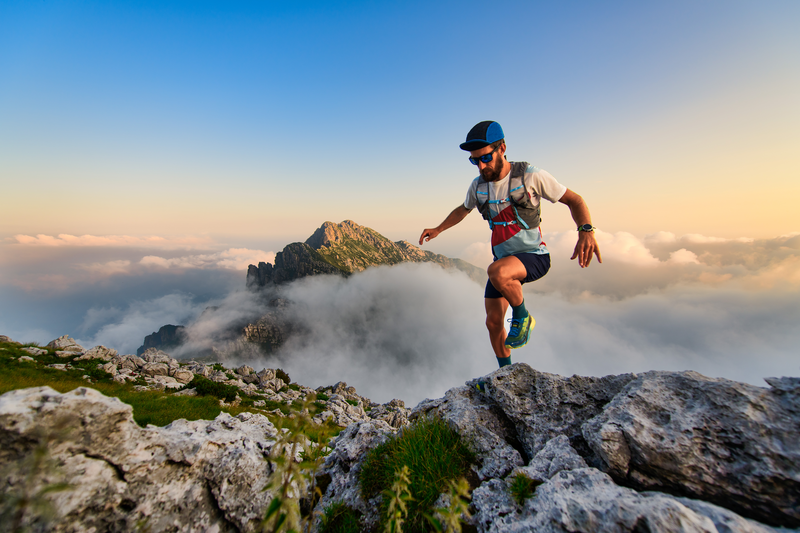
94% of researchers rate our articles as excellent or good
Learn more about the work of our research integrity team to safeguard the quality of each article we publish.
Find out more
ORIGINAL RESEARCH article
Front. Water , 09 June 2023
Sec. Water and Critical Zone
Volume 5 - 2023 | https://doi.org/10.3389/frwa.2023.1115416
This article is part of the Research Topic Threatened Aquatic Gems: Freshwater Springs and Groundwater-Dependent Ecosystems View all 9 articles
Groundwater is an important freshwater supply for agricultural, domestic, and environmental uses and critical buffer against a warming climate, particularly in semi-arid and arid regions of the world. Groundwater dependent ecosystems (GDEs), which rely on groundwater for some or all of their water requirements, include terrestrial vegetation, rivers, springs, wetlands, and riparian zones. These GDEs provide benefits to people ranging from habitat for pollinators to carbon sequestration. Accounting for these benefits, called ecosystem services, can inform management by expanding the potential group of groundwater users to include groundwater dependent ecosystems. Here we develop an approach to inventory the ecosystem services of GDEs by identifying the ecosystem functions of a range of GDEs and assessing how they are linked to a wide range of ecosystem services. We apply this approach as a case study in California, USA, where we found ecosystem services from GDEs is widespread across the state; over 30% of California's pollinator dependent crops may benefit from GDEs, and carbon storage of GDEs is equivalent to 790 million tons, twice as much as California emits annually.
Groundwater is a critical water supply for agriculture, industry, and domestic use, leading to a growing body of research on tradeoffs among water users and optimization among multiple objectives (Velis et al., 2017). However, one aspect of groundwater that is frequently not accounted for is its role in supporting unique ecosystems—groundwater dependent ecosystems (GDEs). GDEs rely on groundwater for some or all of their water requirements (Eamus et al., 2006) and include terrestrial vegetation and aquatic ecosystems. GDEs provide a wide range of benefits to society, including flood risk reduction, carbon sequestration, climate regulation, and recreational opportunities (Murray et al., 2006). When GDEs are impacted by groundwater management decisions, those benefits may be affected. Thus, accounting for the benefits provided by GDEs can offer new information when evaluating acceptable tradeoffs associated with groundwater extraction.
The benefits of nature are frequently categorized and analyzed using the framework of ecosystem services (Millennium Ecosystem Assessment, 2005; Díaz et al., 2018). Ecosystem services are becoming prominent in environmental management because they directly link human activities and changes in the landscape to benefits or impacts on people (Keeler et al., 2012; Apitz, 2013; Munns et al., 2015). These potential benefits include a range of basic regulatory functions of the Earth system (e.g., carbon sequestration, soil formation, water regulation), provision of material goods (e.g., food, building materials, and medicines) and an array of non-material benefits (e.g., inspiration for science and art, recreational opportunities, a sense of place and identity) (Díaz et al., 2018). Because ecosystem services provide a framework for assessment, and even for monetary valuation of nature's benefits, they provide a mechanism to integrate environmental considerations into management and policy decisions (TEEB, 2010; Apitz, 2013; Brauman, 2015a,b; Guerry et al., 2015). As a result, ecosystem services have been embraced by a variety of organizations such as state and federal governments, NGOs, and corporations (Apitz, 2013). Global initiatives codifying and expanding ecosystem services assessment include the Millennium Ecosystem Assessment (Millennium Ecosystem Assessment, 2005; TEEB, 2010), the Common International Classification of Ecosystem Services (Haines-Young and Potschin, 2018), and the Intergovernmental Science-Policy Platform on Biodiversity and Ecosystem Services (IPBES) (Díaz et al., 2018; Bongaarts, 2019).
While ecosystem services provided by groundwater have been well established, such as production and storage of water supply (Griebler and Avramov, 2015), ecosystem services provided by terrestrial and aquatic GDEs has been less well-explored. In addition, individual studies that have assessed benefits of GDEs are known to be locally important [Murray et al., 2006, 2012; Knüppe and Pahl-Wostl, 2011; CGIAR Research Program on Water, Land and Ecosystems (WLE), 2015]. For example, studies have evaluated impacts of groundwater on trout habitat (Power et al., 1999) and on the carbon balance of wetlands (Kayranli et al., 2010). However, these studies have generally not been systematically organized under an ecosystem services rubric nor considered the provision of a suite of benefits. Identifying and classifying the benefits provided by GDEs into an ecosystems services approach provides a way for GDEs to be assessed when evaluating tradeoffs in time and space among groundwater users (Konikow and Kendy, 2005; Zektser et al., 2005; Scanlon et al., 2012), including the effects of groundwater pumping on adjacent wells (Pfeiffer and Lin, 2012) and in nearby rivers (McPhee and Yeh, 2004).
The emergence of environmental considerations in groundwater management (Rohde et al., 2017) has stimulated regional GDE mapping efforts (Doody et al., 2017; Klausmeyer et al., 2018) and frameworks to assess groundwater requirements for GDEs (Eamus et al., 2006; Richardson et al., 2011; Rohde et al., 2018). Most approaches to GDEs focus on their uniqueness, biodiversity, and ecology. While many studies note that GDEs provide ecosystem services, there is little concrete guidance for identifying the specific ecosystem services GDEs provide or how best to quantify them (Murray et al., 2006). Moreover, ecosystem services of GDEs are conflated with ecosystem services of groundwater itself (Figure 1). Building upon the growing body of knowledge about the location and functioning of GDEs and recognizing the growing interest in using ecosystem services and sustainable groundwater management, here we: (1) present an overview of GDEs and the ecosystem services they provide, (2) develop an approach to assess specific benefits provided by GDEs to people, and (3) apply this approach in a case study to identify and quantify three ecosystem services—pollination, carbon sequestration, and water quality regulation—provided by GDEs in California, USA.
Figure 1. Ecosystem service benefits of GDES are distinct from benefits from groundwater and surface water. (A) Groundwater is interconnected with (B) surface water. GDEs (C), which are supported by groundwater, provide distinct benefits to people because of the unique species they support. Here, we focus on the benefits provided by GDEs (C).
GDEs include both terrestrial and aquatic ecosystems such as floodplain and riparian vegetation zones, wetlands, seeps, springs, lakes and rivers (Griebler and Avramov, 2015). These ecosystems are partially or fully reliant on the groundwater levels or chemical characteristics of groundwater (Eamus and Froend, 2006). Groundwater provides a reliable water source to aquatic GDEs either via consistent discharge or seasonal fluctuations (Bergkamp and Cross, 2006), and to terrestrial GDEs via groundwater levels. The degree to which ecosystems rely on groundwater varies according to season, geologic factors, flora, fauna, weather, and climate (Eamus and Froend, 2006; Eamus et al., 2006; Kløve et al., 2011; Wachniew et al., 2014). As illustrated in Figure 2, groundwater conditions within different GDE types can support unique ecosystem functions and life cycle processes, which in turn allows them to deliver a range of ecosystem services.
Figure 2. Ecosystem functions of GDEs that are uniquely supported by groundwater and produce ecosystem services. Different types of GDEs (left hand column), both aquatic (blue) and terrestrial (green), are affected by groundwater (second column). The unique structure of GDEs allows them to function (third column) in ways that produce a range of outputs (fourth column) that are value for a variety of reasons, called ecosystem services (fifth column).
In terrestrial ecosystems, groundwater has a strong impact on the presence and composition of vegetation (Orellana et al., 2012). Because groundwater often buffers terrestrial vegetation during dry summers or drought, groundwater can also enable vegetation to grow more vigorously and over a longer period than would otherwise be possible (Naumburg et al., 2005). These characteristics of GDEs can lead to particular ecosystem services because the composition of species reliant on groundwater alters the flow of carbon, nutrients, energy and material available through the ecosystem.
In aquatic ecosystems, one major role of groundwater is its contribution to surface water flows (Power et al., 1999; Pettit and Froend, 2018), increasing hydrologic connectivity in freshwater systems (Danielopol et al., 2003; Boulton, 2005; Sechu et al., 2023). This supports migratory fish such as steelhead and salmon, which rely on continuously connected surface water from the ocean to their spawning grounds (Fleckenstein et al., 2004; Zektser et al., 2005; Douglas, 2006). Groundwater also regulates water temperature in rivers and streams (Power et al., 1999; Fleckenstein et al., 2004), protecting aquatic species by providing areas of refuge during times of extreme heat or cold (Power et al., 1999). This is crucial for fish eggs, which are sensitive to temperature fluctuations (Hester and Gooseff, 2010; Warren et al., 2012). The hyporheic zone, where surface and ground water exchange occurs (Power et al., 1999; Fleckenstein et al., 2004; Zektser et al., 2005; Saltveit and Brabrand, 2013), is particularly important for fish that take advantage of nutrient content and regulated temperatures to spawn (Baxter and Hauer, 2000; Hester and Gooseff, 2010; Lawrence et al., 2013).
Linking groundwater inputs to GDE function is critical to understanding impacts of groundwater extraction on ecosystem services (Murray et al., 2006). In the Upper San Pedro River basin in Arizona, for example, pumping to meet demand from a growing population has reduced flow in the river thereby diminishing the recreational opportunities provided by the river including diverse plant and animal life along its banks (McPhee and Yeh, 2004). Similarly, groundwater dependent rivers can run dry and become disconnected without groundwater inputs, impacting migratory fish passage (Fleckenstein et al., 2004), and threatening aquatic ecosystems (de Graaf et al., 2019). However, GDEs may not be unique in their ability to provide desired ecosystem services. Murray et al. (2006) notes that only small changes in carbon sequestration capacity may result from loss of terrestrial GDEs, as those ecosystems may be replaced by other non-groundwater dependent species.
To facilitate the identification of ecosystem services provided by GDEs, we provide background information below on a selected list of ecosystem services that are both commonly provided by GDEs and for which there are likely to be data. The ecosystem services described below are not unique to GDEs and can likely be provided by other ecosystem types. However, what is unique here, is the identification and organization of ecosystem services provided by GDEs in an attempt to articulate a fuller range of benefits provided by groundwater.
Below, for each ecosystem service provided by GDEs, we first discuss the mechanisms underlying the production of each service, from which we can determine if a particular GDE produces a given service (called production below). This is critical because different types of GDEs and different biophysical conditions provide different levels of service provision [e.g., wetlands may be either net carbon sources or sinks depending on biophysical context (Kayranli et al., 2010)]. Second, for each type of benefit we identify characteristics of potential beneficiaries (called beneficiaries below). Again, local context is critical because cultural preferences determine which ecosystem services are desired.
For example, food provision from rivers in California differs from that provided in India not only because of biophysical differences between ecosystems but because of differences in taste, history, and institutions of those who cultivate, harvest, and eat the food. Third, we identify any spatial distribution necessary to achieve delivery of an ecosystem service (referred to as spatial distribution). For example, pollination services are delivered only if a beneficiary is within a critical distance. Thus, only pollination-dependent crops planted within foraging distance of an ecosystem that provides pollinator nesting sites or food resources receives those services (Koh et al., 2016). Climate regulation, in contrast, occurs locally as carbon is sequestered in plant biomass and soils but provides a benefit globally because the atmosphere is well-mixed (Lal, 2008). Other ecosystem services require both proximity of a beneficiary and driver. Water quality regulation, for example, is necessary only if pollutants are added to the water, perhaps originating from an upstream city or farm (Brauman et al., 2007). While local context is necessary in determining the production and identifying the beneficiaries, the overall approach is broadly transferrable.
Pollination is the movement of pollen, that fertilizes plants and leads to the production of fruits and vegetables (Díaz et al., 2018). Globally, 75% of major crops rely on insect pollinators (Klein et al., 2007).
Viable pollinator habitat is largely determined by the availability of food resources—a range of floral vegetation blooming throughout the year—and nesting sites—provided by dry ground, as many wild pollinators are ground-nesters (Potts et al., 2010; Chaplin-Kramer et al., 2011; Jha and Kremen, 2013). This habitat is provided by most terrestrial GDEs and seasonally-dry GDE wetlands (Chaplin-Kramer et al., 2011).
Only pollinator-dependent crops benefit from pollination. Natural habitat enhances pollination even when domestic bees are used for pollination because it increases reproduction of domestic bees as well as provides additional pollination by wild bees (Williams and Kremen, 2007). Most farmland growing pollinator-dependent crops thus potentially benefits from pollination services.
To receive pollination services, pollinator-dependent crops must be within traveling distance of wild pollinator habitat. Though foraging distances vary considerably among species, the majority of bees travel under 1 km (Greenleaf et al., 2007). Thus, any farmland growing pollinator-dependent crops within 1 km of GDE habitat will likely gain some benefit.
Terrestrial GDEs located between pollution sources and water bodies can entrain nutrients, sediment, and some other chemicals (Brauman et al., 2007).
GDEs regulate water quality by slowing and filtering the surface water flow. As water infiltrates through the soil and across leaf litter, sediment particles are trapped (Barling and Moore, 1994; Lowrance, 1998). Vegetation reduces nutrient runoff through direct uptake and by increasing soil permeability (Dosskey et al., 2010). Floodplains and the interface between water and soil at a streambank, which are often groundwater dependent, can be particularly effective at removing pollutants (Hoffmann et al., 2009; Lawrence et al., 2013). Factors influencing the ability of vegetation to regulate water quality include slope, groundwater dependent vegetation type, and pollutant type and size, but the size and location of the area available to take up pollutants is the primary determinant (Mayer et al., 2007; Gumiere et al., 2011). In-stream vegetation supported by groundwater may also regulate water quality through channel stabilization and by sequestering pollutants (Montakhab et al., 2012).
Regulation of water quality is beneficial only when people are affected by a change in water quality (Keeler et al., 2012). Impacts could occur through direct consumption of drinking water or indirectly, through recreational or cultural activities. The appropriate definition of beneficiaries will be context specific but may be as broad as people within a watershed, state, or country.
To regulate water quality, a GDE must be situated between a pollutant source and a site where water quality is of interest to beneficiaries. The sources most likely to provide pollutants are agricultural or urban areas, and any surface water body may benefit from improved water quality. GDEs downstream of pollutant sources and upstream of water bodies potentially provide water quality regulation services.
Climate regulation by ecosystems includes carbon sequestration and methane and nitrous oxide emissions affecting global greenhouse gas concentrations as well as local climate interactions such as shading, reflection, and rainfall recycling (Díaz et al., 2018).
Terrestrial GDEs sequester carbon in plant material and in the soil (Anderson-Teixeira and DeLUCIA, 2011). Because groundwater supported vegetation grows for longer periods and more abundantly than would be possible without the addition of groundwater, GDEs potentially sequester more carbon than other types of vegetation (Naumburg et al., 2005; Eamus and Froend, 2006). Wetlands can be either sources or sinks of greenhouse gases depending on their individual characteristics. Wetland soil and plants sequester carbon, but wetlands also release carbon dioxide, methane, and nitrous oxide (Kayranli et al., 2010). Dewatering wetlands would likely cause substantial emissions of greenhouse gasses. For local climate regulation, riparian GDEs help stabilize the temperatures of adjacent streams by providing shade (Barling and Moore, 1994), and like all vegetation, the albedo of GDEs is higher than that of bare ground or developed areas, helping to lower local temperature (Anderson-Teixeira and DeLUCIA, 2011). All terrestrial GDEs likely provide climate regulation.
The impacts of climate regulation are diffuse (Wilbanks and Kates, 1999), so potential beneficiaries encompasses the human population more generally.
The atmosphere is assumed to be well-mixed, so proximity between GDEs sequestering carbon and potential beneficiaries is unnecessary.
Ecosystems can reduce the occurrence as well as the impact of natural hazards including coastal storm surges, riverine floods, landslides, and fires (Díaz et al., 2018).
Both terrestrial and aquatic GDEs can potentially reduce the size and duration of riverine flood peaks by slowing the movement of water over the land (Thomas and Nisbet, 2007) and in the river channel (Bechtol and Laurian, 2005; Elosegi and Sabater, 2013; Palmer et al., 2014). Headwater wetlands, however, may either exacerbate or reduce flooding (Leyer et al., 2012; Acreman and Holden, 2013). The extent to which GDEs can affect the size of a flood peak and under what rainfall conditions they can do so is unclear. Natural ecosystems are most effective at reducing small- to medium-sized floods, but have limited benefit during large flood events (Kochenderfer et al., 2007; Alila et al., 2009; Brookhuis and Hein, 2016). Location, climate, season, slope, and vegetation type all influence the flood mitigation service of GDEs (Andréassian, 2004).
People living within the floodplain will be affected by floods and can benefit from reduced flood peaks.
To benefit from flood regulation, people must live downstream of a GDE. A terrestrial or aquatic GDE potentially provides hazard regulation services to those living in the downstream floodplain.
Flora and fauna supported by GDEs can provide bioenergy, food, materials, and medicines (Díaz et al., 2018).
Wetlands and terrestrial GDEs provide a range of material resources including leafy vegetables, fish, invertebrates, and animals that rely on these food resources (Silvius et al., 2000; Turyahabwe et al., 2013). Species unique to GDEs, such as medicinal plants found in Chinese wetlands (Fang et al., 2006), are likely to be locally important. Groundwater dependent wetlands, for example, support the growth of papyrus, a unique source of fiber used in paper making (Wiedeman and Bayer, 2012). In addition, many terrestrial GDEs, including floodplains, wetlands, and riparian areas, are important agricultural sites due to their fertile soils and abundant water (Verhoeven and Setter, 2010). Aquatic GDEs also support vegetation and animals that have important material uses (Boulton, 2005). GDEs may also have important ties to identity because they have historically provided food, shelter, and materials and carry spiritual, cultural, and recreational significance. Specific animals, such as migratory salmon, are both economically and culturally valuable to many native tribes. Thus, any GDE can provide material benefits, though both the specific GDEs and the types of materials produced will be location specific.
Any person with access to a GDE, either directly or through markets, could benefit depending on cultural preferences. Those who do not have the material means to substitute food, energy, or other material goods provided by GDEs are most reliant on them and are most vulnerable to their disappearance.
Access to a GDE, often defined by proximity, is required for first-order use of material resources provided by GDEs. However, valuable resources provided by a GDE may be traded great distances.
Non-material benefits of nature include learning, experiences, and identity provided by landscapes and the flora and fauna that make them up (Díaz et al., 2018).
In GDEs, water flow, an extended wet season, and increased water volume provide aesthetic features, particularly color, sound, and movement that may improve mental health and enhance recreational activities (Burmil et al., 1999). Recreation is strongly linked with GDEs—the extended wet season and increased water flow contributes to boating (Ward et al., 1996), fishing for specific species dependent upon groundwater contributions to streams for migration or spawning/juvenile rearing (Boulton, 2005), and wetlands and riparian GDEs that often support fowl and other animals that are hunted recreationally (Seavy et al., 2009). These and other recreational experiences can be enhanced or enabled by the aesthetic of flowing water in the dry season. Not all experiential benefits are active; simply being in nature, with or without physical exercise, has been shown to improve cognitive function and mental health (Bratman et al., 2012).
GDEs may have important ties to identity because they have historically provided food, shelter, and materials, and carry spiritual, cultural and recreational significance in conjunction with material good. For example, specific animals, such a migratory salmon, are both economically and culturally valuable to many of the indigenous people of California. Beneficiaries can be grouped into categories with similar characteristics: (i) those who partake in activities in GDEs, including boaters, swimmers, hikers, or even painters; (ii) those who value the historical and cultural aspects of GDEs; and (iii) those with traditional or indigenous connections to GDEs.
In many cases, a GDE must be accessible to provide benefits. In this case, proximity is key and is determined by a user's willingness to travel.
To demonstrate how this approach can be operationalized, we mapped and then evaluated the provision of three ecosystem services in California: pollination, water quality regulation, and climate regulation/carbon sequestration. As the largest agricultural state in the nation with farmland totaling 10 million hectares and agricultural receipts totaling $44 billion, we developed methods to quantify the pollination services provided by GDEs. Additionally, California is committed to reducing greenhouse gas emissions to 80% below 1990 levels by 2050 (Cameron et al., 2017), and while carbon sequestering ecosystems will help meet those goals, this is the first attempt to quantify the contribution of GDEs in potentially reducing emissions.
In 2014, California passed the Sustainable Groundwater Management Act (SGMA) which requires groundwater sustainability agencies (GSAs) to manage groundwater resources for current and future social, economic and environmental benefits (State of California, 2014). Under SGMA, GSAs are required to identify and consider impacts to GDEs in groundwater sustainability plans (GSPs). To assist GSAs in the identification of GDEs in GSPs, The Nature Conservancy (Klausmeyer et al., 2018) mapped the occurrence and distribution of both terretrial and aquatic GDEs statewide. These data were reviewed and eventually adopted as the California Department of Water Resource's Natural Communities Commonly Associated with Groundwater (NCCAG) dataset, which includes terrestrial and aquatic features that need be locally verified (California Department of Water Resources, 2018; Klausmeyer et al., 2018).
Using the NC dataset, we quantified the benefits of the three ecosystem services within SGMA basins: pollination, water quality regulation and climate regulation/carbon sequestration. For each service, we document the type of data we selected as well as the specific data source (Table 1).
We followed the steps in the assessment approach described above to identify: (1) GDEs producing the ecosystem service (Production), (2) potential beneficiaries (Beneficiaries), and (3) the service delivered by determining the appropriate overlap of production and beneficiaries (Spatial distribution). We then aggregated (Aggregation) and normalized our findings to provide informative and interpretable results.
We identified terrestrial GDEs and seasonally-dry GDE wetlands that can provide nesting sites and food resources for wild pollinators defined as all vegetative GDEs in the NCCAG database (Chaplin-Kramer et al., 2011; California Department of Water Resources, 2018).
To identify pollinator-dependent cropland, we identified pollinator-dependent crops grown in California using detailed crop maps and an inventory of crop-dependence on pollinators (Chaplin-Kramer et al., 2011; California Department of Water Resources, 2018). In California, pollinator-dependent crops include alfalfa, almonds, apples, berries, and peaches, while non-pollinator dependent crops are primarily corn, citrus, grapes, potatoes, and rice. We created a binary indicator of pollinator dependence, by marking all cropland designated with ‘Modest' to ‘Essential' dependence on pollinators in Chaplin-Kramer et al. (2011) as pollinator dependent.
We identified pollinator-dependent cropland benefiting from GDEs by locating pollinator-dependent cropland within foraging distance of GDE habitat, 1 km (Figure 3).
Figure 3. Spatial representation of how the crop pollination service (left) and water quality regulation service (right) are quantified. Crop pollination was calculated by dividing the benefitting cropland (orange) by the total pollinator dependent cropland in the basin (orange + gray; left panel). Water quality regulation was calculated by dividing the area GDEs capable of water purification (dark green) by the total area of land within 0.5 km of a water body and 1 km of developed land (dark green + yellow; right panel).
Total acreage of pollinator dependent cropland was summed in each groundwater basin that has the potential to receive the GDE crop pollination service (Figure 3). To normalize our results, we divided the total acres of benefitting cropland by the total acres of pollinator-dependent cropland in the basin.
All GDEs in the NCCAG database (California Department of Water Resources, 2018) that contained plants and soils capable of filtering water were considered to potentially benefit water quality. These included all ecosystems that were not limnetic or riverine.
We designated all surface waters in California within 1,500 m from a pollution source (e.g., developed areas, including urban and farmland) as potential beneficiaries of water quality water quality regulation.
Any GDE within a 500 m buffer of a potentially benefiting surface water feature was considered close enough to impact surface water quality (Figure 3). The overlapping area between the developed buffer and the surface water buffer represents the region where water purification should occur.
We report the area and percentage of land area between potential pollution sources and water bodies occupied by GDEs.
We calculated total carbon storage across the state of California using above and below ground carbon storage data (Gonzalez et al., 2015; California Department of Water Resources, 2018; Ramcharan et al., 2018). Soil carbon stocks were calculated using depth ranges in soil grids (Hengl et al., 2017). We then extracted carbon storage data from all groundwater basins and the GDEs within those basins.
We designated all regions of California as equally benefitting from carbon storage, because local greenhouse gas regulation has a global impact (Wilbanks and Kates, 1999).
We considered all of the GDE carbon storage across California without further spatial filtering.
To aggregate and normalize our results, we report total tons of carbon dioxide equivalent storage in GDE areas as a percentage of total tons of carbon dioxide equivalent storage in each basin. Because each pixel is one hectare in size, the mean CO2 tons/ha can be calculated by finding the mean pixel value in GDE and non-GDE locations in each basin. In order to compare the climate regulation efficiency of GDEs vs. non-GDEs, the mean tons/ha for non-GDEs is divided by the mean tons/ha for GDEs.
Although GDEs occupy a small portion of California (~9,000 km2 or 5.3% of California's groundwater basins), 33% of pollinator dependent crops are within 1 km of a GDE and therefore may benefit from GDE pollinators (Figure 4A). In the Central and Imperial Valleys, ~280,000 hectares of crops (out of ~900,000 hectares of all pollinator-dependent crops) could be benefiting from pollination services that GDEs provide. The concentration of these benefits varies spatially, with higher benefits in the Sacramento Valley (46%), Salinas Valley (54%) and Imperial Valley (82%). Crops receiving these benefits include almonds and alfalfa in the Sacramento Valley and strawberries in the Salinas Valley (Table 2). Although GDEs are not solely responsible for the production of adjacent pollinator-dependent crops, the presence of pollinators in GDEs has the potential to increase crop pollination, thereby contributing to higher yields (Chaplin-Kramer et al., 2011).
Figure 4. (A) Crop pollination service by SGMA groundwater basin. Percent of pollinator-dependent cropland within 1 km foraging distance of a vegetative GDE by groundwater basin. (B) Water quality regulation service by SGMA groundwater basin. Percent of groundwater dependent vegetation within the water filtration area (land within 500 m of surface water and 1,000 m of developed land) by groundwater basin. (C) Climate regulation service by SGMA groundwater basin. Total carbon dioxide equivalent storage in GDEs and (D) Percent of total carbon dioxide equivalent storage provided by GDEs summarized by groundwater basin.
In the State's larger groundwater basins, such as those located in the Central Valley, more than 1,500 hectares of GDEs are located between developed areas and bodies of water; therefore having the potential to regulate or mitigate water quality (Figure 4B). GDEs comprise a relatively large percentage of land in northern and central California. In contrast, there does not appear to be water regulation services in southeastern California, likely due to the lack of surface water in those desert areas. As Figure 4B shows, there are some areas with a large amount of surface water that do not receive the water quality regulation service of GDEs either, such as the northwestern sub-basin of the Sacramento Valley.
Areas with large amounts of woody vegetation, such as groundwater dependent redwood forests in Northern California, offer the greatest total amount of carbon storage (Figure 4C). However, GDEs as a percent of total carbon storage for SGMA basin (Figure 4D) shows that GDEs potentially provide a high percentage of carbon storage in less vegetated regions (such as in the desert regions). The total carbon storage in GDE areas statewide is substantial: combined, areas occupied by GDEs store the equivalent of 790 million tons of carbon dioxide, which is nearly twice as much as California emits annually (Widger, 2019).
Groundwater dependent ecosystems in California provide a range of ecosystem services. By systematically linking groundwater to ecosystem function and ecosystem services, we can begin to identify and quantify which ecosystem service beneficiaries are threatened by groundwater degradation. Our analysis of three of these services provides a blueprint for identifying and quantifying GDE ecosystem services in California and in other states. When the benefits of these ecosystem services are defined with more detail, this could be used to evaluate specific policy questions about where and how to invest in GDE and groundwater conservation. On a local level, the approach we outline here may provide land and water managers a way to consider potential ecosystem services provided by GDEs.
While our results show the sum of pollinated cropland acres, tons of carbon storage and area of water filtration are impressive, the degree to which existing GDEs provide ecosystem services compared to non GDEs needs exploration. Our analysis here did not assess what additional services GDEs provide over other non-groundwater dependent ecosystems; instead our goal was to quantify services provided by GDEs. Overall, our results likely represent an upper bound for the quantification of GDE ecosystem services in SGMA basins. The total acres benefitting cropland, for example, represents all cropland that could potentially be aided by GDE pollination. This does not imply that those areas are fully dependent on GDE pollinators for a successful harvest or that elimination of a nearby GDE would negatively impact crop production. Instead, it demonstrates cropland close to GDEs potentially receive pollination services from GDE pollinators. While our results may represent the upper end of potential benefits of GDEs in SGMA basins, the potential services provided by GDEs may be much larger if applied to all GDEs in the state (those within and outside of SGMA basins).
Ecosystems services are recognized as an important solution for sustainable development and are particularly useful when addressing policy and resource management questions that require a comparison of costs, benefits, and tradeoffs. However, a disconnect often exists in preserving these ecosystem services, as the close interdependencies between water and ecosystem are rarely recognized or valued (Bergkamp and Cross, 2006). Ecosystem services provided by wetlands, forests, springs, rivers, and coastal environments rely on freshwater resources, including groundwater, to maintain the ecological integrity of their ecosystem structure and functioning. As a result, these ecosystems and the services they provide are being affected by water and land resource management and policy decisions that drive groundwater pumping, water infrastructure development (e.g., dams, reservoirs, canals), and land use conversion of natural landscapes to urban and agricultural uses (Reid, 2005).
In recent years, conservation has been recognized as providing co-benefits for people and nature. In 2018, the United Nations declared the Nature-based Solutions, which preserves or mimics the features and complex processes of nature to offer multiple nature-people benefits, as a means to overcome 21st century water challenges (WWAP, 2018). In contrast to centralized traditional human-built (“gray”) infrastructure (e.g., dams, canals), nature-based solutions (“green”) and infrastructure (e.g., managed aquifer recharge) are considered to be a more cost-effective and sustainable alternative (Perrone and Rohde, 2016; Villholth and Ross, 2018; Rohde et al., 2020). As a result, groundwater aquifers have also become viewed as important natural capital that provides not only water supply but also other natural ecosystem services (Bergkamp and Cross, 2006). Identifying the ecosystem services provided by GDEs provides a more complete picture of the natural capital provided by groundwater aquifers.
The data are available archived at UMN. Link: https://conservancy.umn.edu/handle/11299/213889.
All authors listed have made a substantial, direct, and intellectual contribution to the work and approved it for publication.
The authors would like to thank the S.D. Bechtel, Jr. Foundation for their philanthropic financial support to the Nature Conservancy's ongoing groundwater research on GDEs in sustainable groundwater management, The Nature Conservancy's science catalyst fund for funding this work, and David Martin for below ground carbon storage data.
The authors declare that the research was conducted in the absence of any commercial or financial relationships that could be construed as a potential conflict of interest.
All claims expressed in this article are solely those of the authors and do not necessarily represent those of their affiliated organizations, or those of the publisher, the editors and the reviewers. Any product that may be evaluated in this article, or claim that may be made by its manufacturer, is not guaranteed or endorsed by the publisher.
Acreman, M., and Holden, J. (2013). How wetlands affect floods. Wetlands 33, 773–786. doi: 10.1007/s13157-013-0473-2
Alila, Y., Kuraś, P. K., Schnorbus, M., and Hudson, R. (2009). Forests and floods: a new paradigm sheds light on age-old controversies. Water Resour. Res. 45. doi: 10.1029/2008WR007207
Anderson-Teixeira, K. J., and DeLUCIA, E. H. (2011). The greenhouse gas value of ecosystems. Glob. Change Biol. 17, 425–438. doi: 10.1111/j.1365-2486.2010.02220.x
Andréassian, V. (2004). Waters and forests: from historical controversy to scientific debate. J. Hydrol. 291, 1–27. doi: 10.1016/j.jhydrol.2003.12.015
Apitz, S. E. (2013). Ecosystem services and environmental decision making: seeking order in complexity. Integr. Environ. Assess. Manag. 9, 214–230. doi: 10.1002/ieam.1389
Barling, R. D., and Moore, I. D. (1994). Role of buffer strips in management of waterway pollution: a review. Environ. Manage. 18, 543–558. doi: 10.1007/BF02400858
Baxter, C., and Hauer, F. (2000). Geomorphology, hyporheic exchange, and selection of spawning habitat by bull trout (Salvelinus confluentus). Can. J. Fish. Aquat. Sci. - CAN J. Fish. Aquat. Sci. 57, 1470–1481. doi: 10.1139/f00-056
Bechtol, V., and Laurian, L. (2005). Restoring straightened rivers for sustainable flood mitigation. Disaster Prev. Manag. Int. J. 14, 6–19. doi: 10.1108/09653560510583806
Bergkamp, G., and Cross, K. (2006). “Groundwater and ecosystem services: Towards their sustainable use,” in International Symposium on Groundwater Sustainability (ISGWAS) (Gland: International Union for Conservation of Nature), 177–193.
Bongaarts, J. (2019). IPBES, 2019. Summary for policymakers of the global assessment report on biodiversity and ecosystem services of the Intergovernmental Science-Policy Platform on Biodiversity and Ecosystem Services. Popul. Dev. Rev. 45, 680–681. doi: 10.1111/padr.12283
Boulton, A. (2005). Editorial: Chances and challenges in the conservation of groundwaters and their dependent ecosystems. Aquat. Conserv. 15, 319–323. doi: 10.1002/aqc.712
Bratman, G. N., Hamilton, J. P., and Daily, G. C. (2012). The impacts of nature experience on human cognitive function and mental health: nature experience, cognitive function, and mental health. Ann. N. Y. Acad. Sci. 1249, 118–136. doi: 10.1111/j.1749-6632.2011.06400.x
Brauman, K. A. (2015a). Get on the ecosystem services bandwagon. Integr. Environ. Assess. Manag. 11, 343–344. doi: 10.1002/ieam.1654
Brauman, K. A. (2015b). Hydrologic ecosystem services: linking ecohydrologic processes to human well-being in water research and watershed management. Wiley Interdiscip. Rev. Water 2, 345–358. doi: 10.1002/wat2.1081
Brauman, K. A., Daily, G. C., Duarte, T. K., and Mooney, H. A. (2007). The nature and value of ecosystem services: an overview highlighting hydrologic services. Annu. Rev. Environ. Resour. 32, 67–98. doi: 10.1146/annurev.energy.32.031306.102758
Brookhuis, B. J., and Hein, L. G. (2016). The value of the flood control service of tropical forests: a case study for Trinidad. For. Policy Econ. 62, 118–124. doi: 10.1016/j.forpol.2015.10.002
Burmil, S., Daniel, T. C., and Hetherington, J. D. (1999). Human values and perceptions of water in arid landscapes. Landsc. Urban Plan. 44, 99–109. doi: 10.1016/S0169-2046(99)00007-9
California Department of Water Resources (2018). Natural Communities Commonly Associated with Groundwater (NCCAG) Dataset. Available online at: https://gis.water.ca.gov/app/NCDatasetViewer/ (accessed June, 4, 2020).
Cameron, D. R., Marvin, D. C., Remucal, J. M., and Passero, M. C. (2017). Ecosystem management and land conservation can substantially contribute to California's climate mitigation goals. Proc. Natl. Acad. Sci. 114, 12833–12838. doi: 10.1073/pnas.1707811114
CGIAR Research Program on Water Land and Ecosystems (WLE). (2015). Groundwater and Ecosystem Services: A Framework for Managing Smallholder Groundwater-Dependent Agrarian socio-ecologies - Applying an Ecosystem Services and Resilience Approach. Colombo, Sri Lanka: International Water Management Institute (IWMI). doi: 10.5337/2015.208
Chaplin-Kramer, R., Tuxen-Bettman, K., and Kremen, C. (2011). Value of wildland habitat for supplying pollination services to Californian agriculture. Rangelands 33, 33–41. doi: 10.2111/1551-501X-33.3.33
Danielopol, D. L., Griebler, C., Gunatilaka, A., and Notenboom, J. (2003). Present state and future prospects for groundwater ecosystems. Environ. Conserv. 30, 104–130. doi: 10.1017/S0376892903000109
de Graaf, I. E. M., Gleeson, T., Rens van Beek, L. P. H., Sutanudjaja, E. H, and Bierkens,, M. F. P (2019). Environmental flow limits to global groundwater pumping. Nature 574, 90–94. doi: 10.1038/s41586-019-1594-4
Díaz, S., Pascual, U., Stenseke, M., Martín-López, B., Watson, R. T., Molnár, Z., et al. (2018). Assessing nature's contributions to people. Science 359, 270–272. doi: 10.1126/science.aap8826
Doody, T. M., Barron, O. V., Dowsley, K., Emelyanova, I., Fawcett, J., Overton, I. C., et al. (2017). Continental mapping of groundwater dependent ecosystems: a methodological framework to integrate diverse data and expert opinion. J. Hydrol. Reg. Stud. 10, 61–81. doi: 10.1016/j.ejrh.2017.01.003
Dosskey, M. G., Vidon, P., Gurwick, N. P., Allan, C. J., Duval, T. P., and Lowrance, R. (2010). The role of riparian vegetation in protecting and improving chemical water quality in streams1. JAWRA J. Am. Water Resour. Assoc. 46, 261–277. doi: 10.1111/j.1752-1688.2010.00419.x
Douglas, T. (2006). Review of Groundwater-Salmon Interactions in British Columbia. Available online at: https://policycommons.net/artifacts/1186350/review-of-groundwater-salmon-interactions-in-british-columbia/1739478/ (accessed October 19, 2022).
Eamus, D., and Froend, R. (2006). Groundwater-dependent ecosystems: the where, what and why of GDEs. Aust. J. Bot. 54, 91. doi: 10.1071/BT06029
Eamus, D., Froend, R., Loomes, R., Hose, G., and Murray, B. (2006). A functional methodology for determining the groundwater regime needed to maintain the health of groundwater-dependent vegetation. Aust. J. Bot. 54, 97–114. doi: 10.1071/BT05031
Elosegi, A., and Sabater, S. (2013). Effects of hydromorphological impacts on river ecosystem functioning: a review and suggestions for assessing ecological impacts. Hydrobiologia 712, 129–143. doi: 10.1007/s10750-012-1226-6
Fang, L., Liu, H., Cui, J., and Xu, Z. (2006). Traditional use of wetland plants in Dai villages in Xishuangbanna, Yun-nan. Biodivers. Sci. 14, 300. doi: 10.1360/biodiv.060012
Fleckenstein, J., Anderson, M., Fogg, G., and Mount, J. (2004). Managing surface water-groundwater to restore fall flows in the cosumnes river. J. Water Resour. Plan. Manag. 130, 301–310. doi: 10.1061/(ASCE)0733-9496(2004)130:4(301)
Gonzalez, P., Battles, J. J., Collins, B. M., Robards, T., and Saah, D. S. (2015). Aboveground live carbon stock changes of California wildland ecosystems, 2001–2010. For. Ecol. Manag. 348, 68–77. doi: 10.1016/j.foreco.2015.03.040
Greenleaf, S. S., Williams, N. M., Winfree, R., and Kremen, C. (2007). Bee foraging ranges and their relationship to body size. Oecologia 153, 589–596. doi: 10.1007/s00442-007-0752-9
Griebler, C., and Avramov, M. (2015). Groundwater ecosystem services: a review. Freshw. Sci. 34, 355–367. doi: 10.1086/679903
Guerry, A. D., Polasky, S., Lubchenco, J., Chaplin-Kramer, R., Daily, G. C., Griffin, R., et al. (2015). Natural capital and ecosystem services informing decisions: from promise to practice. Proc. Natl. Acad. Sci. 112, 7348–7355. doi: 10.1073/pnas.1503751112
Gumiere, S. J., Le Bissonnais, Y., Raclot, D., and Cheviron, B. (2011). Vegetated filter effects on sedimentological connectivity of agricultural catchments in erosion modelling: a review. Earth Surf. Process. Landf. 36, 3–19. doi: 10.1002/esp.2042
Haines-Young, R., and Potschin, M. (2018). Common Classification of Eocystem Services (CICES) V5.1. Guidance on the Application of the Revised Structure. Nottingham: Fabis Consulting Ltd, 53.
Hapke, C. J., and Reid, D. (2007). National Assessment of Shoreline Change, Part 4: Historical Coastal Cliff Retreat along the California Coast: U.S. Geological Survey Open-file Report 2007-1133. U.S. Geological Survey. Available online at: https://pubs.usgs.gov/of/2007/1133/
Hengl, T., Mendes de Jesus, J., Heuvelink, G. B., Ruiperez Gonzalez, M., Kilibarda, M., Blagotić, A., et al. (2017). SoilGrids250m: Global gridded soil information based on machine learning. PLoS ONE. 12, e0169748. doi: 10.1371/journal.pone.0169748
Hester, E. T., and Gooseff, M. N. (2010). Moving beyond the banks: hyporheic restoration is fundamental to restoring ecological services and functions of streams. Environ. Sci. Technol. 44, 1521–1525. doi: 10.1021/es902988n
Hoffmann, C. C., Kjaergaard, C., Uusi-Kämppä, J., Hansen, H. C. B., and Kronvang, B. (2009). Phosphorus retention in riparian buffers: review of their efficiency. J. Environ. Qual. 38, 1942–1955. doi: 10.2134/jeq2008.0087
Jha, S., and Kremen, C. (2013). Resource diversity and landscape-level homogeneity drive native bee foraging. Proc. Natl. Acad. Sci. 110, 555–558. doi: 10.1073/pnas.1208682110
Kayranli, B., Scholz, M., Mustafa, A., and Hedmark, Å. (2010). Carbon storage and fluxes within freshwater wetlands: a critical review. Wetlands 30, 111–124. doi: 10.1007/s13157-009-0003-4
Keeler, B. L., Polasky, S., Brauman, K. A., Johnson, K. A., Finlay, J. C., O'Neill, A., et al. (2012). Linking water quality and well-being for improved assessment and valuation of ecosystem services. Proc. Natl. Acad. Sci. 109, 18619–18624. doi: 10.1073/pnas.1215991109
Klausmeyer, K., Howard, J., Keeler-Wolf, T., Davis-Fadtke, K., Hull, R., and Lyons, A. (2018). Mapping Indicators of Groundwater Dependent Ecosystems in California: Methods Report. San Francisco, CA.
Klein, A.-M., Vaissière, B. E., Cane, J. H., Steffan-Dewenter, I., Cunningham, S. A., Kremen, C., et al. (2007). Importance of pollinators in changing landscapes for world crops. Proc. R. Soc. Lond. B Biol. Sci. 274, 303–313. doi: 10.1098/rspb.2006.3721
Kløve, B., Ala-aho, P., Bertrand, G., Boukalova, Z., Ertürk, A., Goldscheider, N., et al. (2011). Groundwater dependent ecosystems. Part I: hydroecological status and trends. Environ. Sci. Policy 14, 770–781. doi: 10.1016/j.envsci.2011.04.002
Knüppe, K., and Pahl-Wostl, C. (2011). A framework for the analysis of governance structures applying to groundwater resources and the requirements for the sustainable management of associated ecosystem services. Water Resour. Manag. 25, 3387–3411. doi: 10.1007/s11269-011-9861-7
Kochenderfer, J., Adams, M. B., and Miller, G. (2007). Factors Affecting Large Peakflows on Appalachian Watersheds: Lessons from the Fernow Experimental Forest. Res Pap NRS-3. Newtown Square, PA: U.S. Department of Agriculture, Forest Service, Northern Research Station, 24. doi: 10.2737/NRS-RP-3
Koh, I., Lonsdorf, E. V., Williams, N. M., Brittain, C., Isaacs, R., Gibbs, J., et al. (2016). Modeling the status, trends, and impacts of wild bee abundance in the United States. Proc. Natl. Acad. Sci. 113, 140–145. doi: 10.1073/pnas.1517685113
Konikow, L. F., and Kendy, E. (2005). Groundwater depletion: a global problem. Hydrogeol. J. 13, 317320. doi: 10.1007/s10040-004-0411-8
Lal, R. (2008). Carbon sequestration. Philos. Trans. R. Soc. B Biol. Sci. 363, 815–830. doi: 10.1098/rstb.2007.2185
Lawrence, J. E., Skold, M. E., Hussain, F. A., Silverman, D. R., Resh, V. H., Sedlak, D. L., et al. (2013). Hyporheic zone in urban streams: a review and opportunities for enhancing water quality and improving aquatic habitat by active management. Environ. Eng. Sci. 30, 480–501. doi: 10.1089/ees.2012.0235
Leyer, I., Mosner, E., and Lehmann, B. (2012). Managing floodplain-forest restoration in European river landscapes combining ecological and flood-protection issues. Ecol. Appl. Publ. Ecol. Soc. Am. 22, 240–249. doi: 10.1890/11-0021.1
Lowrance, R. (1998). “Riparian forest ecosystems as filters for nonpoint-source pollution,” in Successes, Limitations, and Frontiers in Ecosystem Science, eds M. L. Pace and P. M. Groffman (New York, NY: Springer), 113–141. doi: 10.1007/978-1-4612-1724-4_5
Mayer, P. M., Reynolds, S. K Jr, McCutchen, M. D., and Canfield, T. J. (2007). Meta-analysis of nitrogen removal in riparian buffers. J. Environ. Qual. 36, 1172–1180. doi: 10.2134/jeq2006.0462
McPhee, J., and Yeh, W. W.-G. (2004). Multiobjective optimization for sustainable groundwater management in semiarid regions. J. Water Resour. Plan. Manag. 130, 490–497. doi: 10.1061/(ASCE)0733-9496(2004)130:6(490)
Millennium Ecosystem Assessment (2005). Ecosystems and Human Well-Being: Synthesis. Washington, DC: Island Press.
Montakhab, A., Yusuf, B., Ghazali, A. H., and Mohamed, T. A. (2012). Flow and sediment transport in vegetated waterways: a review. Rev. Environ. Sci. Biotechnol. 11, 275–287. doi: 10.1007/s11157-012-9266-y
Munns, W. R., Rea, A. W., Mazzotta, M. J., Wainger, L. A., and Saterson, K. (2015). Toward a standard lexicon for ecosystem services. Integr. Environ. Assess. Manag. 11, 666–673. doi: 10.1002/ieam.1631
Murray, B. R., Hose, G. C., Eamus, D., and Licari, D. (2006). Valuation of groundwater-dependent ecosystems: a functional methodology incorporating ecosystem services. Aust. J. Bot. 54, 221–229. doi: 10.1071/BT05018
Murray, S. J., Foster, P. N., and Prentice, I. C. (2012). Future global water resources with respect to climate change and water withdrawals as estimated by a dynamic global vegetation model. J. Hydrol. 448–449, 14–29. doi: 10.1016/j.jhydrol.2012.02.044
Naumburg, E., Mata-gonzalez, R., Hunter, R. G., Mclendon, T., and Martin, D. W. (2005). Phreatophytic vegetation and groundwater fluctuations: a review of current research and application of ecosystem response modeling with an emphasis on great basin vegetation. Environ. Manage. 35, 726–740. doi: 10.1007/s00267-004-0194-7
Orellana, F., Verma, P., Loheide, S. P., and Daly, E. (2012). Monitoring and modeling water-vegetation interactions in groundwater-dependent ecosystems. Rev. Geophys. 50. doi: 10.1029/2011RG000383
Palmer, M. A., Filoso, S., and Fanelli, R. M. (2014). From ecosystems to ecosystem services: Stream restoration as ecological engineering. Ecol. Eng. 65, 62–70. doi: 10.1016/j.ecoleng.2013.07.059
Perrone, D., and Rohde, M. M. (2016). Benefits and economic costs of managed aquifer recharge in California. San Franc. Estuary Watershed Sci. 14. doi: 10.15447/sfews.2016v14iss2art4
Pettit, N. E., and Froend, R. H. (2018). How important is groundwater availability and stream perenniality to riparian and floodplain tree growth? Hydrol. Process. 32, 1502–1514. doi: 10.1002/hyp.11510
Pfeiffer, L., and Lin, C.-Y. C. (2012). Groundwater pumping and spatial externalities in agriculture. J. Environ. Econ. Manag. 64, 16–30. doi: 10.1016/j.jeem.2012.03.003
Potts, S. G., Biesmeijer, J. C., Kremen, C., Neumann, P., Schweiger, O., and Kunin, W. E. (2010). Global pollinator declines: trends, impacts and drivers. Trends Ecol. Evol. 25, 345–353. doi: 10.1016/j.tree.2010.01.007
Power, G., Brown, R. S., and Imhof, J. G. (1999). Groundwater and fish—insights from northern North America. Hydrol. Process. 13, 401–422. doi: 10.1002/(SICI)1099-1085(19990228)13:3<401::AID-HYP746>3.0.CO;2-A
Ramcharan, A., Hengl, T., Nauman, T., Brungard, C., Waltman, S., Wills, S., et al. (2018). Soil property and class maps of the conterminous united states at 100-meter spatial resolution. Soil Sci. Soc. Am. J. 82, 186–201. doi: 10.2136/sssaj2017.04.0122
Reid, W. V. (2005). Millennium Ecosystem Assessment: Ecosystems and Human Well-being. Available online at: https://www.wri.org/research/millennium-ecosystem-assessment-ecosystems-and-human-well-being (accessed October 19, 2022).
Richardson, S., Irvine, E., Froend, R., Boon, P., Barber, S., and Bonneville, B. (2011). Australian Groundwater-dependent Ecosystem Toolbox Part 1: Assessment Framework. Canberra, ACT: National Water Commission.
Rohde, M. M., Froend, R., and Howard, J. (2017). A global synthesis of managing groundwater dependent ecosystems under sustainable groundwater policy. Ground Water 55, 293–301. doi: 10.1111/gwat.12511
Rohde, M. M., Matsumoto, S., Howard, J., Liu, S., Riege, L., and Remson, E. J. (2018). Groundwater Dependent Ecosystems under the Sustainable Groundwater Management Act: Guidance for Preparing Groundwater Sustainability Plans. Available online at: https://groundwaterresourcehub.org/sgma-tools/gsp-guidance-document/ (accessed June, 4, 2020).
Rohde, M. M., Reynolds, M., and Howard, J. (2020). Dynamic multibenefit solutions for global water challenges. Conserv. Sci. Pract. 2. doi: 10.1111/csp2.144
Saltveit, S. J., and Brabrand, Å. (2013). Incubation, hatching and survival of eggs of Atlantic salmon (Salmo salar) in spawning redds influenced by groundwater. Limnologica 43, 325–331. doi: 10.1016/j.limno.2013.05.009
Scanlon, B. R., Faunt, C. C., Longuevergne, L., Reedy, R. C., Alley, W. M., McGuire, V. L., et al. (2012). Groundwater depletion and sustainability of irrigation in the US High Plains and Central Valley. Proc. Natl. Acad. Sci. 109, 9320–9325. doi: 10.1073/pnas.1200311109
Seavy, N. E., Gardali, T., Golet, G. H., Griggs, F. T., Howell, C. A., Kelsey, R., et al. (2009). Why climate change makes riparian restoration more important than ever: recommendations for practice and research. Ecol. Restor. 27, 330–338. doi: 10.3368/er.27.3.330
Sechu, G. L., Nilsson, B., Iversen, B. V., Greve, M. B., and Greve, M. H. (2023). Historical loss of groundwater-dependent terrestrial ecosystems in undrained and artificially drained landscapes in Denmark. Water. 15, 946. doi: 10.3390/w15050946
Silvius, M. J., Oneka, M., and Verhagen, A. (2000). Wetlands: lifeline for people at the edge. Phys. Chem. Earth Part B Hydrol. Oceans Atmosphere 25, 645–652. doi: 10.1016/S1464-1909(00)00079-4
State of California (2014). Sustainable Groundwater Management Act (SGMA). Available online at: https://water.ca.gov/Programs/Groundwater-Management/SGMA-Groundwater-Management (accessed October 19, 2022).
TEEB (2010). Mainstreaming the Economics of Nature: A Synthesis of the Approach, Conclusions and Recommendations of TEEB Launch of final TEEB Report. Available online at: http://www.teebweb.org/mainstreaming-the-economics-of-nature-a-synthesis-of-the-approach-conclusions-and-recommendations-of-teeb-launch-of-final-teeb-report/ (accessed February 24, 2019).
Thomas, H., and Nisbet, T. R. (2007). An assessment of the impact of floodplain woodland on flood flows. Water Environ. J. 21, 114–126. doi: 10.1111/j.1747-6593.2006.00056.x
Turyahabwe, N., Kakuru, W., Tweheyo, M., and Tumusiime, D. M. (2013). Contribution of wetland resources to household food security in Uganda. Agric. Food Secur. 2, 5. doi: 10.1186/2048-7010-2-5
U.S. Geological Survey. (2016). National Hydrography Dataset, High Resolution, v220. Washington, DC: .S. Geological Survey. Available online at: http://nhdftp.usgs.gov/DataSets/Staged/States/FileGDB/HighResolution/NHDH_CA_931v220.zip.
United States Census Bureau. (2016). 2016 Geographic Boundaries. Available online at: https://www.census.gov/programs-surveys/acs/geography-acs/geography-boundaries-by-year/2016.html
United States Department of Agriculture National Agricultural Statistics Service. (2018). Cropland Data Layer. Available online at: https://data.nal.usda.gov/dataset/cropscape-cropland-data-layer
Velis, M., Conti, K. I., and Biermann, F. (2017). Groundwater and human development: synergies and trade-offs within the context of the sustainable development goals. Sustain Sci. 12, 1007–1017. doi: 10.1007/s11625-017-0490-9
Verhoeven, J. T. A., and Setter, T. L. (2010). Agricultural use of wetlands: opportunities and limitations. Ann. Bot. 105, 155–163. doi: 10.1093/aob/mcp172
Villholth, K., and Ross, A. (2018). Overview on Groundwater-Based Natural Infrastructure. Retrieved from: http://gripp.iwmi.org/natural-infrastructure/overview-on-groundwater-based-naturalinfrastructure/
Wachniew, P., Witczak, S., Postawa, A., Kania, J., Zurek, A., Rozanski, K., et al. (2014). Groundwater Dependent Ecosystems and man: conflicting groundwater uses. Geol. Q. 58, 695–706. doi: 10.7306/gq.1168
Ward, F. A., Roach, B. A., and Henderson, J. E. (1996). The economic value of water in recreation: evidence from the california drought. Water Resour. Res. 32, 1075–1081. doi: 10.1029/96WR00076
Warren, D. R., Robinson, J. M., Josephson, D. C., Sheldon, D. R., and Kraft, C. E. (2012). Elevated summer temperatures delay spawning and reduce redd construction for resident brook trout (Salvelinus fontinalis). Glob. Change Biol. 18, 1804–1811. doi: 10.1111/j.1365-2486.2012.02670.x
Widger, B. (2019). California Greenhouse Gas Emissions for 2000 to 2017. Trends of Emissions and Other Indicators. 25. Available online at: https://ww2.arb.ca.gov/ghg-inventory-archive
Wiedeman, H. G., and Bayer, G. (2012). Papyrus the Paper of Ancient Egypt. Washington, DC: ACS Publ.
Wilbanks, T. J., and Kates, R. W. (1999). Global change in local places: how scale matters. Clim. Change 43, 601–628. doi: 10.1023/A:1005418924748
Williams, N. M., and Kremen, C. (2007). Resource distributions among habitats determine solitary bee offspring production in a mosaic landscape. Ecol. Appl. 17, 910–921. doi: 10.1890/06-0269
WWAP (2018). The United Nations World Water Development Report 2018: Nature-Based Solutions for Water. Paris: UNESCO.
Keywords: groundwater depended ecosystems (GDEs), groundwater, ecosystem services, California, regulating benefits, GDEs
Citation: Howard JK, Dooley K, Brauman KA, Klausmeyer KR and Rohde MM (2023) Ecosystem services produced by groundwater dependent ecosystems: a framework and case study in California. Front. Water 5:1115416. doi: 10.3389/frwa.2023.1115416
Received: 03 December 2022; Accepted: 17 May 2023;
Published: 09 June 2023.
Edited by:
Tatenda Dalu, University of Mpumalanga, South AfricaReviewed by:
Jodie Dabovic, NSW Government, AustraliaCopyright © 2023 Howard, Dooley, Brauman, Klausmeyer and Rohde. This is an open-access article distributed under the terms of the Creative Commons Attribution License (CC BY). The use, distribution or reproduction in other forums is permitted, provided the original author(s) and the copyright owner(s) are credited and that the original publication in this journal is cited, in accordance with accepted academic practice. No use, distribution or reproduction is permitted which does not comply with these terms.
*Correspondence: Jeanette K. Howard, amVhbmV0dGVfaG93YXJkQHRuYy5vcmc=
Disclaimer: All claims expressed in this article are solely those of the authors and do not necessarily represent those of their affiliated organizations, or those of the publisher, the editors and the reviewers. Any product that may be evaluated in this article or claim that may be made by its manufacturer is not guaranteed or endorsed by the publisher.
Research integrity at Frontiers
Learn more about the work of our research integrity team to safeguard the quality of each article we publish.