tray or basin and brushed on all sides to dislodge attached organisms. The collected macroinvertebrates are identified using the provided simplified colored identification keys for the selected order EPT, Diptera, and Oligocheta (Plate 1) and counted in the field.
However, the community volunteers may not have the taxonomic experience to identify the invertebrates at much lower taxonomic levels. Thus, to avoid data inaccuracy, macroinvertebrates are identified at the order level for EPT and Diptera and subclass level for Oligochaeta. After counting, the sample is poured back into the stream. The counted results are interpreted in terms of water quality and ecological status of the system. For example, a site with good ecological status has a higher number of EPT than Diptera and Oligochaeta (Table 3).
It is important for the community volunteers to understand that some families in the EPT group (Caenidae, Hydropsychidae, and Baetidae) can tolerate pollution and thus affect the performance of the EPT metric (Masese and Raburu, 2017). In situations where EPT does not give a conclusive ecological status of a site, an experienced macroinvertebrate taxonomist is sought to give the best professional judgment.
Although fish are sensitive to pressures, they have low diversity in high-elevation rivers (Osure et al., 2022; Raburu et al., 2022; Schmidt et al., 2022). Therefore, community-based biomonitoring using fish may be suitable in lowland rivers.
Fish sampling is undertaken using traditional cast-net fishing by community volunteers with assistance from professional fishermen. A cast net with 10 mm mesh size and 4.3 m diameter or 25 mm mesh size and 4.5 m diameter can be used depending on availability (Melcher et al., 2020). The cast net is thrown into the river, retrieved, and the contents are emptied into a bucket. The number of throws is recorded for easy comparison of results. At sites with deep waters, where wading is impossible, canoes can be used for navigation (Melcher et al., 2020). The fish collected are identified in the field to genus level, counted, and the live ones returned to the water. A river with good ecological status should have a higher number of intolerant fish than tolerant fish, as shown in Table 4.
After filling in the assessment sheet, a photograph of the assessment sheet is taken by the volunteer and uploaded on Google Drive or an easy-to-use APP developed for the data management of river water quality and as a backup. The drive or APP used should be easily accessible to volunteers, identified professional macroinvertebrates, fish taxonomists, and government officials (catchment water manager, county government official) to provide the basis for the development of management strategies. Hard copies of assessment sheets should be kept safely by the chairperson or a member of WRUA/WUA or its equivalent for future reference.
Macroinvertebrates and fish are useful bioindicators of river health. The East African countries should embrace this approach as a block for easy comparison of the ecological status of rivers within the region, including the transboundary systems. The countries lagging behind, Kenya and Uganda, should develop their own indices and assessment tools using foundations from the already developed regional indices, such as TARISS from Tanzania. Enhancing citizen science in system health monitoring is the future management strategy for raising awareness, bridging the gap between users, policymakers, and scientific findings, and interpreting social-ecological information. This review advocates for the use of a community-based biomonitoring approach using macroinvertebrates and fish.
NK: Conceptualization, Data curation, Formal analysis, Investigation, Supervision, Validation, Visualization, Writing – review & editing. LO: Data curation, Formal analysis, Investigation, Writing – review & editing. PM: Data curation, Formal analysis, Investigation, Writing – review & editing. AB: Data curation, Formal analysis, Validation, Writing – review & editing. AM: Data curation, Formal analysis, Validation, Writing – review & editing. GS: Validation, Writing – review & editing.
The author(s) declare that no financial support was received for the research, authorship, and/or publication of this article.
The authors are thankful to the Strengthening Community-based Research for River Health and Climate Change Mitigation in Eastern Africa (STREM) Project funded by Africa Uni-Net for providing the necessary infrastructure to complete this study.
The authors declare that the research was conducted in the absence of any commercial or financial relationships that could be construed as a potential conflict of interest.
All claims expressed in this article are solely those of the authors and do not necessarily represent those of their affiliated organizations, or those of the publisher, the editors and the reviewers. Any product that may be evaluated in this article, or claim that may be made by its manufacturer, is not guaranteed or endorsed by the publisher.
Abdelkarim, M. S. (2020). Biomonitoring and bioassessment of running water quality in developing countries: a case study from Egypt. Egypt. J. Aquatic Res. 46, 313–324. doi: 10.1016/j.ejar.2020.11.003
Abraham, J. S., Sripoorna, S., Choudhary, A., Toteja, R., Gupta, R., Makhija, S., et al. (2017). Assessment of heavy metal toxicity in four species of freshwater ciliates (Spirotrichea: Ciliophora) from Delhi, India. Curr. Sci. 113, 2141–2150. doi: 10.18520/cs/v113/i11/2141-2150
Acha, A. Y., William, A. M., and Gideon, A. A. (2022). Use of ciliated protozoans in the saprobic evaluation of hydrosystems in an equatorial zone (south region of Cameroon). Afr. J. Environ. Sci. Technol. 16, 295–310. doi: 10.5897/AJEST2022.3117
Achieng, A. O., Raburu, P. O., Kipkorir, E. C., Ngodhe, S. O., Obiero, K. O., and Ani-Sabwa, J. (2017). Assessment of water quality using multivariate techniques in River Sosiani, Kenya. Environ. Monit. Assess 189:280. doi: 10.1007/s10661-017-5992-5
Achieng, A. O., Masese, F. O., and Kaunda-Arara, B. (2020). Fish assemblages and size-spectra variation among rivers of Lake Victoria Basin, Kenya. Ecological Indicators 118:106745. doi: 10.1016/j.ecolind.2020.106745
Achieng, A. O., Masese, F. O., Coffey, T. J., Raburu, P. O., Agembe, S. W., Febria, C. M., et al. (2021). Assessment of the ecological health of Afrotropical rivers using fish assemblages: a case study of selected rivers in the Lake Victoria Basin, Kenya. Front. Water 2:620704. doi: 10.3389/frwa.2020.620704
Agarwal, A., de los Angeles, M. S., Bhatia, R., Chéret, I., Davila-Poblete, S., Falkenmark, M., et al. (2000). Integrated water resources management. Stockholm: Global water Partnership, 1–67.
Allouche, J. (2016). The birth and spread of IWRM-A case study of global policy diffusion and translation. Water Alternatives 9, 412–433.
APHA (2005). Standard methods for the examination of water and wastewater. 21st Edn. Washington, DC: American Public Health Association.
Areco, M. M., Salomone, V. N., and dos Santos Afonso, M. (2021). Ulva lactuca: a bioindicator for anthropogenic contamination and its environmental remediation capacity. Mar. Environ. Res. 171:105468. doi: 10.1016/j.marenvres.2021.105468
Aschalew, L., and Moog, O. (2015). Benthic macroinvertebrates based new biotic score “ETHbios” for assessing ecological conditions of highland streams and rivers in Ethiopia. Limnologica 52, 11–19. doi: 10.1016/j.limno.2015.02.002
Aura, C. M., Nyamweya, C. S., Owiti, H., Odoli, C., Musa, S., Njiru, J. M., et al. (2021). Citizen science for bio-indication: development of a community-based index of ecosystem integrity for assessing the status of afrotropical riverine ecosystems. Front. Water 2:609215. doi: 10.3389/frwa.2020.609215
Aura, C. M., Odoli, C., Nyamweya, C. S., Njiru, J. M., Musa, S., Miruka, J. B., et al. (2020). Application of phytoplankton community structure for ranking the major riverine catchments influencing the pollution status of a lake basin. Lakes Reserv. Res. Manag. 25, 3–17. doi: 10.1111/lre.12307
Aylagas, E., Atalah, J., Sanchez-Jerez, P., Pearman, J. K., Casado, N., Asensi, J., et al. (2021). A step towards the validation of bacteria biotic indices using DNA metabarcoding for benthic monitoring. Mol. Ecol. Resour. 21, 1889–1903. doi: 10.1111/1755-0998.13395
Baldwin, E., Washington-Ottombre, C., Dell'Angelo, J., Cole, D., and Evans, T. (2015). Polycentric governance and irrigation reform in Kenya. Governance 29, 207–225. doi: 10.1111/gove.12160
Ballesteros, I., Castillejo, P., Haro, A. P., Montes, C. C., Heinrich, C., and Lobo, E. A. (2020). Genetic barcoding of Ecuadorian epilithic diatom species suitable as water quality bioindicators. C. R. Biol. 343, 41–52. doi: 10.5802/crbiol.2
Barbour, M. T., and Paul, M. J. (2010). Adding value to water resource management through biological assessment of rivers. Hydrobiologia 651, 17–24. doi: 10.1007/s10750-010-0287-7
Barbour, M. T., Swietlik, W. F., Jackson, S. K., Courtemanch, D. L., Davies, S. P., and Yoder, C. O. (2000). Measuring the attainment of biological integrity in the USA: a critical element of ecological integrity. Hydrobiologia 422, 453–464. doi: 10.1023/A:1017095003609
Basooma, A., Teunen, L., Semwanga, N., and Bervoets, L. (2021). Trace metal concentrations in the abiotic and biotic components of river Rwizi ecosystem in western Uganda, and the risks to human health. Heliyon 7:e08327. doi: 10.1016/j.heliyon.2021.e08327
Beck, W. M. (1954). Studies in stream pollution biology: I. A simplified ecological classification of organisms. Q. J. Florida Acad. Sci. 17, 211–227.
Becker, D. J., Chumchal, M. M., Broders, H. G., Korstian, J. M., Clare, E. L., Rainwater, T. R., et al. (2018). Mercury bioaccumulation in bats reflects dietary connectivity to aquatic food webs. Environ. Pollut. 233, 1076–1085. doi: 10.1016/j.envpol.2017.10.010
Beentjes, K. K., Speksnijder, A. G., Schilthuizen, M., Hoogeveen, M., Pastoor, R., and van der Hoorn, B. B. (2019). Increased performance of DNA metabarcoding of macroinvertebrates by taxonomic sorting. PLoS One 14:e0226527. doi: 10.1371/journal.pone.0226527
Benito, X., Fritz, S. C., Steinitz-Kannan, M., Tapia, P. M., Kelly, M. A., and Lowell, T. V. (2018). Geo-climatic factors drive diatom community distribution in tropical south American freshwaters. J. Ecol. 106, 1660–1672. doi: 10.1111/1365-2745.12934
Benjamin, J. M., Abuya, D., Omollo, B., and M’Eerimba, C. (2023). Longitudinal patterns of abundance, diversity and functional feeding guilds of benthic communities in east African tropical high-altitude streams. Afr. J. Ecol. 61, 781–793. doi: 10.1111/aje.13177
Bere, T., and Nyamupingidza, B. B. (2014). Use of biological monitoring tools beyond their country of origin: a case study of the south African scoring system version 5 (SASS5). Hydrobiologia 722, 223–232. doi: 10.1007/s10750-013-1702-7
Birk, S., Bonne, W., Borja, A., Brucet, S., Courrat, A., Poikane, S., et al. (2012). Three hundred ways to assess Europe's surface waters: an almost complete overview of biological methods to implement the water framework directive. Ecol. Indic. 18, 31–41. doi: 10.1016/j.ecolind.2011.10.009
Bowler, D., and Bohning-Gaese, K. (2017). Improving the community-temperature index as a climate change indicator. PLoS One 12:e0184275. doi: 10.1371/journal.pone.0184275
Brejao, G. L., Hoeinghaus, D. J., Perez-Mayorga, M. A., Ferraz, S. F., and Casatti, L. (2018). Threshold responses of Amazonian stream fishes to timing and extent of deforestation. Conserv. Biol. 32, 860–871. doi: 10.1111/cobi.13061
Brooks, S. J., Fitch, B., Davy-Bowker, J., and Codesal, S. A. (2019). Anglers’ riverfly monitoring initiative (ARMI): a UK-wide citizen science project for water quality assessment. Freshwater Sci. 38, 270–280. doi: 10.1086/703397
Budambula, N. L. M., and Mwachiro, E. C. (2006). Metal status of Nairobi River waters and their bioaccumulation in Labeo cylindricus. Water Air Soil Pollut. 169, 275–291. doi: 10.1007/s11270-006-2294-x
Byamukama, D., Kansiime, F., Mach, R. L., and Farnleitner, A. H. (2000). Determination of Escherichia coli contamination with chromocult coliform agar showed a high level of discrimination efficiency for differing fecal pollution levels in tropical waters of Kampala, Uganda. Appl. Environ. Microbiol. 66, 864–868. doi: 10.1128/AEM.66.2.864-868.2000
Cabral, A. F., Buosi, P. R. B., Segóvia, B. T., Velho, L. F. M., and Bini, L. M. (2017). Taxonomic sufficiency in detecting hydrological changes and reproducing ordination patterns: a test using planktonic ciliates. Ecol. Indic. 82, 227–232. doi: 10.1016/j.ecolind.2017.07.008
Carew, M. E., Miller, A. D., and Hoffmann, A. A. (2011). Phylogenetic signals and ecotoxicological responses: potential implications for aquatic biomonitoring. Ecotoxicology 20, 595–606. doi: 10.1007/s10646-011-0615-3
Carter, J. L., Resh, V. H., and Hannaford, M. J. (2017). “Macroinvertebrates as biotic indicators of environmental quality” in Methods in stream ecology. (Elsevier: Amsterdam, The Netherland Academic Press), 293–318. doi: 10.1016/b978-0-12-813047-6.00016-4
Celekli, A., Lekesiz, H., and Yavuzatmaca, M. (2021). Bioassessment of water quality of surface waters using diatom metrics. Turk. J. Bot. 45, 379–396. doi: 10.3906/bot-2101-16
Celekli, A., Toudjani, A. A., Gumuş, E. Y., Kayhan, S., and Lekesiz, H. O. (2019). Determination of trophic weight and indicator values of diatoms in Turkish running waters for water quality assessment. Turk. J. Bot. 43, 90–101. doi: 10.3906/bot-1704-40
Chadwick, M. A., Thiele, J. E., Huryn, A. D., Benke, A. C., and Dobberfuhl, D. R. (2012). Effects of urbanization on macroinvertebrates in tributaries of the St. Johns River, Florida, USA. Urban Ecosyst. 15, 347–365. doi: 10.1007/s11252-011-0217-0
Chamia, L. K., and Kutuny, G. K. (2022). Assessment of benthic macroinvertebrates as bioindicators of water quality in river Naka, Chuka, Tharaka-Nithi, Kenya. J. Environ. Sustain. Advanc. Res. 8, 1–9.
Chandler, M., See, L., Copas, K., Bonde, A. M., Lopez, B. C., and Danielsen, F. (2017). Contribution of citizen science towards international biodiversity monitoring. Biol. Conserv. 213, 280–294. doi: 10.1016/j.biocon.2016.09.004
Charles, D. F., Kelly, M. G., Stevenson, R. J., Poikane, S., and Theroux, S. (2021). Benthic algae assessments in the EU and the US: striving for consistency in the face of great ecological diversity. Ecol. Indic. 121:107082. doi: 10.1016/j.ecolind.2020.107082
Chen, Z., Zhong, X., Zheng, M., Liu, W. S., Fei, Y., Ding, K., et al. (2022). Indicator species drive the key ecological functions of microbiota in a river impacted by acid mine drainage generated by rare earth elements mining in South China. Environ. Microbiol. 24, 919–937. doi: 10.1111/1462-2920.15501
Chikodzi, D., Mabhegedhe, M., and Tunha, T. K. (2017). Biomonitoring of Mucheke and Shagashe Rivers in Masvingo, Zimbabwe using macro-invertebrates as indicators of water quality. J. Geosci. Environ. Protect. 5, 221–237. doi: 10.4236/gep.2017.59016
Chutter, F. M. (1998). Research on the rapid biological assessment of water quality impacts in streams and Rivers. WRC Report No. 422/1/98. Water Research Commission, Pretoria, South Africa
Conrad, C. C., and Hilchey, K. G. (2011). A review of citizen science and community-based environmental monitoring: issues and opportunities. Environ. Monit. Assess. 176, 273–291. doi: 10.1007/s10661-010-1582-5
Cordier, T., Alonso-Saez, L., Apothéloz-Perret-Gentil, L., Aylagas, E., Bohan, D. A., Bouchez, A., et al. (2021). Ecosystems monitoring powered by environmental genomics: a review of current strategies with an implementation roadmap. Mol. Ecol. 30, 2937–2958. doi: 10.1111/mec.15472
Crocker, E., Condon, B., Almsaeed, A., Jarret, B., Nelson, C. D., Abbott, A. G., et al. (2019). TreeSnap: A citizen science app connecting tree enthusiasts and forest scientists. Plants People Planet 2, 47–52. doi: 10.1002/ppp3.41
Dahm, V., Hering, D., Nemitz, D., Graf, W., Schmidt-Kloiber, A., Leitner, P., et al. (2013). Effects of physico-chemistry, land use and hydromorphology on three riverine organism groups: a comparative analysis with monitoring data from Germany and Austria. Hydrobiologia 704, 389–415. doi: 10.1007/s10750-012-1431-3
Dallas, H. F. (2021). Rapid bioassessment protocols using aquatic macroinvertebrates in Africa–considerations for regional adaptation of existing biotic indices. Front. Water 3:628227. doi: 10.3389/frwa.2021.628227
Dallas, H. F., Lowe, S., Kennedy, M. P., Saili, K., and Murphy, K. J. (2018). Zambian invertebrate scoring system (ZISS): a macroinvertebrate-based biotic index for rapid bioassessment of southern tropical African river systems. Afr. J. Aquat. Sci. 43, 325–344. doi: 10.2989/16085914.2018.1517081
Dallas, H., and Mosepele, B. (2020). Spatial variability of aquatic macroinvertebrate assemblages in the Okavango Delta, Botswana: considerations for developing a rapid bioassessment tool. Afr. J. Aquat. Sci. 45, 350–363. doi: 10.2989/16085914.2019.1704215
Dalu, T., Froneman, P. W., and Bere, T. (2016). Assessment of water quality based on diatom indices in a small temperate river system, Kowie River, South Africa. Water SA 42, 183–193. doi: 10.4314/wsa.v42i2.02
Debastiani, C., Meira, B. R., Lansac-Tôha, F. M., Velho, L. F. M., and Lansac-Tôha, F. A. (2016). Protozoa ciliates community structure in urban streams and their environmental use as indicators. Braz. J. Biol. 76, 1043–1053. doi: 10.1590/1519-6984.08615
Debels, P., Figueroa, R., Urrutia, R., Barra, R., and Niell, X. (2005). Evaluation of water quality in the Chillán River (Central Chile) using physicochemical parameters and a modified water quality index. Environ. Monit. Assess. 110, 301–322. doi: 10.1007/s10661-005-8064-1
Debenest, T., Silvestrea, J., and Pinelli, E. (2013). Diatoms in ecotoxicology. Encycl. Aquatic Ecotoxicol., 1, 295–304. doi: 10.1007/978-94-007-5704-2_29
Dell'Uomo, A., and Torrisi, M. (2011). The eutrophication/pollution index-diatom based (EPI-D) and three new related indices for monitoring rivers: the case study of the river Potenza (the marches, Italy). Plant Biosyst. 145, 331–341. doi: 10.1080/11263504.2011.569347
Dias, R. J. P., de Souza, P. M., Rossi, M. F., Wieloch, A. H., da Silva-Neto, I. D., and D’Agosto, M. (2021). Ciliates as bioindicators of water quality: a case study in the neotropical region and evidence of phylogenetic signals (18S-rDNA). Environ. Pollut. 268:115760. doi: 10.1016/j.envpol.2020.115760
Dickens, C. W., and Graham, P. M. (2002). The south African scoring system (SASS) version 5 rapid bioassessment method for rivers. Afr. J. Aquat. Sci. 27, 1–10. doi: 10.2989/16085914.2002.9626569
Dolédec, S., and Statzner, B. (2010). Responses of freshwater biota to human disturbances: contribution of J-NABS to developments in ecological integrity assessments. J. N. Am. Benthol. Soc. 29, 286–311. doi: 10.1899/08-090.1
Duarte, S., Fernandes, I., Nogueira, M. J., Cássio, F., and Pascoal, C. (2013). Temperature alters interspecific relationships among aquatic fungi. Fungal Ecol. 6, 187–191. doi: 10.1016/j.funeco.2013.02.001
Dusabe, M. C., Wronski, T., Gomes-Silva, G., Plath, M., Albrecht, C., and Apio, A. (2019). Biological water quality assessment in the degraded Mutara rangelands, northeastern Rwanda. Environ. Monit. Assess. 191, 139–113. doi: 10.1007/s10661-019-7226-5
Dwyer, G. K., Stoffels, R. J., Rees, G. N., Shackleton, M. E., and Silvester, E. (2018). A predicted change in the amino acid landscapes available to freshwater carnivores. Freshwater Sci. 37, 108–120. doi: 10.1086/696128
Edegbene, A. O., Akamagwuna, F. C., Odume, O. N., Arimoro, F. O., Edegbene Ovie, T. T., Akumabor, E. C., et al. (2022). A macroinvertebrate-based multimetric index for assessing ecological condition of forested stream sites draining Nigerian urbanizing landscapes. Sustain. For. 14:11289. doi: 10.3390/su141811289
Edegbene, A. O., Arimoro, F. O., and Odume, O. N. (2019). Developing and applying a macroinvertebrate-based multimetric index for urban rivers in the Niger Delta, Nigeria. Ecol. Evol. 9, 12869–12885. doi: 10.1002/ece3.5769
Elias, J. D., Ijumba, J. N., Mgaya, Y. D., and Mamboya, F. A. (2014). Study on freshwater macroinvertebrates of some Tanzanian rivers as a basis for developing biomonitoring index for assessing pollution in tropical African regions. J. Ecosyst. 2014, 1–8. doi: 10.1155/2014/985389
EMCA (1999). The environmental management and coordination act no.8 of 1999 Kenya gazette supplement. Nairobi, Kenya: Government Printers.
Engel, S. R. (2000). The effectiveness of using volunteers for biological monitoring of streams. Master’s thesis. Blacksburg: Virginia, USA, Virginia Tech.
European Union (2000). Directive 2000/60/EC of the European Parliament and of the Council for Community Action in the Field of Water Policy. Available at: https://eur-lex.europa.eu/legal-content/en/ALL/?uri=CELEX%3A32000L0060 (Accessed October 18, 2023)
Feld, C. K., Birk, S., Bradley, D. C., Hering, D., Kail, J., Marzin, A., et al. (2011). From natural to degraded rivers and back again: a test of restoration ecology theory and practice. Adv. Ecol. Res. 44, 119–209. doi: 10.1016/B978-0-12-374794-5.00003-1
Ferreira, V., Elosegi, A., Tiegs, S. D., von Schiller, D., and Young, R. (2020). Organic matter decomposition and ecosystem metabolism as tools to assess the functional integrity of streams and rivers–a systematic review. Water (Switzerland) 12:3523. doi: 10.3390/w12123523
Fuhrimann, S., Stalder, M., Winkler, M. S., Niwagaba, C. B., Babu, M., Masaba, G., et al. (2015). Microbial and chemical contamination of water, sediment and soil in the Nakivubo wetland area in Kampala, Uganda. Environ. Monit. Assess. 187, 475–415. doi: 10.1007/s10661-015-4689-x
Gabriel, O. A., Azubuike, E. L., Paul, A. O., Sayfullah, O. K., and Tochukwu, N. P. (2017). Abundance and distribution of macro-benthic invertebrates as bio-indicators of water quality in Ikwo River, Ishiagu, south-eastern Nigeria. Int. J. Fauna Biol. Stud. 4, 43–46.
Georgiou, P., Mattas, C., Mattas, K., Lazaridou, D., and Nastis, S. (2024). “Sustainable water resources management based on the DPSIR framework in east and west African countries” in Value chain dynamics in a biodiverse environment: advances in biodiversity, sustainability, and agri-food supply chain development. Mattas, K, Baourakis, G, Zopounidis, C, and Staboulis C editors. (Springer Nature Switzerland: Cham), 77–106.
Gerber, A., and Gabriel, M. J. M. (2002). Aquatic invertebrates of south African rivers : South Africa, Department of Water Affairs and Forestry.
Gichana, Z., Njiru, M., Raburu, P. O., and Masese, F. O. (2015). Effects of human activities on benthic macroinvertebrate community composition and water quality in the upper catchment of the M ara R iver B asin, K enya. Lakes & Reservoirs: Research & Management 20, 128–137. doi: 10.1111/lre.12094
Gichana, Z. M., Njiru, M., Raburu, P. O., and Masese, F. O. (2014). Effects of human activities on microbial water quality in Nyangores stream, Mara River basin. Int. J. Sci. Technol. Res. 3, 153–157.
GoU (Government of Uganda) (1999). National Water Policy, Kampala: Ministry of Water and Environment. Kampala, Uganda: Government of Uganda.
Government of Kenya (2006). Environmental management and coordination (water quality) regulations, 2006, Kenya gazette supplement no 68. Nairobi, Kenya: Government Printer.
Government of Tanzania (2009). The water resources management act. Dodoma, Tanzania: Government Printer.
Gouissi, F. M., Samon, O., Abahi, K. S., Adje, D. D., Tchaou, C. M., Piami, Z. O., et al. (2019). Relationship between macroinvertebrates and physico-chemical parameters to access water quality of the Affon River in Bénin. Adv. Entomol. 7, 92–104. doi: 10.4236/ae.2019.74008
Graham, P. M., Dickens, C. W., and Taylor, R. J. (2004). miniSASS—A novel technique for community participation in river health monitoring and management. African Journal of Aquatic Science 29, 25–35. doi: 10.2989/16085910409503789
Gritzalis, K. C. (2006). Biological monitoring of Mediterranean rivers with special reference to Greece. Biol. Monitor. Rivers, 1, 295–325. doi: 10.1002/0470863781.ch16
Guiry, M. D., and Guiry, G. M. (2015). Algae Base Version 4.2. Galway: World-Wide Electronic Publication, National University of Ireland Available at: http://www.algaebase.org.
Gulin, V., Vlaičević, B., Sertić Perić, M., Rebrina, F., and Matoničkin Kepčija, R. (2022). Taxonomic and functional metrics of ciliates and amoeboid protists in response to stream revitalization. Front. Microbiol. 13:842395. doi: 10.3389/fmicb.2022.842395
Gurney, G. G., Cinner, J. E., Sartin, J., Pressey, R. L., Ban, N. C., Marshall, N. A., et al. (2016). Participation in devolved commons management: multiscale socioeconomic factors related to individuals’ participation in community-based management of marine protected areas in Indonesia. Environ Sci Policy 61, 212–220. doi: 10.1016/j.envsci.2016.04.015
Gustavsson, B. M., Magner, J., Almroth, B. C., Eriksson, M. K., Sturve, J., and Backhaus, T. (2017). Chemical monitoring of Swedish coastal waters indicates common exceedances of environmental thresholds, both for individual substances as well as their mixtures. Mar. Pollut. Bull. 122, 409–419. doi: 10.1016/j.marpolbul.2017.06.082
Haklay, M., Dörler, D., Heigl, F., Manzoni, M., Hecker, S., and Vohland, K. (2021). What is citizen science? The challenges of definition. Sci. Citizen Sci., 13–33. doi: 10.1007/978-3-030-58278-4_2
Harding, W. R., and Taylor, J. C. (2011). The south African diatom index (SADI): A preliminary index for indicating water quality in rivers and streams in southern Africa: Report to the Water Research Commission. Report no. 1701/1/11.
Hecker, S., Wicke, N., Haklay, M., and Bonn, A. (2019). How does policy conceptualize citizen science? A qualitative content analysis of international policy documents. Citizen Science: Theory and Practice 4, 1–16. doi: 10.5334/cstp.230
Hysko, M., Gace, B., and Puto, K. (2010). Microbial water pollution of Vjosa River and the vegetable contamination by irrigation. BALWOIS: water observation and information system for decision support Ohrid, republic of Macedonia May, 25-29.
Juggins, S., and Birks, H. J. B. (2012). Quantitative environmental reconstructions from biological data. Track. Environ. Change Using Lake Sediments 5, 431–494. doi: 10.1007/978-94-007-2745-8_14
Jungwirth, M., Muhar, S., and Schmutz, S.. (2012). Assessing the ecological integrity of running waters, In Proceedings of the International Conference, Held in Vienna, Austria, 149. Springer Science and Business Media
Kaaya, L. T. (2014). Biological assessment of tropical riverine systems using aquatic macroinvertebrates in Tanzania, East Africa. Doctoral Thesis,: University of Cape Town, South Africa.
Kaaya, L. T., Day, J. A., and Dallas, H. F. (2015). Tanzania River scoring system (TARISS): a macroinvertebrate-based biotic index for rapid bioassessment of rivers. Afr. J. Aquat. Sci. 40, 109–117. doi: 10.2989/16085914.2015.1051941
Kaboré, I., Moog, O., Alp, M., Guenda, W., Koblinger, T., Mano, K., et al. (2016). Using macroinvertebrates for ecosystem health assessment in semi-arid streams of Burkina Faso. Hydrobiologia 766, 57–74. doi: 10.1007/s10750-015-2443-6
Kagalou, I., Leonardos, I., Anastasiadou, C., and Neofytou, C. (2012). The DPSIR approach for an integrated river management framework. A preliminary application on a Mediterranean site (Kalamas River-NW Greece). Water Resour. Manag. 26, 1677–1692. doi: 10.1007/s11269-012-9980-9
Kathol, M., Fischer, H., and Weitere, M. (2011). Contribution of biofilm-dwelling consumers to pelagic–benthic coupling in a large river. Freshw. Biol. 56, 1160–1172. doi: 10.1111/j.1365-2427.2010.02561.x
Katukiza, A. Y., Ronteltap, M., van der Steen, P., Foppen, J. W. A., and Lens, P. N. L. (2014). Quantification of microbial risks to human health caused by waterborne viruses and bacteria in an urban slum. J. Appl. Microbiol. 116, 447–463. doi: 10.1111/jam.12368
Kelly, M., Juggins, S., Guthrie, R., Pritchard, S., Jamieson, J., Rippey, B., et al. (2008). Assessment of ecological status in UK rivers using diatoms. Freshw. Biol. 53, 403–422. doi: 10.1111/j.1365-2427.2007.01903.x
Keough, H. L., and Blahna, D. J. (2006). Achieving integrative, collaborative ecosystem management. Conserv. Biol. 20, 1373–1382. doi: 10.1111/j.1523-1739.2006.00445.x
Khatri, N., and Tyagi, S. (2015). Influences of natural and anthropogenic factors on surface and groundwater quality in rural and urban areas. Front. Life Sci. 8, 23–39. doi: 10.1080/21553769.2014.933716
Kleynhans, C. J. (2007). Module D: Fish response assessment index in river eco-classification: Manual for eco-status determination (version 2). Joint Water Research Commission and Department of Water Affairs and Forestry Report. WRC report no. Tt 330/08. Pretoria
Koblinger, T., and Trauner, D. (2013). Benthic invertebrate assemblages in water bodies of Burkina Faso. Master’s thesis. BOKU University, Vienna.
Kulas, A., Gulin, V., Kepcija, R. M., Zutinic, P., Peric, M. S., Orlic, S., et al. (2021). Ciliates (Alveolata, Ciliophora) as bioindicators of environmental pressure: a karstic river case. Ecol. Indic. 124:107430. doi: 10.1016/j.ecolind.2021.107430
Lange-Bertalot, H., Hofmann, G., Werum, M., Kelly, M., and Cantonati, M. (2017). Freshwater benthic diatoms of Central Europe: Over 800 common species used in ecological assessment. Schmitten-Oberreifenberg: Koeltz Botanical Books.
Li, F., Peng, Y., Fang, W., Altermatt, F., Xie, Y., Yang, J., et al. (2018). Application of environmental DNA metabarcoding for predicting anthropogenic pollution in rivers. Environ. Sci. Technol. 52, 11708–11719. doi: 10.1021/acs.est.8b03869
Lobo, E. A., Schuch, M., Heinrich, C. G., Da Costa, A. B., Düpont, A., Wetzel, C. E., et al. (2015). Development of the trophic water quality index (TWQI) for subtropical temperate Brazilian lotic systems. Environ. Monit. Assess. 187, 354–313. doi: 10.1007/s10661-015-4586-3
López-López, E., and Sedeño-Díaz, J. E. (2015). Biological indicators of water quality: the role of fish and macroinvertebrates as indicators of water quality. Environ. Indicat, 643–661. doi: 10.1007/978-94-017-9499-2_37
Lowe, S., Dallas, H., Kennedy, M., Taylor, J. C., Gibbins, C., Lang, P., et al. (2013). The SAFRASS biomonitoring scheme: general aspects, macrophytes (ZMTR) and benthic macroinvertebrates (ZISS) protocols. Produced ACP Sci. Technol. Program. 1–20.
Lunde, K. B., and Resh, V. H. (2012). Development and validation of a macroinvertebrate index of biotic integrity (IBI) for assessing urban impacts to northern California freshwater wetlands. Environ. Monit. Assess. 184, 3653–3674. doi: 10.1007/s10661-011-2214-4
M’Erimba, C. M., Mathooko, J. M., Karanja, H. T., and Mbaka, J. G. (2014). Monitoring water and habitat quality in six rivers draining the Mt. Kenya and Aberdare catchments using macroinvertebrates and qualitative habitat scoring. Egerton J. Sci. Technol. 14, 81–104.
Mamun, M., and An, K. G. (2020). Stream health assessment using chemical and biological multi-metric models and their relationships with fish trophic and tolerance indicators. Ecol. Indic. 111:106055. doi: 10.1016/j.ecolind.2019.106055
Mandal, F. B. (2023). The neglected role of ciliates as bioindicators. Israel J. Ecol. Evol. 1, 1–9. doi: 10.1163/22244662-bja10063
Mangi, H. O., Onywere, S. M., and Kitur, E. C. (2023). Fish productivity response to water quality variations: a case study of nyumba ya mungu dam, in pangani water basin, Tanzania. Int. J. Ecol. 2023, 1–11. doi: 10.1155/2023/7353898
Mann, D. (2017). “Diatoms of the United States 1. Taxonomy, ultrastructure and descriptions of new species and other rarely reported taxa from Lake sediments in the Western USAJP” in Bibliotheca Diatomologica. eds. B. L. Kociolek, D. Bennett, E. Thomas, M. Brady, and C. Graeff, England, UK:Cambridge University Press 103–108.
Mapenzi, L. L., Shimba, M. J., Moto, E. A., Maghembe, R. S., and Mmochi, A. J. (2020). Heavy metals bio-accumulation in tilapia and catfish species in Lake Rukwa ecosystem Tanzania. J. Geochem. Explor. 208:106413. doi: 10.1016/j.gexplo.2019.106413
Masese, F. O., Achieng, A. O., Raburu, P. O., Lawrence, T., Ives, J. T., Nyamweya, C., et al. (2020). Distribution patterns and diversity of riverine fishes of the Lake Victoria Basin, Kenya. Int. Rev. Hydrobiol. 105, 171–184. doi: 10.1002/iroh.202002039
Masese, F. O., Arimoro, F. O., and O'Brien, G. C. (2021). Editorial: advances in biomonitoring for the sustainability of vulnerable African riverine ecosystems. Front. Water 3:772682. doi: 10.3389/frwa.2021.772682
Masese, F. O., Omukoto, J. O., and Nyakeya, K. (2013). Biomonitoring as a prerequisite for sustainable water resources: a review of current status, opportunities and challenges to scaling up in East Africa. Ecohydrol. Hydrobiol. 13, 173–191. doi: 10.1016/j.ecohyd.2013.06.004
Masese, F. O., and Raburu, P. O. (2017). Improving the performance of the EPT index to accommodate multiple stressors in Afrotropical streams. Afr. J. Aquat. Sci. 42, 219–233. doi: 10.2989/16085914.2017.1392282
Masese, F. O., Raburu, P. O., and Muchiri, M. (2009). A preliminary benthic macroinvertebrate index of biotic integrity (B-IBI) for monitoring the Moiben River, Lake Victoria Basin, Kenya. Afr. J. Aquat. Sci. 34, 1–14. doi: 10.2989/AJAS.2009.34.1.1.726
Masese, F. O., Wanderi, E. W., Nyakeya, K., Achieng, A. O., Fouchy, K., and McClain, M. E. (2023). Bioassessment of multiple stressors in Afrotropical rivers: evaluating the performance of a macroinvertebrate-based index of biotic integrity, diversity, and regional biotic indices. Front. Environ. Sci. 11:307. doi: 10.3389/fenvs.2023.1015623
Mataba, G. R., Verhaert, V., Blust, R., and Bervoets, L. (2016). Distribution of trace elements in the aquatic ecosystem of the Thigithe river and the fish Labeo victorianus in Tanzania and possible risks for human consumption. Sci. Total Environ. 547, 48–59. doi: 10.1016/j.scitotenv.2015.12.123
Maurya, G. K., and Pachauri, S. (2022). Fungi: the indicators of pollution. Freshwater Mycol. 16, 277–296. doi: 10.1016/B978-0-323-91232-7.00012-X
Mbao, E. O., Gao, J., Wang, Y., Sitoki, L., Pan, Y., and Wang, B. (2020). Sensitivity and reliability of diatom metrics and guilds in detecting the impact of urbanization on streams. Ecol. Indic. 116:106506. doi: 10.1016/j.ecolind.2020.106506
Mbao, E. O., Nyika, J., Sheng, T., Ochieng, B., Sitoki, L., Oduor, S. O., et al. (2023). A bibliometric study on diatom DNA metabarcording for water quality monitoring: a global perspective. Afr. J. Aquat. Sci. 48, 345–356. doi: 10.2989/16085914.2023.2282493
Mbao, E. O., Odinga, E. S., Nyika, J., Ochieng, B., and Kitaka, N. (2022). A bibliometric study on the use of diatoms in water quality monitoring and bioassessment in Africa across 10-year (2012–2022) period. Aquat. Sci. 84:58. doi: 10.1007/s00027-022-00891-2
Melcher, A., Ouedraogo, R., Oueda, A., Somda, J., Toe, P., Sendzimir, J., et al. (2020). SUSFISHBook-sustainable fisheries and water management; transformation pathways for Burkina Faso. SUSFISH+ Project Consortium. Available at: http://susfish.boku.ac.at (Accessed November 6, 2023)
Mills, K., and Ryves, D. B. (2012). Diatom-based models for inferring past water chemistry in western Ugandan crater lakes. J. Paleolimnol. 48, 383–399. doi: 10.1007/s10933-012-9609-2
Mochizuki, Y., and Yarime, M. (2016). “Education for sustainable development and sustainability science” in Re-Purposing Higher Education and Research. eds. M. Barth, G. Michelsen, I. Thomas, and M. Rieckmann (London: Routledge Handbook of Higher Education for Sustainable Development), 11–24.
Mohammed-Geba, K., Galal, M., Osman, G., and Soliman, M. (2019). Application of DNA barcoding for identifying potential biotechnological candidate organisms from the River Nile, Egypt. Egypt. J. Aquatic Biol. Fisheries 23, 563–575. doi: 10.21608/ejabf.2019.62580
Moog, O., Schmutz, S., and Schwarzinger, I. (2018). “Biomonitoring and bioasssessment” in Riverine ecosystem management. eds. S. Schmutz and J. Sendzimir, (Switzerland: Springer International Publishing), 371–390.
Mthimkhulu, S., Dallas, H., Day, J., and Hoko, Z. (2004). Biological assessment of the state of the water quality in the Mbuluzi River, Swaziland. In In Proceedings of the IWA specialist group conference on water and wastewater management for developing countries.
Musonge, P. L. S., Boets, P., Lock, K., Ambarita, M. N. D., Forio, M. A. E., and Goethals, P. L. (2020). Rwenzori score (RS): a benthic macroinvertebrate index for biomonitoring Rivers and streams in the Rwenzori region, Uganda. Sustainability 12:10473. doi: 10.3390/su122410473
Mutai, E. K. (2013). Impact of water and sediment quality on bacterial densities and diversities in marine pond culture system in Mtwapa Creek, Kenya. Dissertation, University of Eldoret.
Mwakalobo, S., Lazaro, S., Lugomela, C., Lyimo, T. J., and Thomas, J. (2013). Assessment of faecal bacteria contamination in selected coastal waters of Tanzania. J. Biol. Life Sci. 4, 63–82. doi: 10.5296/jbls.v4i2.3414
Mwedzi, T., Bere, T., and Mangadze, T. (2016). Macroinvertebrate assemblages in agricultural, mining, and urban tropical streams: implications for conservation and management. Environ. Sci. Pollut. Res. 23, 11181–11192. doi: 10.1007/s11356-016-6340-y
Mzungu, E., Yakub, S., and Anyimba, E. S. (2022). Macroinvertebrates as bio-indicators of water quality in Omubira stream, in Kakamega County, Kenya. Int. J. Fisheries Aquatic Stud. 10, 70–77. doi: 10.22271/fish.2022.v10.i4a.2707
Naiga, R., and Penker, M. (2014). Determinants of Users' willingness to contribute to safe water provision in rural Uganda. J. Local Self Government 12, 695–714. doi: 10.4335/12.3.695-714(2014)
Naigaga, I., Kaiser, H., Muller, W. J., Ojok, L., Mbabazi, D., Magezi, G., et al. (2011). Fish as bioindicators in aquatic environmental pollution assessment: a case study in Lake Victoria wetlands, Uganda. Physics Chem. Earth 36, 918–928. doi: 10.1016/j.pce.2011.07.066
Nalugwa, A., Jørgensen, A., Nyakaana, S., and Kristensen, T. K. (2010). Molecular phylogeny of Bulinus (Gastropoda: Planorbidae) reveals the presence of three species complexes in the Albertine rift freshwater bodies. Int J Genet Mol Biol 2, 130–139.
Namwaya, D. W., Raburu, P. O., and Lunaligo, L. (2013). Diatoms as bioindicators in monitoring water quality, river Sosiani, Kenya. Afr. J. Educ. Sci. Technol. 1, 128–138. doi: 10.2022/ajest.v1i3.204
Ndebele-Murisa, M. R. (2012). Biological monitoring and pollution assessment of the Mukuvisi River, Harare. Zimbabwe. Lakes & Reservoirs Management. 17, 73–80. doi: 10.1111/j.1440-1770.2012.00497.x
Ngonyani, H., and Mourad, K. A. (2019). Role of water user associations on the restoration of the ecosystem in Tanzania. Water 11:141. doi: 10.3390/w11010141
Njue, N., Gräf, J., Weeser, B., Rufino, M. C., Breuer, L., and Jacobs, S. R. (2021). Monitoring of suspended sediments in a tropical forested landscape with citizen science. Frontiers in Water 3:656770. doi: 10.3389/frwa.2021.656770
Norris, R. H., and Hawkins, C. P. (2000). Monitoring river health. Hydrobiologia 435, 5–17. doi: 10.1023/A:1004176507184
Obiero, K. O., Abila, R. O., Njiru, M. J., Raburu, P. O., Achieng, A. O., Kundu, R., et al. (2015). The challenges of management: Recent experiences in implementing fisheries co‐management in Lake Victoria, Kenya. Lakes & Reservoirs: Research & Management 20, 139–154. doi: 10.1111/lre.12095
Oberg, H., Andersen, T. J., Westerberg, L. O., Risberg, J., and Holmgren, K. (2012). A diatom record of recent environmental change in Lake Duluti, northern Tanzania. J. Paleolimnol. 48, 401–416. doi: 10.1007/s10933-012-9615-4
Obubu, J. P. (2010). Identifying Applicable Bio-assessment and monitoring methods for sustainable management of Ugandan river quality using macro-benthic invertebrates as indicators. MSc thesis. The Netherlands: UNESCO–IPGL.
Obubu, J. P., Mengistou, S., Fetahi, T., Graf, W., and Odong, R. (2021). A critical review of macroinvertebrate-based bioassessment approaches in Africa’s lotic systems: developments, challenges, and legal requirements. Afr. J. Aquat. Sci. 46, 377–389. doi: 10.2989/16085914.2021.1924609
Ochieng, H., Gandhi, W. P., Magezi, G., Okot-Okumu, J., and Odong, R. (2021). Diversity of benthic macroinvertebrates in anthropogenically disturbed Aturukuku River, Eastern Uganda. African Zoology 56, 85–103. doi: 10.1080/15627020.2021.1885309
Ochieng, H., Magezi, G., Gandhi, W. P., Okot-Okumu, J., and Odong, R. (2022). Epipelic diatom diversity as a bioindicator in river Aturukuku, eastern Uganda. Plant Ecol. Diversity 15, 77–92. doi: 10.1080/17550874.2022.2058430
Odhiambo, C. O., Ngugi, C. C., Sharma, R. R., and Oyoo-Okoth, E. (2024). Macroinvertebrate metrics and lipid profiles as potential indicators of land use influence in a high altitude tropical highland stream (Sagana River basin, Kenya). Ecol. Indic. 160:111848. doi: 10.1016/j.ecolind.2024.111848
Ogbeibu, A. E., Omoigberale, M. O., Ezenwa, I. M., and Oboh, I. P. (2013). Application of some biometric indices in the assessment of the water quality of the Benin River, Niger Delta, Nigeria. Trop. Freshw. Biol. 22, 49–64. doi: 10.4314/tfb.v22i1.5
Oigara, D. K., and Masese, F. O. (2017). Evaluation of the south African scoring system (SASS 5) biotic index for assessing the ecological condition of the Mara River, Kenya. Afr. J. Educ. Sci. Technol. 4, 41–51. doi: 10.2022/ajest.v4i2.4
Olal, B. A., Owuor, J. B., Tsingalia, H. M., and Osano, O. (2014). Assessment of microbiological contaminants in community water sources in lower Nyakach division, Kisumu County, Kenya. Biosciences 74, 26935–26942.
Omondi, L.A. (2021). Evaluation of Faecal Indicator Bacteria and antimicrobial resistance of Escherichia coli isolated from river Njoro, Nakuru County, Kenya. Master’s thesis. Egerton University.
Osure, G., Getabu, A., Aura, C., Omondi, R., and Basweti, E. (2022). Longitudinal fish species diversity and distribution in a medium-sized afro-tropical river in the Lake Victoria Basin. Pan Africa Sci. J. 2, 246–274. doi: 10.47787/pasj.v2i2.18
Palmer, R. W., and Taylor, E. D. (2004). The Namibian scoring system (NASS) version 2 rapid bio-assessment method for rivers. Afr. J. Aquat. Sci. 29, 229–234. doi: 10.2989/16085910409503814
Pandey, L. K., Sharma, Y. C., Park, J., Choi, S., Lee, H., Lyu, J., et al. (2018). Evaluating features of periphytic diatom communities as biomonitoring tools in fresh, brackish and marine waters. Aquat. Toxicol. 194, 67–77. doi: 10.1016/j.aquatox.2017.11.003
Pantle, R., and Buck, H. (1955). Die biologische Uberwachung der Gewässer und die Darstellung der Ergebnisse. Green World Foundation Journal. 96, 604–604.
Parmar, T. K., Rawtani, D., and Agrawal, Y. K. (2016). Bioindicators: the natural indicator of environmental pollution. Front. Life Sci. 9, 110–118. doi: 10.1080/21553769.2016.1162753
Pawlowski, J., Kelly-Quinn, M., Altermatt, F., Apotheloz-Perret-Gentil, L., Beja, P., Boggero, A., et al. (2018). The future of biotic indices in the ecogenomic era: integrating (e) DNA metabarcoding in biological assessment of aquatic ecosystems. Sci. Total Environ. 637-638, 1295–1310. doi: 10.1016/j.scitotenv.2018.05.002
Persoone, G., and De Pauw, N. (1979). “Systems of biological indicators for water quality assessment” in Biological aspects of freshwater pollution (Pergamon), 39–75. doi: 10.1016/B978-0-08-023442-7.50007-4
Potapova, M., and Charles, D. F. (2003). Distribution of benthic diatoms in US rivers in relation to conductivity and ionic composition. Freshw. Biol. 48, 1311–1328. doi: 10.1046/j.1365-2427.2003.01080.x
Raburu, P. O., and Masese, F. O. (2012). Development of a fish-based index of biotic integrity (FIBI) for monitoring riverine ecosystems in the Lake Victoria drainage basin, Kenya. River Res. Appl. 28, 23–38. doi: 10.1002/rra.1428
Raburu, P. O., Masese, F. O., and Mwasi, B. N. (2022). Low diversity of fishes in high elevation Afrotromontane streams renders them unsuitable for biomonitoring. Afr. J. Ecol. 60, 1029–1042. doi: 10.1111/aje.13056
Radhakrishnan, R., and Jayaprakas, V. (2015). Free living protozoans as bioindicators in Vembanad Lake, Kerala, India, an important Ramsar site. Int. J. Fisheries Aquatic Stud. 2, 192–197.
Requier, F., Andersson, G. K., Oddi, F. J., and Garibaldi, L. A. (2020). Citizen science in developing countries: how to improve volunteer participation. Front. Ecol. Environ. 18, 101–108. doi: 10.1002/fee.2150
Riato, L., Leira, M., Della Bella, V., and Oberholster, P. (2018). Development of a diatom-based multimetric index for acid mine drainage impacted depressional wetlands. Sci. Total Environ. 612, 214–222. doi: 10.1016/j.scitotenv.2017.08.181
Richards, N. (2019). Water users associations in Tanzania: local governance for whom? Water 11:2178. doi: 10.3390/w11102178
Richards, N., and Syallow, D. (2018). Water resources users associations in the Mara Basin, Kenya: Pitfalls and opportunities for community based natural resources management. Frontiers in Environmental Science, 6:138. Volume 6 – 2018. doi: 10.3389/fenvs.2018.00138
Rolston, A., Jennings, E., and Linnane, S. (2017). Water matters: An assessment of opinion on water management and community engagement in the Republic of Ireland and the United Kingdom. PLoS One 12:0174957. doi: 10.1371/journal.pone.0174957
Ruaro, R., Gubiani, E. A., Hughes, R. M., and Mormul, R. P. (2020). Global trends and challenges in multimetric indices of biological condition. Ecol. Indic. 110:105862. doi: 10.1016/j.ecolind.2019.105862
Salinas-Camarillo, V. H., Carmona-Jimenez, J., and Lobo, E. A. (2020). Development of the diatom ecological quality index (DEQI) for peri-urban mountain streams in the basin of Mexico. Environ. Sci. Pollut. Res. 28, 14555–14575. doi: 10.1007/s11356-020-11604-3
Samson, R., Rajput, V., Shah, M., Yadav, R., Sarode, P., Dastager, S. G., et al. (2020). Deciphering taxonomic and functional diversity of fungi as potential bioindicators within confluence stretch of Ganges and Yamuna Rivers, impacted by anthropogenic activities. Chemosphere 252:126507. doi: 10.1016/j.chemosphere.2020.126507
Schmidt, R. C., Woods, T., and Nyingi, W. D. (2022). Drivers of species richness and beta diversity of fishes in an Afrotropical intermittent river system. Ecol. Evol. 12:e9659. doi: 10.1002/ece3.9659
Segurado, P., Santos, J. M., Pont, D., Melcher, A. H., Jalon, D. G., Hughes, R. M., et al. (2011). Estimating species tolerance to human perturbation: expert judgment versus empirical approaches. Ecol. Indic. 11, 1623–1635. doi: 10.1016/j.ecolind.2011.04.006
Sekadende, B. C., Michael, A., Painter, S. C., Shayo, S., Noyon, M., and Kyewalyanga, M. S. (2021). Spatial variation in the phytoplankton community of the Pemba Channel, Tanzania, during the south-east monsoon. Ocean Coast. Manag. 212:105799. doi: 10.1016/j.ocecoaman.2021.105799
Sendzimir, J., and Schmutz, S. (2018). “Challenges in riverine ecosystem management” in Riverine ecosystem management. eds. S. Schmutz and J. Sendzimir, (Switzerland: Springer International Publishing), 1–16.
Sila, O. N. A. (2019). Physico-chemical and bacteriological quality of water sources in rural settings, a case study of Kenya, Africa. Sci. African 2:00018. doi: 10.1016/j.sciaf.2018.e00018
Singara Charya, M. A. (2015). Fungi: an overview. Plant Biol. Biotechnol. 1, 197–215. doi: 10.1007/978-81-322-2286-6-7
Sokile, C. S., Mwaruvanda, W., and Van Koppen, B. (2005). Integrated water resource management in Tanzania: interface between formal and informal institutions. African Water Laws: Plural Legislative Frameworks for Rural Water Management in Africa, ohannesburg, South Africa. 26–28.
Stevenson, R. J., Pan, Y., and Vandam, H. (2010). “Assessing environmental conditions in rivers and streams with diatoms” in The diatoms: Applications for the environmental and earth sciences, (England, UK: Cambridge University Press).
Sumudumali, R. G. I., and Jayawardana, J. M. C. K. (2021). A review of biological monitoring of aquatic ecosystems approaches: with special reference to macroinvertebrates and pesticide pollution. Environ. Manag. 67, 263–276. doi: 10.1007/s00267-020-01423-0
Syberg-Olsen, M. J., Irwin, N. A., Vannini, C., Erra, F., Di Giuseppe, G., Boscaro, V., et al. (2016). Biogeography and character evolution of the ciliate genus Euplotes (Spirotrichea, Euplotia), with description of Euplotes curdsi sp. nov. PLoS One 11, 11:e0165442. doi: 10.1371/journal.pone.0165442
Tampo, L., Lazar, I. M., Kaboré, I., Oueda, A., Akpataku, K. V., Djaneye-Boundjou, G., et al. (2020). A multimetric index for assessment of aquatic ecosystem health based on macroinvertebrates for the Zio River basin in Togo. Limnologica 83:125783. doi: 10.1016/j.limno.2020.125783
Terry, A., McLaughlin, O., and Kazooba, F. (2015). Improving the effectiveness of Ugandan water user committees. Dev. Pract. 25, 715–727. doi: 10.1080/09614524.2015.1046421
The Water Act (2002). Government of Kenya, Ministry of Water and Irrigation. Available at: http://kenyalaw.org/kl/fileadmin/pdfdownloads/RepealedStatutes/WaterAct_Cap372_.pdf (Accessed September 4, 2023)
The Water Act (2016). Water Services Regulatory Board. Available at: https://wasreb.go.ke/the-water-act-2016 (Accessed November 13, 2023)
Tirjakova, E., and Vdacny, P. (2013). Analysis and evolution of water quality of the upper Váh River (northern Slovakia) by long-term changes in the community structure of ciliates (Protista: Ciliophora). Biologia 68, 667–678. doi: 10.2478/s11756-013-0211-5
Tomas, D., Curlin, M., and Maric, A. S. (2017). Assessing the surface water status in Pannonian ecoregion by the water quality index model. Ecol. Indic. 79, 182–190. doi: 10.1016/j.ecolind.2017.04.033
Triest, L., Lung'ayia, H., Ndiritu, G., and Beyene, A. (2012). Epilithic diatoms as indicators in tropical African rivers (Lake Victoria catchment). Hydrobiologia 695, 343–360. doi: 10.1007/s10750-012-1201-2
Tumusiime, J., Tolo, C. U., Dusabe, M. C., and Albrecht, C. (2019). Reliability of the Tanzania river scoring system (TARISS) macroinvertebrate index of water quality: a case study of the river Mpanga system, Uganda. J. Freshw. Ecol. 34, 541–557. doi: 10.1080/02705060.2019.1631895
Turibamwe, E., and Wangalwa, R. (2020). A comparative study of two biological monitoring systems in assessing water quality: a case of river Birira, Sheema district, Uganda. Water Conserv. Manag. 4, 07–14. doi: 10.26480/wcm.01.2020.07.14
Ukomadu, N. M. I. (2012). Host associations and pollution responses of ciliate epibionts of macroinvertebrates in the six rivers in the Western Cape Province of South Africa. Master's thesis, University of Cape Town.
Urbanič, G., Mihaljević, Z., Petkovska, V., and Pavlin Urbanič, M. (2020). Disentangling the effects of multiple stressors on large rivers using benthic invertebrates—a study of southeastern European large rivers with implications for management. Water 12:621. doi: 10.3390/w12030621
Vermaat, J. E. (2005). “Periphyton dynamics and influencing factors” in Periphyton: Ecology, exploitation and management, (England, UK: CABI Publishing), 35–49.
Wanderi, E. W., Gettel, G. M., Singer, G. A., and Masese, F. O. (2022). Drivers of water quality in Afromontane-savanna rivers. Front. Environ. Sci. 10:972153. doi: 10.3389/fenvs.2022.972153
Wang, X. N., Ding, H. Y., He, X. G., Dai, Y., Zhang, Y., and Ding, S. (2018). Assessing fish species tolerance in the Huntai River basin, China: biological traits versus weighted averaging approaches. Water 10:1843. doi: 10.3390/w10121843
Warnasuriya, S. D., Udayanga, D., Manamgoda, D. S., and Biles, C. (2023). Fungi as environmental bioindicators. Sci. Total Environ. 892:164583. doi: 10.1016/j.scitotenv.2023.164583
Waswala, B. O., Otieno, O. N., and Buoga, J. (2019). Youth engagement for environmental education and sustainable lifestyles. Cult. Environ., 1, 305–317. doi: 10.1163/9789004396685_019
Water Aid (2013). Strengthening WASH services and community resilience through community based water resource management. Burkino Faso: WaterAid.
Watson, M., and Dallas, H. (2013). Bioassessment in ephemeral rivers: constraints and challenges in applying macroinvertebrate sampling protocols. Afr. J. Aquatic Sci. 38, 35–51. doi: 10.2989/16085914.2012.742419
Waya, R. K., Limbu, S. M., Ngupula, G. W., Mwita, C. J., and Mgaya, Y. D. (2014). Spatial patterns of zooplankton distribution and abundance in relation to phytoplankton, fish catch and some water quality parameters at Shirati Bay, Lake Victoria-Tanzania. Tanzania J. Sci. 40, 20–32.
Wen, Y., Schoups, G., and Van De Giesen, N. (2017). Organic pollution of rivers: combined threats of urbanization, livestock farming and global climate change. Sci. Rep. 7:43289. doi: 10.1038/srep43289
Whitelaw, G., Vaughan, H., Craig, B., and Atkinson, D. (2003). Establishing the Canadian community monitoring network. Environ. Monit. Assess. 88, 409–418. doi: 10.1023/A:1025545813057
World Health Organisation (2006). Guidelines for drinking water quality (3rd edition). Available at: https://www.who.int/publications/i/item/9241546743 (Accessed October 15, 2023)
Xie, Y., Wang, J., Wu, Y., Ren, C., Song, C., Yang, J., et al. (2016). Using in situ bacterial communities to monitor contaminants in river sediments. Environ. Pollut. 212, 348–357. doi: 10.1016/j.envpol.2016.01.031
Xu, H., Yong, J., and Xu, G. (2016). Bioassessment of water quality status using a potential bioindicator based on functional groups of planktonic ciliates in marine ecosystems. Mar. Pollut. Bull. 110, 409–414. doi: 10.1016/j.marpolbul.2016.06.033
Yegon, M. J., Masese, F. O., Sitati, A., and Graf, W. (2021). Elevation and land use as drivers of macroinvertebrate functional composition in Afromontane headwater streams. Mar. Freshw. Res. 72, 1517–1532. doi: 10.1071/MF21048
Yillia, P. T., Kreuzinger, N., and Mathooko, J. M. (2008). The effect of in-stream activities on the Njoro River, Kenya. Part II: microbial water quality. Physics Chem. Earth 33, 729–737. doi: 10.1016/j.pce.2008.06.040
Zelinka, M. (1961). Zur Prazisierung der biologische Klassifikation der Reinheit fliessender Gewasser. Arch. Hydrobiol. 57, 389–407.
Zeni, J. O., Hoeinghaus, D. J., and Casatti, L. (2017). Effects of pasture conversion to sugarcane for biofuel production on stream fish assemblages in tropical agroecosystems. Freshw. Biol. 62, 2026–2038. doi: 10.1111/fwb.13047
Zhao, Y., Yi, Z., Warren, A., and Song, W. (2018). Species delimitation for the molecular taxonomy and ecology of the widely distributed microbial eukaryote genus Euplotes (Alveolata, Cil-iophora). Proceed. R. Soc. B Biol. Sci. 285, 2017–2159. doi: 10.1098/rspb.2017.2159
Keywords: biomonitoring, community-based collaboration, environmental changes, citizen science, rivers, integrated water resource management, water quality
Citation: Kitaka N, Omondi LA, Mureithi PW, Bauer A, Melcher A and Ssanyu GA (2024) A critical review of biomonitoring in East African rivers: fostering community-based collaboration for environmental change observation. Front. Water. 6:1360941. doi: 10.3389/frwa.2024.1360941
Received: 24 December 2023; Accepted: 07 May 2024;
Published: 17 July 2024.
Edited by:
Francis O. Arimoro, Federal University of Technology Minna, NigeriaReviewed by:
Ovie Edegbene, Federal University of Health Sciences Otukpo, NigeriaCopyright © 2024 Kitaka, Omondi, Mureithi, Bauer, Melcher and Ssanyu. This is an open-access article distributed under the terms of the Creative Commons Attribution License (CC BY). The use, distribution or reproduction in other forums is permitted, provided the original author(s) and the copyright owner(s) are credited and that the original publication in this journal is cited, in accordance with accepted academic practice. No use, distribution or reproduction is permitted which does not comply with these terms.
*Correspondence: Nzula Kitaka, bmtpdGFrYUBlZ2VydG9uLmFjLmtl
Disclaimer: All claims expressed in this article are solely those of the authors and do not necessarily represent those of their affiliated organizations, or those of the publisher, the editors and the reviewers. Any product that may be evaluated in this article or claim that may be made by its manufacturer is not guaranteed or endorsed by the publisher.
Research integrity at Frontiers
Learn more about the work of our research integrity team to safeguard the quality of each article we publish.