- Department for Hydrogeology and Hydrochemistry, Institute of Geology, Technical University Bergakademie Freiberg, Freiberg, Germany
Urban water contamination has become a pressing concern due to the surge in urbanization, industrialization, and population growth, resulting in increased pollution levels and the introduction of emerging contaminants (ECs) into the environment. The widespread use of pharmaceuticals (PhACs), driven by medical advancements and economic progress, has led to their presence in aquatic systems, posing risks to both ecosystems and human health. These compounds are released into the environment via effluent of wastewater treatment plants (WWTPs) as they are inefficient in totally removing PhACs. This study focused on PhACs in wastewater, surface water and groundwater of a small city like Freiberg region in Germany. Samples were collected along Münzbach’s path, encompassing hospital discharges and WWTP. Analysis for the measurement of PhACs was conducted via Ultra-high-performance liquid chromatography coupled with tandem mass spectrometry (UHPLC–MS/MS). Results revealed that about 46 out of 54 analyzed PhACs were detected across matrices, with positive findings of 46 substances in wastewater, 37 in surface water, and 19 in groundwater. Among 54 analyzed PhACs, six of the active pharmaceutical ingredients (APIs) like atenolol (ATL), carbamazepine (CBZ), caffeine (CAF), diclofenac (DCF), metformin (MET), and sulfamethoxazole (SMX) were focused in this study. Five of the six APIs were present in all matrices, with MET and CAF widely distributed in an aqueous system. CBZ and DCF were found in wastewater and surface water, ATL was scarce in surface water, while SMX was absent in groundwater. It was observed that PhAC concentration was reduced from wastewater to groundwater, with the highest levels near the WWTP, decreasing as effluents flowed through surface water Münzbach and Freiberger Mulde. It was also observed that the groundwater near the surface water shows the presence of APIs mainly due to the hyporheic zone connecting the surface water and groundwater. Overall, PhAC compounds reflect the city population and their age, the water cycle, and the hydrochemical processes of the compounds themselves.
1 Introduction
The increase in the population, urbanization, industrialization and technology have increased many new emerging environmental contaminants (ECs) in the urban water system especially pharmaceuticals (PhACs), pesticides etc. (Biswas et al., 2022). PhACs are one of the major worrying classes of ECs, which mainly arise from their ability to be biologically active compounds and a relatively low degradation rate (Rivera-Utrilla et al., 2013; Samal et al., 2022). The PhACs that are consumed every day by millions of people and animals are not absorbed completely by the body and therefore released into the wastewater (Isaac-Olivé and Navarro-Frómeta, 2019). Studies reported that approximately 90% of the antibiotics consumed by humans are removed via urine or feces (Tiwari et al., 2017; Stenuick, 2021). In the past decades, the residues of PhACs have been discovered in many environmental matrices that include surface water, groundwater and wastewater treatment plants (WWTPs; Hughes et al., 2013; Patel et al., 2019; Silori et al., 2022; Waleng and Nomngongo, 2022). These PhACs enter the WWTPs via bathing, excretion, and unwanted disposal of PhACs in drains, industrial as well as hospital wastewater streams etc. (Adeleye et al., 2022; Biswas et al., 2022; Waleng and Nomngongo, 2022). Some of the PhACs are released directly or indirectly into the soil, surface water and groundwater from either agricultural runoff, landfills, or leakages (Adeleye et al., 2022). These PhACs do not degrade easily and are present for longer periods in the environment due to their stable structure (Samal et al., 2022). The WWTPs are also inefficient in removing all types of PhACs and pose a threat to the aquatic ecosystem (Alfonso-Muniozguren et al., 2021; Ranjan et al., 2022). Since this effluent water does not meet the quality standards for drinking water, its use is limited to particular applications like groundwater recharge, industrial processes, wetlands restoration, or agricultural irrigation (Veil, 2007; Lyu et al., 2016; Gilabert-Alarcón et al., 2018; Carter et al., 2019). In Europe, most of the wastewater is treated in WWTPs and the effluent is discharged into the surface water (Pallares-Vega et al., 2019). Therefore, the release of PhACs into the environment through wastewater effluent or surface runoff from agricultural fields creates an emerging concern. The harmful effect of PhACs has also been examined, indicating a higher risk for aquatic organisms compared to humans being affected by contaminated water containing a trace substance (Olson et al., 2000; Crane et al., 2006).
Several studies on the detection of PhACs in the aqueous system have been carried out in European countries (Bendz et al., 2005; Kosjek et al., 2005; Lindberg, 2006; Bergmann et al., 2011; Hughes et al., 2013; Hamann et al., 2016; Pallares-Vega et al., 2019; Castro-Pastrana et al., 2020). For example, Lindberg (2006) studied the detection of antibiotics in five different WWTPs in Sweden. They found different concentrations of antibiotics in different WWTPs, among which the most common antibiotics were ciprofloxacin, doxycycline, fluoroquinolones, sulfamethoxazole (SMX) and trimethoprim. Castro-Pastrana et al. (2020) reviewed the occurrence of nonsteroidal anti-inflammatory drugs (NSAIDs) in the water system of Asia and Europe. They observed that among all the NSAIDs diclofenac (DCF) is the most frequently detected PhAC in all the studies. Similarly, Kosjek et al. (2005) detected DCF and naproxen as some of the NSAIDs in the river water of Slovenia. Bergmann et al. (2011) confirm the presence of 156 PhACs in environmental media of Germany like surface water, drinking water and groundwater. In this report, they found that most of the PhACs are present in surface water within a concentration range of ng/L to μg/L. Similarly, Rodriguez-Mozaz et al. (2020) studied the presence of antibiotics in the effluent of WWTPs of 7 European countries (Cyprus, Finland, Germany, Ireland, Norway, Portugal and Spain) in the consecutive years of 2015 and 2016. They found that among 53 antibiotics 17 were detected in the WWTP effluent. Hamann et al. (2016) investigated the long-term detection of about 247 compounds in Lek River, Netherlands. These compounds are analyzed irregularly from 1999 to 2013. From their result, they observed that 10 compounds (including CBZ) show constant behavior with no decrease in concentration while other shows fluctuating behavior. A study reported that WWTPs in Dutch discharged an average of about 106 copies of antibiotic-resistant genes per liter effluent of wastewater (Pallares-Vega et al., 2019). Bunting et al. (2021) reviewed around 39 studies to assess the occurrence of PhACs in European groundwater. They examined that the most frequent occurrence of PhACs is caffeine (CAF) and CBZ at a concentration of up to 14.8 μg/L and 2.3 μg/L, respectively. Bendz et al. (2005) reported higher concentrations of atenolol (ATL) and CBZ within a range of μg/L in Hoje River, Sweden. One of the studies (Jurado et al., 2022) was conducted to detect the PhACs in the recharged aquifer by WWTP effluent receiving river water. They analyzed 92 PhACs among which they observed 35 PhACs in all the groundwater samples within a range of ng/L–45 μg/L. Another study (Wilkinson et al., 2022) in the Danube River of Hungary, was conducted to examine the presence of painkillers and antiepileptic medicine. They found that the most frequently detected active substances are CBZ, CAF and metformin (MET), which was detected in more than half of the sampling sites. József et al. (2023) studied the effect of water flow on the concentration of PhACs. They found that the concentration of the selected compounds was highest (μg/L) at the sampling point near the floodgate compared to the other points. They also conclude that the active substances were influenced by the water yield and flow rate generated by floodgates showing higher concentrations. All the above studies are conducted either on a large city having good infrastructure, WWTPs, huge population or on big rivers or porous aquifers. However, studies on PhAC contamination in the aqueous system of small-town cities with minimum facilities and population and the groundwater having poor interconnecting pores have not yet been studied in detail.
The objective of this study is to evaluate the occurrence of PhACs in the urban water system of the Freiberg region in Germany. The water system in Freiberg is scattered, including very small key ponds and a river that flows through the city, connecting to the WWTPs. By selecting Freiberg as a study site, the investigation aims to uncover the sources and potential interactions of PhACs within a small urban environment. To achieve this, the water samples were systematically collected from various sources, including wastewater, surface water, and groundwater, within and around the city of Freiberg. The collected water samples underwent analysis using ultra-high phase liquid chromatography coupled with tandem mass spectrometry (UHPLC–MS/MS). Based on the results of major ion and active pharmaceutical ingredient (API) data, the nature and interaction of water matrices in the environment are discussed. Ultimately, the research seeks to deepen our understanding of pharmaceutical contamination in the urban water system of the Freiberg area, offering valuable insights into the characteristics, distribution, and underlying factors associated with the presence of APIs in urban water matrices.
2 Hydrogeological condition of the study area
Freiberg, a small-town city in the Saxony state of Germany, with a minimal population of around 40,000 inhabitants (City population, 2011), infrastructure, and agriculture, features only one WWTP and hospital selected for this study. This region has a mining history from a period of 800 years showing its influence in the surface water as well as the groundwater system. The long-term mining in the orthogneiss complexes and its ore-bearing veins in Freiberg lead to an extensive input of sulfate by oxidation processes and heavy metals like lead, zinc and arsenic due to ore processing in the groundwater system. The graphic representation of the geology of Freiberg is shown in Supplementary Figure S3. The region features several significant natural receiving rivers within its flat undulating and hilly terrain, among which the Freiberger Mulde and the Münzbach stream, is the focus of this study. These are moving from south to north indicated as upstream and downstream in this study (Figure 1). In terms of hydrogeological classification, the study area falls under the Erzgebirge central zone according to BGR and SDG, (2019). Notably, the upcoming Freiberg gneiss forms a complex fractured aquifer composed of small-scale interconnected complexes. Groundwater movement in this region occurs primarily through fracture and disruption zones (Jordan and Weder, 1995). The Münzbach Valley near Loßnitz houses the Freiberg wastewater disposal facility. This municipal WWTP serves the city of Freiberg and some suburban municipalities by two main sewers. The Münzbach main sewer collects wastewater from the historic city center and the urban districts Zug around the districts of Freiberg—East and South. While, the Saubach main sewer serves the districts of Freiberg-West and North, which also encompass areas such as the community hospital and the Freiberg industrial estate. The Saubach canal feeds into the Münzbach main sewer above the WWTP at the level of Möller Street.
3 Materials and methods
3.1 Field survey and sampling
To investigate the water quality parameters and the contamination with trace compounds mentioned in Supplementary Table S3, 16 samples were collected within the study area (shown in Figure 1) during October 2021 from various water matrices. The water samples originate from domestic wastewater, surface water, and groundwater around the city of Freiberg.
Focused on the wastewater discharge, samples were collected from the Münzbach main sewer between the community hospital and the central WWTP. Particularly, the community hospital and its associated domestic wastewater stream are known as the most significant contributors of PhACs to the wastewater flow (Stenuick, 2021; Samal et al., 2022; József et al., 2023). A total of 4 samples were collected for wastewater, one each from the Central Runoff Community Hospital, Wastewater Inspection Shaft, Central Inflow and Runoff WWTP. While 8 samples from the surface water matrix were collected at a rain storage pond, along the flow of the Freiberger Mulde, and the Münzbach as shown in Figure 1 indicating with symbol SWM (surface water measuring). The samples taken are an accurate representation of the water quality both upstream and downstream of the treated wastewater’s discharge into the Münzbach, which serves as a receiving watercourse. The decision-making process for selecting measuring stations was mainly influenced by the federal state monitoring network for surface water and groundwater matrix (LfULG (Landesamt für Umwelt, Landwirtschaft und Geologie): Sachsen dataset, 2022). In total four groundwater observation wells (GWM) were sampled consisting of observation wells on a heavily contaminated site by heavy metals (GWM Silberhof Street), covered mining dumps (GWM Slag Dump Halsbach and GWM Metallurgic Waste Dump) and a privately utilized shaft well in Friedeburg (GWM Freiberg B15/26). Given the limited yield of crystalline aquifers in Saxony, surface water takes on the role of supplying 75% of public water demand through dams and bank filtration wells (Destatis, 2019). The city of Freiberg, which is subject to annual sampling lacks the analysis of trace compounds in the State Water Monitoring Network. Therefore, the analyses of water samples in this study represent the first examination for the detection of trace compounds.
The collection of samples for the various water matrices was carried out following the technical standards. Wastewater samples were collected according to the procedure mentioned in DIN 34802-11 (02/2009), surface water samples followed DIN EN ISO 5667-6 (12/2016) procedure, while groundwater matrix sampling referred to DIN 34802-13 (12/2021). Samples were taken after the fulfillment of hydrochemical and hydraulic criteria. In the groundwater pumping a Honda WX 10 suction pump was used, while a Grundfos submersible pump MP 1 alongside PVC pipes were used for GWM points whose water level exceeds 8 meters. For an analysis of the major ions, composition regarding cations and anions two water samples were taken from each measuring point, sealed within 30 mL polypropylene bottles. Additionally, on-site sample preparation was conducted for the separation of suspended solids and organics, using membrane filters with a pore size of 0.45 μm made of cellulose acetate. Wastewater samples required secondary laboratory treatment due to high-suspended matter content. To prevent trivalent iron and heavy metal precipitation, samples were acidified with concentrated nitric acid in the field before cation examination. For the analysis of trace compounds, the water samples were collected in narrow-necked amber borosilicate glass bottles without filtration.
3.2 Sample analysis
The physicochemical properties of water samples in the field were assessed utilizing IntelliCAL™ electrodes from Hach (Table 1), encompassing specific measures such as electrical conductivity, redox potential, water temperature, oxygen content, and pH value. During groundwater investigations, measurements were performed within a flow cell, while surface water measurements were taken in the flowing wave until constant values were achieved. For the wastewater matrix, a telescopic sampler was used combined with a 1.5 L bucket. The sampling protocols with photo documentation of each measuring point are shown in Supplementary Figure S1.
Major ions in the extracted water were determined using ion exchange chromatography (IC) from Metrohm. Cation analysis was done in the hydrogeological laboratory at the Institute of Geology using Metrosep C6 (4 × 250 mm) separation column having mobile phase 1 mmol/L sodium bicarbonate and 6 mmol/L sodium carbonate (850 Professional IC pro cation system). While anion analysis was measured using IonPac AS14 A 5 μm (3 × 30 mm) as separation column and 1 mmol/L pipecolic acid and 4 mmol/L nitric acid as mobile phase (Dionex DX 120 system) in the geochemical laboratory at the Institute of Mineralogy, TU Bergakademie, Freiberg. Trace compound analysis such as PhACs (including APIs) which was found in the literature within a concentration range of pg./L to ng/L was conducted externally at the hydrogeochemical laboratory in the Institute of Geology, Mineralogy, and Geophysics of Ruhr University, Bochum. The detection of trace compounds in the collected water samples was measured using UHPLC-MS/MS (Sciex EXION LC & Sciex QTRAP 6500+ MS). The method for the analysis was adopted from the research paper (Mehrtens et al., 2022). The list of trace compounds selected for the analysis of water samples in LC-MS/MS are listed in Supplementary Table S3. The internal standards used for calibration and analysis of the active compounds are as follows: Atenolol D7, AtrazinDesethyl D7, 1H Benzotriazol D4, Citalopram D6, Cetirizine D8, Carbamazepine D10, Caffeine 13C3, Chloridazon D5, Diclofenac D4, Erythromycin 13CD3, Fluoxetine D5, Gabapentin D4, Ibuprofen D3, Mecoprop D3, Metformin D6, Metoprolol D7, Metazachlor D6, Paraxanthin D3, and Valsartan D3 all from Sigma-Aldrich ≥ 99% purity. To analyze the target substances, 1 mL of the water sample was combined with 20 μL of internal standard, subjected to centrifugation (IKA mini G, 10,000 rpm, 10 min at 8°C), and introduced to the HPLC-MS/MS by direct injection of 10 μL. The chromatographic separation of trace compounds was done by passing through the C18 column (Phenomenex Luna Omega 1.5 μm Polar C18). The flow rate used was 0.55 mL/min. The separation was operated at 50°C oven temperature while the injection volume was 50 μL. The eluent A was water and 2 mM/L ammonium formate with 0.01% formic acid while eluent B consisted of methanol. All the chemicals used here are of LC/MS grade. The gradient method was used starting from 10% organic solvent (eluent B) for 0.5 min to 95% for 7 min, 95% for 8.5 min and then ending with an initial situation of 10% for another 8.7 min. The electrospray ionization (ESI) method was adopted with a spray voltage of 5.5 kV in positive mode, −4.5 kV in negative modes, temperature 500°C, GS1 and GS2 was 70 and 50, respectively, and curtain gas was 35 V. Depending on the substance and material effects, internal standards were employed for internal calibration and the quantification limit ranged between 10 and 50 ng/L.
4 Results
4.1 Physicochemical properties of aqueous sample
To characterize the aqueous sample collected from different locations of Freiberg, the study of physicochemical parameters was done on-site and in the laboratory.
4.1.1 Wastewater matrix
The result shows that the wastewater pathway is characterized by a neutral to strongly alkaline environment with pH values between 7.2 to 9.5. The specific electrical conductivity ranges from 377 to 2,112 μS/cm, which is correlated with the total dissolved solids measured from 433 mg/L to 2,468 mg/L. The lowest values are to be shown for the measuring point at the Central Runoff of the municipal WWTP and the highest levels at the Central Runoff of the community hospital. The chemical behavior of the waters is characterized by highly variable oxygen contents from 0.78 mg/L to 8.03 mg/L O2 (≈ 9–82% saturation) at redox voltages between +271 mV (Central Inflow WWTP) and +414 mV (Central Runoff WWTP), respectively. For the hardness, a total hardness of 5.0 to 11.0°dH can be stated with a carbonate hardness between 4.0 and 24.0°dH. The detailed characterization of the wastewater is shown in Supplementary Tables S1, S2. Almost all samples are characterized by an artificial hardness, which is due to a significant proportion of carbonate-rich phases and surfactants from textile washing in predominantly domestic wastewater. The ionic composition is dominated by sodium (33–305 mg/L) and potassium (15–140 mg/L) as the cation and bicarbonate (85–525 mg/L) and chloride (53–380 mg/L) as anions. Other ions are calcium, magnesium and sulfate within a range of 29–60 mg/L, 4–9 mg/L, and 34–145 mg/L, respectively (Supplementary Table S2). Nitrate (0.8–20 mg/L), as well as nitrite (0–4.05 mg/L), occur primarily at the Central WWTP Runoff in high concentrations, characterizing the effective degradation of organic compounds by nitrification and denitrification in the wastewater treatment processes. The wastewater samples examined can be regarded as characteristic based on the examined parameters as well as the occurrence and concentration of the described water constituents. The ion balances vary highly from −35.4% to +9.6% due to the heterogeneous composition and origin of the wastewater streams.
4.1.2 Surface water matrix
The physicochemical characterization of surface water is shown in Supplementary Tables S1, S2. It shows that the electrical conductivity of surface water lies between 235 and 667 μS/cm with the total dissolved solids present from 170 to 445 mg/L. In those low to average mineralized waters, there is an alkaline environment with pH values between 7.6 and 8.2. A redox potential between +320 to +410 mV was observed in addition to high oxygen contents between 9.7 and 10.9 mg/L O2 (≈96%–100% saturation). However, the measuring point at the rain storage pond shows a moderate oxygen supply of 6.77 mg/L O2 (≈68% saturation). In terms of water hardness, a total hardness of 4.5°dH (SWM Münzbach stream) to 10.8°dH (SWM 32900) is measured while carbonate hardness is found to be 2.3 and 6.7°dH. In general, the Freiberg area is characterized by soft water due to the lack of soluble carbonate rocks. Further, the ionic composition of the sample shows the presence of sodium, bicarbonate and chloride within a concentration range of 15–75 mg/L, 52–145 mg/L and 24–115 mg/L, respectively. The potassium (2.1–18 mg/L), calcium (18–49 mg/L), magnesium (3–10 mg/L) and sulfate (23–65 mg/L) are also present in the sample (Supplementary Table S2). The levels of nitrate (1.4–25 mg/L) and nitrite (0–1.9 mg/L) are also elevated. The main sources of chemicals in the surface waters in the study area are mainly due to the wastewater effluent and the leaching of agricultural land.
4.1.3 Groundwater matrix
The characterization of groundwater samples shows a pH value of 4.9 to 5.8, which indicates an acidic environment for those waters (Supplementary Table S1). The wide range of electrical conductivity was observed within a range from 409 μS/cm (GWM Silberhof Street) to 2,620 μS/cm (GWM Metallurgic Waste Dump) while total dissolved solids from 245 to 2,200 mg/L. The average to very high mineral content of the waters and their chemical environment is supported by the result of water hardness. This shows that the sum of alkaline metal ions is almost exclusively bound to non-carbonate species according to the high total hardness between 9.3 and 99.8°dH with low carbonate hardness of 0.7 to 1.9°dH. It is not possible to make a clear characterization of the chemical behavior of groundwater based on the on-site parameters obtained. The high redox voltages of +393 mV to +465 mV as indicators of oxidizing conditions are mainly characterized by low oxygen concentrations between 0.46 and 9.36 mg/L O2 (≈4%–85% saturation). The ion analysis and the hydrogeochemistry of the groundwater shown in Supplementary Table S2 are characterized by cation of calcium (27–345 mg/L) and sodium (12–137 mg/L) as well as sulfate (75–1,375 mg/L) and chloride (11–180 mg/L) as anions. Further, fluoride is detected in high contents with values reaching 5.2 mg/L. The waters show average mineralization for magnesium (8–73 mg/L) and potassium (3–18 mg/L). Bicarbonate (15–45 mg/L) occurs only in low concentrations in the geogenic background of the silicate aquifer. Anthropogenic factors, especially mining activities, contribute to elevated sulfate and fluoride levels due to smelting from sulfide ores and the use of fluorspar in metallurgical processes. Landfills in the study area containing excavation rock, ashes, and slag from mining processes are closely associated with groundwater monitoring points (GWM) in and around Freiberg, emphasizing the lasting impact of mining on groundwater chemistry. Increased concentrations of ammonium (0–3.9 mg/L) and nitrate (13–53 mg/L), along with manganese (0–11 mg/L), suggest anaerobic and reducing conditions in groundwater. The predominantly negative ion balance is attributed to the absence of heavy metals, notably zinc and tin, in the chromatographic analysis.
The water quality assessment conducted in the Freiberg area reveals distinct characteristics among groundwater, surface water, and wastewater streams. To facilitate direct comparison, a hydrochemical facies classification is employed, calculating the molar fraction of major cations (Ca2+, Mg2+, Na+, K+) and anions (HCO3−, CO32−, SO42−, Cl−) in relative ratio percentages. A hydrochemical graph, following Piper (1944), visualizes equivalent ratios. The classification, based on seven defined water types or facies from existing literature (Furtak and Langguth, 1967; Rausch et al., 2023), is applied to both natural and mineral waters, with a similar technique used for wastewater. Figure 2 depicts ion equivalent ratios relative to distinct matrices in the Freiberg area. Groundwater and surface water exhibit significant levels of calcium and sulfate, indicating alkaline earth waters, while wastewater streams contain notable concentrations of sodium, potassium, sulfate, and bicarbonate, classifying them as alkaline water. Detailed evaluation assigns wastewater stream as chloride alkaline water (type G2) showing significant amounts of chloride 42%–52% meq/L (53–382 mg/L), bicarbonate 35%–39% meq/L (85–525 mg/L), and alkaline metals 51%–82% meq/L (48–443 mg/L). Surface water also shows similar hydrochemical properties as wastewater shifting to predominantly chloride alkaline-earth waters (type E2) featuring higher alkaline earth metals equivalents to be 38%–76% meq/L (21–59 mg/L) by moderate chloride and bicarbonate content around 33%–50% meq/L (24–116 mg/L) and 29%–44% meq/L (52–145 mg/L), respectively. Also, the surface water is affected by slightly increased sulfate equivalents (16%–31% meq/L~23–64 mg/L). The influence of household activities on wastewater composition impacts surface water quality, leading to imprecise disparities between the hydrochemical facies of these water matrices. Further, evaluation assigns groundwater to be sulfate alkaline-earth water (type E1), resulting in high levels of calcium (48%–58% meq/L~27–342 mg/L) and sulfate (61%–87% meq/L~75–1,375 mg/L) due to geogenic factors and mining activities. Groundwater quality is significantly impacted by these natural and anthropogenic influences, they were showcasing distinct hydrochemical characteristics compared to surface and wastewater. Both individual ion equivalent ratios and the facies clusters shown in Figure 2 indicate hydrochemical interactions. The basis of that distinct relationship is the prevalent major ions relationship (Na+, K+, Ca2+, Cl−) in the wastewater stream, originating mainly from private households. The input of these ions in the aquatic environment occurs through wastewater effluent, leading to imprecise disparities between the facies of these water matrices. The explicit effect of the wastewater matrix on surface water is evident in the transition from water type G2 to E2, attributed to higher concentrations of alkaline-earth ions in the downstream watercourses. The observed influence of reclaimed waters on specific watercourses emphasizes the connection of these water matrices and highlights the effect of human activities on water quality. Extensive dilution processes are evident from the mixing of different discharges in the Freiberger Mulde River, underscoring the complex interactions between wastewater, surface water and groundwater.
4.2 Occurrence of pharmaceuticals
4.2.1 Occurrence of pharmaceuticals within the water matrices
The collected water samples were analyzed using UHPLC-MS/MS for the occurrence of trace compounds. Almost in all the measuring points, trace PhACs are observed. Concerning the APIs, 46 of the 54 active substances analyzed were detected in the samples taken. The positive findings in the wastewater stream, surface water and groundwater are found to be 46, 37 and 19 active substances, respectively as shown in Supplementary Tables S4, S5. The contents determined vary significantly depending on the origin of the waters.
Figure 3 shows the overall concentration of PhACs found in each of the measuring points, expressed as a 10th power in the ng range, while Supplementary Tables S4, S5 show the individual and total concentration of PhACS found in each measuring point. In general, the highest levels were examined in the wastewater streams. Within the WWTP system, the total concentration of APIs was found to be around 2,399 ng/L (Central Runoff Community Hospital). After the treatment processes total contents of around 52,904 ng/L PhACs were measured at the Central Runoff, which is already several dimensions lower compared to the WWTP inflow. The discharge of the treated effluents into the Münzbach stream leads to a steady and gradual reduction in the total concentration. The WWTP effluent represents a relatively small runoff compared to the surface water discharge resulting in a volume-induced dilution of the water constituents within the receiving water. The SWM points downstream of the Central WWTP Runoff have total contents of around 17,616 ng/L (SWM 32896) and 10,289 ng/L (SWM 32900) after flow paths of 1,200 and 2,700 m, respectively. Downstream the watercourse junction of the Münzbach stream in the Freiberger Mulde River represented by a much higher discharge reduced the total concentration to around 2,630 ng/L (SWM Rothenfurth bridge) as shown in Supplementary Figure S2. While the surface water unaffected by wastewater effluent analyzed the API concentrations between 110 ng/L (SWM 32901) and 1,333 ng/L (SWM 31500) in the study area. Total contaminations of less than 100 ng/L were detected in groundwater samples. At the investigated GWM points, the total levels vary between 50 ng/L (GWM Silberhof Street) and 168 ng/L (GWM Metallurgic Waste Dump). Showcasing the antihypertensive drug atenolol (0–87 ng/L), nicotine (0–59 ng/L), caffeine (0–17.7 ng/L), antidiabetic drug metformin (0–16.4 ng/L), antiepileptic carbamazepine (0–14.5 ng/L) as well as several contrast agents were present in the groundwater.
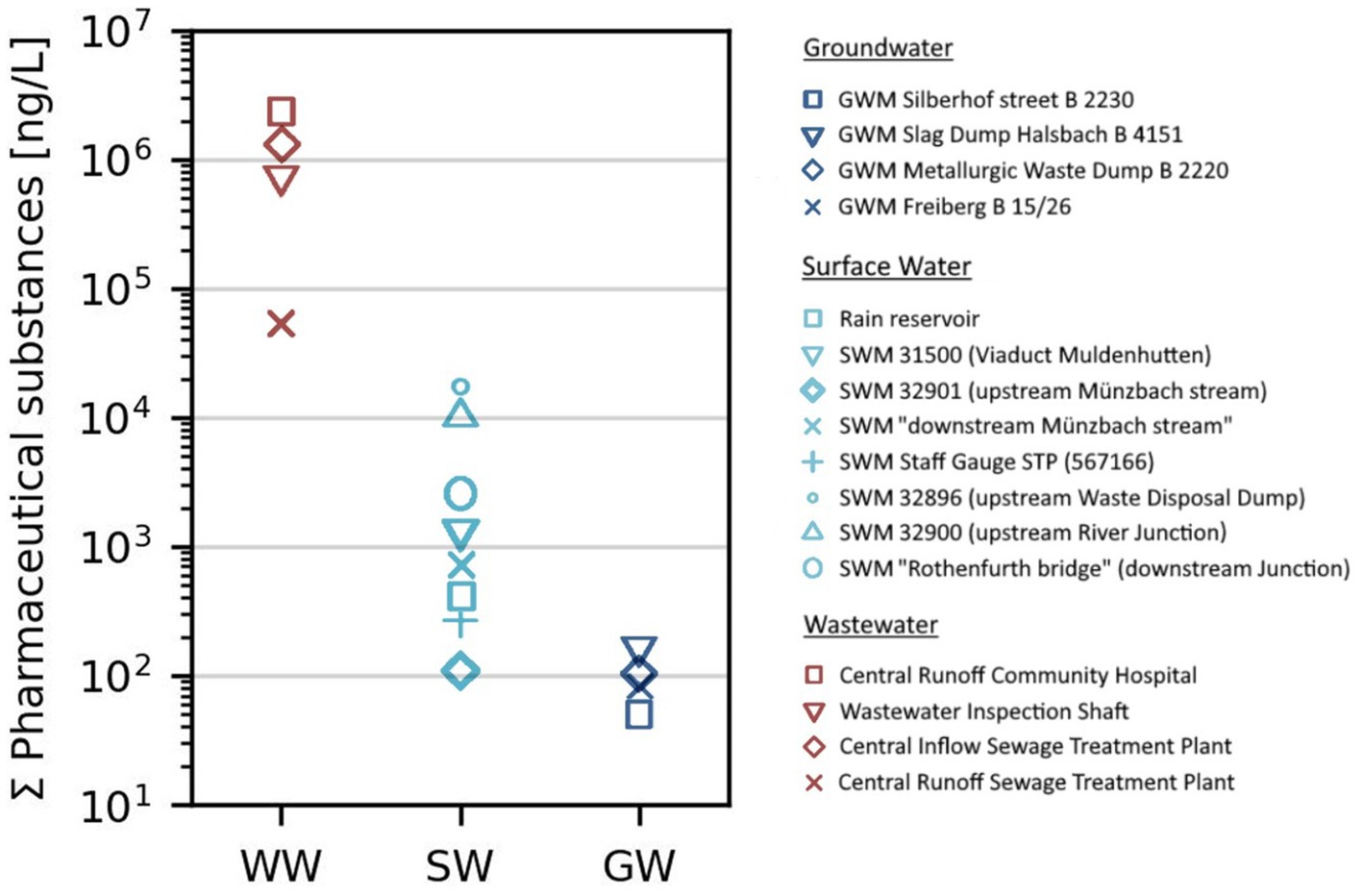
Figure 3. Total concentration of pharmaceuticals present at each measuring point, which includes wastewater (WW), surface water (SW), and groundwater (GW) selected in the Freiberg region, Germany.
Fundamentally, significant removal of trace compounds from the wastewater inflow was observed, indicating the effectivity and selectivity of the WWTP in Freiberg. However, the residues of PhACs in the wastewater effluent are still high which might be harmful to aquatic life and humans.
4.2.2 Occurrence of selected active pharmaceuticals
From the 54 total analyzed substances in total six distinct APIs were chosen to conduct a more detailed and representative evaluation of the water matrices around Freiberg. Those APIs are ATL, CBZ, CAF, DCF, MET, and SMX. The selection was made based on (i) occurrence (ii) environmental relevance and degradation of these APIs in natural systems as well as their (iii) toxic effect on humans and aquatic organisms in the environment. Furthermore, the (iv) use of prescribed medicines and over-the-counter (OTC) drugs, as far known, were also taken into account. The selected APIs are representative of distinct active ingredient groups with their physical properties shown in Table 2. Parallel to the total concentrations, significantly lower levels within the natural water matrices of the wastewater streams were detected for the selected APIs. All positive analytical results and their concentrations are compiled in Supplementary Table S6 by matrices for each substance. About the surface water matrix, the wastewater-influenced measuring points show the highest concentrations for the selected APIs. Each examined pharmaceutical substance can also be quantified in groundwater. The contamination in the groundwater matrices by the selected APIs amounts to approximately 10% of the measured concentration in wastewater without affecting the surface water. Overall, according to the positive findings, 5 of the 6 selected APIs occur in all matrices. MET and CAF can be detected in almost every water sample examined. The PhAC substances CBZ and DCF are primarily present in wastewater and surface water. ATL is rarely found in surface water and SMX was not detected in the groundwater matrix.
In the following, input and occurrence of the individual APIs are described. Figure 4 and Table 3 give an overview of each selected active pharmaceutical substance according to the water matrices given.
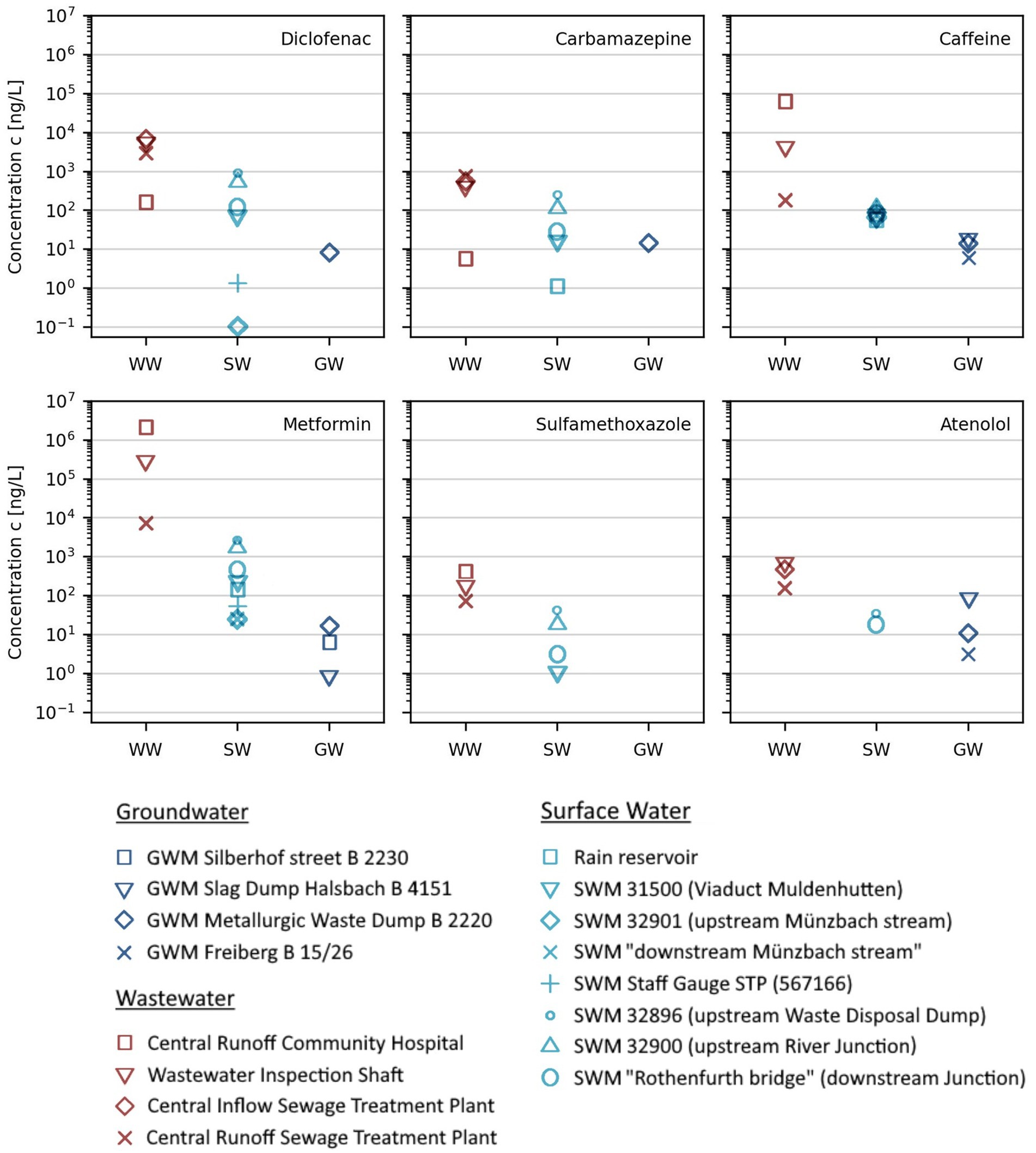
Figure 4. Concentration of selected active pharmaceuticals in wastewater (WW), surface water (SW) and groundwater (GW) matrices of Freiberg.
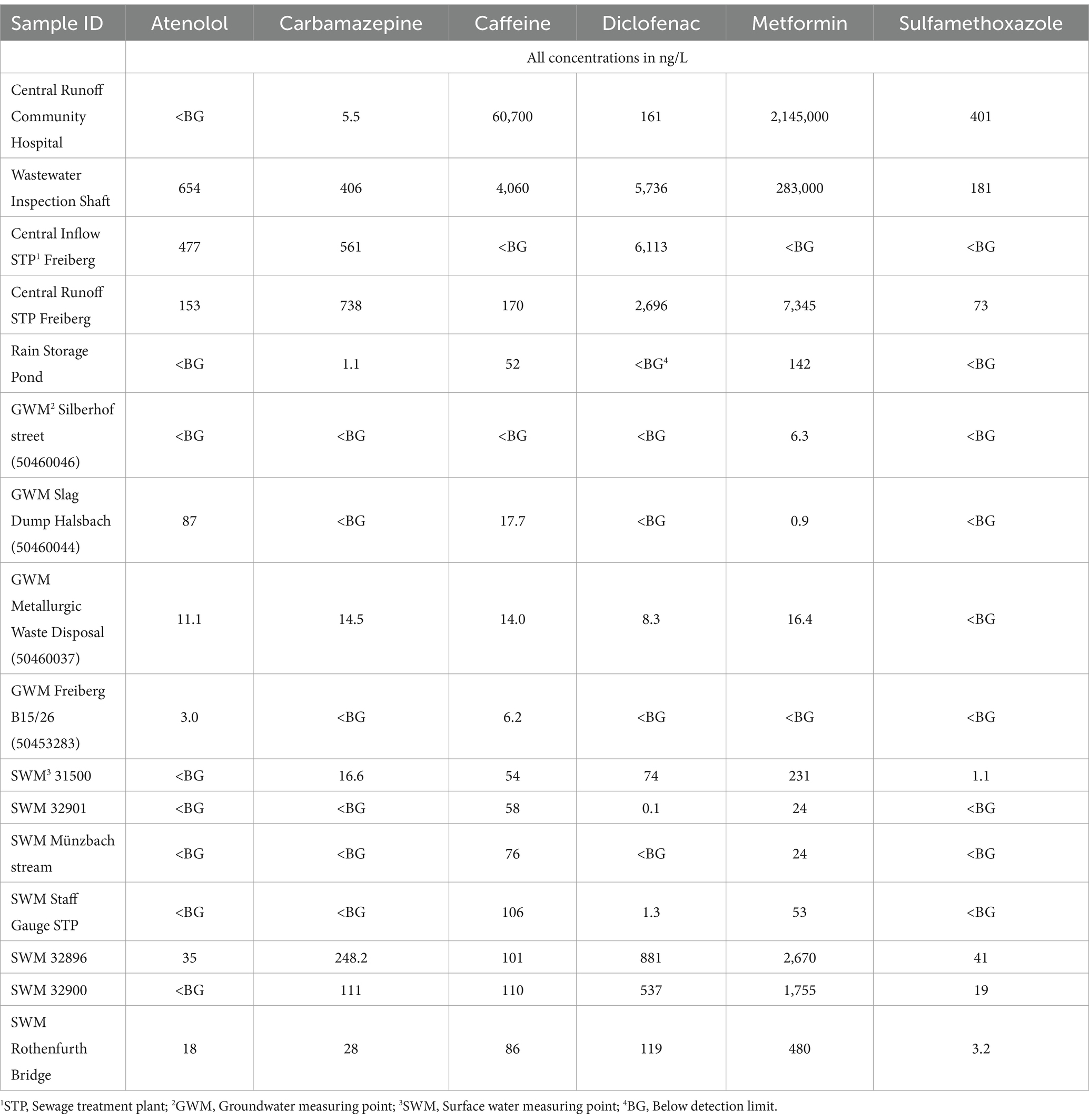
Table 3. Concentration of the selected active pharmaceuticals present in the water samples of Freiberg region.
4.2.2.1 Atenolol
ATL, a frequently prescribed antihypertensive drug from the β1—selective receptor blocker group, is mainly used to treat patients with long-term arterial hypertension. The human body excretes about 50% to 85% of ATL either in the form of its original compound or its metabolites (Rezaei et al., 2022) which further enters into the wastewater system. In the Freiberg area, ATL exhibits high levels in the sewage system (654 ng/L), contrasting with no detection in the hospital drain. The purification performance of the WWTP also shows 67% removal for ATL. The degradation of ATL is proven by the excessive occurrence of the metabolite atenololic acid in wastewater (975–183,000 ng/L) in comparison to the surface water matrix (0–980 ng/L; Supplementary Tables S4, S5; Ravichandran and Philip, 2021). The parent substance is limited to lower concentrations (0–35 ng/L) when wastewater enters the river. This is likely due to the high solubility of ATL in water that decreases the concentration of the parent compound on dilution. However, within the groundwater system, the concentration of ATL (0–87 ng/L) exceeds surface water values showing no significant relation with the contaminants in surface water.
4.2.2.2 Carbamazepine
The prescription antiepileptic drug CBZ is mainly used for psychiatric disorders, partial seizures or trigeminal neuralgia. Its adsorption in the body is very slow and enters the aquatic environment primarily by domestic wastewater (Moya-Llamas et al., 2021). This can also be observed by the detection value, which shows a high concentration of CBZ in the sewage system (406–561 ng/L) as compared to the hospital drainage (5.5 ng/L). The extended half-life (>100 days) and low biodegradability result in minimal elimination of CBZ during the treatment of wastewater. In the WWTP runoff, even higher concentrations (738 ng/L) relative to the inflow (561 ng/L) were detected. On entering the surface water, the concentration of CBZ in WWTP runoff (738 ng/L) gets diluted and decreased to 248.2 ng/L (SWM 32896) with further decreasing to 111 ng/L (SWM 32900). The leaving stream of the Freiberger Mulde affected by wastewater effluent shows a concentration of 28 ng/L while the unaffected receiving water concentration is 16.6 ng/L (SWM 31500). However, among all the groundwater matrices only one measuring point shows a CBZ concentration of 14.5 ng/L. The positive detection of CBZ in this GWM point is likely due to the transport through the hyporheic zone that connects the surface water (SWM 31500) with the groundwater.
4.2.2.3 Caffeine
CAF as an often-used freshwater marker is ubiquitously within the aquatic environment due to its high consumption rate in the form of coffee, soft drinks, tea and chocolates. These are also found as an ingredient in medication, tobacco etc. About all the intake of CAF is metabolized by the liver and around 10% is excreted out from the body (Edwards et al., 2015). High concentrations of CAF have been detected in the community hospital runoff (60,700 ng/L) and the sewer system (4,060 ng/L). The conventional wastewater treatment processes successfully remove about 95% of the CAF in wastewater. The downstream flow of Münzbach and Freiberger Mulde shows a concentration range of 86–110 ng/L, while around the city the concentration of CAF lies within the concentration range of 52 to 106 ng/L. The groundwater contains CAF at low concentrations within a range of 0–17.7 ng/L. It is observed from the data that the CAF concentration is well distributed among the surface water and groundwater of Freiberg City. This is likely due to the higher consumption of CAF among the population. Korekar et al. (2020) also observed a vast distribution of CAF in the aqueous system leading to challenges in its removal from water.
4.2.2.4 Diclofenac
DCF is used as a non-opioid analgesic, available on prescription and OTC medicine. It enters the aquatic environment primarily through wastewater from private households as about 65% of the oral dosage of DCF is released through urine along with their active metabolisms (Alessandretti et al., 2021). In this study area, domestic wastewater contributes significantly to its presence due to higher content within the sewage system (6,113 ng/L) compared to the hospital outflow (161 ng/L) as shown in Table 3. By conventional wastewater treatment, DCF concentrations are reduced by approximately 40% to 50% within the Freiberg WWTP. The occurrence of its metabolite 4-Hydroxy-DCF in wastewater (650–1,200 ng/L), as well as runoff (12–90 ng/L), confirms its degradation (Supplementary Tables S4, S5; Marco-Urrea et al., 2010). Elevated concentrations (119–881 ng/L) of DCF were detected in the downstream (119–881 ng/L) and even in the unaffected watercourses (0–74 ng/L). Similar to CBZ, the detection of DCF is also limited to one GWM point near the surface water with a concentration of 8.3 ng/L.
4.2.2.5 Metformin
The API MET is the longest-serving and most prescribed oral antidiabetic drug in the treatment of type 2 diabetes. In the study area, MET is extensively present and frequently present with CAF. Among all studied APIs in this study, MET exhibits the highest measured concentration in the water matrices. This is likely due to the consumption of MET among the older age population of the Freiberg region. The entry of MET into the Freiberg sewage system primarily occurs through hospital runoff. Notably at the hospital discharge, MET was detected in a maximum environmental concentration of several milligrams per liter (2.15 mg/L) as shown in Supplementary Table S5. Within the sewage system, strong dilution affects the API, leading to a decrease in the concentration of MET from 2.15 mg/L (Central Runoff Hospital) to 0.28 mg/L (Wastewater Inspection Shaft). Due to the very low concentrations at the central WWTP runoff (7,345 ng/L) an almost holistic elimination or degradation by conventional treatment can be suspected. The concentration of MET after WWTP discharge is decreased and observed to be around 2,670 to 480 ng/L. In addition to this significant amount of MET (231 ng/L) is also observed in upstream of the river unaffected by the WWTP. Despite having a higher concentration of MET in the surface water, a low concentration in the groundwater was detected from 0.9 to 16.4 ng/L.
4.2.2.6 Sulfamethoxazole
SMX, mainly used in veterinary medicine for treating urinary tract infections and pneumonia, shows very few positive results within the Freiberg aquatic environment. Environmental input predominantly originates from the hospital drainage (401 ng/L). The concentrations of SMX in the sewage system remain consistently lower (0–181 ng/L). Elimination rates in the Freiberg WWTP can only be estimated due to the highly variable concentrations in the wastewater stream. The lack of analyses for relevant metabolites of SMX prevents its further degradation assessment. A decrease in concentration can be stated compared to the WWTP runoff (73 ng/L). SMX was also detected in surface water affected by wastewater effluent (3.2–41 ng/L). The occurrence within the groundwater matrix could not be established.
5 Discussion
The presence of pharmaceutical residues in wastewater effluent and surface water poses a harmful impact on aquatic life and humans. Nowadays the consumption of PhACs in society has increased and some medicine shows a negative impact on their health. Further, improper collection of these medicines creates water pollution. Our WWTPs are also not designed to treat PhACs completely leaving some residues in the effluent. In most of the countries, the effluent water is discharged directly into the surface water.
This study presents a thorough investigation into the presence of APIs in the aquatic environment of a small town, such as the Freiberg region in Germany, characterized by a sparse population, minimal agriculture, and limited infrastructure. Among the 54 trace compounds analyzed, 46 PhACs were detected in the aqueous system of Freiberg. Of these, 37 were found in surface water, 19 in groundwater, and all 46 were detected in the wastewater system. The study focuses on 6 APIs—ATL, CBZ, CAF, DCF, MET, and SMX selected based on their consumption and occurrence. ATL and CBZ are likely released through wastewater effluent, with a minor contribution from hospital effluent for CBZ. The calculated elimination rates of the selected APIs vary significantly between the central inflow and runoff of the WWTP in Freiberg. CBZ shows no degradation (< 0%), whereas CAF and MET exhibit almost complete elimination (95%–97%). DCF, ATL, and SMX undergo moderate degradation (40%–67%). After treatment in the Freiberg WWTP, the former sewage waters enter the aquatic environment as reclaimed water through nearby watercourses. These compounds once released as wastewater effluent are diluted/degraded showing a comprehensive network of hydraulic pathways from wastewater and surface water to groundwater likely to form a closed loop. ATL exhibits a noteworthy presence in three groundwater samples, indicating significant transport through cracks and fissures. In contrast, minimal transport for CBZ is observed. DCF and SMX also show releases through wastewater effluent and small amounts through hospital waste, with negligible detection in groundwater. CAF is released significantly from hospital waste and wastewater effluent and is found in nearly all groundwater samples. However, the highest concentration, around milligrams per liter for the MET compound was detected in the wastewater. The reason behind the high concentration is the consumption of MET, which is primarily used to treat diabetes in Freiberg’s aging population. Entry pathways into the sewage system vary for different compounds, with domestic wastewater predominantly contributing to ATL, DCF, and CBZ. At the same time, community hospital runoff is a significant source of SMX, MET, and CAF. Compound concentrations in wastewater range from ng/L to μg/L, decreasing in surface and groundwater samples, suggesting a consistent distribution trend during flow.
This study shows that surface water is having a huge impact from wastewater effluent that is inefficient in treating PhACs completely. This leads to pollution of surface water, which is a threat to life. In our study, we observed a concentration of ng/L to μg/L of PhACs residues that will increase in the coming year if not taken into account. In the Freiberg region, we observed scattered contamination of APIs in groundwater likely due to the fractured aquifer having fewer interconnecting pores. APIs with good biodegradability and short half-lives, such as ATL, MET, and CAF, exhibit significantly higher concentrations compared to persistent compounds like CBZ and DCF. Groundwater predominantly contains individual APIs in low double-digit ng concentrations, with rapidly degradable APIs suggesting a potential inflow of freshwater into the aquifer. Potential preferential flow paths in the area include leakage within the sewage system and dynamic interactions in the hyporheic zone. Overall, we can say that the release of trace compounds is influenced by various factors, including consumption patterns, water connectivity treatment plant efficiency, and compound characteristics, necessitating further investigation into water quality parameters to comprehend the release of PhACs into the environment.
Data availability statement
The original contributions presented in the study are included in the article/Supplementary material, further inquiries can be directed to the corresponding author.
Author contributions
Dibyanshu: Writing – original draft, Writing – review & editing. MK: Data curation, Writing – review & editing. TS: Supervision, Writing – review & editing.
Funding
The author(s) declare that financial support was received for the research, authorship, and/or publication of this article. This research was funded by the Alexander von Humboldt Foundation.
Acknowledgments
The authors would like to thank all employees of the Freiberg sewage disposal services for providing data and facts about the Freiberg wastewater treatment plant. Sabine Meissner provided information regarding the groundwater measuring points from SAXONIA Site Development and Management Company mbH. We thank Wiebke Warner from the Hydrogeochemistry working group at the Ruhr University, Bochum for analyzing samples to detect PhACs and other trace compounds.
Conflict of interest
The authors declare that the research was conducted in the absence of any commercial or financial relationships that could be construed as a potential conflict of interest.
Publisher’s note
All claims expressed in this article are solely those of the authors and do not necessarily represent those of their affiliated organizations, or those of the publisher, the editors and the reviewers. Any product that may be evaluated in this article, or claim that may be made by its manufacturer, is not guaranteed or endorsed by the publisher.
Supplementary material
The Supplementary material for this article can be found online at: https://www.frontiersin.org/articles/10.3389/frwa.2024.1335766/full#supplementary-material
References
Adeleye, A. S., Xue, J., Zhao, Y., Taylor, A. A., Zenobio, J. E., Sun, Y., et al. (2022). Abundance, fate, and effects of pharmaceuticals and personal care products in aquatic environments. J. Hazard. Mater. 424:127284. doi: 10.1016/j.jhazmat.2021.127284
Alessandretti, I., Rigueto, C. V. T., Nazari, M. T., Rosseto, M., and Dettmer, A. (2021). Removal of diclofenac from wastewater: a comprehensive review of detection, characteristics and tertiary treatment techniques. J. Environ. Chem. Eng. 9:106743. doi: 10.1016/j.jece.2021.106743
Alfonso-Muniozguren, P., Serna-Galvis, E. A., Bussemaker, M., Torres-Palma, R. A., and Lee, J. (2021). A review on pharmaceuticals removal from waters by single and combined biological, membrane filtration and ultrasound systems. Ultrason. Sonochem. 76:105656. doi: 10.1016/j.ultsonch.2021.105656
Bendz, D., Paxéus, N. A., Ginn, T. R., and Loge, F. J. (2005). Occurrence and fate of pharmaceutically active compounds in the environment, a case study: Höje River in Sweden. J. Hazard. Mater. 122, 195–204. doi: 10.1016/j.jhazmat.2005.03.012
Bergmann, A., Fohrmann, R., and Weber, F. A. (2011). Zusammenstellung von Monitoringdaten zu Umweltkonzentrationen von Arzneimitteln. Texte Umweltbundesamt, p. 66. Available at: http://www.umweltbundesamt.de/sites/default/files/medien/461/publikationen/4188.pdf
BGR and SDG (2019). Hydrogeological Map of Germany 1:250,000 (HÜK250). Hanover: Federal Institute for Geosciences and Natural Resources (BGR) and German State Geological Surveys (SGD). Available at: https://services.bgr.de/grundwasser/huek250
Biswas, P., Vellanki, B. P., and Kazmi, A. A. (2022). Investigating a broad range of emerging contaminants in a set of anthropogenically impacted environmental compartments. Sci. Total Environ. 824:153757. doi: 10.1016/j.scitotenv.2022.153757
Bunting, S. Y., Lapworth, D. J., Crane, E. J., Grima-Olmedo, J., Koroša, A., Kuczyńska, A., et al. (2021). Emerging organic compounds in European groundwater. Environ. Pollut. 269:115945. doi: 10.1016/j.envpol.2020.115945
Carter, L. J., Chefetz, B., Abdeen, Z., and Boxall, A. B. (2019). Emerging investigator series: towards a framework for establishing the impacts of pharmaceuticals in wastewater irrigation systems on agro-ecosystems and human health. Environ Sci Process Impacts 21, 605–622. doi: 10.1039/C9EM00020H
Castro-Pastrana, L. I., Palacios-Rosas, E., Toledo-Wall, M. L., and Cerro-López, M. (2020). Worldwide occurrence, detection, and fate of nonsteroidal anti-inflammatory drugs in water. In: Non-Steroidal Anti-Inflammatory Drugs in Water: Emerging Contaminants and Ecological Impact. ed. L. M. Gómez-Oliván (Springer International Publishing), 55–82.
City population. (2011). Available at: https://www.citypopulation.de/en/germany/sachsen/mittelsachsen/14522180__freiberg/.
Crane, M., Watts, C., and Boucard, T. (2006). Chronic aquatic environmental risks from exposure to human pharmaceuticals. Sci. Total Environ. 367, 23–41. doi: 10.1016/j.scitotenv.2006.04.010
Destatis. (2019). Series 19—Environment Series 2.1.1—Water supply and sanitation. Public water supply and sanitation-Public water supply, 100 p., Wiesbaden: Federal Statistical Office.
Edwards, Q. A., Kulikov, S. M., and Garner-O’Neale, L. D. (2015). Caffeine in surface and wastewaters in Barbados, West Indies. Springer Plus 4, 57–12. doi: 10.1186/s40064-015-0809-x
Fricke, U., Günther, J., Niepraschk-Von Dollen, K., and Zawinell, A. (2019). “Anatomic - therapeutic - chemical classification of daily medical dose for the german pharmeceuticals market. ATC - indices with DDD - references for the german pharmeceuticals market.” Scientific Institute of AOK, 18th edition, (Berlin), 252.
Furtak, H., and Langguth, R. (1967). Zur hydrochemischen Kennzeichnung von Grundwassertypen mittels Kennzahlen. In: Memoires of the 7th International Association of Hydrogeologists, pp. 89–96, Hannover
Gilabert-Alarcón, C., Salgado-Méndez, S. O., Daesslé, L. W., Mendoza-Espinosa, L. G., and Villada-Canela, M. (2018). Regulatory challenges for the use of reclaimed water in Mexico: a case study in Baja California. Water 10:1432. doi: 10.3390/w10101432
Hamann, E., Stuyfzand, P. J., Greskowiak, J., Timmer, H., and Massmann, G. (2016). The fate of organic micropollutants during long-term/long-distance river bank filtration. Sci. Total Environ. 545-546, 629–640. doi: 10.1016/j.scitotenv.2015.12.057
Hughes, S. R., Kay, P., and Brown, L. E. (2013). Global synthesis and critical evaluation of pharmaceutical data sets collected from river systems. Environ. Sci. Technol. 47, 661–677. doi: 10.1021/es3030148
Isaac-Olivé, K., and Navarro-Frómeta, A. E. (2019). Detection of Pharmaceuticals in the Environment. Ecopharmacovigilance 66, 57–74. doi: 10.1007/698_2017_165
Jordan, H., and Weder, H. J. (1995). Hydrogeology—Fundamentals and Methods. Regional Hydrogeology: Mecklenburg—Western Pomerania, Brandenburg and Berlin, Saxony—Anhalt, Saxony, Thuringia, 2nd heavily revised and expanded edition, 603 p., Enke—Verlag, Stuttgart.
József, T., Kiss, S. R., Muzslay, F., Máté, O., Stromájer, G. P., and Stromájer-Rácz, T. (2023). Detection and quantification of pharmaceutical residues in the Pest County section of the river Danube. Water 15:1755. doi: 10.3390/w15091755
Jurado, A., Labad, F., Scheiber, L., Criollo, R., Nikolenko, O., Pérez, S., et al. (2022). Occurrence of pharmaceuticals and risk assessment in urban groundwater. Adv. Geosci. 59, 1–7. doi: 10.5194/adgeo-59-1-2022
Korekar, G., Kumar, A., and Ugale, C. (2020). Occurrence, fate, persistence and remediation of caffeine: a review. Environ. Sci. Pollut. Res. 27, 34715–34733. doi: 10.1007/s11356-019-06998-8
Kosjek, T., Heath, E., and Krbavčič, A. (2005). Determination of non-steroidal anti-inflammatory drug (NSAIDs) residues in water samples. Environ. Int. 31, 679–685. doi: 10.1016/j.envint.2004.12.001
LfULG (Landesamt für Umwelt, Landwirtschaft und Geologie): Sachsen dataset. (2022). Available at: https://www.umwelt.sachsen.de/umwelt/infosysteme/ida (Accessed 07 May 2022).
Lindberg, R. (2006). Environmental chemistry, department of Chemistry, Umeå University, Umea, Sweden. Determination of antibiotics in the Swedish environment with emphasis on Sewage Treatment Plants.
Lyu, S., Chen, W., Zhang, W., Fan, Y., and Jiao, W. (2016). Wastewater reclamation and reuse in China: opportunities and challenges. J. Environ. Sci. 39, 86–96. doi: 10.1016/j.jes.2015.11.012
Marco-Urrea, E., Pérez-Trujillo, M., Cruz-Morató, C., Caminal, G., and Vicent, T. (2010). Degradation of the drug sodium diclofenac by Trametes versicolor pellets and identification of some intermediates by NMR. J. Hazard. Mater. 176, 836–842. doi: 10.1016/j.jhazmat.2009.11.112
Mehrtens, A., Freund, W., Lüdeke, P., Licha, T., and Burke, V. (2022). Understanding flow patterns from the field–controlled laboratory experiments on the transport behavior of veterinary antibiotics in the presence of liquid manure. Sci. Total Environ. 821:153415. doi: 10.1016/j.scitotenv.2022.153415
Moya-Llamas, M. J., Trapote, A., and Prats, D. (2021). Carbamazepine removal from low-strength municipal wastewater using a combined UASB-MBR treatment system. Water Sci. Technol. 83, 1920–1931. doi: 10.2166/wst.2021.096
Olson, H., Betton, G., Robinson, D., Thomas, K., Monro, A., Kolaja, G., et al. (2000). Concordance of the toxicity of pharmaceuticals in humans and in animals. Regul. Toxicol. Pharmacol. 32, 56–67. doi: 10.1006/rtph.2000.1399
Pallares-Vega, R., Blaak, H., van der Plaats, R., de Roda Husman, A. M., Leal, L. H., van Loosdrecht, M. C., et al. (2019). Determinants of presence and removal of antibiotic resistance genes during WWTP treatment: a cross-sectional study. Water Res. 161, 319–328. doi: 10.1016/j.watres.2019.05.100
Patel, M., Kumar, R., Kishor, K., Mlsna, T., Pittman Jr, C. U., and Mohan, D. (2019). Pharmaceuticals of Emerging Concern in Aquatic Systems: Chemistry, Occurrence, Effects, and Removal Methods. Chem. Rev. 119, 3510–3673. doi: 10.1021/acs.chemrev.8b00299
Piper, A. M. (1944). A graphic procedure in the geochemical interpretation of water—analyses. EOS Trans. Am. Geophys. Union 25, 914–928. doi: 10.1029/TR025i006p00914
Ranjan, N., Singh, P. K., and Maurya, N. S. (2022). Pharmaceuticals in water as emerging pollutants for river health: a critical review under Indian conditions. Ecotoxicol. Environ. Saf. 247:114220. doi: 10.1016/j.ecoenv.2022.114220
Rausch, R., Simon, T., and Kiffer, C. (2023). The Erfurt formation: an example of aquifer genesis fissure aquifer. Groundwater. 28, 303–313. doi: 10.1007/s00767-023-00544-5
Ravichandran, M. K., and Philip, L. (2021). Insight into the uptake, fate and toxic effects of pharmaceutical compounds in two wetland plant species through hydroponics studies. Chem. Eng. J. 426:131078. doi: 10.1016/j.cej.2021.131078
Rezaei, R., Aghapour, A. A., Chavshin, A. R., and Bargeshadi, R. (2022). Biodegradation of the atenolol and nitrogen removal using the sequencing batch biofilm reactor. Bioresources 18:101109. doi: 10.1016/j.biteb.2022.101109
Rivera-Utrilla, J., Sánchez-Polo, M., Ferro-García, M. Á., Prados-Joya, G., and Ocampo-Pérez, R. (2013). Pharmaceuticals as emerging contaminants and their removal from water. A review. Chemosphere 93, 1268–1287. doi: 10.1016/j.chemosphere.2013.07.059
Rodriguez-Mozaz, S., Vaz-Moreira, I., Della Giustina, S. V., Llorca, M., Barceló, D., Schubert, S., et al. (2020). Antibiotic residues in final effluents of European wastewater treatment plants and their impact on the aquatic environment. Environ. Int. 140:105733. doi: 10.1016/j.envint.2020.105733
Samal, K., Mahapatra, S., and Ali, M. H. (2022). Pharmaceutical wastewater as emerging contaminants (EC): treatment technologies, impact on environment and human health. Energy Nexus 6:100076. doi: 10.1016/j.nexus.2022.100076
Schwabe, P., and Ludwig, W. D. (2020): Arzneiverordnungs—Report 2020, 926 pSpringer Verlag Berlin, Heidelberg.
Silori, R., Shrivastava, V., Singh, A., Sharma, P., Aouad, M., Mahlknecht, J., et al. (2022). Global groundwater vulnerability for pharmaceutical and personal care products (PPCPs): the scenario of second decade of 21st century. J. Environ. Manage. 320:115703. doi: 10.1016/j.jenvman.2022.115703
Stenuick, J. Y. (2021). Pharmaceutical residues in hospital wastewater: Five case studies from European hospitals. Health Care Without Harm. Available at: https://noharm-europe.org/sites/default/files/documents-files/6831/14-07-2021_Pharmaceutical-residues-in-hospital-wastewater-FINAL.pdf.
Tiwari, B., Sellamuthu, B., Ouarda, Y., Drogui, P., Tyagi, R. D., and Buelna, G. (2017). Review on fate and mechanism of removal of pharmaceutical pollutants from wastewater using biological approach. Bioresour. Technol. 224, 1–12. doi: 10.1016/j.biortech.2016.11.042
Veil, J. A. (2007). Use of reclaimed water for power plant cooling (No. ANL/EVS/R-07/3). Argonne National Lab. (ANL), Argonne, IL (United States).
Waleng, N. J., and Nomngongo, P. N. (2022). Occurrence of pharmaceuticals in the environmental waters: African and Asian perspectives. Environ. Chem. Ecotoxicol. 4, 50–66. doi: 10.1016/j.enceco.2021.11.002
Keywords: pharmaceuticals, trace compounds, wastewater effluent, surface water, groundwater, fractured aquifer
Citation: Dibyanshu, Kern M and Scheytt T (2024) Trace compounds in the urban water cycle in the Freiberg region, Germany. Front. Water. 6:1335766. doi: 10.3389/frwa.2024.1335766
Edited by:
Tanapon Phenrat, Naresuan University, ThailandReviewed by:
Geetanjali Shukla, Argonne National Laboratory (DOE), United StatesTarik Bahaj, Mohammed V University, Morocco
Copyright © 2024 Dibyanshu, Kern and Scheytt. This is an open-access article distributed under the terms of the Creative Commons Attribution License (CC BY). The use, distribution or reproduction in other forums is permitted, provided the original author(s) and the copyright owner(s) are credited and that the original publication in this journal is cited, in accordance with accepted academic practice. No use, distribution or reproduction is permitted which does not comply with these terms.
*Correspondence: Dibyanshu, ZGlieWFuc2h1LmRpYnlhbnNodUBleHRlcm4udHUtZnJlaWJlcmcuZGU=
†These authors have contributed equally to this work and share first authorship