- 1Small Island Sustainability Programme, University of The Bahamas, Nassau, Bahamas
- 2Geosciences Department, Oberlin College, Oberlin, OH, United States
- 3UNESCO Bahamas National Commission for UNESCO-IHP, Nassau, Bahamas
Small island nations make up more than one-quarter of the countries on earth, yet information on the limitations of the hydrologic cycle in small islands and strategies to address freshwater availability are lacking. Typically, all islands are characterized by their limited supply of freshwater, and their governments commonly rely on large-scale interventions to provide residents with potable water. The island of New Providence in The Bahamas is home to the capital city of Nassau and almost three-quarters of the country's population. New Providence has a long history of hydrologic interventions to supply domestic and industrial water. Historic groundwater sources have been over-extracted to severe levels of salinization, and some groundwater aquifers have not recovered after more than 8 years later. Fresh water was barged in from the larger Andros Island for ~40 years, which ultimately became insufficient to meet demand on New Providence. In addition to an aggressive Non-Revenue-Water (NRW) Program, desalination of ocean water via diesel-operated reverse osmosis plants has served as the primary source of potable water for the island. Present concerns have grown due to its contributions to greenhouse gas emissions. The Bahamas is also investigating alternative mechanisms for providing clean drinking water to the country, with Ocean Thermal Energy Conversion (OTEC) under consideration to co-generate clean energy in addition to drinking water. This article reveals that historic interventions to source drinking water for New Providence Island have proven to be unsustainable and ultimately have threatened the freshwater availability. Current efforts to investigate drinking water provision through OTEC are promising, particularly for the potential co-generation of energy and to promote sustainable development. This solution may be promising for other small islands where development is often challenged by water availability and sustainable energy provision.
Introduction: Description of the nature of the problem being addressed and rationale for the proposed innovation
Fresh water sources on small island nations are challenged by limited availability, climate variations, sea level rise, extreme events associated with climate change, and population growth (White and Falkland, 2010; Holding and Allen, 2016; Osorio et al., 2016; Post et al., 2018; Spellman et al., 2021). Small island water supplies typically originate from groundwater, surface water where available, desalination of ocean water, or rainwater harvesting in limited amounts (Holding and Allen, 2016). Groundwater serves as the primary source of drinking water on many islands, particularly on predominantly limestone carbonate islands where surface waters are lacking (White and Falkland, 2010). Groundwater availability is dependent upon recharge through precipitation, evapotranspiration losses, mixing with ocean water, and extraction for human consumption (Post et al., 2018). As small island nations often have limited freshwater, large-scale interventions in both the short- and long-term are common to supply residents with fresh water for domestic, industrial, and agricultural uses.
Precipitation serves as the only source of input into small islands, and deep groundwater is connected laterally to ocean water. Precipitation infiltrates into the subsurface and floats atop deeper saline water in freshwater lenses, known as Ghyben-Hertzberg Lenses (Cant and Weech, 1986; Bryan et al., 2016; Bedekar et al., 2019; Tang et al., 2020). The size of the freshwater lens is constrained by precipitation amounts and geologic influences (Cant and Weech, 1986; Bryan et al., 2016; Post et al., 2018). The vertical profile of freshwater lenses is typically characterized by an upper potable section with low chloride concentrations atop a transition zone with brackish water. Below these zones is saline water with chloride concentrations close to seawater (Cant and Weech, 1986).
Climate change continues to threaten the limited water supplies on small islands. Most freshwater lenses are found near the subsurface and are therefore vulnerable to sea level rise (White and Falkland, 2010). Shifting weather patterns are changing the seasonality of regions, increasing surface air temperatures, and altering the frequency and amount of precipitation (Wu et al., 2020). Rising surface air temperatures cause an increase in evapotranspiration, which may reduce the size of freshwater lenses (Wu et al., 2020). Additionally, extreme events such as hurricanes lead to storm surge and wave overwash that result in physical mixing of saline ocean water and the freshwater lens, effectively contaminating the fresh groundwater being extracted for drinking water (Storlazzi et al., 2018; Yang et al., 2021). Therefore, as populations increase and the effects of climate change intensify, already limited supplies of freshwater storage on small islands are placed under even more stress.
In addition to climate change, many other challenges exist that complicate water provision on small islands. Due to the underlying saltwater beneath freshwater lenses, over-pumping of aquifers can lead to upcoming or lateral saltwater intrusion (Holding and Allen, 2016). Once this occurs, natural recovery of the freshwater lens can take many years. To address shortages in the natural freshwater supply and to aid small islands with meeting the demand for fresh water, reverse osmosis is often used on island locations to desalinate ocean water for drinking. However, this process is very energy intensive and can contribute to greenhouse gas emissions on islands that disproportionately experience the effects of climate change (Li et al., 2018; Liponi et al., 2022).
Water challenges on small islands differ from larger continental countries, particularly on islands where surface waters are lacking. Although small island nations comprise more than one-quarter of the countries on earth, limited information exists on freshwater limitations and strategies to address this problem. Many small islands and coastal regions are investigating alternative mechanisms for providing clean drinking water to coastal populations, with Ocean Thermal Energy Conversion (OTEC) gaining renewed consideration. OTEC is a renewable energy technology that provides both clean energy and drinking water.
This article provides a historical assessment of the island of New Providence in The Bahamas, which has implemented a range of hydrology interventions to provide its growing population with a public water supply, with the results serving as an example for other small islands. While some of the measures were implemented on a temporary basis, the use of alternative sources became long-term strategies. In this article we address: how have the past sources of public water supply impacted local island hydrology? What additional options exist to provide potable water and reduce vulnerability of small islands? The objective was to document the sustainability of the historical interventions to provide water on a small, densely populated island, while discussing the potential for OTEC with the goal of sharing lessons for other small islands facing allied challenges. The environmental cost of extracting fresh water from the natural groundwater resources or the use of desalination is discussed, as well as the possibility of diversifying drinking water sources through OTEC.
Context (setting and population) in which the innovation occurs
The Bahamas is an archipelago of over 700 islands running into the Caribbean, southeast of Florida, USA off the continental shelf of North America (Figure 1). The country experiences a sub-tropical to tropical climate from the north to the south along the archipelago, with ~1,500 mm of annual precipitation in the northern region to 750 mm of annual precipitation in the southern islands (Whitaker and Smart, 1997; United States Army Corps of Engineers, 2004; Dose et al., 2014). The island of New Providence is home to the capital city of Nassau and contains nearly three-quarters of the population (Bahamas Department of Statistics, 2012). New Providence is located in the north-central region of the archipelago. The land size is approximately 20,719 hectares (Cant and Weech, 1986), and, despite containing the majority of the country's population, the island is only the 21st largest by land area in the country (Cant and Weech, 1986).
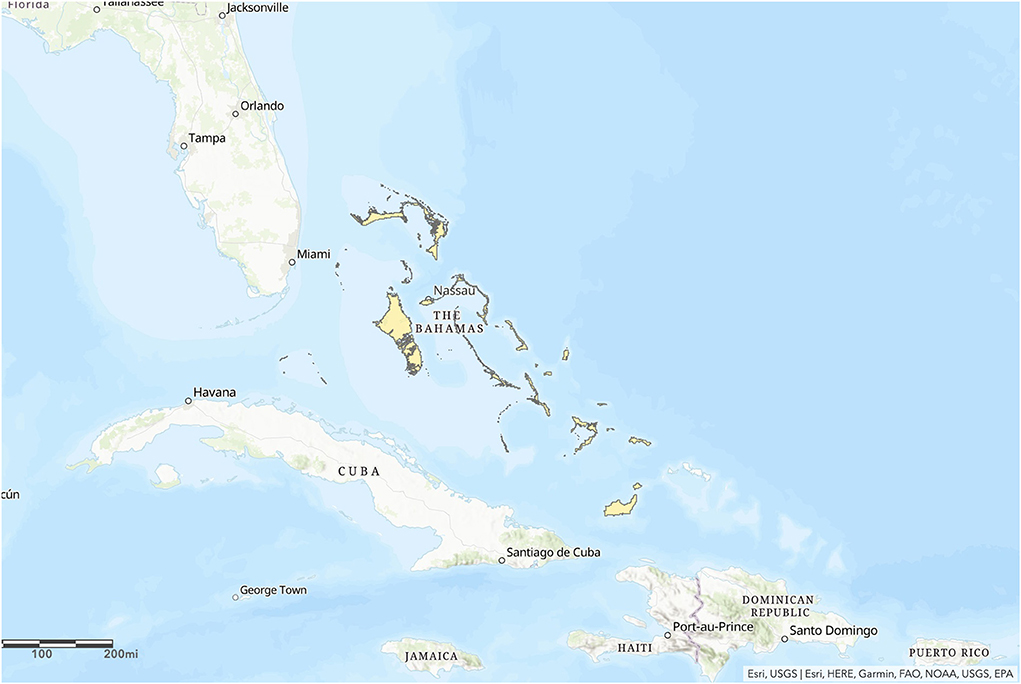
Figure 1. Location of the archipelago of The Bahamas and Nassau, New Providence. Andros, the source of barged water, is the large island located to the west of New Providence.
The mean annual precipitation of New Providence is ~1,250 mm/year (Spellman et al., 2021). The only fresh water storage on the island is in the freshwater lens, which lies at a depth of < 1.5 m from the land surface (United States Army Corps of Engineers, 2004). Cant and Weech (1986) found an average freshwater lens thickness of 9.3 m with a maximum thickness up to 15.5 m. The underlying substrate is Lucayan Limestone, which is a carbonate system characterized by high porosity and low hydraulic conductivity (Whitaker and Smart, 1997). Fresh surface water bodies do not exist on New Providence, as carbonate substrate does not support streams and rivers, although several brackish lakes exist (United States Army Corps of Engineers, 2004). Water availability is low enough in The Bahamas to be considered scarce by the United Nations criteria (Ekwue, 2010; Muralidharan, 2012).
Previous assessments conducted by the United States Army Corps of Engineers (2004) found that the island of New Providence had a calculated water demand of 48,279 m3/day based on an assumption of 0.23 m3 per person per day. Adjusting for population increases, the current demand based on the same assumed usage would be 62,372 m3/day. New Providence has a maximum volume of 43,779 m3/day available through freshwater lenses (United States Army Corps of Engineers, 2004). Therefore, supplemental water sources are necessary to meet the demand, and the island has sourced water through a variety of mechanisms.
Historical interventions for water provision
New Providence has a long history of interventions to meet its growing demand for water that has exceeded the island's natural sources of available fresh water. The primary source of potable water since the 1600's was rain water collection and hand dug wells, until a piped water system was established in 1913 (Water Sewerage Corporation, 2022a). In 1928 “A Bill to provide a Water Supply and Water-borne Sewerage System for the City of Nassau and its Suburbs” was passed by the legislation that established the first wellfield, Blue Hill Waterworks, and the first groundwater pumping station for the island (Water Sewerage Corporation, 2022a). The wellfield at Blue Hills was intended to be a permanent solution. However, over pumping and drought conditions in the 1930's resulted in the salinization of the groundwater resources and abandonment of the wellfield in 1937. To date, the freshwater lens has not naturally recovered, and now the resource exists within close proximity of a power generation facility (Water Sewerage Corporation, 2022a). Salinization of a freshwater lens can readily occur due to storm surge, wave overwash, climate conditions, or overpumping, which underscores a major challenge with relying on groundwater on islands.
Several other wellfields areas were established to provide drinking water, including the Perpalls Waterworks, Windsor Wellfield, Southwest Waterworks, and two wellfields at the Prospect Waterworks (Figure 2). Several wellfields have a history of salinization, deforestation, illegal squatting practices on the surrounding lands, and land-based contamination from oil or fuel spills, other unsustainable land use practices, and waste water contamination from squatters (Water Sewerage Corporation, 2005). Three of six total wellfields were overpumped, resulting in abandonment due to salinization. A fourth wellfield was abandoned due to the proximity of the reserves to adjacent communities. A fifth wellfield was also abandoned due to developments in close proximity, with the pumping station now converted to office space. The final historical wellfield currently remains operational but could be abandoned in favor of development and/or deforestation, which would eliminate groundwater as a source of water for the island. However, present efforts are focused on maintaining the area within the forest reserve inventory or as a protected water resources area. Despite groundwater serving as the only storage of fresh water on the island, small islands generally have very limited land area available for their full development. The pressures for alternative land development, and the impacts from existing land uses continues to create major challenges for utilizing this source.
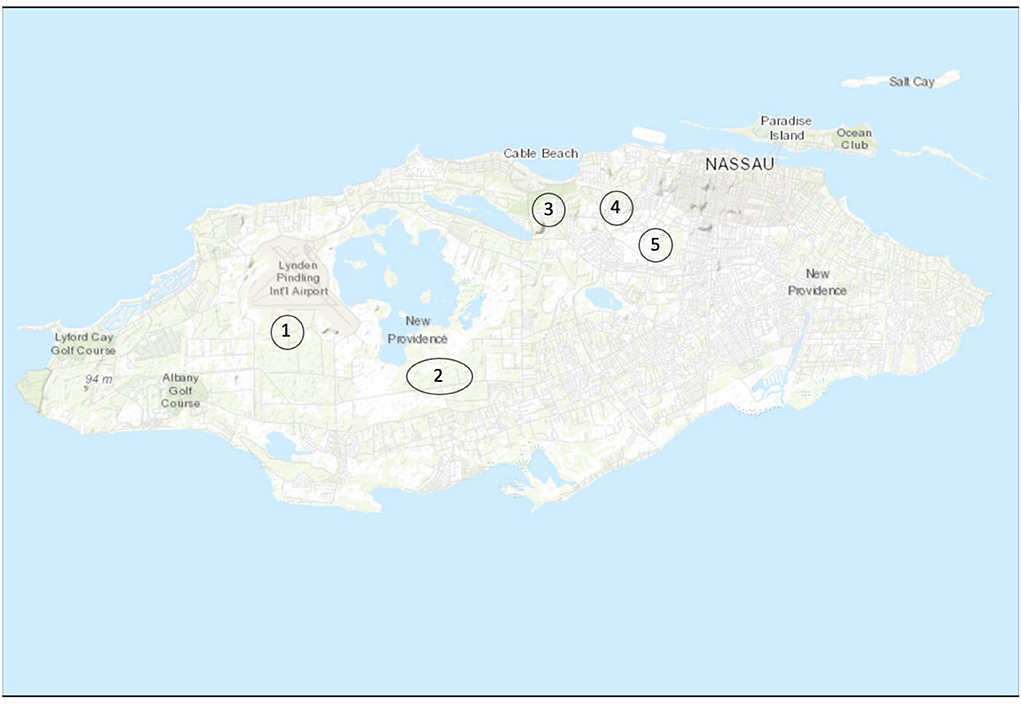
Figure 2. General locations of the five major wellfields in New Providence that have served as sources for public water distributed by the government. The wellfields include (1) Windsor Wellfield, (2) Southwest Waterworks, (3) Prospect Waterworks, (4) Perpalls Waterworks, and (5) Blue Hills Waterworks.
In addition to groundwater, between 1958 and 1959 a distillation plant was constructed on New Providence at Clifton Pier to produce 4,546 m3/day. The distilled water was available in 1960, with several equipment modifications, but the freshwater output of 5,455 m3/day was not achieved for more than a few days, and operations were abandoned due to excessive maintenance and repair expenses. All additional attempts of distillation or desalination in the 1960's through the 1970's were also vacated due to excessive operational expenditure (Water Sewerage Corporation, 2022a).
In the 1970's, groundwater supplies were insufficient to meet the demand of the population, and water rationing was implemented (Water Sewerage Corporation, 2022a). In 1976 the Water and Sewerage Corporation (WSC) was created as the governing body charge with managing and providing supplies for the island of New Providence and the other islands (Water Sewerage Corporation, 2022a). WSC shares the responsibility of water and sanitation oversight with several government agencies and institutions (United States Army Corps of Engineers, 2004). In 1977 WSC began shipping water from Andros, the largest island in the archipelago, located west of New Providence (Water Sewerage Corporation, 2022a). Andros is the island with the greatest availability of freshwater resources of all the islands. The maximum available volume of water is ~1,136,523 m3/day with a calculated water demand of 1,728 m3/day (United States Army Corps of Engineers, 2004). Barged water served as more than half of New Providence's water supply with average volumes of over 21,821 m3/day being shipped in 2000 (United States Army Corps of Engineers, 2004). The shift of local hydrology through the transport of large amounts of water from Andros to New Providence affected both islands, by not only reducing the available water in Andros but increasing the amount of fresh water discharged in New Providence. Barging continued for 34 years until this source was supplemented and eventually fully replaced by desalinated water. The equipment and expenses associated with the shipment of the water escalated, and the cost eventually became unsustainable. Management practices continue to date for the blending of the groundwater resources with desalinated water, in an effort to continue to utilize the groundwater reserves.
In the late 1990's to early 2000's, the WSC investigated alternative sources to supply the capital city, as water demand in Nassau and the costs of shipping water were both increasing. Therefore, a revised plan for desalination as the primary source of water was developed. In 1997, the desalination facility at the Windsor Wellfield opened to produce 11,820 m3/day at < 50 parts per million (ppm) of total dissolved solids to permit blending with the higher salinity groundwater reserves (Consolidated Water Co. Ltd., 2022). In 2005, an additional reverse osmosis/desalination plant was built at the Blue Hills Waterworks (Water Sewerage Corporation, 2022a). Their capacity was initially 27,277 m3/day (Water Sewerage Corporation, 2022a), but was further increased to 54,553 m3/day to meet the water demand for the island (Consolidated Water Co. Ltd., 2022). All reverse osmosis facilities are operated off the fossil-fueled national grid and included stand-by diesel driven generation. The current output of the reverse osmosis plants will most likely become unsustainable for the population growth on the island, and the groundwater supply can eventually be completely eliminated. Upgrades to the existing reverse osmosis supply will have to increase output, based solely on demand. The Government of The Bahamas (2015) recognized that a rapid shift occurred toward desalination, which resulted in its overreliance on processed water through a source that is effectively contributing to climate change. Efforts are now being explored to address the energy concerns, by pairing desalination with the OTEC technology.
Historically, New Providence has experienced consistent water supply issues due to poor quality, low pressure, and increasing non-revenue water (NRW), or water that is lost before reaching the consumer (Burdescu et al., 2020). Additionally, due to low availability, the island has previously experienced extended periods of water disruptions and rationing. In 2008 to 2009, WSC developed a mechanism to reduce NRW by implementing technical interventions, with very significant improvements through the aggressive management of the NRW. Through a performance-based contract, an aggressive NRW program resulted in a reduction from 54 percent losses in the distribution system to 23 percent over a 7-year period, which is comparable to well-performing regional utility companies (Burdescu et al., 2020). The municipal supplied water is heavily subsidized in The Bahamas and therefore the true cost of the water is much higher than the purchase cost (Muralidharan, 2012). Nevertheless, The Bahamas has the highest water tariffs in the Caribbean region, at $2.78 per m3 in 2015 (Burdescu et al., 2020). In general, the cost of groundwater abstraction is significantly cheaper than reverse osmosis and barged water (Muralidharan, 2012).
Currently, desalinated ocean water is the primary source of drinking water, providing 90% of the potable water on the island, supplemented by groundwater (Water Sewerage Corporation, 2022b). However, the government recognizes the contributions to greenhouse gas emissions caused by the energy-intensive desalination process and is investigating more renewable sources (Government of The Bahamas, 2015). Due to a long history of brackish groundwater in the public water supply, in addition to the overall quality of the publicly supplied water, private companies are prominent, and the majority of Bahamians drink bottled drinking water sourced from reverse osmosis plants (Muralidharan, 2012).
Proposed innovation of Ocean Thermal Energy Conversion
Desalination in New Providence is produced through diesel operated plants. While the use of solar and geothermal energy has been investigated to power desalination globally, the potential for utilizing ocean energy has not been examined at length (Li et al., 2018). Many renewable energies harnessing the power of the ocean are still being developed, although some studies have investigated this possibility in developing countries (Hernández-Fontes et al., 2020). OTEC has great potential as a renewable energy source, eliminating the greenhouse gas emissions that are common for small island nations (Li et al., 2022). Although typically investigated as a source of energy, OTEC also has the unique ability of additionally providing potable water (Brauns, 2018).
The use of deep ocean water, such as with OTEC, is not currently practiced in the Caribbean region, and studies on its use in the region are lacking (Arias-Gaviria et al., 2020). Historically, efforts to increase renewable energy initiatives have focused on wind energy, which has been the most cost-effective renewable source; however, increases in oil prices have broadened interest in other renewables, which are now more cost-effective than fossil fuels (Fujita et al., 2012). Of the renewable sources, OTEC is a more continuous source of energy that is less affected by climate conditions, compared with alternative renewable sources (Fujita et al., 2012; International Renewable Energy Agency [IRENA], 2014; Li et al., 2018). The Bahamas is located in a region that is suitable for OTEC (Fujita et al., 2012), and the specific challenges that the country faces with water and energy limitations make this a viable option that may be economically advantageous and beneficial for sustainable development.
Detail to understand key programmatic elements
OTEC capitalizes on the thermal differential between surface water and deep ocean water, requiring a minimum difference of 20°C (Fujita et al., 2012; International Renewable Energy Agency [IRENA], 2014; Vega, 2014; Osorio et al., 2016; Langer et al., 2020; Liponi et al., 2022). In instances where the temperature differential does not reach 20°C, additional measures can be taken to increase the difference (Fujita et al., 2012). A working fluid in the system is converted into vapor at the warmer surface level, powering a turbine and generator, while the cooler deep water turns the vapor back into a liquid (Fujita et al., 2012). In a closed-loop system, the working fluid typically consists of ammonia and is reused continuously (Vega, 2002). In an open-loop system, the working fluid can be ocean water, which is flash-evaporated into desalinated water vapor that can be condensed and used as a drinking water source (Vega, 2002, 2014; Figure 3). OTEC is particularly valuable on small islands as an energy source that also co-generates potable water, when sea water is used as the working fluid and the water vapor is converted into freshwater (Fujita et al., 2012; Muralidharan, 2012; Liponi et al., 2022).
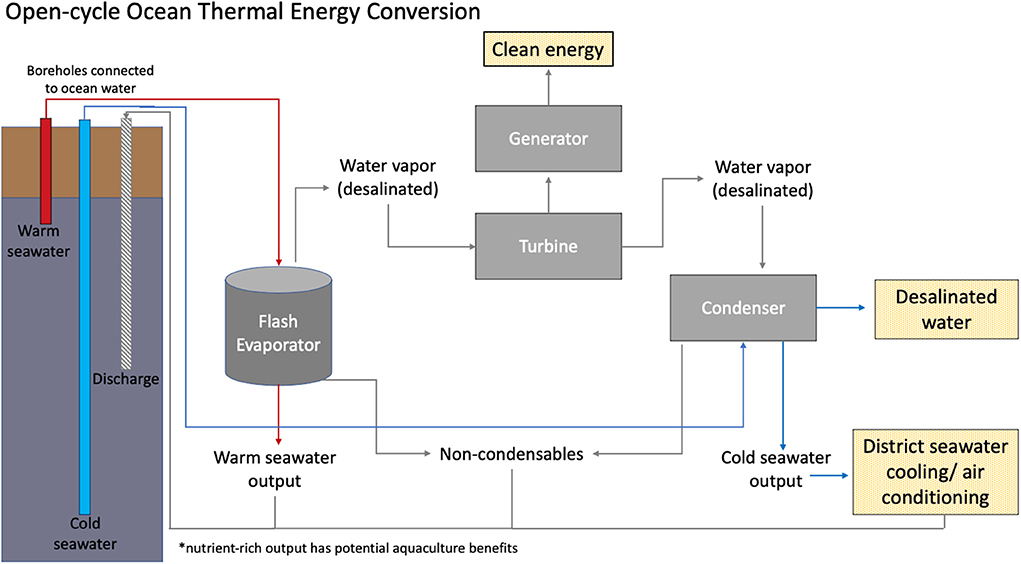
Figure 3. Schematic of an open-cycle Ocean Thermal Energy Conversion using sea water sourced through boreholes. In this system, both energy and desalinated water are produced. Figure adapted from International Renewable Energy Agency [IRENA] (2014).
As ocean water infiltrates laterally underneath the Bahamas islands, colder saline groundwater is found at depth, resulting in a reverse geothermal gradient (Cant, 2012). While other locations may use ocean water directly as the source, Bahamian political constraints have long prevented the direct pumping of ocean water in favor of the use of deep saline groundwater. The inverted geothermal conditions of the groundwater resources have been readily documented; deep seawater wells have been in use for air conditioning for decades. A previous study that collected temperature data from deep boreholes revealed that the temperature of the groundwater decreases with depth, similar to the surrounding sea (Ardaman and Associates Inc., 2013). At a depth of 180 m, water temperature is approximately 21.5°C (Ardaman and Associates Inc., 2013). Projections indicate that at ~900–1,000 m, the water temperature may reach 6°C. As ocean surface temperatures range from 24.5 to 31.2°C (World Sea Temperature, 2022), this temperature differential would be adequate for implementing OTEC. Additional test boreholes may be required to be drilled to obtain more extensive temperature profiles.
The Bahamas is currently investigating the potential for OTEC within the country through projects that would use deep wells connected to ocean water, rather than directly using seawater. Direct sea intake and outfall is not practiced within The Bahamas for seawater reverse osmosis, drainage, or seawater district cooling. Instead, deep groundwater wells are used for all processes since groundwater is highly connected to ocean water (Cant, 2012). Similarly, OTEC in the country will also use groundwater wells for both the source water and the discharge of the effluent.
Discussion
Small islands have the potential to benefit greatly from OTEC (Langer et al., 2020; Vera et al., 2020; Li et al., 2022). Sustainable development of The Bahamas is currently challenged by both water provision and energy constraints. Although desalination of ocean water is useful in The Bahamas and other small islands due to its ability to convert large quantities of saline water to freshwater for domestic use, the environmental toll of desalination is problematic for small islands that are already vulnerable to climate change. Current water provision through desalination is extremely energy intensive, contributing to global greenhouse gas emissions and causing additional environmental issues (Li et al., 2018). Desalination is more energy intensive than other potable water supply options, due to the energy required for the removal of salts from the water, and ~30–50% of the cost of desalinated water is due to the energy consumed (Li et al., 2018). The energy required for desalination is ~10 times the energy required to treat surface water (Li et al., 2018). Although desalination is currently seen as a permanent solution for water provision in The Bahamas and other islands, increasing challenges from energy intensity and climate change suggest alternative mechanisms will become necessary.
Energy provision in the Caribbean is particularly challenged by inefficient energy systems, higher energy imports than energy exports, and heavy reliance on fossil fuels (Arias-Gaviria et al., 2020). This reliance on fossil fuels has resulted in a higher cost of electricity than in developing countries (Singh and Ephraim, 2016). Fossil fuel usage is a growing global concern, particularly due to the threats of spills and contribution to climate change (Fujita et al., 2012). Despite having fewer overall contributions to greenhouse gas emissions compared to larger countries, small islands have some of the highest per capita emissions of greenhouse gases, primarily due to their consumption of fossil fuels (Osorio et al., 2016). As a result, energy provision can be a hinderance to sustainable development, particularly on small islands (Osorio et al., 2016; Arias-Gaviria et al., 2020; Li et al., 2022). The Bahamas produces almost all its energy through fossil fuels. In recent years, more attention has been placed in the country on the use of solar energy, although not on a wide scale. The cost of energy in The Bahamas is among the highest in the world (World Bank, 2022). Alternative methods to provide energy are essential from both an economic and an environmental perspective.
OTEC is a relatively clean energy source, particularly when water is used instead of other working fluids, such as ammonia (Vega, 2002). In addition to energy and water provision, OTEC has other benefits. Deep seawater extracted for OTEC can also be used for air conditioning and sea water district cooling (Vega, 2002; Cant, 2012; Fujita et al., 2012; Singh and Ephraim, 2016). The effluent of OTEC has the potential to yield nutritional supplements that can be used in aquaculture, which is useful in small island nations as additional agricultural sources, improving the overall economic viability (Fujita et al., 2012; Vera et al., 2020). However, deep seawater is richer in nutrients than surface water, and effluent could cause eutrophication of surface waters, unless released at a depth of at least 70 m to reduce this potential (Fujita et al., 2012; Rahman et al., 2022). Nearshore habitats commonly found within Bahamian coastal zones, including coral reefs and seagrasses, are sensitive to nutrients and turbid waters (Rogers, 1990; Fujita et al., 2012), and therefore caution needs to be taken with the location of discharge of the effluent. However, this is not applicable for The Bahamas, which does not directly dispose of wastewaters in the ocean, instead discharging through deep groundwater wells.
Innovative methods of implementation have the potential for even more benefits to countries such as The Bahamas. Osorio et al. (2016) proposed an Ocean Technology Ecopark concept in San Andres Island, Colombia, to incorporate an OTEC plant, alternative uses of deep ocean water, and a research and development center. In The Bahamas, a similar Ecopark could allow for the country to capitalize on additional uses of OTEC and promote further research and development of the technology. Linkages between commercial and academic research is necessary to enhance transparency of OTEC, and particularly to more accurately develop cost estimates (Langer et al., 2020).
Local perception of environmental, economic, and political impacts and engagement of key stakeholders are critical for the success of OTEC projects (Osorio et al., 2016; Hernández-Fontes et al., 2020). Government involvement and prioritization are particularly important for the success of OTEC in The Bahamas and other small islands. The Bahamas is a signatory to the Paris Agreement, pledging to reduce climate change contributions, and a major component of this promise is to reduce its reliance on fossil fuels (Government of The Bahamas, 2015). OTEC can assist with the country meeting these goals.
Acknowledgment of any conceptual or methodological constraints
Despite the many benefits of OTEC, the technological and economic constraints have proven challenging, and environmental risks exist with OTEC (Fujita et al., 2012). Economic barriers to OTEC are significant and not widely discussed in the literature (Langer et al., 2020). Furthermore, economic information on existing projects is not widely available, nor is the information easy to validate; more transparent cost information on OTEC is necessary (Langer et al., 2020). Deep seawater is expensive to ship inland, but OTEC projects established in coastal zones are less expensive (Fujita et al., 2012). By using deep groundwater wells in The Bahamas, the cost is estimated to be less than marine abstraction for the required cold water. Grandelli (1997) calculated that installing a deep cold-water marine pipe would be approximately half of the entire cost of building an OTEC plant in western New Providence, which he estimated at $56.4 million in 1997. Muralidharan (2012) conducted a feasibility study in The Bahamas and found that the use of an OTEC facility to co-generate electricity and water could result in costs much lower than the current prices of either one. A water-energy nexus for The Bahamas may effectively involve the use of the deep groundwater resources and pairing OTEC technologies with desalination for the provision of freshwater and the production of energy (Bowleg, 2022).
The environmental effects of OTEC are also important considerations prior to development. For example, nutrient-rich deep waters have the potential to cause eutrophication when not used for alternative processes, such as aquaculture (Muralidharan, 2012). When deep ocean water is used, small organisms can become entrapped on the intake screens; however, by using deep groundwater boreholes connected to ocean water, this problem would be eliminated. In The Bahamas, the use of deep groundwater discharge wells would alleviate issues related to the cold-water abstraction and release of water associated with direct marine sourcing and return. Discharge water would be released between the depths of intake for surface warm water and deep cold water to avoid the effect on the cold-water intake. Long-term data exist from the operation of seawater district cooling on the island of New Providence, for analysis.
New Providence Island has investigated a variety of sources of fresh water for domestic and commercial use, including rain water, groundwater, barged water, and ocean desalination. All of these sources have resulted in permanent shifts of the island hydrology, as fresh water has been transported across the island, transported between islands, and discharged into deeper saline groundwater, which has reduced the freshwater availability. However, OTEC has the potential to provide an alternative water supply, while also providing energy to the island and potentially yielding several other benefits. By using deep saline groundwater sourced from boreholes, rather than piping ocean water to the land, large transference of water over distances will be avoided. Similarly, cold water that is extracted from the deep subsurface for the OTEC process would be released back into the subsurface at a higher depth, allowing for a minimal transference of water for its usage.
In general, small island nations face extreme challenges in providing fresh water for domestic, commercial, and agricultural uses, as many lack availability of fresh water to meet demand. On many islands, groundwater serves as the primary, and often the only, source of water supply, but climate change is threatening subsurface aquifers with sea level rise, surface flooding, and storm surge that results in the mixing of ocean and fresh water. Islands need to diversify their sources of water supply, but a tailored approach is also necessary for each island's unique situation, including factors such as water demand, population size, availability of surface water or groundwater, the subsurface geology, and climate-influenced conditions. OTEC is a promising solution for The Bahamas, and other small islands may also benefit from implementing this process to relieve certain pressures placed on the natural freshwater sources.
Data availability statement
The original contributions presented in the study are included in the article/supplementary material, further inquiries can be directed to the corresponding author.
Author contributions
KW conceptualized the article. All authors contributed to the intellectual development and writing of the article.
Acknowledgments
The authors wish to acknowledge the support of the Regional Caribbean Climate Change Committee Center and the Intergovernmental Hydrological Programme of UNESCO.
Conflict of interest
The authors declare that the research was conducted in the absence of any commercial or financial relationships that could be construed as a potential conflict of interest.
Publisher's note
All claims expressed in this article are solely those of the authors and do not necessarily represent those of their affiliated organizations, or those of the publisher, the editors and the reviewers. Any product that may be evaluated in this article, or claim that may be made by its manufacturer, is not guaranteed or endorsed by the publisher.
References
Ardaman and Associates Inc. (2013). Phase II Report Exploratory Well Program Summary: Baha Mar Cooling Wells Project Nassau. The Bahamas. p. 58.
Arias-Gaviria, J., Osorio, A. F., and Arango-Aramburo, S. (2020). Estimating the practical potential for deep ocean water extraction in the Caribbean. Renew. Energy 150, 307–319. doi: 10.1016/j.renene.2019.12.083
Bahamas Department of Statistics (2012). 2010 Census of Population and Housing. New Providence, The Bahamas: The Commonwealth of The Bahamas. Available online at: https://www.bahamas.gov.bs/wps/wcm/connect/c17e5637-1f39-484b-8107-a7be2176c51b/NEW+PROVIDENCE+2010+CENSUS+REPORT.pdf?MOD=AJPERES
Bedekar, V. S., Memari, S. S., and Clement, T. P. (2019). Investigation of transient freshwater storage in island aquifers. J. Contam. Hydrol. 221, 98–107. doi: 10.1016/j.jconhyd.2019.02.004
Bowleg, J. (2022). “Climate change, water resources, & renewable energy in the Bahamas,” in: 18th International Conference on Clean Energy, Kuching Sarawak, Malaysia.
Brauns, E. (2018). Towards a worldwide sustainable and simultaneous large-scale production of renewable energy and potable water through salinity gradient power by combining reverse electrodialysis and solar power? Desalination 219, 312–323. doi: 10.1016/j.desal.2007.04.056
Bryan, E., Meredith, K. T., Baker, A., Post, V. E. A., and Anderson, M. S. (2016). Island groundwater resources, impacts of abstraction and a drying climate: Rottnest Island, Western Australia. J. Hydrol. 542:704–718. doi: 10.1016/j.jhydrol.2016.09.043
Burdescu, R., van den Berg, C., Janson, N., and Alvarado, O. (2020). A Benchmark for the Performance of State-Owned Water Utilities in the Caribbean. Washington, DC: International Bank for Reconstruction and Development/The World Bank Group. p. 123. Available online at: https://openknowledge.worldbank.org/handle/10986/33251
Cant, R.V. (2012). “The Bahamas use of deep wells for effluent disposal, and as a source of seawater usable for multi-purposes,” in: Proceedings From the 21st Annual Caribbean Water and Wastewater Association Conference. Nassau, Bahamas. p. 9.
Cant, R.V., and Weech, P.S. (1986). A review of the factors affecting the development of Ghyben-Hertzberg lenses in The Bahamas. J. Hydrol. 84, 333–343. doi: 10.1016/0022-1694(86)90131-9
Consolidated Water Co. Ltd. (2022). Blue Hills: Nassau Bahamas. Available online at: https://cwco.com/portfolio/blue-hills/ (accessed June 26, 2022).
Dose, E. J., Stoeckl, L., Houben, G. J., Vacher, H. L., Vassolo, S., Dietrich, J., and Himmelsbach, T. (2014). Experiments and modeling of freshwater lenses in layered aquifers: Steady state interface geometry. J. Hydrol. 509, 621–630. doi: 10.1016/j.jhydrol.2013.10.010
Ekwue, E. I. (2010). Management of water demand in the Caribbean region: Current practices and future needs. West Ind. J. Eng. 32, 28–35.
Fujita, R., Markham, A. C., Diaz Diaz, J. E., Martinez Garia, J. R., Scarborough, C., Greenfield, P., et al. (2012). Revisiting ocean thermal energy conversion. Mar. Policy 36, 463–465. doi: 10.1016/j.marpol.2011.05.008
Government of The Bahamas (2015). Intended National Determined Contribution (INDC) Under the United Nations Framework Convention on Climate Change. Available online at: https://www4.unfccc.int/sites/submissions/INDC/Published%20Documents/Bahamas/1/Bahamas%20INDC%20Submission.pdf (accessed June 29, 2022).
Grandelli,. P. D. R. (1997). Preliminary Design of an Integrated Ocean Thermal Energy Conversion Cleanpower Plant on NEW Providence Island, Bahamas. [master's thesis] [Honolulu (HI)]: University of Hawaii.
Hernández-Fontes, J. V., Martínez, M. L., Wojtarowski, A., González-Mendoza, J. L., Landgrave, R., and Silva, R. (2020). Is ocean energy an alternative in developing regions? A case study in Michoacan, Mexico. J. Clean. Prod. 266, 121984. doi: 10.1016/j.jclepro.2020.121984
Holding, S., and Allen, D.M. (2016). Risk to water security for small islands: An assessment framework and application. Reg. Environ. Change 16, 827–839. doi: 10.1007/s10113-015-0794-1
International Renewable Energy Agency (IRENA) (2014). Ocean thermal energy conversion: Technology Brief 1. p. 24. Available online at: www.irena.org/-/media/Files/IRENA/Agency/Publication/2014/Ocean_Thermal_ Energy_V4_web.pdf
Langer, J., Quist, J., and Blok, K. (2020). Recent progress in the economics of ocean thermal energy conversion: Critical review and research agenda. Renew. Sust. Energ. Rev. 130:109960. doi: 10.1016/j.rser.2020.109960
Li, D., Fan, C., Zhang, C., and Chen, Y. (2022). Control strategy of load following for ocean thermal energy conversion. Renew. Energy 193, 595–607. doi: 10.1016/j.renene.2022.05.043
Li, X., Siddiqi, A., and Diaz Anadon, L, Narayanamurti, V. (2018). Towards sustainability in water-energy nexus: Ocean energy for seawater desalination. Renew. Sust. Energ. Rev. 82, 3833–3847. doi: 10.1016/j.rser.2017.10.087
Liponi, A., Baccioli, A., Vera, D., and Ferrari, L. (2022). Seawater desalination through reverse osmosis driven by ocean thermal energy conversion plant: Thermodynamic and economic feasibility. Appl. Therm. Eng. 213:118694. doi: 10.1016/j.applthermaleng.2022.118694
Muralidharan, S. (2012). Assessment of Ocean Thermal Energy Conversion. [master's thesis]. [Cambridge (MA)]: Massachusetts Institute of Technology.
Osorio, A. F., Arias-Gaviria, J., Devis-Morales, A., Acevedo, D., Velasquez, H. I., and Arango-Aramburo, S. (2016). Beyond electricity: The potential of ocean thermal energy and ocean technology ecoparks in small tropical islands. Energy Policy 98, 713–724. doi: 10.1016/j.enpol.2016.05.008
Post, V. E. A., Bosserelle, A. L., Galvis, S. C., and Sinclair, P. J. Werner, A. D. (2018). On the resilience of small-island freshwater lenses: Evidence of the long-term impacts of groundwater abstraction on Bonriki Island, Kiribati. J. Hydrol. 564:133–148. doi: 10.1016/j.jhydrol.2018.06.015
Rahman, A., Farrok, O., and Haque, M. M. (2022). Environmental impact of renewable energy source based electrical power plants: Solar, wind, hydroelectric, biomass, geothermal, tidal, ocean, and osmotic. Renew. Sust. Energ. Rev. 161:112279. doi: 10.1016/j.rser.2022.112279
Rogers, C. S. (1990). Responses of coral reefs and reef organisms to sedimentation. Mar. Ecol. Prog. 62, 185–202. doi: 10.3354/meps062185
Singh, A., and Ephraim, J. (2016). Ocean energy: The new energy frontier for the Eastern Caribbean small island developing states. Energy Policy 99, 1–3. doi: 10.1016/j.enpol.2016.09.024
Spellman, P., Pritt, A.B.C., and Salazar, N. (2021). Tracking changing water budgets across the Bahamian archipelago. J. Hydrol. 598:126178. doi: 10.1016/j.jhydrol.2021.126178
Storlazzi, C.D., Gingerich, S.B., van Dongeren, A., Cheriton, O.M, Swarzenski, P.W., Quataert, E., Voss, C.I., et al. (2018). Most atolls will be uninhabitable by the mid-21st century because of sea-level rise exacerbating wave-driven flooding. Sci. Adv. 4:eaap9741. doi: 10.1126/sciadv.aap9741
Tang, Y., Rathore, S.S., Lu, C., and Luo, J. (2020). Development of groundwater lens for transient recharge in strip islands. J. Hydrol. 590:125209. doi: 10.1016/j.jhydrol.2020.125209
United States Army Corps of Engineers (2004). Water Resource Assessment of The Bahamas. p. 114. Available online at: www.sam.usace. army.mil/Portals/46/docs/military/engineering/docs/WRA/Bahamas/BAHAMAS 1WRA.pdf
Vega, L. A. (2002). Ocean thermal energy conversion primer. Mar. Technol. Soc. J. 36, 25–35. doi: 10.4031/002533202787908626
Vega, L. A. (2014). Wave Energy Conversion and Ocean Thermal Energy Conversion Potential in Developing Member Countries. Mandaluyong: Asian Development Bank. doi: 10.1007/978-1-4614-5820-3_695
Vera, D., Baccioli, A., Jurado, F., and Desideri, U. (2020). Modeling and optimization of an ocean thermal energy conversion system for remote islands electrification. Renew. Energy 162, 1339–1414. doi: 10.1016/j.renene.2020.07.074
Water and Sewerage Corporation (2005). Summary of Land Acquisitions for Water Supply Purposes: New Providence. Summary Report. p. 14.
Water Sewerage Corporation (2022a). The History of the Water and Sewerage Corporation. Available online at: https://wsc.com.bs/wp-content/uploads/2021/07/WSC-Historical-Timeline.pdf (accessed June 22, 2022).
Water Sewerage Corporation (2022b). Water and Sewerage Corporation. Available online at: https://wsc.com.bs/ (accessed June 24, 2022).
Whitaker, F. F., and Smart, P. L. (1997). “Hydrogeology of the Bahamian archipelago,” in Geology and Hydrogeology of Carbonate Islands. Developments in Sedimentology Vol. 54, eds H. L. Vacher and T. Quinn (Elsevier), 183–216. doi: 10.1016/S0070-4571(04)80026-8
White, T., and Falkland, I. (2010). Management of freshwater lenses on small Pacific islands. Hydrogeol. J. 18, 227–246. doi: 10.1007/s10040-009-0525-0
World Bank (2022). Getting Electricity: Price of Electricity. World Bank. GovData360. Available online at: https://govdata360.worldbank.org/indicators/ (accessed June 25, 2022).
World Sea Temperature (2022). Nassau Water Temperature. Available online at: https://www.seatemperature.org/central-america/bahamas/nassau.htm#:~:text=The%20warmest%20water%20temperature%20is,%C2%B0F%20%2F%2023.3%C2%B0C (accessed June 28, 2022).
Wu, W-Y., Lo, M-H., Wada, Y., Famigletti, J. S., Reager, J. T., Yeh, P. J-F., et al. (2020). Divergent effects of climate change on future groundwater availability in key mid-latitude aquifers. Nat. Commun. 11:3710. doi: 10.1038/s41467-020-17581-y
Keywords: potable water, small islands, The Bahamas, Ocean Thermal Energy Conversion, freshwater lenses
Citation: Welsh K and Bowleg J (2022) Interventions and solutions for water supply on small islands: The case of New Providence, The Bahamas. Front. Water 4:983167. doi: 10.3389/frwa.2022.983167
Received: 30 June 2022; Accepted: 08 September 2022;
Published: 30 September 2022.
Edited by:
Raymond Mark Lee, Washington College, United StatesReviewed by:
Alban Kuriqi, Instituto Superior Técnico, Universidade de Lisboa, PortugalHitesh Panchal, Government Engineering College Patan, India
Luis Vega, Consultant, Kailua, United States
Copyright © 2022 Welsh and Bowleg. This is an open-access article distributed under the terms of the Creative Commons Attribution License (CC BY). The use, distribution or reproduction in other forums is permitted, provided the original author(s) and the copyright owner(s) are credited and that the original publication in this journal is cited, in accordance with accepted academic practice. No use, distribution or reproduction is permitted which does not comply with these terms.
*Correspondence: Kristen Welsh, a3Jpc3Rlbi51bndhbGFAdWIuZWR1LmJz