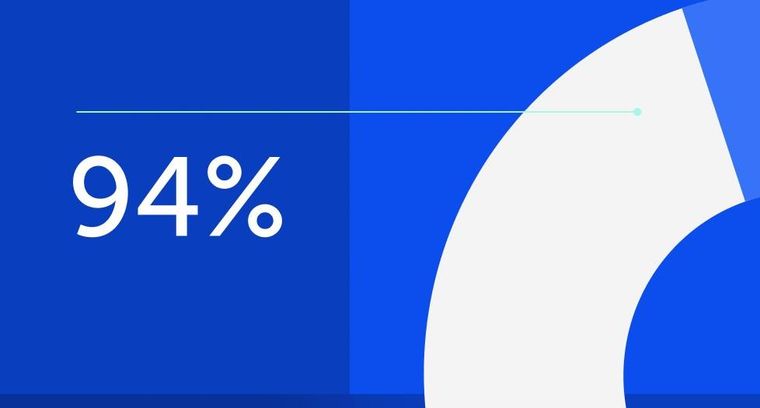
94% of researchers rate our articles as excellent or good
Learn more about the work of our research integrity team to safeguard the quality of each article we publish.
Find out more
PERSPECTIVE article
Front. Water, 05 July 2023
Sec. Water and Critical Zone
Volume 5 - 2023 | https://doi.org/10.3389/frwa.2023.1196465
This article is part of the Research TopicWomen in Critical Zone ScienceView all 7 articles
The critical zone has been the subject of much discussion and debate as a term in the ecosystem, soil and earth system science communities, and there is a need to reconcile how this term is used within these disciplines. I suggest that much like watershed and soil ecosystems, the critical zone is an ecosystem and is defined by deeper spatial and temporal boundaries to study its structure and function. Critical zone science, however, expands the scope of ecosystem and soil science and more fully embraces the integration of earth sciences, ecology, and hydrology to understand key mechanisms driving critical zone functions in a place-based setting. This integration of multiple perspectives and expertise is imperative to make new discoveries at the interface of these disciplines. I offer solid examples highlighting how critical zone science as an integrative science contributes to ecosystem and soil sciences and exemplify this emerging field.
- Much like watershed and soil ecosystem, we can view the critical zone as an ecosystem and define deeper space and time boundaries to study its structure and function.
- Critical zone science more fully embraces the integration of earth sciences and biological sciences to understand the critical zone in a place-based science setting.
- The integration of multiple perspectives is imperative to make new discoveries at the interface of these disciplines.
I am ecosystem scientist with a PhD in soil science from UC Berkeley. Indeed, I am the last of a long line of scientists who grew out of a disciplinary soil science program at Berkeley (and Hans Jenny's perspective) as it was replaced by a more integrative program, Environmental Science Policy and Management. I'd posit to say that I am an early generation of a critical zone scientist before there was such a term.
In graduate school, I was a hybrid scientist—I coupled hydrology and biogeochemistry to understand soil solution losses from tropical forest ecosystems of contrasting soil age (300 and 4,100,000 years) and their responses to anthropogenic nitrogen additions (Lohse and Dietrich, 2005; Lohse and Matson, 2005). I needed to understand and integrate soil hydrology, geology, soil biogeochemistry, and ecology to address my research questions. To accomplish this goal, I assembled an interdisciplinary dissertation committee, an ecosystem ecologist, geomorphologist, and soil scientist. While taking on the challenge to “confront all of the processes that maintain or change ecological systems, whether these processes were (sic) biological, physical or geological” goes back to Hutchinson (1948) and remains an inspirational call to arms, the boundaries between disciplines and departments had sharpened in my graduate school time rather than become more diffuse and permeable. As a result, venturing across discipline boundaries required the initiative and confidence to feel at times the dilettante or master of none. To this day, many programs and departments remain siloed owing to financial and other social structures, creating artificial barriers and disincentives to collaborate as well as train interdisciplinary scientists. As an aside, relatively recent (re)organization of universities, at UC Merced, for example, with the School of Natural Sciences and no department boundaries, show signs that institutions are softening these boundaries and making them more permeable. At the end of my dissertation, my research domain was at the interface of ecology, soil science and earth system science; I did not fit in one academic box, as an ecologist, soil scientist, or a hydrologist. Indeed, it took me 5 years and two postdocs to find my first faculty position in part because I did not fit squarely into a faculty position. At interviews, I found myself a chameleon. Eventually, though, I found a home at a university where my interdisciplinary background was appreciated and encouraged, and disciplines were not siloed, and I developed a successful research program tackling questions at the interface of hydrology and biogeochemistry. I currently direct the Reynolds Creek Critical Zone Observatory, one of nine NSF funded Critical Zone Observatories, collaborate on several Critical Zone Thematic Clusters, a newer NSF funded program, and view myself as critical zone scholar.
As a scientist, I am often asked “How is critical zone science different than soil science? How is the critical zone different than an ecosystem? How do I reconcile this tension around the use of the term, critical zone?” Here I suggest that much like a watershed ecosystem (Likens et al., 1970, 1977) or a soil ecosystem (Jenny, 1941), we can reconcile these tensions by recognizing the critical zone as a critical zone ecosystem (Richter and Billings, 2015; Richter et al., 2018). As defined, an ecosystem includes the organisms and their interaction with their environment, and boundaries of ecosystems are defined with a particular question in mind so that ecosystems can vary in spatial scale but they can also vary in temporal scale. A critical zone ecosystem encompasses the top of the canopy to the bottom of the groundwater and includes the biological, chemical, physical and long-term geological processes shaping its structure and function (National Research Council, 2001). In many ways, critical zone science thus more fully expresses the original intent of the word “ecosystem” for going deep as originally advocated by Tansley (1935), Lindeman (1942), and Hutchinson (1948) and more recently by Richter and Billings (2015). For example, Lindeman cored lake sediments because he understood that the sediments below contained clues about lake metabolism and thus extended the lower boundary of his ecosystem of study. Critical zone science thus often delves into deeper spatial and temporal scale questions than previously addressed by soil science and ecology.
While we can reconcile the critical zone as an ecosystem and study its critical zone ecology—the distribution of microbes at greater depths and their capacity to stabilize or destabilize carbon, for example, it is important to recognize that critical zone science truly embraces the integration of earth sciences, ecology, hydrology, and geology to understand the critical zone in a place-based science setting. Multiple perspectives examine the same problem/place through different lenses and approach the system in slightly different ways. Thus, a more integrated approach that harkens backs to early naturalist studies (von Humboldt, 1849; Darwin, 1859; Richter et al., 2018) is achieved through critical zone science (Richter et al., 2018). In practice, longer time scales are often considered, and critical cross scale couplings can also be explored and allow present day process rates to be contextualized. Finally, because critical zone science approaches a problem from multiple perspectives, it also works at the interfaces between fields and allows for gaps in knowledge to be filled.
An example that exemplifies critical zone science is the exploration of the lower boundaries of the critical zone and better quantification of moisture in weathered rock. In many respects, this transition zone or boundary, the interface between soils and rock, has been neglected by soil scientists and geologists because it is not in the purview of either field; it is not fully soil, and it is no longer rock. Critical zone scientists have been digging deeper and “peeling the onion” through modeling and new measurement tools to understand these interfaces between bedrock and saprolite (immobile regolith) and then mobile regolith, the portion of the regolith that shows evidence of mobility and weathering (Riebe et al., 2004; Rempe and Dietrich, 2014; St. Clair et al., 2015). Findings from one CZO, for example, developed a simple empirical model that allows prediction of spatially continuous thickness of the mobile regolith (TMR) using topographic metrics within a catchment and minimal soil measurements (Patton et al., 2018). These findings allow advancements in models of soil carbon, hydrology, weathering, and landscape evolution where the spatial distribution of soil thickness has been a key unknown. Other studies are finding considerable exchangeable moisture in the weathered bedrock (Salve et al., 2012; Rempe and Dietrich, 2018), and that this represents a significant component of the water budget in all critical zone systems and important for resiliency of forests to drought (Bales et al., 2018).
Critical zone science has also contributed to a knowledge gap in the carbon cycle through the quantification of inorganic carbon associated with rocks. Soil inorganic carbon (SIC) stores are significant in arid and semi-arid regions, and pedogenic carbonate often follows a morphogenetic development sequence where carbonates coat rocks, form masses and nodules and eventually may become engulfed and cemented (Gile et al., 1966). The inorganic carbon associated with these carbonate coatings associated with rocks and masses are not quantified by soil scientists because they are >2 mm in size, the operational definition of soil, and carbonate on rocks is difficult to measure owing to the heterogeneity and scale. Consequently, inorganic carbon on rocks is largely ignored by soil scientists and largely overlooked by geologists though isotope records of carbonates are used in reconstructing late Quaternary paleoecological and paleoclimatic conditions (Cerling, 1984; Cerling and Hay, 1986; Cerling and Quade, 1993). As part of the Reynolds Creek CZO, soil scientists identified this problem, and geologists on the team looked at this problem from a rock perspective, and said, “We have a solution”. Rocks with carbonate coatings were pulverized to quantify bulk inorganic carbon on rock, and SIC was also quantified (Stanbery et al., 2023). While this is a brute force and deceptively simple method, these analyses advanced our understanding of the contribution of rock carbonate coatings to total inorganic carbon pools. Findings showed that in some cases, rock carbonate coating can represent as much as 40% of total inorganic carbon in soil profiles (Stanbery et al., 2017) suggesting that studies that only examine the fine fraction of soils (<2 mm) are overlooking a significant store of carbon in gravelly parent materials in arid and semi-arid regions.
A final example stems from discoveries made by going deeper—regarding new taxa and distribution of microbes and carbon in the deep subsurface and role of plants in contributing weathering agents deep in the critical zone. Patton et al. (2019) for example, was able to combine the TMR method described above with soil organic carbon (C) to accurately predict the total amount of soil profile C to saprolite at 3 m resolution, and thus estimate the entire watershed C and nitrogen (N) stores. Findings showed that a significant amount of carbon was stored deep in the critical zone such that total soil C stores were vastly underestimated. Another study by Billings et al. (2018) used long-term managed forests in Calhoun CZO to show the consequence of deforestation, the loss of deep roots, and associated biogenic acids, on weathering and consequently soil formation (Billings et al., 2018). Finally, a cross CZO study of 19 deep soil profiles showed that while similarity in bacteria and archaea taxa and diversity generally decreased with soil depth, five phyla increased with depth, and some profiles did not show declines in diversity (Brewer et al., 2019). More extracellular enzymes were stored at depth than anticipated (Dove et al., 2020). Remaining questions about controls on the distribution of microbial communities and the role that these deeper enzymes play in biogeochemical cycling are being addressed by new research associated with the Critical Zone Thematic Cluster program and other fundings.
As we move forward in scientific discoveries of the earth surface, some of the most exciting discoveries will be at the interface between disciplines and among different scientists, rather within their discipline. Critical zone science offers a scientific platform (Brantley et al., 2017) from which this discovery can take place and a more holistic view of the earth surface. While funding is currently oriented around critical zone thematic clusters, funding programs may morph yet again. I believe that there are opportunities, for example, for advancing our understanding of how humans are impacting the critical zone by measuring “key” critical zone properties and processes such as critical zone architecture and soil formation and weathering across long-term ecological research networks (LTER) and long-term agroecosystem research (LTAR) sites. This approach would require vetting within the community to prioritize these key measurements. Opportunities abound for ecologists, soil scientists, and geologists to contribute to contribute to our understanding of the critical zone.
The original contributions presented in the study are included in the article/supplementary material, further inquiries can be directed to the corresponding author.
The author confirms being the sole contributor of this work and has approved it for publication.
The Reynolds Creek Critical Zone Observatory (NSF 1331872) and Network Cluster: Geomicrobiology and Biogeochemistry in the Critical Zone (2012878) supported this work.
This piece is dedicated to Dr. Thomas Meixner, a great advocate of critical zone science and a stalwart supporter of women in science. The author sincerely thank Drs. Sharon Billings and Daniel Richter for thoughtful comments and encouragement to write this perspective.
The author declares that the research was conducted in the absence of any commercial or financial relationships that could be construed as a potential conflict of interest.
All claims expressed in this article are solely those of the authors and do not necessarily represent those of their affiliated organizations, or those of the publisher, the editors and the reviewers. Any product that may be evaluated in this article, or claim that may be made by its manufacturer, is not guaranteed or endorsed by the publisher.
Bales, R. C., Goulden, M. L., Hunsaker, C. T., Conklin, M. H., Hartsough, P. C., O'Geen, A. T., et al. (2018). Mechanisms controlling the impact of multi-year drought on mountain hydrology. Sci. Rep. 8, 690. doi: 10.1038/s41598-017-19007-0
Billings, S. A., Hirmas, D., Sullivan, P. L., Lehmeier, C. A., Bagchi, S., Min, K., et al. (2018). Loss of deep roots limits biogenic agents of soil development that are only partially restored by decades of forest regeneration. Elementa Sci. Anthrop. 6, 34. doi: 10.1525/elementa.287
Brantley, S. L., McDowell, W. H., Dietrich, W. E., White, T. S., Kumar, P., Anderson, S., et al. (2017). Designing a network of critical zone observatories to explore the living skin of the terrestrial Earth. Earth Surf. Dynam. Discuss. 5, 841–860. doi: 10.5194/esurf-5-841-2017
Brewer, T. E., Aronson, E. L., Arogyaswamy, K., Billings, S. A., Botthoff, J. K., Campbell, A. N., et al. (2019). Ecological and genomic attributes of novel bacterial taxa that thrive in subsurface soil horizons. MBio 10, e01318–01319. doi: 10.1128/mBio.01318-19
Cerling, T. E. (1984). The stable isotopic composition of modern soil carbonate and its relationship to climate. Earth Planet. Sci. Lett. 71, 229–240. doi: 10.1016/0012-821X(84)90089-X
Cerling, T. E., and Hay, R. L. (1986). An isotopic study of paleosol carbonates from Olduvai Gorge. Quaternary Res. 25, 63–78. doi: 10.1016/0033-5894(86)90044-X
Cerling, T. E., and Quade, J. (1993). Stable carbon and oxygen isotopes in soil carbonates. Geophys. Monogr. 78, 217–231. doi: 10.1029/GM078p0217
Darwin, C. (1859). On the Origin of Species by Means of Natural Selection, or, the Preservation of Favoured Races in the Struggle for Life. London: J. Murray.
Dove, N. C., Arogyaswamy, K., Billings, S. A., Botthoff, J. K., Carey, C. J., Cisco, C., et al. (2020). Continental-scale patterns of extracellular enzyme activity in the subsoil: an overlooked reservoir of microbial activity. Environ. Res. Lett. 15, 1040. doi: 10.1088/1748-9326/abb0b3
Gile, L. H., Peterson, F. F., and Grossman, R. B. (1966). Morphological and genetic sequences of carbonate accumulation in desert soils. Soil Sci. 101, 347–360. doi: 10.1097/00010694-196605000-00001
Hutchinson, G. E. (1948). Circular causal systems in ecology. Ann. N. Y. Acad. Sci. 50, 221–246. doi: 10.1111/j.1749-6632.1948.tb39854.x
Likens, G. E., Bormann, F. H., Johnson, N. M., Fisher, D. W., and Pierce, R. S. (1970). Effects of forest cutting and herbicide treatment on nutrient budgets in Hubbard Brook Watershed-ecosystem. Ecol. Monogr.40, 23. doi: 10.2307/1942440
Likens, G. E., Bormann, F. H., Pierce, R. S., Eaton, J. S., and Johnson, N. M. (1977). Biogeochemistry of a Forested Ecosystem. New York: Springer-Verlag.
Lindeman, R. L. (1942). The trophic-dynamic aspect of ecology. Ecology 23, 399–417. doi: 10.2307/1930126
Lohse, K. A., and Dietrich, W. E. (2005). Contrasting effects of soil development on hydrological properties and flow paths. Water Resour. Res. 41:W12419. doi: 10.1029/2004WR003403
Lohse, K. A., and Matson, P. A. (2005). Consequences of nitrogen additions for soil processes and soil solution losses from wet tropical forests. Ecol. Appl. 15, 1629–1648.
National Research Council (2001). Basic Research Opportunities in Earth Science. Washington, DC: The National Academies Press.
Patton, N. R., Lohse, K. A., Godsey, S. E., Seyfried, M. S., and Crosby, B. T. (2018). Predicting soil thickness on soil mantled hillslopes. Nat. Commun. 9, 3329. doi: 10.1038/s41467-018-05743-y
Patton, N. R., Lohse, K. A., Seyfried, M. S., Godsey, S. E., and Parsons, S. (2019). Topographic controls on soil organic carbon on soil mantled landscapes. Sci. Rep. 9:6390. doi: 10.1038/s41598-019-42556-5
Rempe, D. M., and Dietrich, W. E. (2014). A bottom-up control on fresh-bedrock topography under landscapes. Proc. Natl. Acad. Sci. U. S. A. 111, 6576–6581. doi: 10.1073/pnas.1404763111
Rempe, D. M., and Dietrich, W. E. (2018). Direct observations of rock moisture, a hidden component of the hydrologic cycle. Proc. Nat. Acad. Sci. 115, 2664–2669. doi: 10.1073/pnas.1800141115
Richter, D., and Billings, S. A. (2015). ‘One physical system': Tansley's ecosystem as Earth's critical zone. New Phytol. 206, 900–912. doi: 10.1111/nph.13338
Richter, D. D., Billings, S. A., Groffman, P. M., Kelly, E. F., Lohse, K. A., McDowell, W. H., et al. (2018). Strengthening the biogeosciences in environmental research networks. Biogeosci. Discuss. 15, 4815–4832. doi: 10.5194/bg-15-4815-2018
Riebe, C. S., Kirchner, J. W., and Finkel, R. C. (2004). Sharp decrease in long-term chemical weathering rates along an altitudinal transect. Earth Planet. Sci. Lett. 218, 421–434. doi: 10.1016/S0012-821X(03)00673-3
Salve, R., Rempe, D. M., and Dietrich, W. E. (2012). Rain, rock moisture dynamics, and the rapid response of perched groundwater in weathered, fractured argillite underlying a steep hillslope. Water Resour. Res. 48, W11528. doi: 10.1029/2012WR012583
Stanbery, C., Ghahremani, Z., Huber, D. P., Will, R., Benner, S. G., Glenn, N., et al. (2023). Controls on the presence and storage of soil inorganic carbon in a semi-arid watershed. Catena 225, 106980. doi: 10.1016/j.catena.2023.106980
Stanbery, C., Pierce, J. L., Benner, S. G., and Lohse, K. (2017). On the rocks: quantifying storage of inorganic soil carbon on gravels and determining pedon-scale variability. Catena 157, 436–442. doi: 10.1016/j.catena.2017.06.011
St. Clair, J., Moon, S., Holbrook, W. S., Perron, J. T., Riebe, C. S., Martel, S. J., et al. (2015). Geophysical imaging reveals topographic stress control of bedrock weathering. Science 350, 534–538. doi: 10.1126/science.aab2210
Tansley, A. (1935). The use and abuse of vegetational concepts and terms. Ecology 16, 284–307. doi: 10.2307/1930070
Keywords: critical zone, ecosystem, soil science, carbon, water
Citation: Lohse KA (2023) Reconciling critical zone science with ecosystem and soil science—a personal-scientist perspective. Front. Water 5:1196465. doi: 10.3389/frwa.2023.1196465
Received: 29 March 2023; Accepted: 19 June 2023;
Published: 05 July 2023.
Edited by:
Lixin Jin, The University of Texas at El Paso, United StatesReviewed by:
Gregory Todd Carling, Brigham Young University, United StatesCopyright © 2023 Lohse. This is an open-access article distributed under the terms of the Creative Commons Attribution License (CC BY). The use, distribution or reproduction in other forums is permitted, provided the original author(s) and the copyright owner(s) are credited and that the original publication in this journal is cited, in accordance with accepted academic practice. No use, distribution or reproduction is permitted which does not comply with these terms.
*Correspondence: Kathleen A. Lohse, a2xvaHNlQGlzdS5lZHU=
†ORCID: Kathleen A. Lohse orcid.org/0000-0003-1779-6773
Disclaimer: All claims expressed in this article are solely those of the authors and do not necessarily represent those of their affiliated organizations, or those of the publisher, the editors and the reviewers. Any product that may be evaluated in this article or claim that may be made by its manufacturer is not guaranteed or endorsed by the publisher.
Research integrity at Frontiers
Learn more about the work of our research integrity team to safeguard the quality of each article we publish.