- 1Department of Agricultural Economics, University of Zabol, Zabol, Iran
- 2Department of Agricultural Economics, Faculty of Agricultural Engineering and Rural Development, Agricultural Sciences and Natural Resources University of Khuzestan, Mollasani, Iran
The effects of policies on farmers' profit have a key role in their adoption by the farmers and their operationalization at the basin level. The present research explored the effects of water resources conservation policies on optimal cropping pattern and farmers' profit in the Zayanderud basin located Iran using a combined hydrological-economic model composed of WEAP and multi-objective (MOP) models for the assessment of the effects of the A2 climate change scenario with or without the conservation policies. The results showed that climate change will reduce crop yields by 0.5–4%, will decrease water availability by 12–19%, and will increase crop water requirements by 0–4%. It was also found that the adoption of the policy of changing irrigation technology along with the policy of limiting groundwater use will alleviate the effects of climate change on the farmers' profit, will enhance their planned return per unit area by 5%, and will increase the economic efficiency of water use from 5,283 to 6,002 IRR/m3. But, the policy of increasing water price cannot improve the farmers' profit at the basin level and the economic efficiency of water use although it can reduce water use. So, proving the improvement of profit and livelihood of the farmers by applying combined water resources conservation policies can play a significant role in motivating farmers to accept these policies, operationalizing optimal water resources management, and resolving conflicts over water use in this basin.
1. Introduction
Water scarcity has become a serious problem in most low-income countries, and it is increasingly aggravated due to the dramatic growth of the demand for water by different sectors (agricultural, urban, and industrial) (Gohar et al., 2015). The competition on access to available water among the sectors and provinces and the arising conflicts are substantially growing in arid and semi-arid parts of the world. Since irrigation development is the key to the growth of the agricultural sector and the livelihood of rural people, there is an inconsistency between the conservation of water resources and the farmers' interests (Varela-Ortega et al., 2011; Kalbali et al., 2021).
On the other hand, policymakers in critical areas in terms of water shortage are faced with more difficult choices in developing policies on the conservation of water resources to adapt to climate change (Kahil et al., 2016b). Climate change is one of the toughest challenges human being is facing now and will face in the future. This phenomenon, which is a threat to mankind, has influenced different sectors profoundly. As such, climate change is a factor responsible for the variations in available water (Forni et al., 2016; Shirzadi Laskookalayeh et al., 2022). It is, thus, of crucial significance to search the impacts of climate change in attempts to develop and implement water resources conservation policies (Harou et al., 2009; Medellín-Azuara et al., 2010). Furthermore, awareness of farmers' economic decisions under climate change and managerial conditions is important for the analysis of environmental sustainability and available socioeconomic options. Therefore, the implementation of water resources conservation policies under climate change conditions, which aim to improve farmers' revenue, can contribute to not only the sustainability of water resources but also the improvement of the economic efficiency of water use.
On the other hand, the indiscriminate use of nitrogenous fertilizers by farmers and the subsequent discharge of nitrates from agricultural resources have had long-term impacts on groundwater and surface water resources, undermining their quantity and quality (Shirzadi Laskookalayeh et al., 2022). Therefore, it seems imperative to develop a proper pattern for the optimal allocation of water in watersheds so that the quantitative and qualitative goals of the users are accomplished and the water resources in the watershed are consumed sustainably.
In the past that there was no water scarcity (in other words, when the regulated supply of water exceeded the demand for it), water used to be allocated based on physical principles and a set of observed requirements. However, decision-makers presently need to evaluate the economic performance of current and future allocations of water (George et al., 2011). The mathematical programming method (PMP) is an approach widely used for optimal and economic allocation of water resources. Since different users at a watershed level have conflicting goals, the multi-objective optimization models are more efficient in settling these conflicts.
On the other hand, hydrological simulation models reveal the delicate nature of hydrological systems and the management of water, as well as the temporal and spatial limitations of infrastructure. A simulation model can show the different interactions of systems (environmental, urban, agricultural, and industrial systems) in a watershed, the spatial and temporal interdependencies, and the impacts of climate change and economic activities on environmental systems (Reed and Kollat, 2012; Girard et al., 2015). The water evaluation and planning model can feature these characteristics and show the continuing effects of climate change under different regions (Satti et al., 2015; Chenani et al., 2021). Integrated economic-hydrological modeling is a good instrument for developing water resource protection policies on a watershed scale. By integrating economic-hydrological models, it would be possible to include the geographical distribution of water resources, water transfer and storage infrastructure, and water-based economic activities and to establish a unified framework for water-related ecosystems (Kahil et al., 2016a). The advantage of this approach is that it encompasses the internal relationships of hydrological and economic components for the comprehensive assessment of the conflicts that the policies adopted for water resources may pose (Esteban and Albiac, 2012). Water allocation to different uses in a watershed by using an ensemble of economic and hydrological tools is growing in importance because water demands are growing and supply is failing to satisfy these demands (George et al., 2011). When designing integrated models, the economic and hydrological dimensions are considered so that the delicacies of the interactions between water and economy can be tackled.
2. Literature review
Various studies have employed hydrological-economic modeling to explore the effects of water resource conservation policies at a watershed level. Some are listed in Table 1. These models have mostly ignored the climatic conditions and the simulation of the hydrological status in these conditions at the watershed level; rather, they have mostly focused on using the reduction of available water to assess the effects of climate change and drought (Esteve et al., 2015; Mirzaei et al., 2022). It is, therefore, imperative to use comprehensive hydrological-economic modeling that maximizes the economic benefits of all water-using sectors and ensures environmental sustainability and, besides, considers cropping patterns, climate, and soil status at the watershed level and their impacts on regional hydrological conditions and crop yields. To this end, it is necessary to combine hydrological simulation tools with economic models (Esteve et al., 2015; Forni et al., 2016). A comparison of previous studies in terms of water resources conservation policies signify that various policies have proven to be efficient and effective depending on the watershed. For example, the policies of subsidization for water conservation (water storage) and increasing water price have been evaluated for the basin of the Zayanderud River in Iran (Nikouei et al., 2012; Nikouei and Ward, 2013). Therefore, the present study aims to shed light on the impacts of climate change on farmers' profit and evaluate the effects of adopting water resources conservation policies on this factor at the basin level.
To accomplish this goal, a hydrological-economic modeling framework is proposed to answer the questions and challenges of the effects of water resources conservation strategies in the study site, i.e., the basin of the Zayanderud. A review of the literature shows that this basin has never been subject to such a study in which the hydrological simulation of system is combined with an economic multi-objective optimization model. Indeed, the preceding studies have mostly considered the connections of hydrological components in the context of water inflows and outflows. But, to assess the impacts of climate change scenarios, it is necessary to hydrological simulate a basin by using data on climate, soil, crop cultivation, and geography of the region. So, empirical functions should be developed for the individual farming units to simulate evapotranspiration, surface flows and runoffs, relative changes in soil moisture, and deep infiltration into groundwater (Li et al., 2017).
3. Study site
The drainage basin of the Zayanderud is a major basin in the central plateau of Iran covering an area of over 41,000 km2 (Figure 1). Based on the long-term statistics, the discharge of the Zayanderud Dam is about 1.5 billion m3/yr, the amount of water of the river consumed by the agricultural sector, including surface and groundwater resources, is over 4,400 million m3/yr, and the amount of chemical fertilizers and pesticides applied to the farms of this basin (about 220,000 ha) is over 100,000 and 450 t, respectively (Abedi Kupa'i et al., 2010). Since irrigation efficiency in this sector is very low, as estimated by research at about 42 percent, it is crucial to improve the economic efficiency of irrigation water use (Anonymous, 2016a). On the other hand, the mean precipitation of the basin is 130 mm, and its mean monthly temperature varies from 3 to 29°C, indicating its unfavorable climatic conditions. Additionally, recent droughts and the consequent limitation of water resources in this basin, the excessive growth of groundwater abstraction, population concentration, and the development of industrial and agricultural activities along the river have made the supply of high-quality water for different sectors, including the Gavkhouni marsh located at the end of the basin, a key challenge (Anonymous, 2016b). It is, thus, imperative to develop and implement policies for the conservation of water resources with an emphasis on satisfying the economic goals of farmers under climate change at the basin level in the future.
4. Materials and methods
4.1. Conceptual framework
Figure 2 depicts a general understanding of the concept of water resources management in the Zayanderud basin under climatic uncertainties. It is evident that a rotational model was employed. At first, the optimal cropping pattern and economic benefit per m3 water were determined for different farming units (with or without adaptive strategies) by solving a multi-objective model to satisfy the benefits of the farming units and the environmental needs. Then, while applying adaptive strategies, the water demand of each unit was fed into the WEAP model as an input, and the monthly flows for the supply of the water demands were simulated in the context of different climatic scenarios. In the second stage, the crop yields and water demand simulated by WEAP at the base state and different adaptive policies under various climatic conditions were inputted into the economic-environmental multi-objective optimization model to derive the optimal cropping pattern. Finally, the WEAP was applied to simulate the hydrological conditions of the basin. In the context of this model, the effects of water resources protection policies he economic situation of farmers can also be surveyed.
4.2. The WEAP hydrological simulation model under different climatic scenarios
Hydrological simulation under different climatic scenarios should be performed in WEAP system (software package) and its integration with the MABIA tool. The climate change scenario used in the study was derived from Nikouei and Ward (2013) in which the A2 and B2 climate change scenarios were assessed for the Zayanderud basin. That study simulated the two scenarios by using the HadCM3 (GCM) model for the basin in the 2040 horizon. The results showed that the effects of the A2 scenario were much more severe on the precipitation rate, mean basin surface temperature, and the rate of water flow of the river than the B2 scenario. In other words, scenario A2 is a pessimistic scenario and scenario B2 is optimistic. Due to the weather conditions and consecutive droughts in Isfahan province (Mardani Najafabadi et al., 2019), pessimistic scenario A2 is examined in this study. This scenario at the level of the Zayanderud basin resulted in a 5.8% decrease in the river's flow rate, a 4.6% increase in temperature, and a 10% decrease in precipitation. Therefore, the present study evaluated the impacts of the most pessimistic climate change scenario (A2).
The WEAP model is based on the principle of water balance and with a comprehensive view of infrastructure, water flows and water transfer channels, redistributes water between water demand nodes in different sub-basins (Yates et al., 2005). This model investigates a hydrological cycle using a time series of climatic data and simulation of rainfall-runoff processes. Figure 3 displays the network of nodes and inflows and outflows within the study site of the Zayanderud River and the implementation of this network in WEAP software (Figure 3).
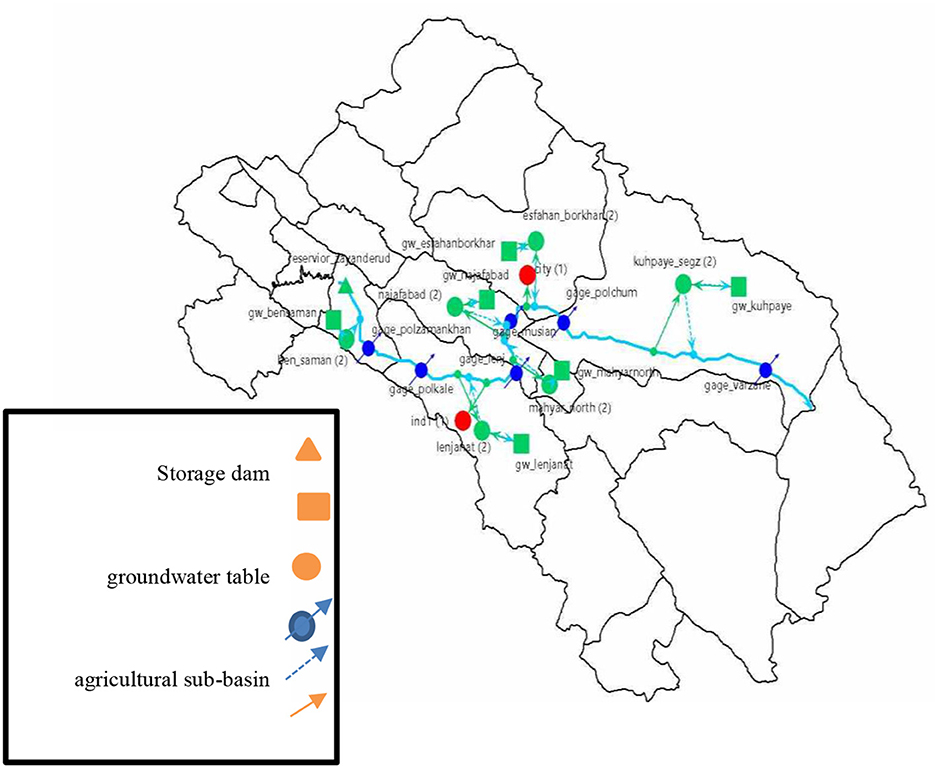
Figure 3. The network of nodes and inflows and outflows within the study site of the Zayanderud River in WEAP software.
This model employs empirical functions to describe and simulate evapotranspiration, runoff and surface flows, soil moisture variations, the baseflow trend of the river, and deep infiltration to the groundwater for each agricultural unit. One of the main advantages of WEAP is the simulation of water systems using an integrated approach that leads to its policy orientation. The WEAP solves a water mass balance equation for each node and link of the system in time steps. To supply the users' demands and the downstream flow rate, water is distributed based on the demand priorities, resource superiority, mass balance equation, and other constraints. The water demand of a demand node (SD) is defined as the sum of the demands of its base branches (Br). A base branch is a branch that there are no branches under it.
In Equation (1), AnuDem is the annual demand, TotActLev is the total activity level, and WatUseRat is the water use rate. The monthly demand represents the water requirement of a node for its own use in each month, whereas the source requirement is the actual amount of the demand made from the reserve resources. The source requirement considers the demand and adjusts it with the amount of reuse inside the node and managerial strategies to reduce the demand and internal losses. So, we have
In which MonSouReq is the monthly source requirement, MonDem is the monthly demand, ReuRat is the reuse rate, DemSidMonSto is the demand-side monthly storage, and LosRat is the loss rate.
At this stage, the input flows of the nodes and links in the system and the outputs considered for the month are calculated. This calculation includes the water abstracted from the storage resources to meet the demands. In order to supply the requirements maximally by applying the defined constraints, a linear program (LP) is used. So, if SD is the node demand and Scr is the supply source, we have
In which Inp represents the input and TraLinOut represents the transfer line's output flow. The governing equations of the transfer line are defined by Equations (4)-(7).
in which the subscripts of DS and Src are the flow from the source to the demand node, TraLinOut is the output of the transfer line, TraLinInp is the input of the transfer line, TraLinLos is the water loss of the transfer line, TraLinLosSys is the water loss of the transfer line from the system, TraLinLosGro is the water loss of the transfer line that goes to the groundwater table, MaxFloVol is the maximum flow volume, MaxFloPer is the maximum flow percentage, and SouReq is the source requirement. The output water amount from the reservoir is also estimated by the following equation:
In which OutFlo is the output flow, and DowOutFlo is the output flow to the downstream. This equation is solved by the following constraint in which ResAva shows the reserve available for output.
To estimate crop yields, net water requirements, and the amount of available water in different sub-basins, the MABIA tool was used. This tool is an instrument to simulate daily transpiration, evaporation, and crop growth and also includes units for the estimation of evapotranspiration. The present study employed the MABIA tool and its integration with WEAP for the hydrological simulation and the assessment of the impacts of climate change on this simulation at the basin level.
4.3. Hydrological-economic model at the basin level
A hydrological-economic model considers the mutual relations of different uses (agricultural, urban, and industrial) and the available water (derived from the WEAP simulation model). The inputs of the hydrological model simulated by the WEAP model for the horizon 2040.
4.3.1. Economic objective
The economic objective is to maximize the total benefit of water uses all sectors (S) include the agricultural (a), urban (u), and industrial (i) within the hydrological and land constraints at the basin level.
In Equation (10), TotBen is the total benefit and TotCos is the total cost of water use by the agricultural, urban, and industrial sectors. The following equation separately shows how the benefits of each sector are calculated.
in which Pric is the crop (c) price, Yei, j, k is the crop yield with different irrigation technologies (k) in different regions (j) as derived from the output of the WEAP model and its integration with the MABIA tool, CulArec, j, k shows the decision variable of the model, WatPri is the price of each unit of water use, ProCosc, j, k is the production cost excluding the cost of water use for each crop with different irrigation technologies in different regions, NetWatReqc, j, k shows the net water requirement of crops with different irrigation technologies in different regions, β and α are coefficients of urban and industrial water use, respectively, and WatUrburb and WatIndind are the decision variables of water use in urban and industrial sectors. Finally, the multi-objective model of the study was solved by the lexicographic method with priorities for urban, agricultural, and industrial goals, respectively.
4.3.2. Hydrological and land constraints
The hydrological constraint shows that the amount of water use of the crops (c) with different irrigation technologies (k) in each region (j) is less than the volume of water available in different regions.
in which AvaWatj is the water available in different regions per each unit of the cultivated area (available land), and AvaLanj is the available land of different regions whose parameters, including NetWatReq (crop net water requirement) and AvaWat (available water of the regions), were the result of the WEAP model.
Total land constraint shows that the total cultivated area with different irrigation technologies in a certain region should be less than the available land of that region.
Now we could apply water resources conservation policies to the multi-objective optimization programming model and explore the impacts of the individual policies on the optimal cropping pattern and subsequently on the livelihood of farmers under different climatic conditions. The present research focused on the following conservation policies:
Base conditions in which no policy as to the conservation of water resources is adopted (p).
Constraint on groundwater use (through applying constraints on using well water and issuing permits for digging water wells) (s1).
A combination of groundwater use limitation policy and irrigation technology change (a change for surface irrigation to sprinkler and drip irrigation) (s1+s2).
A combination of groundwater use limitation policy, irrigation technology change, and higher water price (s1+s2+s3).
5. Results and discussion
5.1. Hydrological simulation
The simulation is first verified and assessed for Zayanderud basin under base conditions and the A2 climate change scenario for three variables of crop yield, crop water requirement, and the amount of water supply (available water) in different regions of the Zayanderud basin for the 2011–2039 period (the 2040 horizon).
5.1.1. Verification of simulation
The simulation made in the WEAP software was verified with the so-called PEST instrument. PEST users automatically compare the outputs of a model with observational data and adjust the model parameters to increase its calibration accuracy. This tool uses the data recorded in hydrometry stations (water flow volume) to estimate the accuracy and calibrate of the model. To verify the simulation and calibrate the model, the present research employed the observational data recorded for monthly water inflow volume to the Zaman Khan Bridge (ZKB) hydrometry station in 2011 (available data) and compared it with the output of the simulated water flow of Ben-Saman located before the hydrometry station (Figure 4). The comparison showed that the model was calibrated with high precision because the simulated upstream outflow of the hydrometry station almost perfectly matches the actual inflow observed in the ZKB hydrometry station.
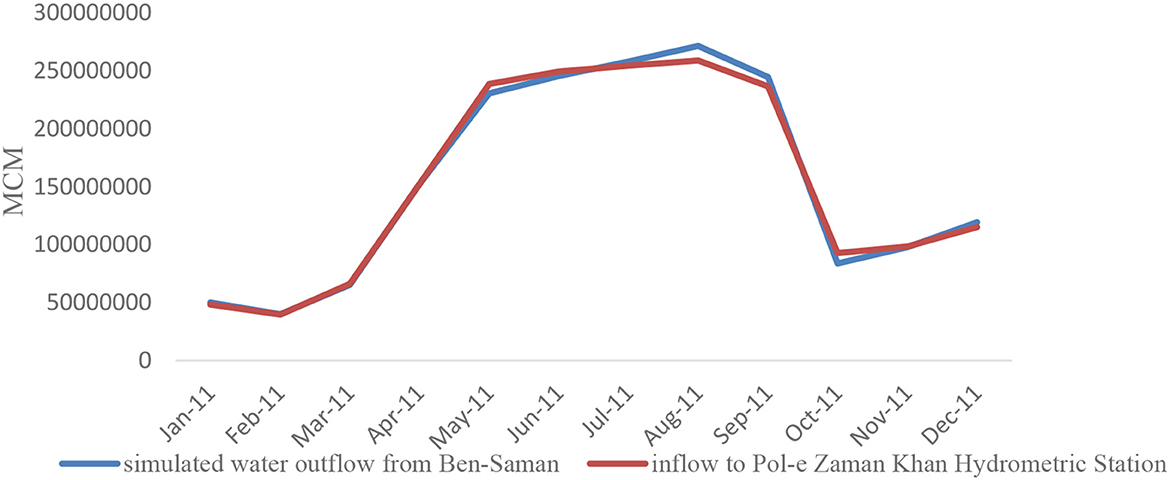
Figure 4. The comparison of observational data on the flow volume of the river with the simulated data.
5.1.2. Simulation crop yield
The MABIA tool was used to simulate the yield of different crops with and without the A2 climate change scenario and under the application of conservative policy of limitation on groundwater use (S1) over the 2011–2039 period for different regions of the basin. The results were derived and estimated for the regions separately at the basin level (Table 2).
Table 2 shows that under climate change and limitation on groundwater use, the yield of all crops will decrease by 0.4–4%. Vegetables and potatoes will suffer the greatest loss in their yields. The least changes in yield under these conditions were observed to be for rice, grain corn, and forage corn. So, it can be understood that climate change and limiting groundwater use to bring a balance in groundwater tables will not greatly affect the yield of crops at the basin level.
5.1.3. Simulation of net water requirement
The net water requirement of different crops was simulated using an integration of the MABIA tool and WEAP model for different regions with and without the A2 climate change scenario and by applying the conservative policy of groundwater use limitation over the 2011–2039 period. Table 3 presents the results for each region at the basin level.
Based on Table 3, the net water requirement of most crops will increase by climate change and imposing limitations on groundwater use. The sugar beet crop shows the greatest increase in net water requirement. Also, almost no changes will happen in the net water requirement of rice and vegetables under the climate change scenario and limited use of groundwater resources. In general, it is inferred that the net water requirements of the crops at the basin level are not significantly affected by climate change and imposing limitations on groundwater use aimed at creating a balance in groundwater tables.
5.1.4. Simulation of available water
This section presents the results for the impact of climate change on the water supply to different regions of the basin when limitations are applied to groundwater use (Table 4). This assessment reveals which region's water scarcity will be more strongly affected by climate change.
According to Table 4, climate change will reduce water availability in all sub-basins. The Lenjanat sub-basin will show the greatest decline in its available water (~19%) due to climate change and limiting groundwater use, whereas the lowest decline (~12%) in water availability will happen in the Northern Mahyar sub-basin. It is, therefore, concluded that climate change and imposing limitations on groundwater use will adversely affect water supply in the future.
5.2. Impacts of climate change and water resources conservation policies
First, the multi-objective model was solved by the lexicographic method with the priorities for urban, agricultural, and industrial goals under the base conditions and optimal allocation of water among the sectors. The results in Table 5 show that the water use of all sectors under the status quo in the base year is higher than the optimal water allocated by the model. This means that by optimal allocation of water to the sector, water use can be reduced remarkably, especially in the agricultural and industrial sectors, so that the use of the optimal cropping pattern in the agricultural sector will allow reducing water use by about 31%. Similar results have been reported by Shirzadi et al. (2020), Shirzadi Laskookalayeh et al. (2022), and Kalbali et al. (2021) for different basins. However, the share of the water used by the agricultural sector does not significantly differ between the status quo and the optimal conditions, and this sector is accountable for over 85% of the water use at the basin level in both conditions. So, the effects of water resources conservation policies under climate change are explored below.
Table 6 shows the gross return per ha after the application of the A2 scenario and the water resources conservation policies. The results indicate that climate change and the policy of limiting groundwater use (A2 + S1) can reduce the total gross return of the farmers by about 5% vs. the base conditions. This reflects that climate change and limiting groundwater use will impair the farmers' revenue. The results reveal that the North Mahyar sub-basin will be influenced by climate change and the policy of groundwater use limitation most negatively (~14%). This is related to the fact that the optimal cropping pattern in this sub-basin shifts from high-yielding (e.g., sugar beets) to low-yielding products with lower water requirements (Figure 5). In contrast, climate change and the policy of groundwater use limitation will have no impact on the farmers' profit in the Kuhpayeh-Sagzi sub-basin because, according to Figure 5, the optimal cropping pattern does not differ from the current conditions significantly.
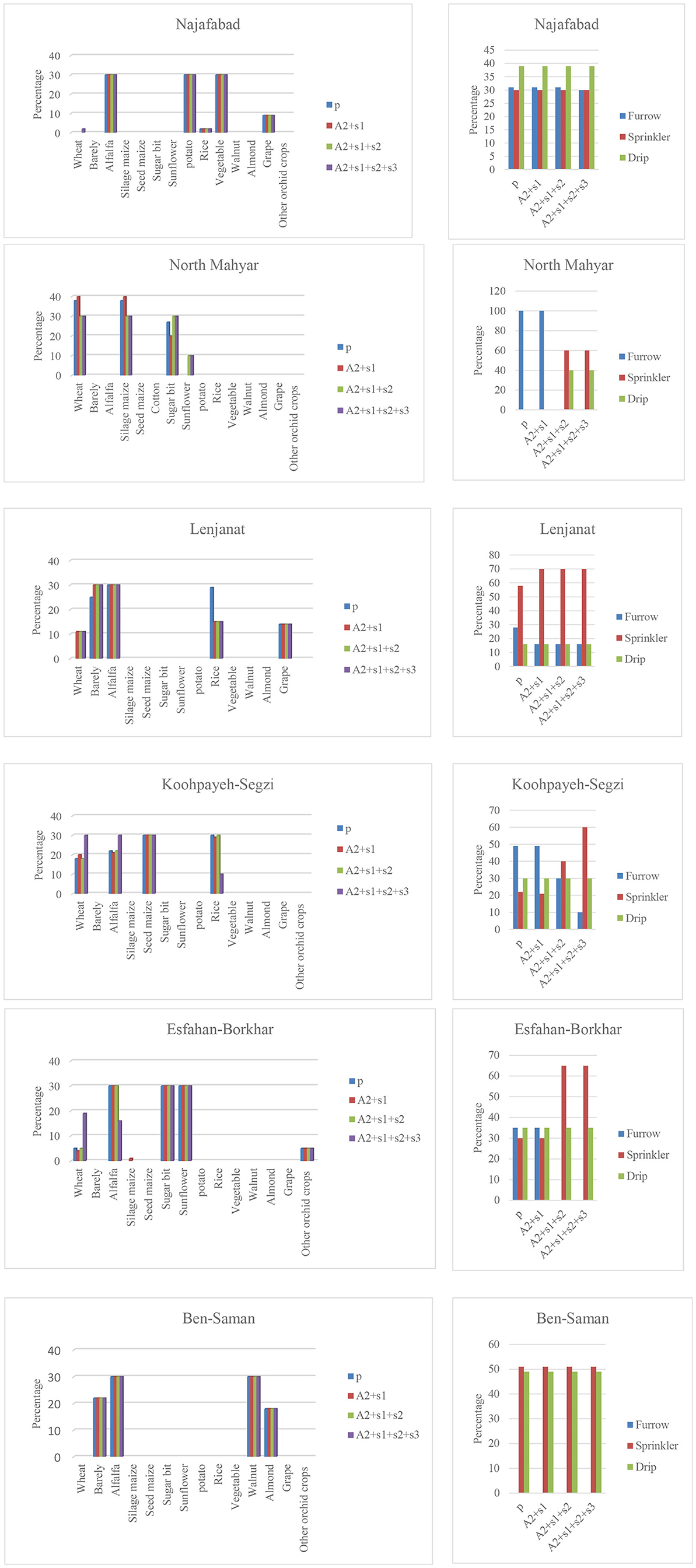
Figure 5. The optimal cropping pattern for different sub-basins in the context of the water resources conservation policies.
The results for the adoption of the policy of changing irrigation technology from surface to modern technology (sprinkler and drip) reveal that this policy will mitigate the negative impacts of climate change on the farmers' profit or gross return so that it will increase the farmers' gross return to about 62 million IRR/ha, which is equivalent to the gross return of the base conditions. In other words, the policy of using modern irrigation systems will increase the farmers' gross return by 3 million IRR/ha vs. climate change and limited groundwater use conditions. Based on Table 6, this policy can increase the economic efficiency of water use from 5,283 to 6,002. So, it is concluded that under climate change conditions, this policy accompanied by the policy of limited groundwater use can contribute to reducing water use, thereby improving the economic efficiency of water use, with no adverse impact on farmers' profit. The results show that the adoption of the policy of changing irrigation technology under the climate change and limited groundwater use conditions can even increase the farmers' gross return vs. the base conditions in three sub-basins of Northern Mahyar, Isfahan-Borkhar, and Kuhpayeh-Sagzi. This increase is remarkably as high as +64% for the Northern Mahyar sub-basin. It is observed in Figure 5 that this policy in the Northern Mahyar sub-basin results in the replacement of a crop like wheat with sugar beet in the optimal cropping pattern. Also, crops with surface irrigation technologies replace crops with modern irrigation technologies. However, this policy cannot increase farmers' profit vs. climate conditions and limited groundwater use in the sub-basins of Najafabad, Lenjanat, and Ben-Saman. This is associated with the fact that the optimal cropping pattern of these sub-basins does not change vs. that under climate change and limited groundwater use conditions (Figure 5).
Based on the results, the policy of increasing water prices, changing irrigation technology to modern technologies, and limiting groundwater use not only has no effect on modifying the climate change consequences on farmers' profit, but it will also weaken their revenue so that the farmers' gross return will decrease after the adoption of this policy by about 15% vs. the base conditions and by about 10% vs. the conditions of climate change and the adoption of the policy of limiting groundwater use (Table 6). Although this combination of policies can play an effective role in reducing water use, the economic efficiency of water use shows that the reduction of the farmers' gross return exceeds the reduction of water use. Indeed, the economic efficiency of water use is decreased from 6,002 to 5,901 IRR/ha when the policy of higher water price is adopted (Table 7). Thus, the policy of increasing water price along with the other policies cannot be effective because it jeopardizes the farmers' profit on the one hand and fails to improve the economic efficiency of water use despite reducing water use at the basin level on the other. The results show that the combination of these policies reduces the farmers' profit in all sub-basins except for the Northern Mahyar sub-basin (Table 5). The positive effect of some policies on improving farmers' revenue has been established in other research works (Varela-Ortega et al., 2011; Danshgar et al., 2021). The inconsistency of the results proves the dependence of water resources management on spatial and temporal conditions as has been reported in other studies too (Esteve et al., 2015; Danshgar et al., 2021).
6. Conclusions
The research evaluated the impacts of water resources conservation policies on the farmers' profit at the Zayanderud basin. The results revealed that the total water use across the basin can be reduced by about 28% through optimal water allocation among the opposing sectors. But climate change will aggravate the conditions in the future, so it is imperative to present approaches to alleviating these effects and conserving water resources in the agricultural sector. To assess the effects of climate change on hydrological conditions, the present study employed the WEAP tool for the hydrological simulation and the assessment of the impacts of climate change. The results indicated that climate change will reduce crop yields and water availability in regions and increase the water requirement of crops. It can be inferred from various studies that the adoption of policies and strategies as to water resources management requires their acceptance by farmers. In this sense, policies that do not jeopardize the farmers' profit and livelihood and may even potentially improve them have a greater chance for acceptance.
So, the present study analyzes farmers' incomes by applying climate change conditions as well as conservation policies. One of the effective policies in balancing groundwater is the change of irrigation technologies along with the policy of limiting the use of this water source, which can reduce the effects of climate change on the gross revenue of the farmers. Increasing the economic efficiency of water consumption will also be one of the positive consequences of implementing this policy. But, the policy of increasing water price will not increase farmers' profit and the economic efficiency of water use, although it will reduce water use. Hence, if it is proven to the farmers that the combined water resources conservation policies will enhance their revenue, they can be effectively motivated to accept them, rendering it possible to optimally manage water resources and resolve the conflicts over water use in the basin.
The results for the different sub-basins in the Zayanderud basin shows that it is feasible to recognize region-specific appropriate policies (Westerhoff and Smit, 2008). In addition, unlike the public belief, the adoption of some of these policies in some units may increase the farmers' gross return in addition to improving the hydrological performance (Esteve et al., 2015; Shirzadi Laskookalayeh et al., 2022). The results prove the dependence on regional and behavioral conditions of farmers. So, it is recommended to carry out studies on each particular basin and each particular region because there is no single pattern or policy that can be viably applied for water resources management of all basins and regions. As such, one of the main tasks of policymakers for accomplishing a successful water resources management practice is to develop region-specific policies on the conservation of water resources. Then, the success of these policies will require motivating farmers in order to increase the social acceptance of the policies for which increasing the profitability of the activities by the adoption of the policies can be an effective incentive. It is also recommended to focus on other motivational approaches by analyzing farmers' behavior in each region in order to facilitate operationalizing policies at a basin level. Considering that the nature of climate change phenomena is random, it seems that the use of other mathematical planning models, especially models that include uncertainty conditions, can be a great help to solve this problem. Of course, the use of other climate change reports can also give researchers and decision makers a more appropriate view.
Data availability statement
The raw data supporting the conclusions of this article will be made available by the authors, without undue reservation.
Author contributions
Material preparation and data collection and analysis were performed by MN and SZ. The first draft of the manuscript was written by HK. All authors commented on previous versions of the manuscript, contributed to the study conception and design, and read and approved the final manuscript.
Conflict of interest
The authors declare that the research was conducted in the absence of any commercial or financial relationships that could be construed as a potential conflict of interest.
Publisher's note
All claims expressed in this article are solely those of the authors and do not necessarily represent those of their affiliated organizations, or those of the publisher, the editors and the reviewers. Any product that may be evaluated in this article, or claim that may be made by its manufacturer, is not guaranteed or endorsed by the publisher.
References
Abedi Kupa'i, J., Nasri, Z., Talebi, K., Mamanpoosh, A., and Mousavi, S. F. (2010). Chemical quality and pollution of zayandehrud water to diazinon at upstream and its self-purification capacity. Agri. Nat. Res. Sci. Technol. 15, 1–19.
Anonymous. (2016a). Comprehensive Reports of Water Resources in the Zayanderud Basin. Isfahan: Isfahan Regional Water Company.
Anonymous. (2016b). Reports of Integrated Management of Use and Water Resource Abstraction Control of the Zayandehrud Basin in the Upstream Zayanderud Dam. Isfahan: Iran's Water Resources Management Company.
Blanco-Gutiérrez, I., Varela-Ortega, C., and Purkey, D. R. (2013). Integrated assessment of policy interventions for promoting sustainable irrigation in semi-arid environments: a hydro-economic modeling approach. J. Environ. Manag. 128, 144–160. doi: 10.1016/j.jenvman.2013.04.037
Chenani, E., Yazdanpanah, M., Baradaran, M., Azizi-Khalkheili, T., and Mardani Najafabadi, M. (2021). Barriers to climate change adaptation: Qualitative evidence from southwestern Iran. J. Arid Environ. 189, 104487. doi: 10.1016/j.jaridenv.2021.104487
Danshgar, H., Bagheri, m., and Mardani Najafabadi, M. (2021). Evaluation of consequences of climate change and adaptation strategies in bushkan plain of Bushehr Province. J. Agricult. Econo. Develop. 35, 63–78. doi: 10.22067/jead.2021.17812.0
Esteban, E., and Albiac, J. (2012). The problem of sustainable groundwater management: the case of La Mancha aquifers, Spain. Hydrogeol. J. 20, 851–863. doi: 10.1007/s10040-012-0853-3
Esteve, P., Varela-Ortega, C., Blanco-Gutiérrez, I., and Downing, T. E. (2015). A hydro-economic model for the assessment of climate change impacts and adaptation in irrigated agriculture. Ecol. Econ. 120, 49–58. doi: 10.1016/j.ecolecon.2015.09.017
Forni, L. G., Medellín-Azuara, J., Tansey, M., Young, C., Purkey, D., and Howitt, R. (2016). Integrating complex economic and hydrologic planning models: an application for drought under climate change analysis. Water Res. Econo. 16, 15–27. doi: 10.1016/j.wre.2016.10.002
George, B., Malano, H., Davidson, B., Hellegers, P., Bharati, L., and Massuel, S. (2011). An integrated hydro-economic modelling framework to evaluate water allocation strategies I: model development. Agricult. Water Manag. 98, 733–746. doi: 10.1016/j.agwat.2010.12.004
Girard, C., Rinaudo, J.-D., Pulido-Velazquez, M., and Caballero, Y. (2015). An interdisciplinary modelling framework for selecting adaptation measures at the river basin scale in a global change scenario. Environ. Modell. Software 69, 42–54. doi: 10.1016/j.envsoft.2015.02.023
Gohar, A. A., Amer, S. A., and Ward, F. A. (2015). Irrigation infrastructure and water appropriation rules for food security. J. Hydrol. 520, 85–100. doi: 10.1016/j.jhydrol.2014.11.036
Gohar, A. A., and Ward, F. A. (2010). Gains from expanded irrigation water trading in Egypt: An integrated basin approach. Ecol. Econ. 69, 2535–2548. doi: 10.1016/j.ecolecon.2010.07.030
Gohar, A. A., Ward, F. A., and Amer, S. A. (2013). Economic performance of water storage capacity expansion for food security. J. Hydrol. 484, 16–25. doi: 10.1016/j.jhydrol.2013.01.005
Gürlük, S., and Ward, F. A. (2009). Integrated basin management: Water and food policy options for Turkey. Ecol. Econ. 68, 2666–2678. doi: 10.1016/j.ecolecon.2009.05.001
Harou, J. J., Pulido-Velazquez, M., Rosenberg, D. E., Medellín-Azuara, J., Lund, J. R., and Howitt, R. E. (2009). Hydro-economic models: Concepts, design, applications, and future prospects. J. Hydrol. 375, 627–643. doi: 10.1016/j.jhydrol.2009.06.037
Kahil, T., Ward, F., Albiac, J., Eggleston, J., and Sanz, D. (2016a). Hydro-economic modeling with aquifer-river interactions to guide sustainable basin management. J. Hydrol.. doi: 10.1016/j.hydrol.2016.05.057
Kahil, T., Ward, F., Albiac, J., Eggleston, J., and Sanz, D. (2016b). Hydro-economic modeling with aquifer–river interactions to guide sustainable basin management. J. Hydrol. 539, 510–524. doi: 10.1016/j.jhydrol.2016.05.057
Kalbali, E., Ziaee, S., Najafabadi, M. M., and Zakerinia, M. (2021). Approaches to adapting to impacts of climate change in northern Iran: the application of a hydrogy-economics model. J. Clean. Prod. 280, 124067. doi: 10.1016/j.jclepro.2020.124067
Li, J., He, L., Chen, Y., Song, X., and Lu, H. (2017). A bilevel groundwater management model with minimization of stochastic health risks at the leader level and remediation cost at the follower level. Stochastic Environ. Res. Risk Assess. 31, 2547–2571. doi: 10.1007/s00477-016-1351-2
Mardani Najafabadi, M., Mirzaei, A., Azarm, H., and Nikmehr, S. (2022). Managing water supply and demand to achieve economic and environmental objectives: application of mathematical programming and ANFIS models. Water Res. Manag. 36, 3007–3027. doi: 10.1007/s11269-022-03178-1
Mardani Najafabadi, M., Ziaee, S., Nikouei, A., and Ahmadpour Borazjani, M. (2019). Mathematical programming model (MMP) for optimization of regional cropping patterns decisions: a case study. Agricult. Sys. 173, 218–232. doi: 10.1016/j.agsy.2019.02.006
Medellín-Azuara, J., Harou, J. J., and Howitt, R. E. (2010). Estimating economic value of agricultural water under changing conditions and the effects of spatial aggregation. Sci. Total Environ. 408, 5639–5648. doi: 10.1016/j.scitotenv.2009.08.013
Mirzaei, A., Azarm, H., Yazdanpanah, M., and Mardani Najafabadi, M. (2022). Socio-economic, social-capital, and psychological characteristics and climate change adaptive behavior of farmers in Iran. Clim. Res. 87, 1–12. https://www.int-res.com/abstracts/cr/v87/p1-12/
Nikouei, A., and Ward, F. A. (2013). Pricing irrigation water for drought adaptation in Iran. J. Hydrol. 503, 29–46. doi: 10.1016/j.jhydrol.2013.08.025
Nikouei, A., Zibaei, M., and Ward, F. A. (2012). Incentives to adopt irrigation water saving measures for wetlands preservation: An integrated basin scale analysis. J. Hydrol. 464, 216–232. doi: 10.1016/j.jhydrol.2012.07.013
Reed, P. M., and Kollat, J. B. (2012). Save now, pay later? Multi-period many-objective groundwater monitoring design given systematic model errors and uncertainty. Adv. Water Res. 35, 55–68. doi: 10.1016/j.advwatres.2011.10.011
Satti, S., Zaitchik, B., and Siddiqui, S. (2015). The question of Sudan: a hydro-economic optimization model for the Sudanese Blue Nile. Hydrol. Earth Syst. Sci. 19, 2275–2293. doi: 10.5194/hess-19-2275-2015
Shirzadi Laskookalayeh, S., Mardani Najafabadi, M., and Shahnazari, A. (2022). Investigating the effects of management of irrigation water distribution on farmers' gross profit under uncertainty: a new positive mathematical programming model. J. Clean. Prod. 351, 131277. doi: 10.1016/j.jclepro.2022.131277
Shirzadi, S., Mardani, M., and Shahnazari, A. (2020). Water consumption and distribution management in tajan irrigation and drainage network with emphasis on water resource sustainability. J. Water Res. Agri. 33, 673–689.
Varela-Ortega, C., Blanco-Gutiérrez, I., Swartz, C. H., and Downing, T. E. (2011). Balancing groundwater conservation and rural livelihoods under water and climate uncertainties: an integrated hydro-economic modeling framework. Glob. Environ. Change 21, 604–619. doi: 10.1016/j.gloenvcha.2010.12.001
Ward, F. A., and Pulido-Velazquez, M. (2009). Incentive pricing and cost recovery at the basin scale. J. Environ. Manag. 90, 293–313. doi: 10.1016/j.jenvman.2007.09.009
Westerhoff, L., and Smit, B. (2008). The rains are disappointing us: dynamic vulnerability and adaptation to multiple stressors in the Afram Plains, Ghana. Mitig. Adap. Strateg. Glob.Change 14, 317. doi: 10.1007/s11027-008-9166-1
Keywords: climate change, cropping pattern, economic efficiency of water, multi-objective programming, WEAP-MABIA, Zayanderud basin
Citation: Kavand H, Ziaee S and Mardani Najafabadi M (2023) The impact of water conservation policies on the reallocation of agricultural water-land resources. Front. Water 5:1138869. doi: 10.3389/frwa.2023.1138869
Received: 06 January 2023; Accepted: 28 February 2023;
Published: 17 March 2023.
Edited by:
Naser Valizadeh, Shiraz University, IranReviewed by:
Somayeh Naghavi, University of Jiroft, IranMasoud Bijani, Tarbiat Modares University, Iran
Hassan Azarm, Shiraz University, Iran
Copyright © 2023 Kavand, Ziaee and Mardani Najafabadi. This is an open-access article distributed under the terms of the Creative Commons Attribution License (CC BY). The use, distribution or reproduction in other forums is permitted, provided the original author(s) and the copyright owner(s) are credited and that the original publication in this journal is cited, in accordance with accepted academic practice. No use, distribution or reproduction is permitted which does not comply with these terms.
*Correspondence: Mostafa Mardani Najafabadi, bS5tYXJkYW5pJiN4MDAwNDA7YXNucnVraC5hYy5pcg==