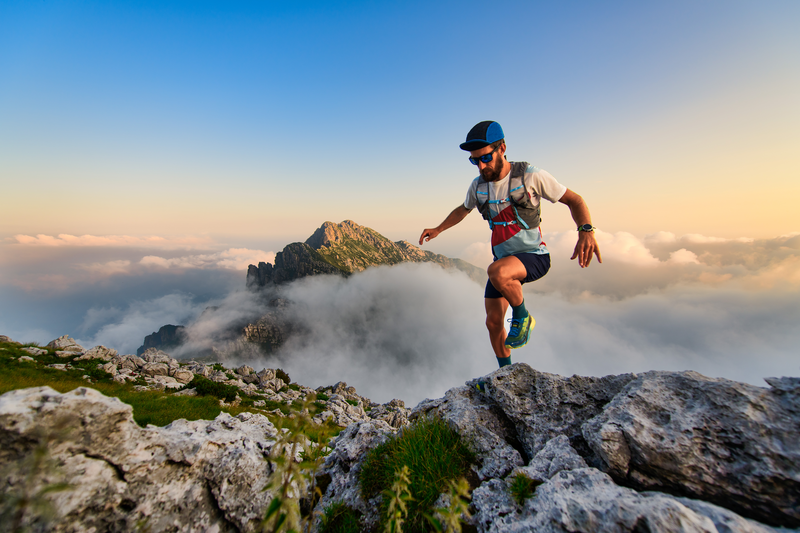
94% of researchers rate our articles as excellent or good
Learn more about the work of our research integrity team to safeguard the quality of each article we publish.
Find out more
ORIGINAL RESEARCH article
Front. Water , 01 September 2023
Sec. Water and Climate
Volume 5 - 2023 | https://doi.org/10.3389/frwa.2023.1245207
This article is part of the Research Topic Climate Strategies and Deep Uncertainty View all 11 articles
The Galapagos Islands, a hotspot of ecological richness, face challenging climatic and development conditions which undermine regional water security. Yet, the way by which these conditions may change in the future is highly uncertain. In this study, we applied for the first time an uncertainty-based approach in the Galapagos Islands to understand thresholds and potential scenarios of risks to water security of agricultural catchments in the Galapagos Island. We applied a water systems model to the agricultural catchments as well as climate and land use scenarios to estimate physical changes in water availability and implications for drought and extreme flow thresholds. Our results highlight the key role of baseflow and its important sensitivity to precipitation changes in determining water security states in the Islands. In fact, a decrease in just about 25% of total water flows, from historical conditions, in the Islands would drive drought conditions resembling those of the emergency state of 2016. We also note that under a land use scenario which promotes sustainable practices, the robustness of the Islands to climate variations increases. Our study then provides the basis for an application of uncertainty-based approaches to enhance resilience of the agricultural water systems as well as other systems. Our results also emphasize the need to design flexible and comprehensive policies in the water, agricultural and interconnected sectors which consider the interlinkages of climate with other social and economic variables.
The Galapagos Islands are a global hotspot of ecological, biodiversity, and evolutionary richness. Indeed, their role in understanding evolutionary and global change dynamics has become critical during the Anthropocene (Trueman and d'Ozouville, 2010; Walsh and Mena, 2013). The relevant role of the Islands, owes, among others, to their location about 1,000 km off the coast of Ecuador where a complex interplay of ocean currents and winds influence their climate. These mechanisms are mainly governed by interactions of the Inter-Tropical Convergence Zone (ITCZ) as well as El Niño Southern Oscillation (ENSO) (Houvenaghel, 1974; Chavez and Brusca, 1991; Sachs and Ladd, 2010).
The Galapagos consist of 13 major islands. Of these ones, the largest ones and mainly populated are Santa Cruz, San Cristobal, and Isabela. Yet, as result of their climatology and complex geography, overall the Islands face challenging hydroclimatological conditions. For instance, mean annual precipitation between 1981 and 2017 in the lowlands, where demographic areas are typically located, is about 500 mm/year; whereas in the highlands, where most of the agricultural activities take place and are completely surrounded by the National Park, precipitation ranges between 1,000 and 1,650 mm/year (Paltán et al., 2021). At the same time, hydrology of the Islands is governed by subsurface water processes. This leads to groundwater to dominate terrestrial water fluxes, leaving San Cristobal as the only island with some minor and intermittent water bodies. As result, across the islands, the water available to sustain various uses and needs is generally deemed to be scarce and difficult to reach (D'Ozouville et al., 2010).
Also, precipitation in the Galapagos Islands mainly concentrates during the wet and warm season of December to May. Besides, the tropical location of the Islands exposes them to climatic systems such as the ENSO. During its warm phase of El Niño, the Islands are exposed to torrential rains and floods, whereas in the negative phase of La Niña, the lack of rains and droughts impact the Islands. For example, during the 1997–98 El Niño event, severe rainstorms damaged urban infrastructure leading to important economic losses and disruptions. On the other hand, poor rainfall conditions in 2016 resulted from La Niña, critically impacted over 55% of the land dedicated for agriculture causing sectorial losses and environmental damages (MAGAP, 2016). In fact, during this event the Ecuadorian Government decreed a state of emergency for the Islands.
Apart from their exceptional natural characteristics, the Galapagos is also home to about 25,500 inhabitants [INEC, 2015; Instituto Nacional de Estadística y Censos (INEC), 2015]. In fact, about 15,700, 7,100, and 2,350 people live in Santa Cruz, San Cristobal, and Isabela Island, respectively. In addition, the vibrant regional tourism industry stimulates migrant flows from the Ecuadorian mainland and has resulted in a demographic explosion in the last several years. According to the Ecuadorian National Statistics Institute-INEC, Galapagos' population increased by 31% in 2020 (33,000 people). Also, the demographics of the Islands change significantly if the approximately 270,000 annual tourists that visit the Islands are also considered [Dirección del Parque Nacional Galápagos (Directory of the Galapagos National Park), 2019].
Naturally, this population, which fluctuates because of tourism, is in demand of services from the interlinked water and agricultural sectors. This has provoked an increased dependence on imported products from the mainland as well as water demand for the agricultural sector which helps to sustain growing food needs of the Islands (Sampedro et al., 2020). Yet, this demand for water and other services is already limited since 97% of the territory of the Galapagos is protected, leaving a short margin for human interventions. As result of this interplay across local challenging conditions, water security in the Galapagos Islands is already under stress.
At the same time, the challenges of water security in the Galapagos Islands are more acute if conditions of global change are also considered. Over 70% of farmers already report emergent droughts, potentially resulting from climate change, as one of the main challenges for the food supply systems in the Galapagos (Barrera et al., 2019). Also land use dynamics are changing in the Islands. Principally, new patterns emerged from dominating family-based agricultural systems to an abandonment of them. Typically, these new abandoned lands become monocultures of invasive plant species or pastures where crop dynamics follow the new demands of the population and variations in the preferences of the tourism sector. It is important to note that the booming tourism has provided Galapagos residents with greater spending power and, as a result, their consumption patterns and dietary habits have changed. This has been noted particularly in a greater demand for animal protein and processed food which is normally imported from the Ecuadorian mainland.
Nonetheless, the extent at which climatic, demographic, and land use changes may affect water security in the Galapagos Islands is still deeply uncertain. This is initially caused by the poor network of hydroclimatological observations in the Islands. This results in scarce available data which would sufficiently describe the historical hydrological patterns of the region. In fact, only in San Cristobal and Santa Cruz there are relevant studies of the aquifers whereas there are no publicly available hydrogeological observations across the other Islands (D'Ozouville et al., 2010; Pryet et al., 2012; Violette et al., 2014). Thus, the lack of baseline descriptions of the terrestrial hydrological characteristics of the Islands still impedes the wide integration of water security with agriculture and sustainable development in the Galapagos.
This situation is aggravated if future climate is considered and its associated uncertainties for the region. Current Global Circulations Models (GCMs) suggest that the Galapagos Islands could be about 1.4 and 1.9°C warmer than historical conditions. Also, mean annual precipitation are projected increase by 20% and 70% by 2050 (Paltán et al., 2021). Yet this apparent wetting future may be biased as future projections are thought to be constrained by the poor performance of GCMs in the region. In fact, GCMs may are believed to be overestimating the projected wetting trends across the Islands due to general the poor capacity of climatic models to capture Eastern-Pacific ENSO dynamics (Seager et al., 2019; Paltán et al., 2021).
Furthermore, the pathways of land use and development that the Islands may follow are still not very clear. The COVID-19 crisis caused oscillations not just in the number of transient (e.g., tourism) populations of the Islands, but also in terms of local expectations of the types of land use developments which would better accommodate changes in the agricultural sector. For example, potential shifts in land uses that makes food security in the Islands less dependent on the continent. Ultimately, this interplay of uncertainties limits the understanding of water security in the Galapagos Islands. Similarly, it affects the way the agricultural sector.
Despite these uncertainties, decision makers and stakeholders in the Galapagos Islands require a level of understanding of the repercussions that plausible conditions of change may have in key water security and key sectors of the Islands. This is particularly important for the agricultural sector for its role not just in maintaining local food security, but also for its role in both job creation and maintaining the agricultural frontier with the Natural Park.
The aim of this paper is to examine the vulnerabilities of water security of agricultural systems of the Galapagos Islands under uncertain future climate and land-use pathways. Here we apply a bottom-up and uncertainty-based approach to understand the response of physical drivers of water security in the agricultural catchments of the three main Islands of the Galapagos (Santa Cruz, San Cristobal, and Isabela) to stressors and sources of change. We integrate reanalysis and satellite climatological data along with land use and climate scenarios (informed from the Coupled Model Intercomparison Project, CMIP5,-derived GCMs) within a water systems model to estimate baseline and changing water characteristics of agricultural systems of the Islands. We also provide a description of terrestrial hydrological processes in the Galapagos Islands. This characterization supports our understanding of the sensitivity of critical thresholds of water security of the agricultural sector to uncertain future climate and land use. By addressing these uncertainties, we are able to describe different scenarios of the agricultural sector which may pose increase the vulnerability levels of water security in the Islands. Our findings then aim also to show the hydrological and land-use risks which may impact development in the Galapagos Islands under a context of change and uncertainty.
Provide baseline results to guide the context of water decisions in the Islands under a context of change and uncertainty and thus, contribute to efforts toward integral water security in the Islands.
This study examines the vulnerabilities of water security of agricultural catchments in the agricultural areas of the three main populated Galapagos Islands. Here we follow a bottom-up approach, which unlike traditional planning methodologies that dictate specific adverse risk conditions as specified by GCMs or other types of models, which examines intrinsic characteristics of system and test them against a wide range of plausible scenarios. As such, this approach follows the examination of critical thresholds of a system against drivers of change. Such an examination in turn allows the review of robust range of policies and strategies, which may respond adequately to a wide range of changing conditions rather than prescribed scenarios, could be drawn. As result, bottom-up approaches have been largely acknowledged in policy design for water resources in contexts where uncertainty from climatic or non-climatic sources prevails (Hallegatte, 2009; Stakhiv, 2011; Hall et al., 2012; Jensen and Wu, 2016; Brown et al., 2020; Rodríguez et al., 2021).
We believe that an uncertainty-based approach is suitable for vulnerabilities evaluation and decision making in the water-agriculture, and other sectors, in Galapagos Islands. This is because these approaches enable the diagnostic of water security in the Galapagos given the disparities in climate projections and land use future pathways. Second, this type of approach also supports overcoming the lack of consistent direct hydroclimatic observations in the Galapagos Islands which limits the development of robust regional hydrological and water system models.
The bottom-up diagnostic used in this study follows the decision scaling approach (Brown et al., 2012). In this study it consists of three main stages: 1. Diagnostic of the agricultural water system of the Galapagos Islands; 2. Scenarios of Change for these systems; and 3. the Evaluation of the Vulnerability and Sensitivity of the Agricultural Water Systems of the Galapagos Islands (Figure 1).
Figure 1. Conceptual framework of vulnerability assessment (Bottom-up approach) for water availability in the Galapagos Islands.
The study area examined herein corresponds to the agricultural catchments of the three main human-populated Islands of the Galapagos: San Cristobal, Santa Cruz, and Isabela. In these three islands, the main agricultural practices take place in their humid highlands (Figure 2). This region typically extends from what is commonly known as the “transition zone” at about 200 m.a.s.l. to some of the highest points of the islands (~900 m.a.s.l. in Isabela). These regions face the south or windward side of the islands, which receive high levels of precipitation during the warm season (December-May) and remain enveloped in clouds during the cool season (June-November).
Figure 2. Agricultural catchments for three main populated Islands of the Galapagos: (A) Santa Cruz, (B) San Cristobal, and (C) Isabela.
In the Galapagos Islands, just 3.3% of the land is rural or urban (the remaining 96.7% is under conservation). However, the variation of both climatic factors and soils has created the conditions for a highly diversified agriculture (Chiriboga et al., 2006). In Santa Cruz about 23% of the agricultural area is used for crop production, 33% for livestock activities, 23% is covered by invasive plants, while about 21% of the surface was identified as native vegetation (Laso et al., 2019). San Cristobal has the largest area of abandoned farms which cover 77% of the agricultural zone with native vegetation and invasive plants, whereas about 14% corresponds to crop production and the remaining area is used to livestock production. In Isabela, invasive species cover the largest fraction of its agricultural area (47%) while about 13% is covered by native vegetation and the remaining area is used for crops and livestock activities.
Moreover, catchments in San Cristobal are defined by a series of perennial streams and networks of aquifers which result in water springs and surface water bodies. Santa Cruz and Isabela in turn are dominated by brackish water, characterized by basal aquifers at lower elevations and deep boreholes at higher elevations where water is fresher (Violette et al., 2014). Brackish water at lower elevation in Santa Cruz island results from both seawater intrusion and aquifer over-exploitation and it is contaminated with both organic and inorganic matter (López and Rueda, 2009; Liu and d'Ozouville, 2011). At higher elevations, water is less saline since it is extracted from deep boreholes (Violette et al., 2014). Also, in Santa Cristobal, Santa Cruz, and Isabela there are 55, 24, and 6 microcatchments, respectively, within the agricultural areas.
This initial stage describes the main characteristics of the agricultural water systems of the three islands; thus, it provides baseline characteristics of the water balance for the study area. To do so, we use the Water Evaluation and Planning System (WEAP) model (Yates et al., 2005). WEAP was configured for the Galapagos using the Soil Moisture rainfall-runoff method. This is a one-dimensional model that uses a two-bucket representation, the first one representing a root zone layer and the second one representing a deep layer.
To estimate the characteristics of the water balance of the Galapagos Islands we used historical temperature and rainfall values retrieved from the ECMWF Re-Analysis product or ERA5 (with a spatial resolution of 10 km). The dataset provides as with a consistent historical climatological baseline from 1981 to 2019. This dataset is the one that, in comparison to other precipitation products available for the Galapagos, better approximates the regional meteorological observations (Paltán et al., 2021). Thus, the hydrological results obtained from this simulation are our baseline hydrological estimates.
Additional physical data inputs such as land use/land cover maps (Laso et al., 2019), soil parameters (Pryet et al., 2012; González Iñiguez, 2013), and topographic features derived from a Digital Elevation Model (DEM) at 3 arc-second spatial resolution (Lehner et al., 2008) were used to drive our WEAP model. For the main land uses in San Cristobal island we assumed the root zone conductivity (infiltration rate) and deep conductivity (percolation rate) values from D'Ozouville et al. (2010) and applied them to other Islands. Lastly, we created elevation bands to represent the elevation gradient of precipitation and temperature. As such, we were able to represent the “fog conditional” contribution to precipitation. We estimated this contribution to be 20% in the highlands following seasonal conditions as indicated by previous estimates (Pryet et al., 2012). The time range of our historical climatological series is 1981–2019 which is also the reference period of our historical simulations.
The water balance components obtained from our WEAP model were evapotranspiration, surface runoff, interflow, and base flow. Altogether, the three latter elements constitute the water availability (total flow) of the Islands. Obtained soil moisture values also describe infiltration and percolation processes. The obtained water balance variables were calculated in a monthly time-step for the historical period analyzed herein. It is important to note that an adequate aquifer simulation in the Islands is not possible due to the lack of groundwater data which would describe volume, recharge percentages, or water levels.
Lastly, to validate the model's ability to simulate our modeled infiltration, percolation and evapotranspiration rates (monthly average) we compared the output values of our WEAP model against the estimations given by the Water Commission of Ecuador (SENAGUA by its acronym in Spanish) in their plan of water resources for Galapagos (CISPDR, 2015). We also compare our evapotranspiration, baseflow and runoff estimates against the average annual results available of the hydrological balance developed by Pryet et al. (2012) and Violette et al. (2014). The results of the SENAGUA analysis are available for the three Islands whereas the studies by Violette and Pyret are just available for San Cristobal and Santa Cruz, respectively. The results of these previous studies provide an initial perspective of the water balance in the Galapagos Islands. We were constrained from applying a formal calibration and validation protocol due to the absence of available observed hydrological data in the Islands.
The second stage incorporates a range of climatic and land use change scenarios which may have implications for water security in the Galapagos Islands and their agricultural water systems. These scenarios were used to drive a stress-test to the baseline water balance of the Islands' agricultural catchments calculated in the previous stage.
The climate stress tests were conducted against a range of uncertain temperatures and precipitation estimations. Here, mean annual temperature was adjusted additively by 0.5°C increments, up to 3°C to the our historical baseline (1981–2019, as described in our previous step). Changes in precipitation are multiplicative and range from −75% to +250% which were applied to our baseline. The precipitation and temperature change factors were computed from a 10% change and an 0.5°C additive change, respectively. This results in a total of 60 climate combinations, or new climate scenario, which were generated at the grid-level across the study area.
Next, our land use scenarios are based on 2035 development trajectories as estimated by Sampedro et al. (2020) under three different scenarios (Table 1). A business-as-usual scenario (BAU) suggests that current conditions are maintained. For Scenario 1, the farmlands are abandoned by the lack of labor force due to better opportunities outside the agricultural sector, mainly in the tourism industry. This in turn also results in plants invading native vegetation remnants. Finally, for Scenario 2 the amount of agricultural land is managed through agroforestry systems and other climate-resilient practices. Under Scenario 2 the abandoned farms are reactivated leading to the conservation of the remnant of native/endemic forest.
Table 1. Scenarios of land use in the Galapagos Islands by 2035 as suggested by Sampedro et al. (2020) and Barrera et al. (2021).
BAU and Scenario 1 were developed used a system dynamic modeling approach to create a series of future scenarios, as reported by Sampedro et al. (2020). Such an approach used secondary data collected from several governmental and non-governmental stakeholders related with Galapagos' foods system. Relevant stakeholder also participated in the validation of these scenarios. As such, BAU scenario estimates a rapid growth of local population and tourism industry. Such conditions are coupled meat import restrictions that in turn stimulate local livestock production; thus demanding large portions of agricultural area and decreasing the native vegetation remnants. As of scenario 1, the growing tourism industry and food imports are the most important drivers of invasive plants in the islands. Tourism industry influences the abandonment of agricultural land which consequently us covered by invasive plants. Such plants include guava or blackberry.
Scenario 2 was based on the results of surveys that were principally carried in 2018 as reported by Barrera et al. (2021). These results showed that the key stakeholders in Galapagos such as farmers and local institutions are conscious of the importance of the transformation from conventional agricultural practices to sustainable agriculture strategies that integrate local social and environmental needs under the effects of climate change. Currently, only 8% of the farmers have implemented sustainable agricultural practices due to the lack of knowledge capacity, support of government institutions, among others.
This stage estimates the sensitivity of water security of the agricultural water systems to climate and land use change. Here we estimated the response of the total water yield (availability) as the basis to estimate vulnerability of water security. For this, we initially defined two water security sensitivity thresholds for the agricultural water catchments based on the reference conditions obtained from the 1981–2019 historical baseline. The first one indicates the La Niña 2016 total flow conditions which shows a drought threshold. This event was chosen due to the economic and socio-political repercussions it brought which led to declaring a state of emergency in the Islands. The second water security threshold represents high flows. For this purpose, we use the historical 80th percentile (P80 or the flow that is exceeded 80% of the time) of historical simulated total flows as threshold of high flows.
Then, the sensitivity and responses of total water availability (or the sum of total flows) were estimated against the 60 climate scenarios obtained in the previous stage. Next, the three land use scenarios were integrated via changing the base land cover/use maps of our WEAP model, resulting in 180 scenarios of climate and land use.
At the same time, in bottom-up type of examinations GCMs are not directly used to directly infer future hydrological conditions (or in this case, to drive and run our WEAP model) as this would propagate uncertainty across results. Conversely, GCM projections are brought in to inform and contextualize risks. Thus, for this contextualization we calculated deltas of change between the historical and future conditions of precipitation and temperature as simulated by selected GCMs (fraction of change for precipitation and absolute differences for temperature). Such deltas of change were applied to our gridded ERA-5 baseline across the islands. This in turn permit us to obtain such a bias correction approach permit us to obtain future precipitation and temperature estimates for the two time periods of this analysis: 2030 (2021–2040, or near term); 2050 (2041–2060 or medium term). Such future precipitation and temperature estimates of our study area are then used to contextualize the sensitivity of agriculture water systems in the Islands to climate change.
The selected GCMs (derived from CMIP5) were the following: CNRM-CM5, CSIROMk3-6-0, FGOALS-g2, GFDL-ESM2G, GFDL-ESM2M, GISS-E2-H, GISS-E2-R, HadGEM2-CC, HadGEM2-ES, IPSL-CM5A-LR, IPSL-CM5A-MR, MIROC-ESM, and NorESM1-M. It is important to note that, projections of four of these GCMs (CSIRO-Mk3-6-0, GISS-E2-R, IPSL-CM5A-MR, MIROC-ESM) which were used in this study, are the official climate projections used by the Ecuadorian Ministry of Environment to diagnose climate risks across the country. At the same time, the other GCMs used here were selected as these ones are the ones that have been reported to adequately capture climatic and El Niño patterns in the Galapagos region (Kim and Jin-Yi, 2012; Bellenger et al., 2014; Paltán et al., 2021). Thus, we believe that these multi-model ensembles are valid to understand future climate conditions in the Islands.
Our results find that baseflow is the dominant hydrological component across the three islands studied herein. In fact, on average, 34% of the water available in the Islands percolates there. On the other hand, surface runoff contributed almost 10% of the annual flows, and interflow contributed just 6%. Across the Islands, we also estimate that in San Cristobal, surface runoff only contributes about 1% of the total streamflow despite this Island being the only one with perennial streams. We attribute this to the limited ability of the ERA5 data to capture precipitation in San Cristobal and thus a poor capacity of this product to detect rainfall changes along the altitude gradient in this Island (Paltán et al., 2021). This deficiency in turn leads our model to not sufficiently saturate the soil while also overestimating evapotranspiration rates and thus to not produce enough surface runoff. However, this result was not seen for either Santa Cruz or Isabela Islands for which the model was able to capture precipitation patterns adequately well for more details on precipitation biases please refer to Paltán et al. (2021).
We also find that typically between 37 and 64% of the annual rainfall is lost to evapotranspiration (Figure 3). We also note that evapotranspiration is notably higher in San Cristobal than in the rest of the Islands. These results are similar to those reported in previous studies which gives an indication of the adequacy of our model (Table 2), particularly for the baseflow component.
Figure 3. Monthly average contributions of hydrological fluxes to the water balance for the period 1981-2019 for: (A) San Cristobal, (B) Santa Cruz, and (C) Isabela. The figure shows the variability of the different components of the water balance as a percentage of the total water availability. Annual totals consider only precipitation, baseflow, evapotranspiration, surface runoff and interflow.
Table 2. Comparison of yearly average (1981–2019) estimates of hydrological fluxes obtained in this study with similar studies across the three Islands studied here.
Our results also reflect the natural influence of the climate seasonality of the islands in the terrestrial hydrology of the Islands. As result, surface runoff peaks are dominant in Isabela and Santa Cruz during the wet months. In these two islands, in April and May surface runoff may account for 17% of the overall water flows. This peak is then followed by a period of aquifer recharge from May to June. Then, in the dry season, the water balance is essentially sustained by the baseflow and dominated by evapotranspiration processes.
Baseflow is also directly linked with the storage of water in the soil, which follows the rainfall seasonality and also represents a combination of infiltration, percolation and aquifer recharge. Our results show that principally baseflow, via soil moisture changes, drives the land water availability in the Islands; particularly during the dry seasons where these processes maintain the recharge of aquifers (Figure 4). These results in turn emphasize the key role that soil moisture has in general energy and water balance processes through its impact in evapotranspiration processes (Seneviratne et al., 2010). Thus, in the Galapagos, soil moisture changes seem to influence not only soil wetness dynamics but also hydrological fluxes such as baseflow, where its moisture content represent all the available water in the soil for plants, aquifer recharge and evapotranspiration.
Figure 4. Seasonality of changes in soil moisture for (A) San Cristobal, (B) Santa Cruz, and (C) Isabela.
We find that the 2016 La Niña event, set as the drought threshold for this analysis, represented a decrease of about 25% in median annual total flow across the three Islands (Figure 5). This was caused by a similar decrease in precipitation. In San Cristobal the flow decrease was of about 25%, in Santa Cruz of 28%, and 21% for Isabela. On the other hand, the historical 80th percentile (P80), used here to represent high flows, represents an increase of 127% in total flow over historical conditions. This flow increase is 148% in San Cristobal, 127% in Santa Cruz, and 107% in Isabela. We also find that El Niño events of 1981–82 and 1997–98 lead to an extraordinary increase in mean annual total flow of over 500%. Hence, physical water security conditions in the Islands are maintained when these thresholds are not surpassed; conversely, once they are reached or exceeded, the agricultural water systems of the Islands become vulnerable to water insecurity.
Figure 5. Climate response surface map of historical total flow conditions (1981–2019) to changes in climate. The color bar shows percent of change of total flow when historical baseline conditions are modified by precipitation (x-axis) and temperature changes (y-axis). Projected precipitation and temperature changes for near-term (red) and medium-term (black) are indicated for individual GCM projections biased corrected for the Galapagos Islands. The thresholds of dry and wet condition are represented with dotted lines. For reference, the dotes lines follow the color gradient bar and the x axis.
Next, our results indicate that total flow is mainly sensitive to precipitation changes as opposed to temperature changes, which do not seem to play a major role in driving total flow changes. Importantly, to reach the 2016 La Niña-drought threshold, an 18% decrease in annual precipitation from historical conditions will be needed across the Islands. In the wet season, given its key role in maintaining hydrological processes in the Islands, this is more critical as decrease of about 12% of precipitation from historical conditions could cause the Islands to meet this water security threshold. This finding suggests that physical water security in the Galapagos Islands is principally vulnerable to potential shifts during the wet season. On the other hand, to achieve our high flows of P80 as a water security threshold, a mean increase in precipitation of about 80% is needed across the islands. This corresponds to an increase in 85%, 85%, 70%, from historical conditions, in San Cristobal, Santa Cruz, and Isabela, respectively.
Moreover, we note that in both San Cristobal and Santa Cruz, 66 out of the 67 GCMs used for this analysis projected future mean annual conditions that, although conditions become warmer and wetter, total flow falls within the ranges defined by the assumed thresholds or expected conditions. The result is total flow conditions which are greater than those observed in the 2016 La Niña event but lesser that the P80 high flow conditions. In Isabela, the proportion of GCMs with total flows in the expected condition range is 63 out of 67 GCMs. More importantly, for the water and agricultural sectors, two (3%) GCMs in both Santa Cruz and Isabela and one (2%) in San Cristobal projected changes that would lead to a poor wet season. These scenarios would lead the Islands to experience total flow conditions similar to, or worse than, those observed during the 2016 La Niña event. Additionally, seven (10%) GCMs in San Cristobal, nine (13%) in Santa Cruz and 10 (15%) in Isabela project conditions where high base flows will be exceeded (>P80) during the wet season. Lastly, we find that 99% of the GCMs used in this study agree that expected historical baseflow conditions will be maintained during the dry season. Nonetheless, this apparent wet, although optimistic, trend should be interpreted with care given the reported biases of GCMs in the Galapagos and the detected drying trend in the region (Paltán et al., 2021).
Naturally, given the relevance of baseflow in the total flow of the Galapagos Islands, we also note that this variable is the one where changes in climate are more evident (Figure 6). For example, a reduction of 14% in precipitation from historical conditions would in turn lead to baseflow conditions similar to those experienced in 2016, across the Islands, and thus make the agricultural water systems insecure. This is a decrease of about 13% of annual historical precipitation conditions in Isabela and 15% for both San Cristobal and Santa Cruz. At the same time, none of the GCMs used here detect such annual drought conditions. Conversely, 2% of GCMs projected high baseflow annual conditions in both San Cristobal and Santa Cruz, and 6% of them in Isabela. Yet, here again, it is important to highlight the poor performance that GCMs have in the region.
Figure 6. Climate response surface map of historical baseflow conditions (1981–2019) to changes in climate. The color bar shows percent of change of total flow when historical baseline conditions are modified by precipitation (x-axis) and temperature changes (y-axis). Projected precipitation and temperature changes for near-term (red) and medium-term (black) are indicated for individual GCM projections biased corrected for the Galapagos Islands. The thresholds of dry and wet condition are represented with dotted lines. For reference, the dotes lines follow the color gradient bar and the x axis.
Also, we note that mean annual precipitation historical conditions in Isabela need to decrease by 15% for baseflow to reach drought conditions and increase about 60% to reach extreme flows. As such, the range of expected (within our defined ranges) water security (between drought and extreme baseflow conditions) is just about 75 percentual points. Conversely, San Cristobal required a decrease of about 25% and an increase in 100% to reach baseflow drought and extreme flows accordingly, whereas in Santa Cruz these values are −20% and +125%. These findings first suggest that the Galapagos Islands are more sensitive to experience baseflow drought conditions as just a minor decrease in historical precipitation conditions (from −15% to−25%) is needed to reach the drought conditions defined by the 2016 event. Second, Isabela is the island where shifts between dry, expected and high baseflow conditions, and thus shifts between water security and insecurity, are more sensitive to changes in climate. This is also particularly problematic during the wet season (where the range between drought and extreme baseflow conditions is about 60%). In Isabela this could be explained by the large amount of agricultural and invasive species land uses which in turn may decrease infiltration and water storage. Ultimately, these changes in baseflow may also be reflected in the shifts in runoff conditions, which also contribute to total flow conditions during the wet months, particularly in San Cristobal and Isabela.
Next, we evaluate the way in which water security, via total water availability, in the Islands responds to changes in land use in addition to the anticipated changes in climate. First, we note that when the changes in land use evaluated herein are introduced, total flows do not appreciably change across the Islands. This could be related to the similar way by which these land uses saturate the soil which in turn do not considerably change runoff, interflow and baseflow characteristics (which together constitute total flow).
During the wet season, when sustainable agricultural practices are considered in land use management (Scenario 2), temperature does not seem to play a role in water availability changes and thus water (in)security conditions (Figure 7). However, during the dry season, we observed a slight sensitivity of total flow to temperature changes, particularly in San Cristobal and Isabela under BAU and Scenario 1 conditions. Results also suggest that agroforestry systems seem to better buffer microclimate conditions, decreasing the daily range of solar radiation and temperature in the agricultural landscapes.
Figure 7. Surface response maps of the impact of changes in both climate conditions and land use on water availability (total flow) in the dry season. Projected precipitation and temperature changes for near-term (red) and medium-term (black) are indicated for individual GCM projections biased corrected for the Galapagos Islands.
For the baseflow analysis, we first find that two of the land use scenarios (BAU and Scenario 1) analyzed here do not importantly impact mean annual baseflow conditions (Figure 8). Yet, we find that both temperature and precipitation play an important role in driving baseflow, the dominant hydrological component, in San Cristobal and Santa Cruz under both scenarios. In both Islands, as temperatures increase by 1.5°C or more, baseflow recedes toward a water insecurity zone of drier conditions. This may be explained by both higher evapontranspirative raters and the negative impact that high temperatures may have on the generation of fog, drizzles, and heavy mists in the highlands or locally known as garúa.
Figure 8. Surface response maps of the impact of changes in both climate conditions and land use on baseflow in the dry season. Projected precipitation and temperature changes for near-term (red) and medium-term (black) are indicated for individual GCM projections biased corrected for the Galapagos Islands.
Moreover, we find that Scenario 2 has considerable affects the range of water security in the islands under future changes in climate. Importantly, this scenario increases the high flow conditions range (Figures 8, 9). When this scenario is introduced, baseflow (and this, total water) available during the wet season increases and approximates our identified thresholds of high flows. As a result, in Santa Cruz and Isabela, the number of GCMs that are mapped under drought conditions falls from about 17% to 6%. This result in turn suggests that Land Use scenario 2 in turn favors more robust conditions of agricultural areas to face potential future droughts. Nonetheless, we also find that in the three islands 6% more of GCMs project high baseflow conditions (Figure 8). So general increase in baseflow conditions, across scenarios, may also lead to oversaturation of soils and an increase in high flow conditions which may cause, among others, crop damages.
Figure 9. Surface response maps of the impact of changes in both climate conditions and land use on baseflow in the wet season. Projected precipitation and temperature changes for near-term (red) and medium-term (black) are indicated for individual GCM projections biased corrected for the Galapagos Islands.
In this study, we applied for the first time, to our knowledge, an uncertainty-based approach in the Galapagos Islands. This was used to understand the thresholds and potential vulnerability scenarios which may affect water security in the Galapagos Islands. At the same time, we explored how changes in total water land fluxes contribute to estimate changes in the water sector of agricultural areas in the Galapagos Islands. As such, our thresholds of water (in)security are those determined by the 2016 La Niña drought conditions and high flow estimates (P80). We believe that the application of a physical-driven metric to describe water security provides an initial approximation of drivers of change and risk in complex ecosystems. With this estimation we pave the way to include additional institutional, operational, and infrastructure criteria which overall robustly describe water security (Grey and Sadoff, 2007; Hall et al., 2014).
In our study we first acknowledge, the already understood role that baseflow has in maintaining the hydrological fluxes in the Galapagos Islands (comprising about 35% of total annual water fluxes). As a result, this element appears to be the key hydrological terrestrial driver which regulates physical water (in)security in the Islands. At the same time, evapotranspirative fluxes critically impact water availability in the region since more than 60% of the annual rainfall is lost to evapotranspiration. In line with this, the key role that soil moisture has in sustaining baseflow and also, water security conditions is also noted in this study. Thus, we note the need of actions to maintain and conserve the baseflow while potential strategies to manage and reduce evapotranspirative losses. Yet, further local studies are needed to evaluate the interactions across climate, soil moisture and hydrological fluxes in key catchments of the Galapagos Islands.
Next, our findings show that changes in precipitation have large impacts on fluctuations in total water flows and constitute the key climatological driver of water security in the Galapagos Islands. For instance, we find that a decrease in approximately 25% of total water flows, from historical conditions, would drive drought conditions resembling those of the emergency state of 2016. Conversely, if total water flows, more than double, or increase by about 120%, from historical conditions, the threshold of high flows would be met.
Similarly, we identify the important sensitivity of baseflow to precipitation change. We detect that an average reduction of about 15% in precipitation from historical conditions would in turn lead to baseflow conditions similar to those experienced in 2016 across the three Islands studied here. We also note that historical precipitation conditions in Isabela just need to decrease 15% (increase 60%) to reach drought and (high) baseflow conditions. Such short margin of change (about 75 percentual points) implies that Isabela is the most sensitive Island to climate fluctuations which may drive water (in)security.
Moreover, via the combinations of climate and land-use change scenarios, we did not find a notable effect of land-use change in the seasonal and annual total flow changes for the agricultural systems across the three islands. However, taking into account baseflow, the dominant component flow, our results suggest that a potential increase in water demand driven by water-intense land use changes combined with a reduction in precipitation during the wet season would make the agricultural catchments importantly vulnerable to droughts. Similarly, when the water-intense land use scenarios are incorporated and temperature increases reach 1.5°C or more, we find that in San Cristobal and Santa Cruz baseflow recedes toward a water insecurity zone of drier conditions. Thus, we emphasize the need for monitoring the combined implications of both climate and land use, as opposed to individual assessments which may mislead conclusions about the real impact on the agricultural water systems of the Islands of both these uncertain drivers of change.
On the other hand, our findings showed that under a sustainable scenario of land use (Scenario 2), which considers agroecology principles, water security and hydroclimatic robustness conditions of the Galapagos Islands improve. So, these results emphasize the need for transforming traditional agricultural production in Galapagos into sustainable and ecologically informed practices which include crop diversification, tree integration in the agricultural system, and integral management practices, among others. For example, in Isabela, livestock and grazing, a water intense activity, is the principal activity in their agricultural catchments. Yet this island does not account for water distribution systems while being the one where fluctuation between water security levels are greatest as described previously. As result, farmers completely depend on the Ministry of Agriculture (MAG) and private water tanks (CISPDR, 2015) to sustain this water-intense activity. This leads the Islands in an important situation of vulnerability driven by unstainable water and agricultural practices.
Additionally, water quality also constitutes an important challenge to water security in the Galapagos Islands. In fact, water quality conditions in the region have been found not to be suitable for drinking and domestic use as well as for agricultural practices (Walsh et al., 2010; Mateus et al., 2019; Grube et al., 2020). Since brackish water has been used for irrigation for at least a decade, this long-term use of saline water is responsible for altering soil properties. In general, the contamination of solid and affect the already limited freshwater availability in the Islands as well as in the water that drains into the water table. Thus, changes in land cover and uses as well as shifts in climate may in turn also result in further exacerbations of water quality conditions across the Islands. This problematic yet remains a challenge despite the constant efforts and funds dedicated to address this issue. A closer examination of the links across water quality and water security, using bottom-up approaches, remain a gap which requires further examination.
Lastly, our results also pave the way toward a next phase of uncertainty-based diagnostics in the study area where these thresholds can be stress-tested against a number of climate scenarios which are stochastically (via weather generators or similar tools) generated and explore deeper the climatic uncertainty assessed herein. Additionally, this study established the bases to incorporate additional drivers of risk which have not been examined here. They include water quality and contamination from organic wastes, fertilizers, and pesticides in freshwater, impact of tourism, biodiversity and others.
The lack of available data of hydroclimatological models is potentially the major barrier to provide a deeper examination of hydrology and water security in the Galapagos Islands. A clear example is the lack of enough meteorological observations to cover the wide geographic and altitudinal variation of the Islands. Also, the insufficient maintenance of the existing meteorological stations as well as the entire absence of instruments to read the geographical diversity and terrestrial hydrological variables such as streamflow, soil moisture or aquifer dynamics, limits the understanding of the water cycle and its implications for the Galapagos Islands. Similarly, the lack of robust hydrological and hydrogeological observations constrains a sophisticated calibration and validation of the water model used here. This in turn may lead our findings not to sufficiently understand water seasonality dynamics.
Additionally, while ERA5 provides a key input to our study our reported limitations should inform future uses of this dataset in the Galapagos Islands. Particularly, the inadequate capturing of rainfall spatial variability along the islands which is especially relevant in the altitudinal gradient in San Cristobal, should be managed with care. At the same time, further studies may need to refine the risk contextualization with using updated CMIP6 model outputs. Yet, this step would also require examining the GCMs which better capture regional climatological dynamics in the Galapagos. As result, the interpretation of water dynamics described here should consider these limitations.
This study provides the first framework for understanding and planning of water resources in the Galapagos Islands under a context of uncertainty. This becomes critical for the Islands given the lack of regional agreement and understanding in terms of the future hydroclimatic and land use pathways that the Islands will follow. The latter responds not just to the biases of climate models for the region, but also to the lack of historical observations. This is accompanied by the unclear pathway that the agricultural sector will in fact take.
As such, the uncertainty-based framework applied herein sustains the basis for an application of these approaches to enhance resilience of the agricultural water systems as well as other systems. So, via the application of this approach, we show the first diagnostic of how different water fluxes constitute the water balance of the agricultural systems in the Islands. We highlight the important role of soil moisture dynamics in sustaining the baseflow, which is in fact critical to sustain water security. Thus, next efforts should focus toward understanding further the relationships across climate, soil moisture, infiltration, and overall groundwater. Similarly, a more dedicated examination is needed to understand evapotranspirative responses across different land use and vegetation scenarios.
Also, the critical thresholds for water security estimated here provide the basis for an initial diagnostic of resilience levels for the Islands. In fact, an immediate implication of our findings is the critical need to closely track precipitation patterns which may decrease by about 25–30% and thus result in a lack of water availability resembling the conditions of the drought emergency of 2016. This potential consequence is more critical during the wet season. On the contrary, when precipitation and water availability increase in over 100% from their historical baseline conditions, the Islands would experience high flow conditions (understood here at a P80 threshold). So, the findings shown here already define an initial priority: the need to establish a system that monitors and measures climate and land surface variables to permit a deeper understanding of the hydroclimatic sensitivity points analyzed here.
Next, a more comprehensive understanding of resilience would require the diagnostic of the sensitivity thresholds of individual crops, water and interconnected systems, and infrastructure assets. This for example involves estimating the threshold response of high-value crops in the Islands, based on the contribution to family income such as citric trees, tomato or coffee, to decreases in precipitation and lack of available water. Also, this includes estimating the degree at which the basic irrigation systems in the Islands (which often rely purely on water delivered by trucks) are sensitive to flashfloods.
Also, our results emphasize the need to design flexible policies in the water-agricultural sector which account for fluctuations in climate. This is particularly relevant for Isabela, where we detect that the margin of range, from historical baseline conditions, between drought and high flow conditions is rather narrow when compared to the other Islands. This flexibility is also needed due to the reported poor performances of climate models for the region. While models show an apparent robustness of agricultural water systems in the Galapagos, this should be interpreted with precaution and decision makers should rather encourage the application of what if? types of scenarios assessments as well as uncertainty approaches to design policies. Naturally, such flexibility in policies should also account for methodological limitations found in this study as described previously.
Moreover, we stress the need to integrate climate with other variables, such as land use change, for comprehensive decision making. For example, we are able to identify that when sustainable land use practices are introduced in the Islands, water availability in the wet season increases, thus, suggesting an increase in resilience. Similarly, when land use is introduced in the models, the vulnerability of water security of Islands to a temperature increase of 1.5°C manifests. We also emphasize the need to evaluate the adequacy of CMIP6 and Regional Climate Models (RCMs) to inform hydroclimatological processes in the Galapagos Islands. This would in turn permit a deeper examination of extremes and risk conditions of water, food, and other systems in the Islands. As such, climate, land use, demographics, and other variables, should not be seen as independent variables which require single-focus interventions. We thus emphasize the need to understand water security in an integral way.
At the same time, our results also highlight how bottom-up type of approach help to diagnose the links across water security and agriculture in development regions where uncertainties in data, climate and land use trajectories prevail. Particularly, our results show that characterizing risk and vulnerabilities offer an opportunity to understand drivers of change which may affect water security and food systems in arid and Island regions. Additional replications of these approaches, while accounting with imperfect satellite observations, reanalysis products, and models, provide an initial yet fundamental glimpse of the strategies increase water security and resilience in these regions. Naturally, the refinement of these data sources, the generation and use of additional climatological data (such as CMIP6 projections), land use, demographic and other time series which describe risk along with the development of models to refine the human role in water (via operation of water systems, urbanization, and others) would be an immediate next step.
Hence, we expect that this study establishes the basis for an integral water security and resilience assessment of the Islands which accounts for not just agricultural systems but also water supply and sanitation, water for energy uses, and indeed, water and conservation dynamics. This integral assessment should also account for other criteria that are not evaluated here. They include institutions and regulations, access and equity dimensions of water, infrastructure, water quality, and general criteria which help to design for resilience to face uncertain future changing conditions. Ultimately, the wide embracing of the uncertainty which dominates the Islands is the key to design of resilient and robust policies.
The raw data supporting the conclusions of this article will be made available by the authors, without undue reservation.
HP: conceptualization, writing—original draft, and methodology. FB: conceptualization, writing—review and editing, and methodology. MN, CMa, and CMe: conceptualization, writing—review and editing, methodology, and formal analysis. All authors contributed to the article and approved the submitted version.
This project was funded by Universidad San Francisco de Quito.
The authors declare that the research was conducted in the absence of any commercial or financial relationships that could be construed as a potential conflict of interest.
All claims expressed in this article are solely those of the authors and do not necessarily represent those of their affiliated organizations, or those of the publisher, the editors and the reviewers. Any product that may be evaluated in this article, or claim that may be made by its manufacturer, is not guaranteed or endorsed by the publisher.
Barrera, V., Monteros-Altamirano, Á., Valverde, M., Escudero, L., Allauca, J., and Zapata, A. (2021). Characterization and classification of agricultural production systems in the Galapagos Islands (Ecuador). Agricult. Sci. 12, 481–502. doi: 10.4236/as.2021.125031
Barrera, V. H., Escudero, L, Valverde, M., and Allauca, J. (2019). Productividad y Sostenibilidad de Los Sistemas de Producción Agropecuaria de Las Islas Galápagos-Ecuador. Quito: INIAP.
Bellenger, H., Guilyardi, E., Leloup, J., Lengaigne, M., and Vialard, J. (2014). ENSO representation in climate models: From CMIP3 to CMIP5. Clim. Dynam. 42, 1999–2018. doi: 10.1007/s00382-013-1783-z
Brown, C., Boltz, F., Freeman, S., Tront, J., and Rodriguez, D. (2020). Resilience by design: a deep uncertainty approach for water systems in a changing world. Water Secur. 9, 100051. doi: 10.1016/j.wasec.2019.100051
Brown, C., Ghile, Y., Laverty, M., and Li, K. (2012). Decision scaling: Linking bottom-up vulnerability analysis with climate projections in the water sector. Water Resour. Res. 48. doi: 10.1029/2011WR011212
Chavez, F. P., and Brusca, R. C. (1991). “The Galapagos Islands and their relation to oceanographic processes in the Tropical Pacific,” in Galapagos Marine Invertebrates. Cham: Springer, 9–33.
Chiriboga, R., Fonseca, B., and Maignan, S. (2006). Desarrollo de Políticas y Estrategias de Manejo Del Sector Agropecuario y Su Relación Con Las Especies Introducidas En La Provincia de Galápagos. Producto Dos: Caracterización De Los Sistemas De Producción En Galapagos En Relación Con El Fenómeno de Las Especies Invasoras Santa Cruz-Puerto Ayora.
CISPDR (2015). “Plan Regional de Los Recurso Hídricos de Las Islas Galápagos.” in Galápagos, Ecuador. Available online at: http://www.odepa.gob.cl (accessed June, 2022).
Dirección del Parque Nacional Galápagos (Directory of the Galapagos National Park) (2019). Informe Anual Visitantes a Las de Galápagos Á reas Protegidas de Galápagos. San Cristobal, Galapagos: Ministerio del Ambiente de Ecuador. Available online at: http://www.galapagos.gob.ec/wp-content/uploads/2020/01/INFORME-ANUAL-DE-VISITANTES-2019.pdf (accessed June, 2022).
D'Ozouville, N., Pryet, A., Violette, S., de Marsily, G., Deffontaines, B., and Auken, E. (2010). “Hydrogeology of the Galapagos Islands,” in AGU Fall Meeting Abstracts, 2010:V51D-06.
González Iñiguez, A. A. (2013). “Cálculo Del Balance Hídrico a Nivel de Suelo En La Zona Agrícola de La Cuenca Pelikanbay En La Isla Santa Cruz-Galápagos, Ecuador,” in Quito 2013.
Grey, D., and Sadoff, C. W. (2007). Sink or Swim? Water Security for Growth and Development. Water Policy 9, 545–571. doi: 10.2166/wp.2007.021
Grube, A. M., Stewart, J. R., and Ochoa-Herrera, V. (2020). The challenge of achieving safely managed drinking water supply on San Cristobal Island, Galápagos. Int. J. Hyg. Environ. Health 228, 113547. doi: 10.1016/j.ijheh.2020.113547
Hall, J. W., Grey, D., Garrick, D., Fung, F., Brown, C., Dadson, S. J., and Sadoff, C. W. (2014). Coping with the curse of freshwater variability. Science 346, 429–430. doi: 10.1126/science.1257890
Hall, J. W., Lempert, R. J., Keller, K., Hackbarth, A., Mijere, C., and McInerney, D. J. (2012). Robust climate policies under uncertainty: a comparison of robust decision making and info-gap methods. Risk Analy. 32, 1657–1672. doi: 10.1111/j.1539-6924.2012.01802.x
Hallegatte, S. (2009). Strategies to adapt to an uncertain climate change. Global Environm. Change 19, 240–247. doi: 10.1016/j.gloenvcha.2008.12.003
Houvenaghel, G. T. (1974). Equatorial undercurrent and climate in the Galapagos Islands. Nature 250, 565. doi: 10.1038/250565a0
Jensen, O., and Wu, X. (2016). Embracing uncertainty in policy-making: the case of the water sector. Policy Soc. 35, 115–123. doi: 10.1016/j.polsoc.2016.07.002
Kim, S. T., and Jin-Yi, Y. (2012). The two types of ENSO in CMIP5 models. Geophys. Res. Lett. 39, L11704. doi: 10.1029/2012GL052006
Laso, F. J., Benítez, F. L., Rivas-Torres, G., Sampedro, C., and Arce-Nazario, J. (2019). Land cover classification of complex agroecosystems in the non-protected highlands of the Galapagos Islands. Remote Sens. 12, 65. doi: 10.3390/rs12010065
Lehner, B., Verdin, K., and Jarvis, A. (2008). New global hydrography derived from spaceborne elevation data. Eos, Transact. Am. Geophys. Union 89, 93–94. doi: 10.1029/2008EO100001
Liu, J., and d'Ozouville, N. (2011). “Water Contamination in Puerto Ayora: Applied Interdisciplinary Research Using Escherichia Coli as an Indicator Bacteria,” in Galápagos Report 2012. Puerto Ayora: Charles Darwin Foundation.
López, J., and Rueda, D. (2009). “Water Quality Monitoring System in Santa Cruz, San Cristóbal, and Isabela,” in Galapagos Report 2010. Puerto Ayora: Charles Darwin Foundation.
MAGAP (2016). “Ganadería, Acuacultura y Pesca Plan de Bioagricultura Para Galápagos.” Quito, Ecuador: MAGAP.
Mateus, C., Guerrero, C. A., Quezada, G., Lara, D., and Ochoa-Herrera, V. (2019). An integrated approach for evaluating water quality between 2007–2015 in Santa Cruz Island in the Galapagos Archipelago. Water 11, 937. doi: 10.3390/w11050937
Paltán, H. A., Benitez, F. L., Rosero, P., Escobar-Camacho, D., Cuesta, F., and Mena, C. F. (2021). Climate and sea surface trends in the Galapagos Islands. Scient. Rep. 11, 14465. doi: 10.1038/s41598-021-93870-w
Pryet, A. (2011). Hydrogeology of volcanic islands: a case-study in the Galapagos Archipelago (Ecuador). (Doctoral dissertation).
Pryet, A., Domínguez, C., Tomai, P. F., Chaumont, C., d'Ozouville, N., Villacís, M., and Violette, S. (2012). Quantification of cloud water interception along the windward slope of Santa Cruz Island, Galapagos (Ecuador). Agricult. For. Meteorol. 161, 94–106. doi: 10.1016/j.agrformet.2012.03.018
Rodríguez, D. J., Paltán, H. A., García, L. E., Ray, P., and St. George Freeman, S. (2021). Water-related infrastructure investments in a changing environment: a perspective from the World Bank. Water Policy 23, 31–53. doi: 10.2166/wp.2021.265
Sachs, J. P., and Ladd, S. N. (2010). Climate and oceanography of the galapagos in the 21st century: expected changes and research needs. Galapagos Res. 67, 50–54. Available online at: http://hdl.handle.net/1834/36288
Sampedro, C., Pizzitutti, F., Quiroga, D., Walsh, S. J., and Mena, C. F. (2020). Food supply system dynamics in the Galapagos islands: agriculture, livestock and imports. Renew. Agricult. Food Syst. 35, 234–248. doi: 10.1017/S1742170518000534
Seager, R., Cane, M., Henderson, N., Lee, D.-R., Abernathey, R., and Zhang, H. (2019). Strengthening tropical pacific zonal sea surface temperature gradient consistent with rising greenhouse gases. Nat. Clim. Chang. 9, 517–522. doi: 10.1038/s41558-019-0505-x
Seneviratne, S. I., Corti, T., Davin, E. L., Hirschi, M., Jaeger, E. B., Lehner, I., Orlowsky, B., and Teuling, A. J. (2010). Investigating soil moisture–climate interactions in a changing climate: a review. Earth-Sci. Rev. 99, 125–61. doi: 10.1016/j.earscirev.2010.02.004
Stakhiv, E. Z. (2011). Pragmatic approaches for water management under climate change uncertainty. JAWRA. 47, 1183–1196. doi: 10.1111/j.1752-1688.2011.00589.x
Trueman, M., and d'Ozouville, N. (2010). Characterizing the Galapagos terrestrial climate in the face of global climate change. Galapagos Res. 67, 26–37. Available online at: http://hdl.handle.net/1834/36285
Violette, S., d'Ozouville, N., Pryet, A., Deffontaines, B., Fortin, J., and Adelinet, M. (2014). “Hydrogeology of the Galapagos Archipelago: An Integrated and Comparative Approach between Islands,” in The Galapagos: A Natural Laboratory for the Earth Sciences. AGU Advancing Earth and Space Science, eds Harpp, K. S., Mittelstaedt, E., d'Ozouville, N., and Graham, D. W. (Washington, DC: American Geophysical Union).
Walsh, S. J., McCleary, A. L., Heumann, B. W., Brewington, L, Raczkowski, E. J., and Mena, C. F. (2010). Community expansion and infrastructure development: implications for human health and environmental quality in the Galápagos Islands of Ecuador. J. Latin Am. Geograp. 24, 137–159. doi: 10.1353/lag.2010.0024
Walsh, S. J., and Mena, C. F. (2013). “Perspectives for the Study of the Galapagos Islands: Complex Systems and Human–Environment Interactions,” in Science and Conservation in the Galapagos Islands: Frameworks & Perspectives, eds Walsh, S. J., and Mena, C. F.(New York, NY: Springer New York), 49–67.
Keywords: water security, uncertainty, climate change, Galapagos Islands, resilience, food security
Citation: Paltán HA, Benítez FL, Narvaez M, Mateus C and Mena CF (2023) Water security and agricultural systems in the Galapagos Islands: vulnerabilities under uncertain future climate and land use pathways. Front. Water 5:1245207. doi: 10.3389/frwa.2023.1245207
Received: 27 June 2023; Accepted: 17 August 2023;
Published: 01 September 2023.
Edited by:
Edmundo Molina-Perez, Tecnologico de Monterrey, MexicoReviewed by:
Antonia Hadjimichael, The Pennsylvania State University (PSU), United StatesCopyright © 2023 Paltán, Benítez, Narvaez, Mateus and Mena. This is an open-access article distributed under the terms of the Creative Commons Attribution License (CC BY). The use, distribution or reproduction in other forums is permitted, provided the original author(s) and the copyright owner(s) are credited and that the original publication in this journal is cited, in accordance with accepted academic practice. No use, distribution or reproduction is permitted which does not comply with these terms.
*Correspondence: Homero A. Paltán, aG9tZXJvLnBhbHRhbmxvcGV6QG91Y2Uub3guYWMudWs=
Disclaimer: All claims expressed in this article are solely those of the authors and do not necessarily represent those of their affiliated organizations, or those of the publisher, the editors and the reviewers. Any product that may be evaluated in this article or claim that may be made by its manufacturer is not guaranteed or endorsed by the publisher.
Research integrity at Frontiers
Learn more about the work of our research integrity team to safeguard the quality of each article we publish.