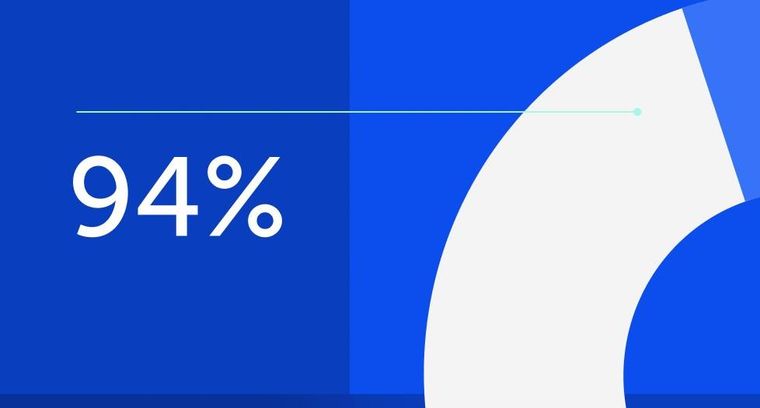
94% of researchers rate our articles as excellent or good
Learn more about the work of our research integrity team to safeguard the quality of each article we publish.
Find out more
PERSPECTIVE article
Front. Water, 29 November 2023
Sec. Environmental Water Quality
Volume 5 - 2023 | https://doi.org/10.3389/frwa.2023.1325300
This article is part of the Research TopicQuality of Water Used for Irrigation PurposesView all 4 articles
Harmful algal blooms (HABs) have garnered increasing attention due to their adverse effects on water quality, aquatic ecosystems, and animal and human health. Prior research suggests that HAB-contaminated water containing toxins can significantly affect the development of plant structures and inhibit essential physiological processes. However, the potential benefits and risks of using HAB-contaminated water sourced from local water bodies for agricultural irrigation is not completely understood. This perspective paper delves into the origins and impacts of HABs, the environmental and agricultural repercussions of their use in irrigation, and management strategies to mitigate associated risks of HAB-contaminated water in sustainable agriculture. Future studies are needed to validate the practical benefits of HABs in agricultural irrigation for the enhancement of soil health and overall crop growth and productivity.
Harmful algal blooms (HABs) are commonly perceived as a global environmental issue due to their extensive magnitude and devastating impacts on aquatic, animal, and human health. These blooms are characterized by sudden and excessive accumulations of microalgae, with cyanobacteria being a prominent and priority group (Anderson et al., 2008; Paerl and Otten, 2013). Specifically, the visibility of bloom material or the production of toxins from HAB organisms are concerning as they may bioaccumulate in aquatic organisms and contaminate drinking water consumed by animals, wildlife, and people, and inadvertently cause poisoning (Anderson et al., 2008; Iiames et al., 2021). If left untreated, HAB toxins can produce various health symptoms upon exposure including mild skin rash, throat irritation, and gastroenteritis in more severe cases (Iiames et al., 2021; Lim et al., 2023). The severity of symptoms is largely dependent on the species, type of toxin and concentration level, as well as the route and media of exposure (Svirčev et al., 2022). At the socio-economic level, HABs can cause noxious odors and unsightly views, recreational closures, pet sickness, and deteriorated aquatic conditions for organismal survival and ecosystem productivity (Carmichael and Boyer, 2016; Kourantidou et al., 2022).
As HABs continue to proliferate in local waterways, there is a growing urgency to address the challenges and opportunities associated with HAB-contaminated water use in agricultural irrigation, especially in semi-arid regions where water scarcity remains an ongoing environmental challenge (Morante-Carballo et al., 2022). As such, the utilization of alternative water sources, including reclaimed wastewater and, more recently, HAB-contaminated water, is being considered as one potential viable solution for the agricultural sector (Machado et al., 2017b; Helmecke et al., 2020). Research has indicated that HAB-contaminated water may improve the nutritional quality and growth and development of agricultural crops (Machado et al., 2017a,b). Despite these advantages, there are some limitations of using HAB-contaminated water such as impaired morphological and physiological changes in plant tissue, declined crop productivity, and groundwater contamination (Machado et al., 2017b). Consequently, these issues could present formidable challenges to agricultural workers, food security, and food safety (Machado et al., 2017b; Melaram et al., 2022).
In this perspective paper, we provide a comprehensive examination of the intricate relationship between HAB-contaminated water and agricultural irrigation. It will explore the underlying causes and consequences of HABs and discuss both the potential benefits and challenges associated with using HAB-contaminated water for irrigation. Moreover, it will delve into management strategies aimed at mitigating the risks involved and emphasize the significance of sustainable water use practices.
HABs typically arise due to nutrient pollution, elevated water temperatures, and stagnant water conditions in fresh and marine environments (Anderson et al., 2008; Rastogi et al., 2015; Birk et al., 2023). Toxic bloom formation has been documented in various freshwater sources worldwide including lakes, ponds, and rivers (Rastogi et al., 2014). It is essential to consider that HAB organisms and toxins can infiltrate both groundwater and surface water (Abesh et al., 2022). Contamination of these water bodies can be attributed to agricultural runoff, algal cell rupture during bloom senescence, and soil leaching. The far-reaching impacts of HABs encompass water quality deterioration, fish kills, animal and human illnesses, and severe economic losses, underscoring the gravity of HABs in aquatic and agricultural ecosystems (Carmichael and Boyer, 2016). Therefore, understanding the causes and consequences of HABs is crucial for addressing the complexities of contamination in agricultural settings. In the following sections, we highlight some of the major environmental and agricultural implications associated with HAB-contaminated water.
HAB-contaminated water for irrigation has profound consequences for aquatic ecosystems, creating a complex web of environmental implications ranging from nutrient loading to aquatic toxicity.
A key environmental implication of using HAB-contaminated water for irrigation is nutrient loading. Anthropogenic activities are often the culprit for nutrient loading and can include agricultural runoff, fertilizer runoff from lawns, industrial and municipal wastewater treatment plants, faulty septic tanks, and atmospheric deposition (Foulon et al., 2019). HABs often thrive in nutrient-rich environments, and the introduction of nitrogen and phosphorus into water bodies can ignite eutrophication, a process characterized by excess algal or plant overgrowth (Melaram et al., 2022). Eutrophication disrupts the natural balance of aquatic ecosystems by increasing turbidity levels and reducing light penetration and dissolved oxygen, compromising water quality and biodiversity (Anderson et al., 2008; Yang et al., 2008). Consequently, the impacts may extend to aquaculture, drinking water supplies, real estate, and water recreation (Van Dolah et al., 2016).
Environmental toxicity resulting from HAB represents another pressing concern for aquatic ecosystems. Changes in phytoplankton community composition and dominance by toxin-producing species are closely linked to the frequency and severity of HABs, with implications for the entire aquatic food web. This shift in community structure can disrupt the balance of nutrient cycling, further exacerbating eutrophication and environmental degradation. Scientific research highlights the critical need to understand these complex interactions and their cascading effects on ecosystems and human well-being (Smayda, 1997; Anderson et al., 2008; Paerl and Otten, 2013).
In summary, the environmental implications of utilizing HAB-contaminated water for agricultural irrigation are far-reaching and interconnected. The repercussions are not limited to aquatic ecosystems but extend to human activities and livelihoods closely tied to these environments. A comprehensive and ecosystem-based approach to environmental management is vital for addressing these challenges effectively. This entails proactive measures to minimize nutrient loading, mitigate eutrophication, and preserve the delicate balance of aquatic ecosystems, thereby safeguarding not only the environment but also to public health.
The utilization of HAB-contaminated water for irrigation introduces a complex interplay of challenges and potential benefits, profoundly influencing the agricultural landscape. These implications touch upon various aspects of agricultural productivity and environmental stewardship, emphasizing the intricate trade-offs that demand consideration.
Perhaps the foremost challenge arising from using HAB-contaminated water for irrigation is the risk of crop contamination (Chorus and Bartram, 1999). Because HABs can produce harmful toxins, there is a potential for these toxins to concentrate in edible crop tissue. Past studies have shown that HAB toxin uptake can hinder sucrose metabolism, photosynthesis, and seedling development (Siegl et al., 1990; Kós et al., 1995; Abe et al., 1996). Toxin absorption not only jeopardizes plant growth and development but can also pose significant health risks to animals and consumers of the food industry. Consequently, HAB-contaminated water can lead to market rejection of agricultural crops, resulting in significant economic losses for farmers (Machado et al., 2017b).
Another challenge involves the potential degradation of soil health. HAB biomass and toxins have the ability to contaminate groundwater supplies, which can subsequently transport these substances into agricultural soils (Melaram et al., 2022). This can ultimately hinder plant growth and reduce agricultural productivity. Soil health deterioration is a concern not only for the current crop cycle but also for the long-term sustainability of agricultural land (Kibblewhite et al., 2008).
Furthermore, HAB-contaminated water in agriculture can perpetuate the cycle of HABs in the environment. When HAB-contaminated water is returned to water bodies through agricultural runoff, it can introduce additional nutrients and toxins, contributing to the persistence of HABs (Anderson et al., 2008). This cyclical problem poses a significant challenge for environmental management and exacerbates the negative consequences of HABs.
Paradoxically, HAB-contaminated water can offer a benefit in the form of nutrient enrichment. Decaying HABs, for example, can alter nutrient concentrations in the form of nitrogen and phosphorus and introduce organic matter into the water column, both of which are essential for agricultural soils and crop growth (Wang et al., 2020). In some agricultural contexts where nutrient availability is limiting, HAB-contaminated water may also provide a supplementary source of nutrients, potentially improving crop yields and overall agricultural productivity (Machado et al., 2017b).
Agriculture often faces the challenge of water scarcity in arid and semi-arid regions. In these areas, HAB-contaminated water can act as an alternative water source to enhance drought resilience (El Refaey et al., 2020). This alternative water source could offer a buffer against water shortages and ensures a more reliable water supply for agriculture, especially during periods of extended drought (Ndeketeya and Dundu, 2022).
In essence, HAB-contaminated water for agricultural irrigation introduces a dynamic landscape of challenges and potential benefits. These challenges encompass crop contamination, soil health deterioration, and the perpetuation of HABs, emphasizing the importance of careful management and mitigation strategies in dependable water sources (Sukenik and Kaplan, 2021). Simultaneously, benefits such as nutrient enrichment and enhanced drought resilience highlight the potential opportunities that must be weighed against the risks. Sustainable use of HAB-contaminated water in agriculture requires a comprehensive approach that balances the potential gains with the need for environmental and public health protection.
Mitigating the risks associated with the use of HAB-contaminated water in agriculture demands a multifaceted and proactive approach. It necessitates a combination of strategies including water treatment and filtration, the implementation of early warning systems, informed crop selection and rotation, nutrient management practices to curtail runoff, and diversification of water sources (Abbas et al., 2020; Khan et al., 2021; Alotaibi et al., 2022; Fraker et al., 2023; Yan et al., 2023; Zeng et al., 2023). These comprehensive management strategies are pivotal in achieving a harmonious equilibrium between sustaining agricultural productivity and preserving our environment.
Employing advanced water treatment and filtration technologies is a primary line of defense against the perils of HAB-contaminated water (Abbas et al., 2020; Zeng et al., 2023). Advanced treatments including activated carbon, ozonation, or ultraviolet (UV)-C irradiation are effective in reducing or eliminating HAB organisms and their toxins (Pandhal et al., 2018; Abbas et al., 2020; Li et al., 2020). They can offer substantial enhancements in water quality and safety, while ensuring cost-effective measures for treating HAB toxins. In addition, these methods provide more effective and sustainable alternatives compared to traditional methods like using copper sulfate, which, while effective in killing phytoplankton and algae, can cause health hazards to other organisms and the environment (Leal et al., 2018; Mehdizadeh Allaf et al., 2023). Implementing advanced treatments at a larger scale requires extensive planning and resources, and thus represents a long-term investment for water treatment companies. In the case of ozonation and UV-C irradiation, balancing the need for effective treatment with energy efficiency is a critical challenge (Pandhal et al., 2018; Li et al., 2020). Careful planning, investment, and regulatory compliance are essential to successfully address these challenges and realize the benefits of these technologies in agriculture.
The implementation of early warning systems is crucial in monitoring the presence of HABs in water sources. These systems combine remote sensing, meteorological data, and real-time monitoring of water quality to provide timely alerts about HAB occurrences. More recently, the application of airborne data such as unmanned aerial vehicle data has demonstrated promise in HAB monitoring owing to their high-quality data, affordability, and practicality (Khan et al., 2021). By adopting this proactive approach, agricultural communities can respond promptly, making informed decisions regarding water use.
Careful consideration of crop selection and rotation is also essential in minimizing the risk of HAB contamination (Koropeckyj-Cox et al., 2021). Compared to legumes, crops such as lettuce, corn, and tomatoes exhibit greater susceptibility to HAB toxin accumulation (Saqrane et al., 2009; Melaram et al., 2022). By strategically choosing and rotating crops, farmers can reduce the potential for toxin absorption and, consequently, enhance food safety and crop viability.
To control nutrient runoff from agricultural fields, nutrient management practices are indispensable. This involves employing precision farming techniques, implementing vegetative buffer zones, and adopting controlled release fertilizers. Such practices can significantly reduce the influx of excess nutrients into water bodies, mitigating the conditions conducive to HAB development. This knowledge is pivotal for enhancing our capacity to respond to shifting climate patterns and the emergence of HABs in endemic areas.
Reducing reliance on a single water source, particularly one prone to HAB contamination, is a prudent strategy. Incorporating alternative sources, such as reclaimed wastewater or desalinated water, can enhance water security while minimizing the dependence on HAB-prone sources (Alotaibi et al., 2022; Yan et al., 2023).
Comprehensive management strategies are pivotal in addressing the multifaceted challenges posed by HAB-contaminated water in agriculture. By integrating water treatment, early warning systems, informed crop choices, nutrient management, and water source diversification, agricultural communities can effectively navigate the complex terrain of HABs. These strategies not only enhance crop productivity but also play a pivotal role in safeguarding our environment and communities.
HAB present a multifaceted challenge, profoundly affecting various societal aspects including water quality, aquatic ecosystems, crops, and human well-being. These environmental incidents are mostly caused by accumulations of microalgae, notably freshwater cyanobacteria, which can introduce both potential benefits and risks to agriculture. This duality underscores the pressing need for further research and proactive measures to safeguard the agricultural industry.
From an agricultural perspective, HABs introduce an interesting, yet an important paradox. That is, HABs feed off nitrogen and phosphorus enrichment, but can serve as a source of essential nutrients when algal cells die and rupture in local water bodies. As a result, these nutrients may vary in their concentration levels and enter the irrigated water supply to sustain crop growth and development. In regions grappling with water scarcity, the use of HAB-contaminated water has the potential to bolster agricultural production. Therefore, future research endeavors should strive to optimize HAB-contaminated water resources for irrigation while effectively mitigating associated risks.
Moreover, contamination of crops by HAB toxins poses a grave threat to food safety and public health. The excessive biomass of microalgae can detrimentally impact soil health, leading to adverse consequences for agricultural crops such as reduced growth and photosynthetic capacity. It is imperative that research delves into mechanisms of toxin accumulation in crops, soil health preservation, and strategies to disrupt the persistent cycle of highly toxic HAB occurrences in the environment. In this way, agricultural workers and higher order consumers can avoid coming into direct contact with contaminated produce, thereby preventing risk of illness and disease.
The management of HAB-contaminated water in agriculture necessitates a comprehensive approach, combining robust research with sustainable practices. Understanding the dynamics of HABs is pivotal, necessitating an exploration of shifting patterns and underlying drivers to predict and mitigate their environmental and health impacts. Simultaneously, the development of efficient water treatment technologies for HAB toxin removal is of vital importance as they contribute to potable water for drinking, recreation, and irrigation.
The study of nutrient management strategies to prevent nutrient runoff and mitigate HAB proliferation also holds significant promise. Identifying crop varieties less susceptible to HAB contamination can enhance agricultural resilience, while an enhanced focus on food safety monitoring is crucial for public health protection. This multifaceted research agenda represents the cornerstone for addressing the complex challenges presented by HABs in an agricultural context.
The future of agriculture in the face of HAB challenges hinges on a combination of research and sustainable practices that effectively mitigate their impact while safeguarding agricultural productivity as well as the health of ecosystems and humans. Achieving this balance remains an ongoing, multidisciplinary effort to ensuring agricultural resilience amid evolving environmental challenges.
The original contributions presented in the study are included in the article/supplementary material, further inquiries can be directed to the corresponding author.
AN: Conceptualization, Writing – original draft. RM: Writing – review & editing.
The author(s) declare that no financial support was received for the research, authorship, and/or publication of this article.
The authors declare that the research was conducted in the absence of any commercial or financial relationships that could be construed as a potential conflict of interest.
All claims expressed in this article are solely those of the authors and do not necessarily represent those of their affiliated organizations, or those of the publisher, the editors and the reviewers. Any product that may be evaluated in this article, or claim that may be made by its manufacturer, is not guaranteed or endorsed by the publisher.
Abbas, T., Kajjumba, G. W., Ejjada, M., Masrura, S. U., Marti, E. J., Khan, E., et al. (2020). Recent advancements in the removal of cyanotoxins from water using conventional and modified adsorbents—a contemporary review. Water 12, 2756. doi: 10.3390/w12102756
Abe, T., Lawson, T., Weyers, J. D. B., and Codd, G. A. (1996). Microcystin-LR inhibits photosynthesis of Phaseolus vulgaris primary leaves: implications for current spray irrigation practice. New Phytol. 133, 651–658. doi: 10.1111/j.1469-8137.1996.tb01934.x
Abesh, B. F., Liu, G., Vázquez-Ortega, A., Gomezdelcampo, E., and Roberts, S. (2022). Cyanotoxin transport from surface water to groundwater: Simulation scenarios for Lake Erie. J. Great Lakes Res. 48, 695–706. doi: 10.1016/j.jglr.2022.02.009
Alotaibi, G. F., Alasmari, R. S., and Alzowaid, A. N. (2022). A review of harmful algal blooms (HABs) and their potential impacts on desalination facilities. Desalination Water Treat. 252, 1–17. doi: 10.5004/dwt.2022.28280
Anderson, D. M., Burkholder, J. M., Cochlan, W. P., Glibert, P. M., Gobler, C. J., Heil, C. A., et al. (2008). Harmful algal blooms and eutrophication: Examining linkages from selected coastal regions of the United States. Harmful Algae. 8, 39–53. doi: 10.1016/j.hal.2008.08.017
Birk, S., Miller, J. D., MacMullin, A., Patterson, R. T., and Villeneuve, P. J. (2023). Perceptions of freshwater algal blooms, causes and health among new brunswick lakefront property owners. Environ. Manage. 71, 249–259. doi: 10.1007/s00267-022-01736-2
Carmichael, W. W., and Boyer, G. L. (2016). Health impacts from cyanobacteria harmful algae blooms: implications for the North American Great Lakes. Harmful Algae. 54, 194–212. doi: 10.1016/j.hal.2016.02.002
Chorus, I., and Bartram, J. (1999). Toxic Cyanobacteria in Water: A Guide to Their Public Health Consequences, Monitoring and Management. ed. EF Spon. Boca Raton, FL: CRC Press.
El Refaey, A., Amer, F. M., and Gaber, H. M. (2020). Drainage water quality enhancement for safe irrigation reuse and algal blooms mitigation. Med. J. Soil. Sci. 1, 28–43.
Foulon, E., Rousseau, A. N., Benoy, G., and North, R. L. A. (2019). global scan of how the issue of nutrient loading and harmful algal blooms is being addressed by governments, non-governmental organizations, and volunteers. Water Qual. Res. J. 55, 1–23. doi: 10.2166/wqrj.2019.013
Fraker, M. E., Aloysius, N. R., Martin, J. F., Keitzer, S. C., Dippold, D. A., Yen, H., et al. (2023). Agricultural conservation practices could help offset climate change impacts on cyanobacterial harmful algal blooms in Lake Erie. J. Great Lakes Res. 49, 209–219. doi: 10.1016/j.jglr.2022.11.009
Helmecke, M., Fries, E., and Schlute, C. (2020). Regulating water reuse for agricultural irrigation: risks related to organic micro-contaminants. Environ. Sci. Europe. 32, 1–10. doi: 10.1186/s12302-019-0283-0
Iiames, J. S., Salls, W. B., Mehaffey, M. H., Nash, M. S., Christensen, J. R., Schaeffer, B. A., et al. (2021). Modeling anthropogenic and environmental influences on freshwater harmful algal bloom development detected by MERIS over the central United States. Water Resour. Res. 57, e2020WR028946. doi: 10.1029/2020WR028946
Khan, R. M., Salehi, B., Mahdianpari, M., Mohammadimanesh, F., Mountrakis, G., Quackenbush, L. J., et al. (2021). Meta-analysis on harmful algal bloom (HAB) detection and monitoring: a remote sensing perspective. Remote Sensing. 13, 4347. doi: 10.3390/rs13214347
Kibblewhite, M. G., Ritz, K., and Swift, M. J. (2008). Soil health in agricultural systems. Philos. Trans. R. Soc. Lond. B. Biol. Sci. 363, 685–701. doi: 10.1098/rstb.2007.2178
Koropeckyj-Cox, L., Christianson, R. D., and Yuan, Y. (2021). Effectiveness of conservation crop rotation for water pollutant reduction from agricultural areas. Trans ASABE. 64, 691–704. doi: 10.13031/trans.14017
Kós, P., Gorz, G., Suranyi, G., and Borbely, G. (1995). Simple and efficient method for isolation and measurement of cyano-bacterial hepatotoxins by plant tests (Sinapis alba L). Anal. Biochem. 225, 49–53. doi: 10.1006/abio.1995.1106
Kourantidou, M., Jin, D., and Schumacker, E. J. (2022). Socioeconomic disruptions of harmful algal blooms in indigenous communities: the case of Quinault Indian nation. Harmful Algae. 118, 102316. doi: 10.1016/j.hal.2022.102316
Leal, P. R., Moschini-Carlos, V., López-Doval, J. C., Cintra, J. P., Yamamoto, J. K., Bitencourt, M. D., et al. (2018). Impact of copper sulfate application at an urban Brazilian reservoir: A geostatistical and ecotoxicological approach. Sci. Total Environ. 618, 621–634. doi: 10.1016/j.scitotenv.2017.07.095
Li, S., Tao, Y., Zhan, X. M., Dao, G. H., and Hu, H. Y. (2020). UV-C irradiation for harmful algal blooms control: A literature review on effectiveness, mechanisms, influencing factors and facilities. Sci. Total Environ. 723, 137986. doi: 10.1016/j.scitotenv.2020.137986
Lim, C. C., Yoon, J., Reynolds, K., Gerald, L. B., Ault, A. P., Heo, S., et al. (2023). Harmful algal bloom aerosols and human health. EBioMedicine. 93, 104604. doi: 10.1016/j.ebiom.2023.104604
Machado, J., Azevedo, J., Freitas, M., Pinto, E., Almeida, A., Vasconcelos, V., et al. (2017a). Analysis of the use of microcystin-contaminated water in the growth and nutritional quality of the root-vegetable, Daucus carota. Environ. Sci. Pollut. Res. Int. 24, 752–764. doi: 10.1007/s11356-016-7822-7
Machado, J., Campos, A., Vasconcelos, V., and Freitas, M. (2017b). Effects of microcystin-LR and cylindrospermopsin on plant-soil systems: a review of their relevance for agricultural plant quality and public health. Environ. Res. 153, 191–204. doi: 10.1016/j.envres.2016.09.015
Mehdizadeh Allaf, M., Erratt, K. J., and Peerhossaini, H. (2023). Comparative assessment of algaecide performance on freshwater phytoplankton: Understanding differential sensitivities to frame cyanobacteria management. Water Res. 234, 119811. doi: 10.1016/j.watres.2023.119811
Melaram, R., Newton, A. R., and Chafin, J. (2022). Microcystin contamination and toxicity: implications for agriculture and public health. Toxins. 14:350. doi: 10.3390/toxins14050350
Morante-Carballo, F., Montalván-Burbano, N., Quiñonez-Barzola, X., Jaya-Montalvo, M., and Carrión-Mero, P. (2022). What do we know about water scarcity in semi-arid zones? A global analysis and research trends. Water. 14, 2685. doi: 10.3390/w14172685
Ndeketeya, A., and Dundu, M. (2022). Alternative water sources as a pragmatic approach to improving water security. Res. Conserv. Recycl. Adv. 13, 1–10. doi: 10.1016/j.rcradv.2022.200071
Paerl, H. W., and Otten, T. G. (2013). Harmful cyanobacterial blooms: causes, consequences, and controls. Microb. Ecol. 65, 995–1010. doi: 10.1007/s00248-012-0159-y
Pandhal, J., Siswanto, A., Kuvshinov, D., Zimmerman, W. B., Lawton, L., Edwards, C., et al. (2018). Cell lysis and detoxification of cyanotoxins using a novel combination of microbubble generation and plasma microreactor technology for ozonation. Front. Microbiol. 9, 678. doi: 10.3389/fmicb.2018.00678
Rastogi, R. P., Madamwar, D., and Incharoensakdi, A. (2015). Bloom dynamics of cyanobacteria and their toxins: environmental health impacts and mitigation strategies. Front. Microbiol. 6, 1254. doi: 10.3389/fmicb.2015.01254
Rastogi, R. P., Sinha, R. P., and Incharoensakdi, A. (2014). The cyanotoxin-microcystins: current overview. Rev. Environ. Sci. Bio/Technol. 13:215–249. doi: 10.1007/s11157-014-9334-6
Saqrane, S., Ouahid, Y., Ghazali, I. E., Oudra, B., and Bouarab, L. (2009). Campo FFd. Physiological changes in Triticum durum, Zea mays, Pisum sativum and Lens esculenta cultivars, caused by irrigation with water contaminated with microcystins: a laboratory experimental approach. Toxicon. 53, 786–796. doi: 10.1016/j.toxicon.2009.01.028
Siegl, G., MacKintosh, C., and Stitt, M. (1990). Sucrose-phosphatevsynthase is dephosphorylated by protein phosphatase 2A in spinach leaves. FEBS Lett. 270, 198–202. doi: 10.1016/0014-5793(90)81267-R
Smayda, T. J. (1997). Harmful algal blooms: Their ecophysiology and general relevance to phytoplankton blooms in the sea.. Limnol. Oceanogr. 45, 1137–1153. doi: 10.4319/lo.1997.42.5_part_2.1137
Sukenik, A., and Kaplan, A. (2021). Cyanobacterial harmful algal blooms in aquatic ecosystems: a comprehensive outlook on current and emerging mitigation and control approaches. Microorganisms 9, 1472. doi: 10.3390/microorganisms9071472
Svirčev, Z., Chen, L., Sántha, K., Drobac Backovi,ć, D., Šušak, S., Vulin, A., et al. (2022). A review and assessment of cyanobacterial toxins as cardiovascular health hazards. Arch. Toxicol. 96, 2829–2863. doi: 10.1007/s00204-022-03354-7
Van Dolah, E. R., Paolisso, M., Sellner, K., and Place, A. (2016). Employing a socio-ecological systems approach to engage harmful algal bloom stakeholders. Aquatic Ecol. 50, 577–594. doi: 10.1007/s10452-015-9562-z
Wang, S., Wang, W., Chen, J., Zhang, B., Zhao, L., Jiang, X., et al. (2020). Characteristics of dissolved organic matter and its role in lake eutrophication at the early stage of algal blooms—a case study of Lake Taihu, China. Water 12:2278. doi: 10.3390/w12082278
Yan, H., Song, K., Guo, H., Shen, M., Xu, A., Zhao, L., et al. (2023). A concise way to prevent bloom risk in ecological use of reclaimed water: Determination of the threshold by model calculation. Water Cycle 4, 216–220. doi: 10.1016/j.watcyc.2023.10.002
Yang, X. E., Wu, X., Hao, H. L., and He, Z. L. (2008). Mechanisms and assessment of water eutrophication. J. Zhejiang Univ. Sci. B. 9, 197–209. doi: 10.1631/jzus.B0710626
Keywords: agriculture, irrigation, harmful algal blooms, environmental, management
Citation: Newton AR and Melaram R (2023) Harmful algal blooms in agricultural irrigation: risks, benefits, and management. Front. Water 5:1325300. doi: 10.3389/frwa.2023.1325300
Received: 21 October 2023; Accepted: 08 November 2023;
Published: 29 November 2023.
Edited by:
Petros Kokkinos, Hellenic Open University, GreeceReviewed by:
Matthew Daniel Stocker, Agricultural Research Service (USDA), United StatesCopyright © 2023 Newton and Melaram. This is an open-access article distributed under the terms of the Creative Commons Attribution License (CC BY). The use, distribution or reproduction in other forums is permitted, provided the original author(s) and the copyright owner(s) are credited and that the original publication in this journal is cited, in accordance with accepted academic practice. No use, distribution or reproduction is permitted which does not comply with these terms.
*Correspondence: Amanda Rose Newton, YW1hbmRhcm9zZW5ld3RvbkBnbWFpbC5jb20=
†These authors have contributed equally to this work
Disclaimer: All claims expressed in this article are solely those of the authors and do not necessarily represent those of their affiliated organizations, or those of the publisher, the editors and the reviewers. Any product that may be evaluated in this article or claim that may be made by its manufacturer is not guaranteed or endorsed by the publisher.
Research integrity at Frontiers
Learn more about the work of our research integrity team to safeguard the quality of each article we publish.