- 1CHN Energy Shendong Coal Group Co., Ltd., Ordos, China
- 2School of Civil Engineering, Chang'an University, Xi'an, China
Following the final biological treatment, the oil wastewater is intended for reuse in factory floor cleaning. However, the presence of varying concentrations of oil-in-iron characteristic wastewater has led to a sudden surge in sludge SV to 90%, adversely affecting water treatment efficiency. In this study, we conducted an analysis of microbial community structure and selected pepA and 16S rRNA primers to assess the proportions of zoogloea and total bacteria in sludge bulking. Iron concentration plays a pivotal role, and it should be maintained at or 0.6 mgL−1. By selective discharging of sludge to maintain 1,700 mgL−1, we minimized iron enrichment, thereby enhancing the sludge settling performance. Maintaining dissolved oxygen (DO) at 3.5 mgL−1 supports the aerobic sludge's ability to replenish iron in its system, while the oil content should be controlled at 145.33 mgL−1 to reduce the release of iron into the water. The order of significance is as follows: sludge concentration > Fe amount > DO > oil content. Implementing this approach was applied in the field for 1 week and effectively reduced the SV from 90% to approximately 43%. The interaction between quorum sensing molecules related to sludge bulking and iron, leading to the formation of complexes, underscores the significance of controlling iron levels. This study offers a valuable case for practical application of quorum quenching technology in oil wastewater, presenting a rapid, efficient, and cost-effective solution to address the issue of sludge bulking.
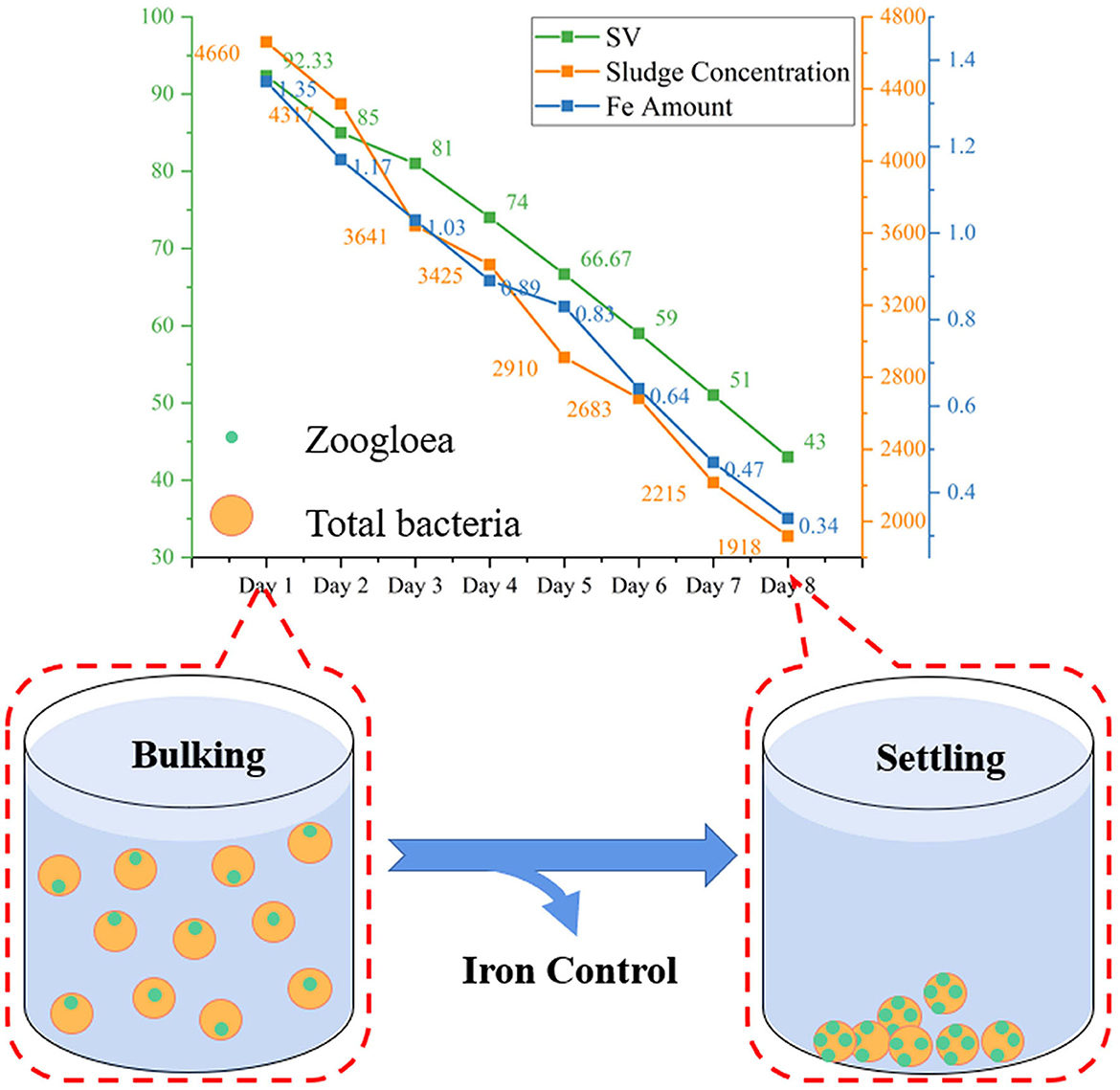
Graphical Abstract. Fe controls the reproduction of zoogloea and sludge bulking in oil-in-iron wastewate.
Highlights
• Sludge bulking was characterized by counting Zoogloea using pepA primers
• High Fe levels lead to sludge bulking and should be reduced to < 0.6 mgL−1
• Maintaining the oil content at 145.33 mgL−1 while minimizing Fe release assists in controlling sludge SV
• Selective discharge of sludge to 1,700 mgL−1 reduces iron enrichment and improves settling performance
• SV was reduced from 90% to 43% after Fe control was applied for 8 days.
1 Introduction
Oil wastewater was successively treated via separation, dissolved air flotation, and biodegradation. Subsequently, the treated was repurposed for factory floor cleaning. Nevertheless, due to the unstable quality of inflow water quality, the liquid underwent an abrupt transition from being light yellow and odorless to dark and malodorous. Simultaneously, the mixed liquid suspended solid (MLSS) content surged by 10,000 mg L−1, coinciding with an iron concentration increment of 1.0 mg L−1. This prompted the introduction of the water into the bioreactor, leading to a rapid elevation in the food-to-micro (F/M) ratio, which included heavy metals detrimental to the sludge. This resulted in a three-fold increase in sludge settling velocity (SV). Consequently, the quality of the effluent deteriorated, a situation frequently encountered in industrial wastewater treatment processes.
Sludge bulking can be attributed to several factors, including a low F/M ratio, insufficient dissolved oxygen (DO), nutrition deficiency, fatty acid supplementation, and low temperatures. These factors result in a loose floc structure in the activated sludge, a reduction in sedimentatin rate, and an increase in both MLSS and biochemical oxygen demand (BOD) in the effluent, thereby falling short of the required water standards (Li et al., 2020). Furthermore, during sludge bulking, significant variations occur in the production of soluble extracellular metabolites (SEM). The intensification of berberine and isorhamnetin within SEM synergistically contributes to the development of filamentous bulking (Li et al., 2023). Effective control of sludge bulking can be achieved by implementing substrate gradient devices in aerobic or anaerobic reactor treatment, intensifying bioreactor aeration, and supplementing essential nutrients (Tandoi et al., 2017). In addition, to control sludge bulking, chlorine, ozone, hydrogen peroxide, and flocculants are utilized to control sludge bulking. However, these methods are associated with higher costs, increased sludge volume, potential interference with nitrification, and adverse impacts on subsequent sludge performance. The installation of an anaerobic biological selector can aid in mitigating sludge bulking by creating conditions of high concentration of substrate and low oxygen levels, limiting the use of oxygen electron acceptors by filaments.
The development of sludge bulking is influenced by the selective preference of zoogloea in the sludge for substrates, leading to their preferentially while inhibiting the growth of filamentous bacteria. Consequently, it takes 22 days to rectify the bulking (Xuan et al., 2018). Moreover, when foraging for filamentous bacteria, rotifers play a pivotal role in reducing the density of Microthrix parvicella filaments and rapid multiplication. By reducing the population of filamentous bacteria, rotifers contribute to improved sludge settling properties (Fiałkowska and Pajdak-Stós, 2008). However, this approach necessitates the extraction and cultivation of considerable number of rotifers. To effectively control sludge bulking, quorum-sensing-mediated filamentation behaviors, quorum quenching technology has been developed to control sludge bulking effectively (Sharma et al., 2020). This technology disrupts cell-to-cell communication by interfering with signaling pathways and effectively inhibits growth of filamentous microorganisms (Feng et al., 2022; Lu et al., 2023b). Biophysical analysis has shown that quorum-sensing molecules form complexes with iron, leading to a 39.7% enhancement in activated sludge settleability (Diggle et al., 2007). Thus, controlling iron presents a promising avenue for the application of quorum quenching technology. However, only a limited number of sites leverage the unique characteristics of iron in wastewater to address sludge bulking control.
In the context of biological water treatment, the sludge SV witnessed a rapid surge from 27% to 90%, accompanied by a change in color changed from yellow to brown and the emergence of unpleasant odors. This led to a novel approach for harnessing the characteristics of iron in oil wastewater to control sludge bulking. The iron level, sludge concentration, DO, and the oil content were adjusted to induce sludge acclimation for SV reduction. To address this challenge, we analyzed the structure of microbial community, zoogloea and total bacteria were quantitatively measured using fluorescence, and subsequently proposed an operational scheme for effectively reducing sludge SV. This scheme was tested through an 8-day debugging process in a wastewater treatment plant (WWTP) to demonstrate its feasibility. This approach not only offers a compelling case for the application of iron-mediated quorum quenching technology but also presents a novel option for effectively controlling sludge bulking in the field.
2 Materials and methods
2.1 Materials and equipment
In the experiment, oil wastewater from a WWTP located in Erdos of China was collected and stored at 4°C prior to testing. Analytical reagent grade Ferrous gluconate (Fe≈222 mgL−1), glucose, and other chemical reagents used in the experiment were obtained from Sigma-Aldrich (MO, USA).
2.2 Investigating the impact of adjusting DAF influent and bioreactor influent on sludge SV
The WWTP was located in Erdos of China. Continuous treatment of oil wastewater was performed by separation, dissolved air flotation (DAF), and biodegradation, with DAF No. 1 and No. 2 operating in series. E.1 & 2 and E.S.1 & 2 were the effluent and effluent supernatant of DAF No. 1 and No. 2, respectively. Based on the analysis of in-situ data (see Table 1), the dissolved oxygen in the bioreactor experiment was set to three gradients. Experimental Design for Evaluating the Effects of Different DAF Effluents on Sludge SV (see Table 2)
2.3 Evaluating the influence of Fe amount, oil content, sludge concentration, and dissolved oxygen (DO) on sludge SV
(1) To obtain Fe concentrations of 0.2, 0.5, 0.8, 1.1, and 2.0 mgL−1, 50 ml of varying concentrations of ferrous gluconate were added to 100 ml of sludge respectively. (2) To obtain oil contents of 68, 145.33, 245.33, 345.33, and 445.33 mgL−1, 50 ml of different concentrations of contaminated oil were added to 100 ml of sludge respectively. (3) Glucose was added to 128, 104, 85, 68, and 55 ml of Milli-Q water, with a chemical oxygen demand (COD) of 68 mgL−1. Activated sludge was placed in a cylinder for 2 h to obtain concentrated sludge (3,880 mgL−1), and subsequently, 100 ml of sludge was mixed with the glucose solution until the concentrations reached 1,700, 1,900, 2,100, 2,300 and 2,500 mgL−1. (4) Eight liters of sludge were removed from the bioreactor. After 2 h of aeration, the DO was maintained at 6–7 mg L−1. One-liter sludge samples were extracted from the bioreactor and placed in three conical flasks. One flask was kept under continuous aeration, another was left standing to maintain the DO at 0.2–1 mgL−1, and the last flask was agitated to maintain the DO at 3–4 mgL−1.
Several experiments were conducted on the changes of SV over time, revealing that there was almost no change after 12 h. Therefore, the cultivation time was set to 12 h (see Supplementary Figure 4).The SV of the sludge was measured after the mixture was shaken at 12–13 rpm for 12 h. For gene detection, 1 ml of sludge was used. Unless otherwise specified, the sludge concentration was always approximately 2,683 mgL−1.
2.4 Analysis of microbial community structure in sludge
The second-generation gene sequencing method based on 16S rRNA was used for analyzing the microbial community structure. Samples were entrusted to Beijing Kinko Biotechnology Co for testing.
2.5 Design and performance of PepA primer for microbial community structure analysis and zoogloea proportion
The extraction of bacterial DNA was performed in accordance with the kit instructions (AG Acrey Biotechnology). As shown in Table 3, quantitative real-time polymerase chain reaction (RT-PCR) (Applied Biosystems StepOnePlus Real-Time PCR System, USA) was used for gene PCR amplification of zoogloea and total bacteria.
Zoogloea were classified into Zoogloea ramigera, Zoogloea resiniphila, and Zoogloea caeni. Zoogloea resiniphila.isolate SB3 is a representative bacterial species (An et al., 2016). PepA in the PEP-CTERM protein gene has a high level of transcription and is involved in the formation of Zoogloea resiniphila. Its expression was used to build a floc structure that allowed sludge settling (Gao et al., 2018). Therefore, the pepA gene of Zoogloea resiniphila.isolate SB3 was used in the primer design. Thus, qPCR specific primers were used to detect the expression of pepA in sludge bulking. The sequences of pepA primer are displayed in Table 4.
2.6 Study of microbial structure
To date, the 16S rRNA gene sequence, which is found in virtually all bacteria, has been the most commonly used to study bacterial phylogeny and taxonomy (Janda and Abbott, 2007). Therefore, the 16S rRNA gene was used in the primer design. The sequences of 16S rRNA primer are displayed in Table 5. In addition, the NCBI Reference Sequences of the two genes were WBTZ01000035.1 and NZ_CP032515.1:422791-425865, respectively. To confirm the reproducibility of the experimental results, all experiments were performed over three iterations. Unless otherwise stated, all SVs appearing in this article are SV30. In addition, the unit of SV is %.
3 Results and discussion
3.1 Specificity of primers designed by pepA gene
Due to the predominance of filamentous fungi and their small proporting in activated sludge, they does not exert a significant impact (Lu et al., 2023a). The bacterial community of activated sludge was analyzed using 16S rRNA. As shown in Figure 1A, the main bacteria were Betaproteobatia (15%), Gammaproteobatia (10%), and Bacteroides (7%). Deltaproteobacteria and Epsilon proteobacteria accounted for 5%, while Pedosphaera, Flavobacteria, and Alphaproteobacteria accounted for 3% of the total. Collectively, these bacteria accounted for 51% of the total population. A total of 9 representative strains were selected from each group, with Betaproteobactia including Verminephrobacter aporrectodeae subsp. tuberculatae (34%) and Zoogloea resiniphila TS9 (24%). The matching results between pepA primers and the genes of each representative strain are shown in Figure 1B. Because high-level transcription of the pepA gene was involved in the formation of Zoogloea (see details in Section 2.3), the macrogenomes of strains were compared with the pepA gene in the National Center of Biotechnology Information (NCBI) gene library. Neisseria meningitidis 1076, Rickettsia rickettsii str. Iowa, Desulfovibrio desulfuricans, Helicobacter pylori 10700, Trypanosoma grayi, Flavobacterium columnare, and Bacteroides fragilis lack the pepA gene, with completely mismatched genes. The gene annotation of Escherichia coli str. K-12 substr. MG1655 revealed the presence of a pepA gene fragment that was only 50% matched with the pepA gene of Zoogloea resiniphila TS9. Moreover, the pepA genes of the two bacteria were distantly related. Considering these aspects, this study employed the pepA gene of Zoogloea resinifila TS9 as a reference for primer design, which was specific to the genomes of other strains in the sludge. Furthermore, 16S rRNA primers were used to count the total bacterial number (Qin et al., 2022).
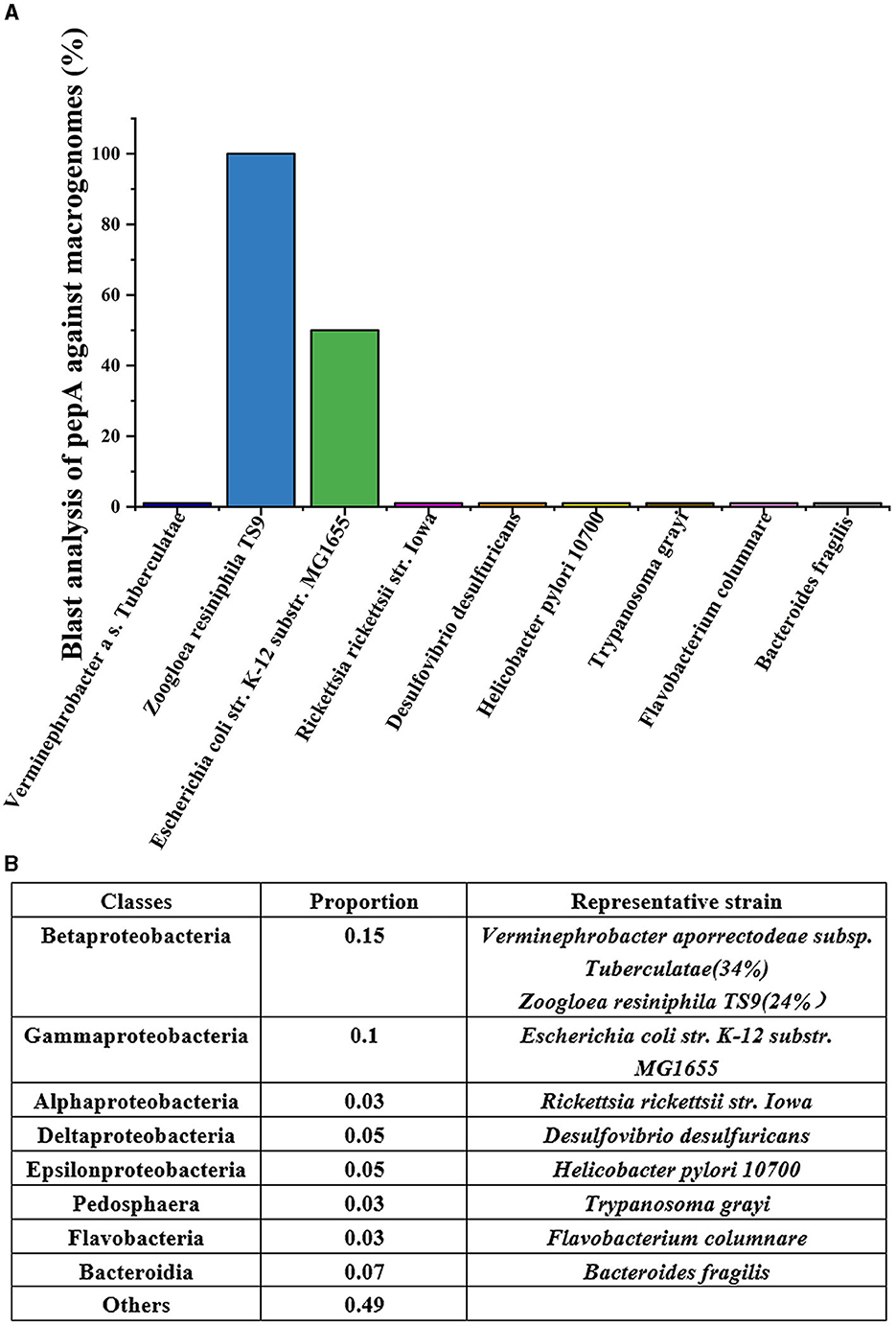
Figure 1. Matching between pepA primer and macrogenome (A) and analysis of microbial community structure of main strains in sludge (B).
3.2 Effect of sludge concentration on SV
As shown in Figure 2, the concentration of inoculated sludge decreased in the range of 1,700–2,500 mgL−1, which substantially reduced the SV. The measurement of the PCR cycle threshold (Ct) value served to indicate the number of cells, where higher cell concentrations corresponded to a lower number of required cycle thresholds. An increase in pepA Ct value indicates a decrease in pepA gene expression (Spencer et al., 2016; Rehman et al., 2022). The Ct value corresponding to the total number of bacteria increased considerably as sludge concentration decreased, whereas the Ct value corresponding to pepA decreased gradually. The standard curve of Ct value vs. cell numbers is shown in Supplementary Figure 3, based on the assumption that each cell only contains one set of pepA genes. This indicated that the number of zoogloea increased gradually, and the decrease in the number of filamentous bacteria was relatively faster, in accordance with the basic principle of the decrease in the total number of bacteria. This resulted in a reduction in sludge flocs, improved sedimentation performance, and SV recovery. The increase in sludge concentration reduced the amount of substrate available to a single bacterium, whereas filamentous bacteria have the advantage of growing at low substrate concentrations and reproducing in large numbers. Moreover, a rise in sludge causes an increase in flocs and a spike in resistance in the settling process, resulting in a decrease in sedimentation speed and an increase in SV30 (Martins et al., 2003). Thus, reducing the sludge concentration can lower the SV and improve sludge settling performance. All heavy metals are toxic to bacterial life and inhibit microbial processes at medium and high concentrations (Buaisha et al., 2020). The selective discharge of sludge reduces iron enrichment. To improve the settling performance, the sludge concentration should be controlled within the range of 1,700–2,500 mgL−1. However, the SV of normal sludge should be controlled at 15–30%, and the SV can only be adjusted to approximately 40% by regulating the sludge concentration. Because of the demand for bio-concentration for degradation, sludge cannot be discharged indefinitely, hence, alternative strategies must be determined to control the sludge SV.
3.3 Effect of influent Fe on sludge SV
Iron is essential for life and is pivotal for important metabolic reactions such as the synthesis of hemoglobin, ferrithionein, and other ferritin. A suitable quantity of Fe2+ can be employed as a bridge ion to build a network with extracellular polysaccharide polymers (Ren et al., 2018). The extracellular polymer of zoogloea contains a large number of anion groups (Dugan and Pickrum, 1972). These anion groups can combine with Fe to form complexes that can be removed (Dugan, 1987). Fe2+ is beneficial for the growth of sludge. For example, Fe is necessary for the synthesis of ferrithione and cytochrome enzymes, which are associated with electron transfer in the respiratory chain and are crucial for microbial respiration. However, excess Fe can inhibit the respiration of sludge (Ma et al., 2015) and potentially cause the destruction of sludge cell structures, flocs, and extracellular polymers (Zhang et al., 2019). According to the analysis shown in Figure 3, the Fe content increased from 0.2 to 2 mgL−1, and sludge SV increased from 31% to 41%. These values were directly proportional to one another.
The Ct value of total bacteria represented by 16S rRNA declined as Fe content rose, whereas the Ct value of zoogloea increased. This suggests that the number of filamentous bacteria gradually increased, while the number of zoogloea gradually decreased, resulting in poor sludge settling performance and increased SV. The excessive addition of heavy metal ions reduces the content of polysaccharides and proteins in sludge bacteria, resulting in a reduction in the extracellular polymeric substances (EPS) content. The abundance, richness, and diversity of microorganisms was reduced because the loss of EPS protection initiates the death and decrease of certain zoogloea (Tsai et al., 2005). Filamentous bacteria proliferated, but the relative abundance of hydrophobic amino acids used in protein synthesis was considerably reduced (Li et al., 2020). Proteins serve as protective layers on the surface. Due to the decrease in hydrophobicin and the weakening of the protective layer, a relatively substantial quantity of Fe may enter filamentous bacteria to promote growth. Sludge bulking is caused by a reduction in zoogloea bacteria and an increase in filamentous bacteria. Therefore, iron enrichment indirectly causes sludge bulking due to the inhibition of zoogloea life activities. Iron is a key component influencing activated sludge SV and its concentration should be maintained below 0.6 mgL−1.
3.4 Effect of inlet oil content and dissolved oxygen on sludge SV
As shown in Figure 4A, the oil content increased from 68 to 445.33 mgL−1, and the sludge SV was stable at approximately 32%. The lowest SV was observed at the oil content of 145.33 mgL−1. The surface volume ratio (A/V) of filamentous bacteria is greater than that of non-filamentous bacteria, offering advantages in terms of growth when the oil content is limited (Martins et al., 2003). Therefore, when the oil content was 68 mgL−1, the Ct value of pepA primer was the highest, and the corresponding number of zoogloea was the lowest. The Ct value of the 16S rRNA primer was the lowest, and the increase of total bacterial population was primarily determined by the growth of filamentous bacteria. This ultimately resulted in poor sludge settling performance. When the oil content increased to 145.33 mgL−1, the Ct value of pepA primer decreased slightly, indicating that the zoogloea grew marginally. The Ct value of the 16S rRNA primer increased rapidly, and the reduction of the total bacterial population was dependent on the slow growth of filamentous bacteria. However, the bacteria fed with high substrate concentration exhibit a high saturation constant Ks and a maximum growth rate μmax (Chudoba et al., 1973). When the oil content exceeds 145.33 mgL−1, previous experiments have shown that more iron is released into water (Qin et al., 2023). This may be the reason for inducing a decrease in total cell number and a slight increase in the number of zoogloea. The SV was slightly higher than 30%, suggesting a slight viscous bulking. This is because a large number of hydrophilic and highly viscous substances are produced by the proliferation of zoogloea. Microbes absorb a wide range of organic substances but are unable to metabolize them. A large number of highly viscous polysaccharides accumulate outside the cells, causing a rapid increase in cell surface attachments and making it difficult to settle, resulting in viscous bulking (Shi et al., 2022). Thus, the oil acts as a high concentration matrix that may lead to sludge viscous bulking.
As shown in Figure 4B, sludge SV decreased initially and then increased when DO increased from 0.6 to 6.5 mgL−1. The decline in the Ct value of zoogloea was greater than that of total bacteria, indicating that both populations were proliferating, and zoogloea might be the primary driver of total bacteria growth. The number of sludge bacteria peaked at 6.5 mgL−1 DO. Although low DO inhibited the growth of microorganisms, filamentous bacteria easily absorbed DO due to their large surface area and growth advantages (Lau et al., 1984; Martins et al., 2003). Sludge with DO < 1.5 mgL−1 did not flocculate and exhibited poor sedimentation performance. Sludge with DO > 2 mgL−1 had a large particle size and optimal sedimentation performance (Sürücü and Çetin, 1990). Notably, a DO of 3.5 mgL−1 is beneficial for aerobic sludge to update iron in the body (Chen et al., 2018). To offer the sludge microbes a growth advantage, the DO must be enhanced. Therefore, the minimum SV was 40% when the DO was 3.5 mgL−1. However, excess DO indicates strong aeration and flow shear force, which operate on the larger flocs in the sludge to cause its collapse, decrease the sedimentation performance, and increase the SV. In summary, maintaining the oil concentration at 145.33 mgL−1 and the DO at 3.5 mgL−1 was conducive to controlling the sludge SV.
3.5 Correlation analysis between SV and Fe content, maintenance oil content, sludge concentration, and dissolved oxygen (DO)
The closer the absolute value of the coefficient is to 1, the stronger the correlation (Table 6). Therefore, SV was strongly correlated with the Fe and sludge concentrations, moderately correlated with DO, and weakly correlated with oil content. The order of correlation with SV was as follows: sludge concentration > Fe amount > DO > oil content.
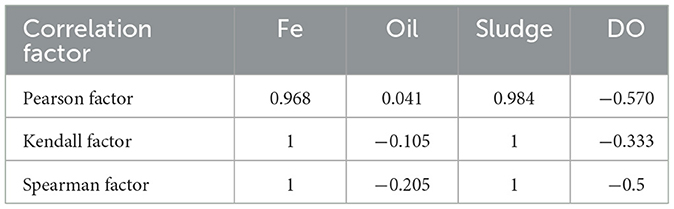
Table 6. Correlation analysis between SV and Fe amount, maintenance oil content, sludge concentration, dissolved oxygen (DO).
3.6 Practical application of sludge SV control by the method
Adopting the principles of sludge SV management outlined herein, the operation effect in the WWTP is depicted in Figure 5. Figure 5A shows the continuous treatment of wastewater with an MLSS of 13,080 mgL−1 by DAF No. 1 and No. 2. The effluent supernatant was used for sludge culture for 12 h, and its SV ranged between 30 and 35%. The SV of sludge cultured in MilliQ water was 30% with minimal variation. However, the SV of sludge cultured in effluent treated by air floaters No. 1 and No. 2 rose dramatically, reaching 56.33% and 41.33%, respectively. In summary, influent water quality, environmental factors, and operation can all contribute to sludge bulking (Guo et al., 2014). The MLSS of the effluent from the floater impacts the sludge SV. Due to the excessive levels of heavy metal ions, such as iron ions, in oil wastewater, it affects the formation of sludge. Moreover, the SV of effluent sludge from air floaters 1 and 2 increased by 21% and 11%, respectively. The water from floater 1 had a greater capacity to improve SV than that from floater 2. In addition, a low F/M ratio, high or low DO, suspended residence time (SRT), carbon to nitrogen ratio (C/N), pH, sludge concentration, and other factors in sludge culture contribute to bulking (Zaidi et al., 2022). Because floaters 1 and 2 were linked in series, the Fe and COD concentrations in the No. 2 air floater influent were lower than those of the No. 1 floater. However, the No. 2 floater exhibited a more visible control of SV change; hence, we believe that Fe concentration played a major role in sludge bulking.
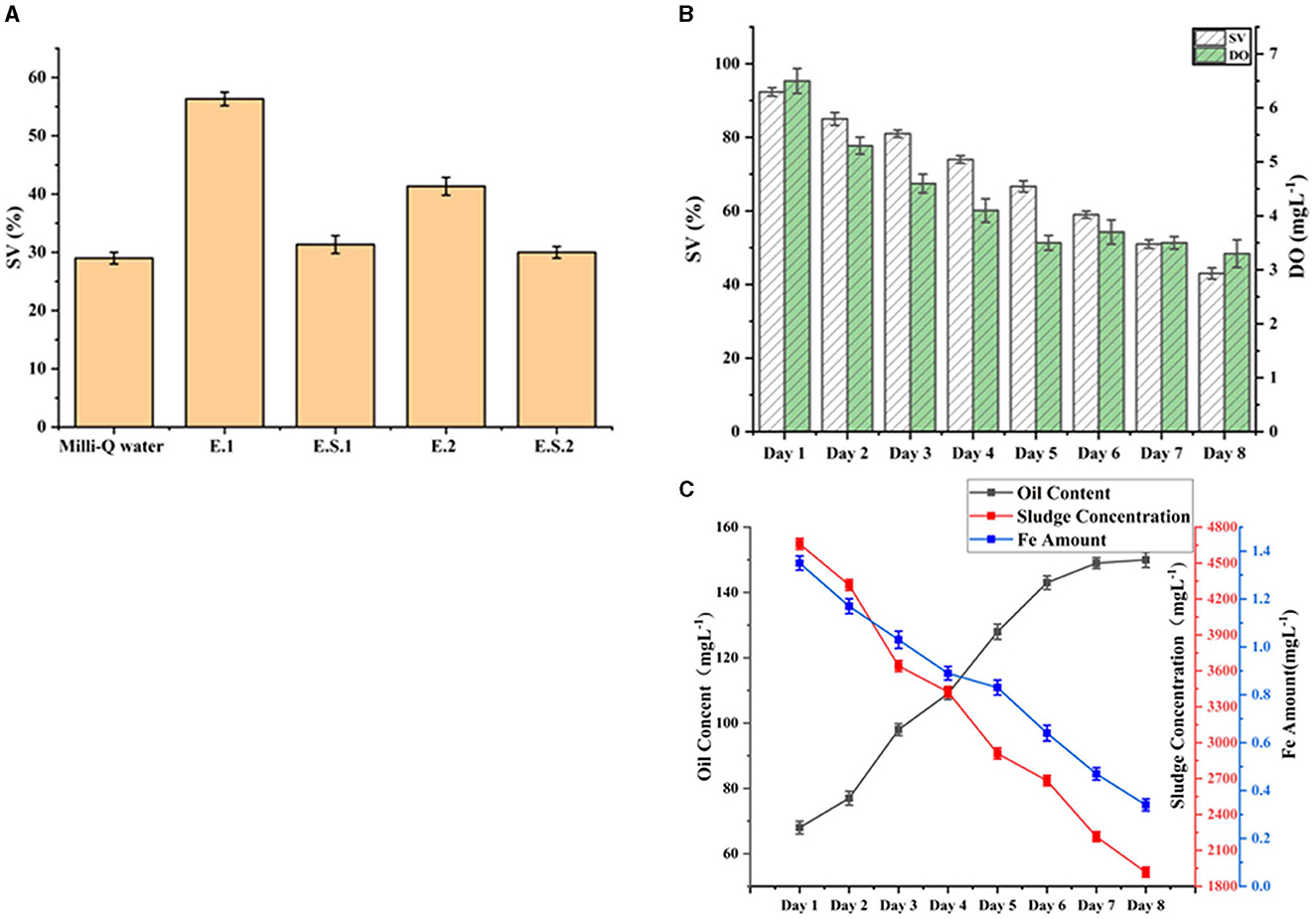
Figure 5. Practical application of sludge SV control (B, C) by the method. (A) Is the effect of different influent water on sludge SV.
As shown in Figures 5B, C, the influent sludge, Fe content, and DO were all controlled for 8 consecutive days. The sludge concentration decreased from 4,660 to 1,918 mgL−1, the Fe concentration decreased from 1.35 to 0.34 mgL−1 and the DO decreased from 6.5 to 3.3 mgL−1. However, the oil content increased from 68 to 150 mgL−1. As previously stated, the oil was a high-concentration substrate that had minimal impact on the control of sludge SV. The color of the sludge changed from black to yellow and the odor disappeared. The SV decreased by 5–8% every day, and from 92% to 43% during the 8 days.
The results show that high Fe leads to sludge bulking, and reducing Fe concentration to less than 0.6 mgL−1, selectively discharging sludge to maintain concentration at around 1,700 mgL−1, and controlling oil content and DO at about 145.33 mgL−1 and 3.5 mgL−1, respectively, is the most effective and rapid way to prevent sludge from bulking.
4 Conclusion
We discovered that susceptibility of oil wastewater to sludge bulking was influenced by changes in the Fe quantity, oil content, sludge concentration, and DO in the influent. These factors affects not only the quality of the effluent but also its suitability for reuse in the factory washing processes. In this study, the pepA gene of Zoogloea resinifila TS9 was used as a reference for primer design, ensuring specificity to the genomes of other strains present in the sludge. Moreover, 16S rRNA primers were used to measure the total number of bacteria. Generally, a reduction in sludge concentration leads to decreased SV and improved sludge settling performance. We suggest that sludge concentrations should be maintained within the range of 1,700–2,500 mgL−1. However, this approach can only control the SV to approximately 40%. Because of the demand for bio-concentration for degradation, sludge cannot be discharged indefinitely, alternative strategies must be identified to control the sludge SV.
The Fe concentration is a key factor in sludge SV and should be maintained under 0.6 mgL−1. Moreover, oil, owing to its high-concentration matrix, exerts a noticeable impact on sludge SV control, inevitably leading to sludge viscous bulking. The presence of 145.33 mgL−1 of oil restricted the release of iron into water, while maintaining a DO level of at 3.5 mgL−1 was conducive for the aerobic sludge to refresh iron in its system. Both methods were deemed for controlling sludge SV. The following was the order of correlation with SV: sludge concentration > Fe amount > DO > maintenance oil content. High Fe induces sludge bulking, considering this aspect, the controlling method was designed to minimize the Fe content to less than 0.6 mgL−1, selectively discharge the sludge to maintain the concentration around 1,700 mgL−1, and control the oil content and DO to approximately 145.33 mgL−1 and 3.5 mgL−1, respectively. The field application lasted approximately 8 days, and SV was reduced from 90% to approximately 43%, with a daily decline rate of up to 5–8%, for a total reduction of more than 50%.
Based on the formation of complexes between quorum sensing molecules and Fe, the application of controlling Fe in oil wastewater plays an important role in inhibiting sludge bulking. Further studies on the interaction between Fe and quorum sensing molecules to control sludge bulking are needed. This research provides a case study for the future application of quorum sensing technology, offering a fast, efficient, and low-cost superior solution to the problem of sludge bulking in oil wastewater.
Data availability statement
The datasets presented in this study can be found in online repositories. The names of the repository/repositories and accession number(s) can be found in the article/Supplementary material.
Author contributions
XS: Conceptualization, Validation, Writing – original draft. ZS: Methodology, Writing – original draft. XT: Conceptualization, Writing – original draft. XZ: Formal analysis, Software, Writing – original draft. JZ: Formal analysis, Software, Writing – review & editing. RW: Formal analysis, Software, Writing – review & editing. JQ: Conceptualization, Writing – review & editing.
Funding
The author(s) declare financial support was received for the research, authorship, and/or publication of this article. This work was financially supported by the Natural Science Foundation of China (Grant No. 51808044), Key R&D Program of Shaanxi Province, China (2022KXJ-119), Shendong Coal Branch Technology Innovation Project of China Shenhua Energy Co., Ltd. (Grant No. CEZB210304069), and the Fundamental Research Funds for the Central Universities, CHD (Grant No. 300102281502).
Conflict of interest
XS, ZS, XT, and XZ were employed by CHN Energy Shendong Coal Group Co., Ltd.
The remaining authors declare that the research was conducted in the absence of any commercial or financial relationships that could be construed as a potential conflict of interest.
Publisher's note
All claims expressed in this article are solely those of the authors and do not necessarily represent those of their affiliated organizations, or those of the publisher, the editors and the reviewers. Any product that may be evaluated in this article, or claim that may be made by its manufacturer, is not guaranteed or endorsed by the publisher.
Supplementary material
The Supplementary Material for this article can be found online at: https://www.frontiersin.org/articles/10.3389/frwa.2023.1289276/full#supplementary-material
References
An, W., Guo, F., Song, Y., Gao, N., Bai, S., Dai, J., et al. (2016). Comparative genomics analyses on EPS biosynthesis genes required for floc formation of Zoogloea resiniphila and other activated sludge bacteria. Water Res. 102, 494–504. doi: 10.1016/j.watres.2016.06.058
Buaisha, M., Balku, S., and Yaman, E. Z. (2020). Heavy metal removal investigation in conventional activated sludge systems. Civil Eng. J. 6, 470–477. doi: 10.28991/cej-2020-03091484
Chen, H., Zhao, X., Cheng, Y., Jiang, M., Li, X., and Xue, G. (2018). Iron robustly stimulates simultaneous nitrification and denitrification under aerobic conditions. Environ. Sci. Technol. 52, 1404–1412. doi: 10.1021/acs.est.7b04751
Chudoba, J., Grau, P., and Ottova, V. (1973). Control of activated-sludge filamentous bulking-II. Selection of microorganisms by means of a selector. Water Res. 7, 1389–1406. doi: 10.1016/0043-1354(73)90113-9
Diggle, S. P., Matthijs, S., Wright, V. J., Fletcher, M. P., Chhabra, S. R., Lamont, I. L., et al. (2007). The Pseudomonas aeruginosa 4-quinolone signal molecules HHQ and PQS play multifunctional roles in quorum sensing and iron entrapment. Chem. Biol. 14, 87–96. doi: 10.1016/j.chembiol.2006.11.014
Dugan, P. (1987). The Function of Microbial Polysaccharides in Bioflocculation and Biosorption of Mineral Ions. Amsterdam: Elsevier Science.
Dugan, P., and Pickrum, H. (1972). Removal of mineral ions from water by microbially produced polymers. Purdue Univ. Eng. Ext. Ser. Eng. Bull 141, 1019–1038.
Feng, Z., Lu, X., Chen, C., Huo, Y., and Zhou, D. (2022). Transboundary intercellular communications between Penicillium and bacterial communities during sludge bulking: inspirations on quenching fungal dominance. Water Res. 221, 118829. doi: 10.1016/j.watres.2022.118829
Fiałkowska, E., and Pajdak-Stós, A. (2008). The role of Lecane rotifers in activated sludge bulking control. Water Res. 42, 2483–2490. doi: 10.1016/j.watres.2008.02.001
Gao, N., Xia, M., Dai, J., Yu, D., An, W., Li, S., et al. (2018). Both widespread PEP-CTERM proteins and exopolysaccharides are required for floc formation of Zoogloea resiniphila and other activated sludge bacteria. Environ. Microbiol. 20, 1677–1692. doi: 10.1111/1462-2920.14080
Guo, J., Peng, Y., Wang, S., Yang, X., and Yuan, Z. (2014). Filamentous and non-filamentous bulking of activated sludge encountered under nutrients limitation or deficiency conditions. Chem. Eng. J. 255, 453–461. doi: 10.1016/j.cej.2014.06.075
Janda, J. M., and Abbott, S. L. (2007). 16S rRNA gene sequencing for bacterial identification in the diagnostic laboratory: pluses, perils, and pitfalls. J. Clin. Microbiol. 45, 2761–2764. doi: 10.1128/JCM.01228-07
Lau, A. O., Strom, P. F., and Jenkins, D. (1984). The competitive growth of floc-forming and filamentous bacteria: a model for activated sludge bulking. J. Water Pollut. Control Federat. 56, 52–61.
Li, W.-M., Liao, X.-W., Guo, J.-S., Zhang, Y.-X., Chen, Y.-P., Fang, F., et al. (2020). New insights into filamentous sludge bulking: the potential role of extracellular polymeric substances in sludge bulking in the activated sludge process. Chemosphere 248, 126012. doi: 10.1016/j.chemosphere.2020.126012
Li, Z., Cun, S., Han, G., Guo, X., Liu, B., Huang, T., et al. (2023). New insight into soluble extracellular metabolites during sludge bulking process based on excitation-emission matrix spectroscopy and ultrahigh-performance liquid chromatography-mass spectrometry. Environ. Res. 219, 115161. doi: 10.1016/j.envres.2022.115161
Lu, X., Feng, Z., Cui, B., and Zhou, D. (2023a). Low pH induces simultaneous fungal bulking and nitrogen removal deterioration in an activated sludge reactor: mechanisms based on microbial transboundary coevolution. J. Clean. Product. 389, 136047. doi: 10.1016/j.jclepro.2023.136047
Lu, X., Yan, G., Fu, L., Cui, B., Wang, J., and Zhou, D. (2023b). A review of filamentous sludge bulking controls from conventional methods to emerging quorum quenching strategies. Water Res. 236, 119922. doi: 10.1016/j.watres.2023.119922
Ma, S., Zeng, S., Dong, X., Chen, J., and Olsson, G. (2015). Modification of the activated sludge model for chemical dosage. Front. Environ. Sci. Eng. 9, 694–701. doi: 10.1007/s11783-014-0732-3
Martins, A. M., Heijnen, J. J., and Van Loosdrecht, M. C. (2003). Effect of feeding pattern and storage on the sludge settleability under aerobic conditions. Water Res. 37, 2555–2570. doi: 10.1016/S0043-1354(03)00070-8
Qin, J., Fang, J., Gao, F., He, Y., Su, M., Zhang, Y., et al. (2022). Oriented bio-feeding control of the anaerobic biodegradation of ethinyl estradiol. Chemosphere 311(Pt 1),137007. doi: 10.2139/ssrn.4102662
Qin, J., He, Y., Shangguan, B., Wang, R., Wang, X.-H., Qin, C., et al. (2023). Treating mechanical washing wastewater with iron-in-oil characteristics by changing the fate of iron. Water Sci. Technol. 87, 866–878. doi: 10.2166/wst.2023.024
Rehman, M. U., Naqvi, S. S., Ullah, R., Arshad, N., Ammad, M., Ain, Q. U., et al. (2022). Elucidation of correlation between SARS-CoV-2 RdRp and N gene cycle threshold (Ct) by RT-PCR with age and gender. Clin. Chim. Acta 533, 42–47. doi: 10.1016/j.cca.2022.06.008
Ren, X., Chen, Y., Guo, L., She, Z., Gao, M., Zhao, Y., et al. (2018). The influence of Fe2+, Fe3+ and magnet powder (Fe3O4) on aerobic granulation and their mechanisms. Ecotoxicol. Environ. Saf. 164, 1–11. doi: 10.1016/j.ecoenv.2018.07.072
Sharma, A., Singh, P., Sarmah, B. K., and Nandi, S. P. (2020). Quorum sensing: its role in microbial social networking. Res. Microbiol. 171, 159–164. doi: 10.1016/j.resmic.2020.06.003
Shi, H.-X., Wang, J., Liu, S.-Y., Guo, J.-S., Fang, F., Chen, Y.-P., et al. (2022). Potential role of AHL-mediated quorum sensing in inducing non-filamentous sludge bulking under high organic loading. Chem. Eng. J. 454, 140514. doi: 10.1016/j.cej.2022.140514
Spencer, S., Chung, J., Thompson, M., Piedra, P. A., Jewell, A., Avadhanula, V., et al. (2016). Factors associated with real-time RT-PCR cycle threshold values among medically attended influenza episodes. J. Med. Virol. 88, 719–723. doi: 10.1002/jmv.24373
Sürücü, G., and Çetin, F. D. (1990). Effects of temperature, pH and DO concentration on settleability of activated sludge. Environ. Technol. 11, 205–212. doi: 10.1080/09593339009384858
Tandoi, V., Rossetti, S., and Wanner, J. (2017). Activated Sludge Separation Problems: Theory, Control Measures, Practical Experiences. IWA Publishing.
Tsai, Y.-P., You, S.-J., Pai, T.-Y., and Chen, K.-W. (2005). Effect of cadmium on composition and diversity of bacterial communities in activated sludges. Int. Biodeteriorat. Biodegrad. 55, 285–291. doi: 10.1016/j.ibiod.2005.03.005
Xuan, X., Zhang, L., Zhao, J., Wang, Z., Cheng, Y., and Long, B. (2018). Remediation of filamentous bulking granule and its substrate degradation kinetics. Chem. Indus. Eng. Prog. 37, 3245–3251.
Zaidi, N. S., Syafiuddin, A., Sillanpää, M., Bahrodin, M. B., Zhan, L. Z., Ratnasari, A., et al. (2022). Insights into the potential application of magnetic field in controlling sludge bulking and foaming: a review. Bioresour. Technol. 358, 127416. doi: 10.1016/j.biortech.2022.127416
Keywords: sludge bulking, settling velocity, Fe amount, zoogloea, dissolved oxygen
Citation: Shi X, Su Z, Tao X, Zhou X, Zhao J, Wang R and Qin J (2023) Fe controls the reproduction of zoogloea and sludge bulking in oil-in-iron wastewater. Front. Water 5:1289276. doi: 10.3389/frwa.2023.1289276
Received: 05 September 2023; Accepted: 28 November 2023;
Published: 20 December 2023.
Edited by:
Yiqi Liu, South China University of Technology, ChinaReviewed by:
Duoying Zhang, Heilongjiang University, ChinaChongjun Chen, Suzhou University of Science and Technology, China
Copyright © 2023 Shi, Su, Tao, Zhou, Zhao, Wang and Qin. This is an open-access article distributed under the terms of the Creative Commons Attribution License (CC BY). The use, distribution or reproduction in other forums is permitted, provided the original author(s) and the copyright owner(s) are credited and that the original publication in this journal is cited, in accordance with accepted academic practice. No use, distribution or reproduction is permitted which does not comply with these terms.
*Correspondence: Jinyi Qin, amlueWkucWluJiN4MDAwNDA7Y2hkLmVkdS5jbg==