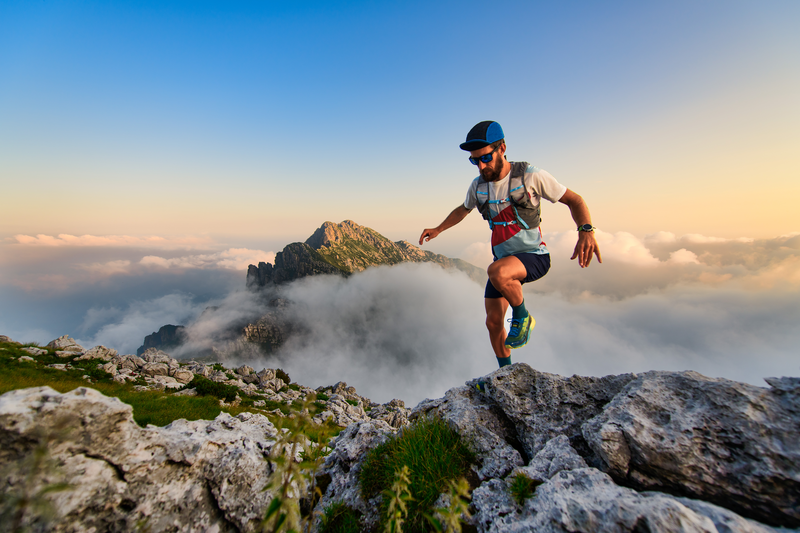
94% of researchers rate our articles as excellent or good
Learn more about the work of our research integrity team to safeguard the quality of each article we publish.
Find out more
ORIGINAL RESEARCH article
Front. Water , 13 October 2022
Sec. Water and Human Health
Volume 4 - 2022 | https://doi.org/10.3389/frwa.2022.959689
This article is part of the Research Topic Reducing Drinking Water Microbial Risks: Towards Bridging the Gap between Evidence and Action View all 8 articles
COVID-19 shutdowns drastically increased the frequency and duration of water stagnation events in building plumbing systems, urging local authorities to issue guidance for the safe reopening of buildings mostly by recommissioning flushing. The objectives of this study were to document the dynamic changes of bacterial indicators [adenosine triphosphate (ATP), total and intact cell counts (TCC, ICC)] and the prevalence of Legionella pneumophila (Lp) in 20–21 showerheads in a large building before (16-week building closure) and then shortly (24 h) and monthly (4-week of distal water stagnation) after targeted recommissioning flushing. Following the 16-week shutdown, the highest mean of ATP (10 pg ATP/mL), TCC (1.7 × 106 count/mL) and ICC (5.2 × 105 count/mL) were measured in first draw samples. This bacterial amplification was mostly attributable to detachment from biofilm present in the distal devices and immediate connecting piping. Culture-based (mean of 4 487 MPN/L) and quantitative polymerase chain reaction (qPCR; mean of 63 822 gu/L) concentrations of Lp were respectively measured in 81 and 90% of first draw samples. Individual flushing of showerheads for 5 min resulted in 1.2–278-fold decreases in ATP, whereas TCC and ICC were lowered by 1.1- and 0.7-log on average. A one-log reduction in culture-based and qPCR Lp was only achieved in 63 and 29% of paired water samples, resulting in less than one-log reduction in mean risk values per exposure, thus demonstrating the limited effects of fixture-flushing for risk reduction. Clear short-term (24 h) benefits of device recommissioning flushing included lowered values of all bacterial indicators and Lp levels systematically under the common alert threshold of 1 000 MPN/L in first draws. However, after a period of 1 month without water use, these benefits were mostly lost with considerable rebounds of concentrations to similar levels than those measured following the 16-week building closure. Results highlight the temporary benefits of device recommissioning flushing for the control of Lp in shower systems, especially in buildings colonized by Legionella.
In early 2020, multiple sanitary measures were put in place to limit the spread of the COVID-19 virus, including the closure of non-essential venues and services. Consequently, numerous buildings were left vacant or partially occupied, thus increasing the frequency and duration of water stagnation events in building plumbing systems or at specific outlets.
Among concerns about water quality deterioration over the course of stagnation, microbial growth is well documented. Short water stagnation times (< 24 h) typically result in large increases in ATP concentrations, total and intact cell counts, or heterotrophic plate counts (HPC; Lautenschlager et al., 2010; Zlatanović et al., 2017; Bédard et al., 2018; Peng et al., 2020; Rahmatika et al., 2022). This suggests that short stagnation events may favor culturability and that microorganisms tend to remain metabolically active. However, longer periods of water stagnation (up to 2 weeks) commonly show plateaued bacterial concentrations (Lautenschlager et al., 2010; Manuel et al., 2010; Zlatanović et al., 2017; Bédard et al., 2018; Peng et al., 2020). Indeed, prolonged stagnation is likely to result in depleted-nutrient environments that may limit long-term bacterial growth.
Considering that many waterborne pathogens can survive in oligotrophic environments characterized by low levels of nutrients (e.g., stagnant drinking water), health concerns were raised after the extended (in terms of months) closure of buildings during the COVID-19 pandemic. Due to increasing incidence rates of Legionellosis cases in the United States and elsewhere (NASEM, 2019), most recommendations recently issued to address the safe reopening of buildings after the COVID-19 pandemic shutdowns were focused on Legionella bacteria (AWWA, 2020; CSA, 2020; Government of Quebec, 2020; Proctor et al., 2020; CDC, 2021). More specifically, Legionella pneumophila is responsible for most Legionellosis cases (Fields et al., 2002), for which the risk of infection is most important when susceptible populations are exposed to high concentrations and the production of water aerosols (NASEM, 2019) in the respirable-size range of 1–10 μm in diameter (Allegra et al., 2016).
Routine flushing of taps to replace stagnant water with fresh water carrying an oxidant residual (cold water) or a high temperature (hot water) are identified as key control measures in several Legionella control regulations and guidelines, which are most often based on risk and type of population present in the building (Australian Government, 2015; NHS England, 2016; ESCMID, 2017; NASEM, 2019; ASHRAE, 2020; AWWA, 2020; VHA, 2021). Preventative flushing is also a common practice for the commissioning of new buildings and after the completion of construction work or in response to delayed occupancy. Flushing and disinfection are recommended for the commissioning of whole buildings or sectors of a new building after a period of more than 3 weeks (ASHRAE, 2018). ASHRAE Standard 188 also calls for preventative flushing if the occupancy of a new building sector is delayed after commissioning for a period of more than 2 weeks, but less than 4 weeks. Additionally, several guidance and standards warrant for the preventative flushing of low flow and stagnant areas in existing buildings, some setting a limit of 2 weeks for flushing after which disinfection must be (re)conducted (ASHRAE, 2018; CSA, 2020; Proctor et al., 2020).
Because of the widespread extreme water stagnation in buildings with no or low occupancy, a flurry of recommendations for the safe reopening of buildings was issued during the COVID-19 pandemic, with flushing being the most recommended corrective action (Proctor et al., 2020). The need to carry out recommissioning flushing was justified by water quality concerns (e.g., lead, copper, and Legionella), the duration of building shutdown, or the integrity of the entire building plumbing system (AWWA, 2020; CSA, 2020; Government of Quebec, 2020; Proctor et al., 2020; CDC, 2021). In all cases, buildings with vulnerable populations, such as healthcare facilities, and those with numerous aerosol producing devices (e.g., showers) were identified as high-risk priorities to investigate for the presence of Legionella pneumophila.
Evidence to support the definition of efficient remedial flushing (e.g., during recommissioning) practices is however limited, despite quite frequent prolonged shutdowns in buildings depending on their use (e.g., schools, seasonal venues). Individual flushing of cold-water taps for 3–6 min proved to be beneficial for reducing bacterial-ATP (cell-bound) concentrations by 6-fold on average (Lautenschlager et al., 2010), intact and total cell counts by more than 1-log (Bédard et al., 2018), and qPCR Legionella spp. by up to 2-log (Wang et al., 2012; Hozalski et al., 2020). Yet, there is a scarcity of data regarding the short- and long-term benefits of flushing toward Legionella pneumophila in building plumbing systems. Moreover, there is very scarce information on the water quality before the stagnation events, therefore limiting the extrapolation of findings after flushing.
The objectives of this study were to: (1) assess water quality of showers in a large sports complex following an extended period of stagnation during the COVID-19 pandemic shutdown (16-week), and (2) evaluate the short- (24 h) and long-term (4-week) effectiveness of targeted recommissioning flushing on general bacterial indicators and the occurrence of Legionella pneumophila. This study provides insights into the efficacy of flushing procedures which have been widely recommended as the first level of remedial action in most recommissioning flushing guidance recommendations following shutdown due to COVID-19 pandemic restrictions.
A five-story sports complex built in 1976 (Canada) was selected for this study given its large number of showers (n = 114). The building plumbing system was supplied at one point of entry by municipal chlorinated water (0.41–0.64 mg Cl2/L). The hot water system included four hot water tanks (30–58°C) in series delivering hot water through a single central recirculating line. The prevalence of Legionella pneumophila in the municipally distributed water was previously found to be very low with rare positives detectable only in concentrated large volumes (Prévost et al., 2019).
The building was closed to the public from March 13 to July 6, 2020, due to COVID-19 pandemic restrictions orders. After this, normal summer activities (day camps) resumed on a daily basis, although the access to showers remained closed. Most showers sampled were part of a large grouped shower system in which one single bimetallic strip thermostatic mixing valve (TMV) supplies mitigated water to 20–22 showerheads (Supplementary Figures S1A,B). These showerheads were manually activated by a pressure-button with each activation resulting in a flow lasting 10 s−1 min. A few other showerheads sampled were either manually activated by a pressure-button or with a user-adjusted TMV and received mitigated water by a single TMV supplying less than four showerheads at once (Supplementary Figures S1C–E).
None of the pre-set TMV could be bypassed nor set differently during the study period. Pipe materials were not systematically identified as building plumbing was not easily accessible, except for large grouped shower systems in which copper was found from the TMW to each timer valves, and flexible plastic hoses from the latter to each showerhead.
Sampling was first performed on July 2, 2020, to assess the water quality following a 16-week period of low building occupancy (< 1% of occupancy) at 21 selected showerheads distributed over seven locker rooms across the building (Supplementary Figure S1). On July 14, 2020, all showerheads (n = 114) connected to the building plumbing system, including the ones investigated in this study, were successively flushed for 5 min each with mitigated water (25–39°C) as part of the recommissioning flushing program. No systemic flushing of the cold and hot water systems by opening all water points of use in the building was conducted although recommended by several recent guidance documents (AWWA, 2020; CSA, 2020; Government of Quebec, 2020; Proctor et al., 2020; CDC, 2021). Therefore, the recommissioning program conducted should be viewed as partial device recommissioning targeted at high-risk areas only (showers). It is believed that few building managers have thoroughly conducted complete flushing of buildings after extended closures, partially because of the important resources to conduct full flushing, the lack of available information on the water systems or other considerations such as water conservation. Sampling was conducted again on the next day to evaluate the water quality within a short (24 h) period of time after targeted recommissioning flushing. Showers then remained unused despite the reopening of the building for day camps purposes. A third sampling event was carried out on August 19, 2020, to assess the long-term (4 weeks) impacts of targeted recommissioning flushing with no regular water use at showers. One showerhead from locker room E (Supplementary Figure S1C) could not be accessed for the last sampling event. All showers investigated remained untouched throughout the whole study period, with the exception of sampling days, hence the term distal water stagnation used in this present study.
In a subset of two showerheads, profile sampling was conducted over the course of the following year from the third recommissioning sampling event (August 19, 2020), without shower usage in the meantime, to measure total and intact bacterial cell counts after respective periods of distal water stagnation of 2 and 5 months. Profile flushing duration was based on the mitigated water volume between the outlet and the TMV (6 L) in the small grouped shower system (Supplementary Figure S1E) and on the volume between the outlet and the hot water recirculation line (250 L) in the large grouped shower system (Supplementary Figure S1A). Profile sampling was repeated after a successive 1 h distal water stagnation.
For each large grouped shower system, sampling was performed by starting with the showerhead closest to the TMV and moving to the farthest. First draw samples (1 L) and 5 min flushed samples (1 L) were collected using sterile plastic bags attached to each showerhead so that exposure and generation of aerosols were reduced during sampling. A volume averaging 250 mL was drawn after each sample collection into a plastic beaker for onsite physico-chemical measurements (data not shown). Whenever free chlorine concentrations exceeded 0.05 mg/L, one mL of sodium thiosulfate (10% v: v) was added to the sample.
In the field, an aliquot of 100 mL was transferred from each well-mixed one-liter sample to a sterile polypropylene container and kept at ambient temperature until analyzed in the laboratory. Within 12 h of sampling, aliquots were analyzed for the detection and quantification of L. pneumophila by culture-based enzymatic method with the 100 mL potable water Legiolert/Quanti-Tray kit (IDEXX Laboratories Canada Corp., Markham, Ontario, Canada) and incubated at 39 ± 0.5°C for 7 days. Results were latterly determined by counting positive wells and expressed as most probable number (MPN) per liter concentrations (10–22 726 MPN/L).
Additional 50 mL aliquots were transferred from each well-mixed one-liter sample to distinct sterile polypropylene containers and kept on ice until transported to the laboratory. The protocol followed and the materials used were those provided by the manufacturer of the Dendridiag® SW kit (GL-Biocontrol, Cal Alpha, France), which has been specifically designed for bacterial-ATP quantification in drinking water. Water samples were filtered on 0.45 μm (Ø 33 mm) PES sterile membranes (CLEARLine® Biosigma S.p.A, via Valletta, Italy) to eliminate free ATP or other inhibitors that could interfere with bacterial-ATP detection (Hammes et al., 2008; Arroyo et al., 2017; Van Nevel et al., 2017). ATP was then extracted with a solution for cell lysis and immediately quantified through bioluminescence assay with the Kikkoman PD-30 Lumitester™ apparatus (Kikkoman Corp., Noda, Japan). The kit had a detection limit of 0.1 pg ATP/mL, and samples were processed within 12 h of sampling.
Within 24 h of sampling, aliquots of approximatively 5 mL per sample were set aside in sterile tubes to assessed total and intact bacterial cell counts through flow cytometry on the BD AccuriTM C6 Plus Flow cytometer, along with the automatic sampling BD CSamplerTM arm (BD-Biosciences, Mississauga, ON, Canada). Flow cytometry assays were performed according to Cazals et al. (2022). A volume ratio of water sample to reagent of 300:3 μL was used for co-staining samples firstly with the SYBR® Green I fluorochrome to discriminate all bacterial cells, then with propidium iodide (PI) which stains only dead cells. The FL1 (533 nm) green fluorescence and FL3 (> 670 nm) red fluorescence density plots were selected for the enumeration of total and intact cell counts. The EAWAG water quality software analysis template for damaged and intact bacteria gating in drinking water samples was adopted for all water samples to ensure reproducibility and comparison among results (Gatza et al., 2013). Viability of bacterial cells was therefore based on the integrity of cell membranes.
Remaining contents (600–800 mL) of bulk water samples were filtered on sterile 0.2 μm (Ø 47 mm) Supor® PES membranes (PALL Corp., Mississauga, ON, Canada) upon reception. Membranes were gently folded upon themselves and placed into sterile tubes for conservation at −80°C. DNA was then extracted using the bead beating and centrifugation method adapted from Yu and Mohn (1999). For each sample, 1 mL of an extraction buffer (50 mM Tris-HCl, 5 mM EDTA, 3% SDS, and RNase 20 μg/mL) was added to a FastPrepTM Lysing Matrix A tube containing the thawed membrane and agitated (6 m/s, 40 s) in the FastPrep-24 bead beater (MP Biomedicals, Solon, OH, USA) over two successive cycles, after which tubes were centrifuged for 5 min at 13 200 rpm. DNA purification was performed through impurities precipitation with ammonium acetate (100–300 μL) and DNA precipitation with isopropanol (100–750 μL) and glycogen (5 μL, 0.02% v: v), followed by serial ethanol washes (1 mL 70% ethanol). DNA was finally resuspended in 100 μL sterile qPCR grade water (Fisher Bioreagents, Waltham, MA, USA).
Quantification of L. pneumophila by real-time qPCR was performed in duplicates using the iQ-Check® Quanti L. pneumophila Real-Time PCR kit (Bio-Rad Laboratories, Mississauga, ON, Canada). Inhibition testing and standard curve efficiency were assessed according to the manufacturer's recommendations. Fluorescence signals were recovered on the Rotor-Gene Q instrument (QIAGEN Sciences Inc., Germantown, MD, USA), and expressed into gene units (gu) per liter. No inhibition was detected, and qPCR efficiencies and correlation coefficients (R2) respectively ranged 86–121% and 0.99003–0.99818.
Risk characterization of both Legionellosis health outcomes (Pontiac fever – Sub-clinical infection and Legionnaires' disease – Clinical severity infection) was performed through quantitative microbial risk assessment (QMRA) using mean culture-based L. pneumophila concentrations as point estimates for first draw and flushed samples. Exposure assessment to L. pneumophila was adapted from Hamilton et al. (2019) to the specifics of the investigated building. A breathing rate associated to a moderate activity (USEPA, 2011) was considered since users typically take a shower after practicing physical activities, as well as a shower duration of 6.8 min represented by a right-skewed lognormal distribution (Wilkes et al., 2005) and a value of one shower per day were used. Dose-response models for conventional (> 13 lpm) and low-flow (< 7 lpm) showerheads developed by Hamilton et al. (2019) were used accordingly to the R script code published by Hozalski et al. (2020). Finally, a disability-adjusted-life year (DALY) burden per Legionnaires' disease infection of 0.97 (0.90–1.05, uniform distribution; Van Lier et al., 2016) was also selected for the dose-response model.
Normality of distributions of microbiological water quality indicators was first assessed generating histograms. Given that variables were all non-parametric, the Wilcoxon test was used to assess statistical differences between paired first draw and flushed samples, as well as between sampling events. For statistical purposes, any sample with a culture-based or a qPCR L. pneumophila concentration outside the detection limits of each method was respectively set at 5 or 30 000 MPN/L, or at 10 gu/L. Correlation among variables was evaluated through Spearman's rank correlation test by considering separately first draws and flushed samples due to the flushing intervention.
Significance level was set at a p-value of 0.05 unless stated otherwise and all analysis and graphs (except for line graphs which were produced on Microsoft Excel version 16.59) were performed on RStudio version 4.1.1 (2021.09.0).
Overall, 124 water samples from showerheads located in a large sports complex were analyzed for general bacterial indicators and L. pneumophila. First draw and 5 min flush samples were collected after a 16-week building shutdown, shortly (24 h) following targeted recommissioning flushing, and 4-week after the former intervention without water use in between (distal water stagnation). Summary statistics are available as Supplementary Table S1.
Measured bacterial indicators followed similar trends during the study. The highest mean bacterial-ATP (10.0 pg ATP/mL; Figure 1A), total cell counts (1.7 × 106 count/mL; Figure 1B) and intact cell counts (5.2 × 105 count/mL; Figure 1C) were measured in first draws (n = 21) after the prolonged 16-week building closure (Figure 1). These indicators respectively reached values as high as 24.2 pg ATP/mL, 3.8 × 106 count/mL, and 1.1 × 106 count/mL (Supplementary Table S1). Targeted recommissioning flushing resulted in significant (p < 0.001) reductions in these microbiological concentrations in first draw samples collected 24 h after flushing, and maximum values thereby measured remained below previously recorded mean. However, concentrations generally increased back during the monthly distal water stagnation period, indicating that detachment or regrowth of bacteria likely occurred near the points of use. Viability percentages in first draw samples ranged from 5 to 54% (Figure 1D).
Figure 1. Box plot representations of (A) bacterial-ATP, (B) total cell counts, (C) intact cell counts, and (D) viability ratios, per sampling period. Gray bars – First draw samples (n = 20–21); White bars – Five-min flushed samples (n = 20 – 21); Horizontal black line – Median; Red cross – Mean; Boxes – 25–75th percentiles; Whiskers – Minimal and maximal values; Black dots – Raw data.
Throughout the study, flushed samples (n = 62) showed significantly lower (p < 0.01) bacterial indicators concentrations than their first draws counterparts, although differences were slightly less marked 24 h after the recommissioning intervention. Globally, bacterial-ATP were reduced by 1.2–278-fold in flushed samples, whereas viability ratios were reduced by 0.5–48%. In terms of total and intact bacterial cell numbers, flushed samples were on average (per sampling event) 0.5–0.9-log and 0.7–1.4-log lower than first draws.
In order to investigate the relative contribution of growth during extended stagnation and detachment from biofilm, two showerheads from one small grouped shower system (Supplementary Figure S1E) and one large grouped shower system (Supplementary Figure S1A) were sampled again after respective periods of distal water stagnation of 2–5 months. Profile sampling of mitigated water collected from both showerheads after prolonged distal water stagnation (Figures 2A,B) showed that total and intact bacterial cell counts were of the same orders of magnitude (105–106 count/mL) in the first liter of collected water than what was measured after the 16-week building closure (Figures 1B,C). Flushing the mitigated water volume of 6 liters between the showerhead and the TMV in the small grouped shower system resulted in total and intact cell numbers reduced by 0.7- and 0.8-log (Figure 2A). In the large grouped shower system, total and intact cell numbers were more markedly reduced, respectively by 1.7- and 2.7-log (Figure 2B), as water was flushed until reaching the hot water recirculation line (250 liters). Following a 1 h period of distal water stagnation, both total and intact cell counts measured in the first few liters of water from each showerhead remained at similar levels than those found in the last flushed sample collected 1 h before. However, as sample collection proceeded, a gradual increase in total and intact cell counts was observed in the showerhead from the small grouped shower system (Figure 2A), as well as incidental increases in the showerhead from the large grouped shower system (Figure 2B). This was likely due to higher levels of bacterial indicators found in the upstream plumbing system (i.e., upstream the TMV) or repeated shear forces induced by sample collection.
Figure 2. Total (TCC) and intact cell counts (ICC) profiles over cumulated flushing water volumes in two showerheads from a (A) small grouped shower system and a (B) large grouped shower system.
Throughout the study period, highest mean culture-based (Figure 3A) and qPCR L. pneumophila concentrations (Figure 3B) were measured in both first draw (Legiolert culture: 4 487 MPN/L; qPCR: 63 822 gu/L) and flushed samples (Legiolert culture: 1 622 MPN/L; qPCR: 3 461 gu/L) following the prolonged 16-week building closure. Culture-based L. pneumophila concentrations spanned over the complete range of the culture-based enzymatic method used (< 10 MPN/L to more than 22 726 MPN/L), whereas qPCR concentrations spiked up to more than 106 gu/L. Conversely, lowest mean values were detected 24 h after targeted recommissioning flushing in first draw samples (Legiolert culture: 100 MPN/L; qPCR: 6 839 gu/L) and flushed samples (Legiolert culture: 23 MPN/L; qPCR: 687 gu/L). After the monthly distal water stagnation, mean concentrations bounced back to comparable levels than those found after the 16-week shutdown in both first draw samples (Legiolert culture: 2 368 MPN/L; qPCR: 47 007 gu/L) and flushed samples (Legiolert culture: 450 MPN/L; qPCR: 3 158 gu/L).
Figure 3. Box plot representations of (A) culture-based Legionella pneumophila and (B) qPCR Legionella pneumophila, per sampling period. Gray bars – First draw samples (n = 20–21); White bars – Five-min flushed samples (n = 20–21); Horizontal black line – Median; Red cross – Mean; Boxes – 25–75th percentiles; Whiskers – Minimal and maximal values; Black dots – Raw data; Horizontal dotted lines – Limit of detection (LoD) or limit of quantification (LoQ). Any sample with a culturable or a qPCR L. pneumophila concentration outside the detection limits of each method was respectively set at 5 or 30 000 MPN/L, or at 10 gu/L.
Five water samples (out of 124) had a higher culture-based concentration of L. pneumophila than its qPCR counterpart, as likewise reported by Dowdell et al. (2022; manuscript submitted to Environmental Science and Technology). This was likely due to DNA losses during the extraction phase or poorly mixed split water volumes. Nonetheless, specifically for first draw and flushed samples, qPCR concentrations were on average (per sampling event) 0.2–4.0-log and 0.4–3.4-log higher than culture-based results. However, mean log differences between the two L. pneumophila quantification methods used were generally larger in flushed samples, probably due to a greater proportion of dead or viable-but-non-cultivable L. pneumophila cells upstream in the hot water system. Linear regression analysis with first draw samples (n = 62) further yielded a stronger correlation coefficient (R2 = 0.64) than for flushed samples (n = 62; R2 = 0.51; Figure 4).
Figure 4. Scatterplot of first draw (red points) and five-min flushed samples (blue points) according to their culture-based (MPN/L) and qPCR (gu/L) L. pneumophila concentrations. Red or blue lines – Linear regression with associated correlation coefficient (R2).
Overall, individual flushing of showers for 5 min with mitigated water resulted in average log reductions (per sampling event) of 0.4–1.1 and 0.4–0.6 respectively for culture-based (Figure 3A) and qPCR L. pneumophila concentrations (Figure 3B). Considering paired samples, mean reductions in the concentration between first draw and flushed samples were always significant (p < 0.05) over the study period, with the exception of qPCR results measured during the last sampling event (p = 0.07). Contrary to expectations, increases ranging 0.1–1.8-log in culture-based L. pneumophila concentrations were observed in a subset of paired water samples (n = 7 out of 62) in different showerheads. In four of these, a comparable increase ranging 0.3–1.4-log in qPCR L. pneumophila concentrations was seen, whereas decreasing qPCR concentrations were measured for the others.
Following the 16-week building closure, 81% (17/21) of showerheads were positive (> 10 MPN/L) for culture-based L. pneumophila in first draw samples (Table 1). Comparably, 90% (19/21) of showerheads had detectable L. pneumophila DNA (qPCR) in first draw samples. This proportion slightly increased to 95% (20/21) in flushed samples. The targeted recommissioning flushing intervention then lowered the proportion of positive culture-based L. pneumophila samples to 48% (10/21) in first draw samples, and to an even lower proportion (24%, 5/21) after a brief 5 min flush. Culture-based concentrations of all water samples also remained under 1 000 MPN/L. However, 90% (19/21) and 86% (18/21) of first draw and flushed samples still yielded detectable L. pneumophila DNA through qPCR. On the last sampling event, after a period of distal water stagnation of 4-week following the targeted recommissioning flushing, L. pneumophila DNA through qPCR was detectable in all water samples. The proportion of positive samples to culture-based L. pneumophila increased accordingly to 85%, although the fraction of first draw samples over 1 000 MPN/L (25%) observed was less than after the initial 16-week building closure (48%; Table 1).
Table 1. Proportion of positive samples to culturable Legionella pneumophila (MPN/L) categorized in common sub-categories for drinking water.
Two types of shower systems were investigated, including large grouped shower systems in which multiple (n > 20) showerheads were supplied with mitigated water from a single TMV (Supplementary Figures S1A,B), and small grouped shower systems in which a small number of showerheads (n < 4) were fed by a single TMV (Supplementary Figures S1C–E). These two shower systems differed for the most part by the total volume of mitigated water found between the TMV and each showerhead. This volume was approximatively of 300 liters of mitigated water in the large grouped shower systems, whereas it was less than 6 liters in the smaller ones.
Following the prolonged 16-week building closure, sampled showerheads associated with large grouped shower systems (n = 13) showed similar mean culture-based L. pneumophila concentrations and total and intact cell numbers than showerheads associated with small grouped shower systems (n = 6). However, significantly higher (p < 0.05) bacterial-ATP and qPCR L. pneumophila concentrations in first draw samples were measured in showerheads from large grouped shower systems. Targeted recommissioning flushing appeared to bring more benefits in first draw samples collected from the large grouped shower systems as lower concentrations of all microbiological measurements were indeed measured, with the exception of L. pneumophila through qPCR. This may be attributable to the larger volume of water moved by the flushing of multiple showerheads at once, resulting in a greater turnover of water than in small grouped shower systems, which were oddly located further from the hot water recirculation line.
Through Spearman's rank test, bacterial-ATP was found to have a strong and significant (p < 0.001) positive relationship with intact cell counts in first draw samples (rho = 0.8, n = 62; Supplementary Figure S2A), but a weak (rho = 0.32, n = 62), yet significant (p < 0.05), correlation in flushed ones (Supplementary Figure S2B). This last observation could be attributed to the reduced bacterial-ATP sensitivity and higher variability commonly reported at lower cell concentrations (Hammes et al., 2010), given that flushed samples generally exhibited lower cell densities. Indeed, flushed samples generally showed low bacterial-ATP (< 1 pg ATP/mL), but values spanned over 3 orders of magnitude in intact cell counts (103–106 count/mL). Hence, ATP-per-cell values, represented by the amount of bacterial-ATP over the number of cells with an intact membrane (Hammes et al., 2010; Lautenschlager et al., 2010), could be evaluated adequately at least in first draws. Despite higher bacterial-ATP and intact cell counts predominantly measured in first draw samples after the 16-week building closure and the monthly distal water stagnation period, ATP-per-cell ratios did not follow the same trends observed for the other bacterial indicators (Supplementary Table S1). Mean values in first draw samples did not change significantly (p = 0.34–0.96) across the sampling events. Nonetheless, values calculated for flushed samples were significantly (p < 0.05) lower.
A significant (p < 0.05) but weak correlation was observed between the proportion of intact cell counts (viability ratios) and culture-based concentrations of L. pneumophila in first draw samples (rho = 0.25, n = 62; Supplementary Figure S2A) and in flushed samples (rho = 0.31, n = 62; Supplementary Figure S2B). Therefore, culture-based concentrations were classified in dichotomous variables (below or higher than 1 000 MPN/L) to evaluate differences in viability ratios among sample types. Statistically different (p < 0.05) mean of the fraction of intact cells between water samples below 1 000 MPN/L (first draw samples: 25%; flushed samples: 13%) or above 1 000 MPN/L (first draw samples: 38%; flushed samples: 32%) were found (Supplementary Figure S3). Finally, despite a weak (rho = 0.34) but significant (p < 0.01) correlation between culture-based and qPCR L. pneumophila concentrations in first draw samples (Supplementary Figure S2A), no such outcome was observed in the set of flushed samples.
Mean of per exposure risk and DALY values calculated with culture-based concentrations of L. pneumophila through the QMRA approach are available in Supplementary Table S2. Overall, reported values displayed similar trends than L. pneumophila concentrations. Throughout the study, individual flushing of showerheads for 5 min only resulted in less than one log decreases in per exposure risk and DALY values for both Legionellosis health outcomes considered. For example, following the 16-week building shutdown, per exposure mean risk values for first draw samples considering a Pontiac fever endpoint were respectively of 1.4 × 10−2 and 2.6 × 10−3 for conventional (> 13 lpm) and low-flow (< 7 Lpm) showerheads, whereas these values were reduced to 4.1 × 10−3 and 7.8 × 10−4 after a brief 5 min flush. Rather considering a Legionnaires' disease endpoint, per exposure mean risk values for first draw samples were of 1.4 × 10−5 and 2.6 × 10−6 for conventional and low-flow showerheads, and were subsequently lowered to 4.7 × 10−6 and 8.8 × 10−7 (Supplementary Table S2).
A prolonged building shutdown (16-week) resulted in elevated concentrations of microbial indicators in first draw and flushed samples, indicative of significant water quality losses both in distal points of use (showerheads) and upstream in the building plumbing system.
Values of bacterial-ATP ranging from 0.7 to 24.2 pg ATP/mL (Figure 1A) were measured in first draw samples, exceeding previously reported values for potable water in the built environment in Europe (Lautenschlager et al., 2010; Duda et al., 2015; Zlatanović et al., 2017). The wide variations in measured values could further be evocative of the detection of different bacterial profiles, as ATP produced by cells vary across species, or conditions of growth (Zhang et al., 2019). Although ATP has not been correlated to increased health risks, nor it can predict accurately the presence of opportunistic pathogens (Duda et al., 2015), a cellular concentration over 10 pg ATP/mL is often suggested as a threshold for the implementation of corrective actions in some commercial kits. Nonetheless, the detection of high amounts of bacterial-ATP following the extended 16-week building shutdown reflects important bacterial activity or detachment from the biofilm, whereas the upsurge observed after the recommissioning intervention indicates possible bacterial regrowth.
Similarly, mean total and intact bacterial cell counts respectively reached 1.7 × 106 count/mL (Figure 1B) and 5.2 × 105 count/mL (Figure 1C) in first draw samples after the 16-week building closure and bounced back to 7.2 × 105 count/mL and 2.4 × 105 count/mL at the end of the monthly period of distal water stagnation which followed the targeted recommissioning flushing. Overall, these cell densities in first draw samples after long periods of no use in the distal parts of the building plumbing system largely exceed formerly published values after shorter stagnation times (Lipphaus et al., 2014; Zlatanović et al., 2017; Montagnino et al., 2022; Rahmatika et al., 2022). However, they are consistent with values measured in cold and hot water at taps after varying lengths of stagnation in buildings located in the same water distribution system than the sports complex sampled in the present study (Bédard et al., 2018; Dias et al., 2019). Observations from this study also appear consistent, yet with slightly higher values of total cell counts, than those measured after a 7-week shutdown in Swiss research buildings during the COVID-19 pandemic (Rhoads et al., 2022). Mean values for the proportion of intact cells (Figure 1D) were in agreement with prior observations of first draw samples in chlorinated water containing 15–41% of viable cells after 24 h to 10 days of distal water stagnation (Bédard et al., 2018).
Surprisingly, results of ATP-per-cell (Supplementary Table S1) in first draws after long periods of distal water stagnation were in the same order of magnitude than those calculated in previous studies for shorter stagnation times (< 2 days; Siebel et al., 2008; Lautenschlager et al., 2010). The use of such ratio is beneficial to account for the heterogeneity of ATP concentrations produced by different bacterial species, or in contrasting growth conditions (e.g., starvation vs. exponential growth phase; Hammes et al., 2010). Indeed, starved bacterial cells are likely to have ATP-per-cell values considerably lower as a consequence of reduced metabolic activity (Hammes et al., 2010), which was not observed in this study. These differences could also reflect higher nutrient loadings in the water source feeding the studied building, although dissolved organic carbon (DOC) consumption across the distribution system rarely exceed 0.1 mg C/L (Dias et al., 2017).
A weakness of most, if not all studies conducted during the COVID-19 pandemic, is the lack of baseline data collected during normal building operations before extended stagnation occurred. Also, the information on how microbial quality changes progressed during the building shutdown period is limited. Although it cannot be inferred that increasing stagnation duration will systematically exacerbate distal bacterial amplification, results from this study and report from Bédard et al. (2018) suggest that extended stagnation from one-hour to 4 months can lead to very high levels of total and intact cell counts at the point of use and its immediate upflow plumbing.
The observed distal amplification of bacteria in first draw samples collected after the prolonged 16-week building closure, and to a lesser extent after the monthly period of non-water use, can simultaneously be the result of bacterial growth and detachment from the adjacent biofilm. When flow resumes after stagnant conditions, it exerts important shear forces at the biofilm-water interface (Paul et al., 2012). Consequently, weakly embedded bacteria or biofilm pieces can be dislodged and dispersed into the bulk water phase through a sloughing phenomenon (Stoodley et al., 2001). Peng et al. (2020) demonstrated that short stagnation times (< 6 h) resulted in increases in free-floating bacteria (planktonic growth), whereas biofilm-attached bacteria contributed more to growth in stagnant water during longer stagnation times (12–24 h). Following long periods of distal water stagnation, detached bacteria will accumulate in the bulk water and be washed out, thus likely resulting in larger amounts of biomass in water samples. As shown by Bédard et al. (2018), an increase in total cell counts and culturable bacteria (HPC) after stagnation periods ranging 2–10 days was mostly associated with detachment, reflecting the high surface to volume ratio in the distal point of use and immediate connecting pipe. The interplay between growth and flow induced detachment is also shown by the smaller amplification in the first distal volume after a shorter stagnation of 1 h (Bédard et al., 2018). In a medium-sized building, a tap unused for several months showed total bacterial cell counts more than 1-log higher in the first liter of water than in taps more frequently used (Lipphaus et al., 2014).
To assess whether the elevated concentrations of bacterial indicators were mostly due to detachment from the biofilm, two showerheads from this study were sampled (profile sampling) after periods of distal water stagnation of 2 and 5 months. Typically, meaningful bacterial growth is unlikely to be significant in less than 1 h of stagnation (Lautenschlager et al., 2010). This was demonstrated in both showerheads by similar intact cell numbers measured between the last collected flushed sample after extended periods of no use and the first sample collected in the subsequent short stagnation time (Figures 2A,B). In profiling results, the differences between bacterial cell counts in the first liter of water and the last sample collected can indicate if detachment occurred. Indeed, results showed much larger differences, mirrored by rapid decreases in total and intact cell counts, in the first liter of water collected following the two- and five-month distal water stagnation periods than after the 1 h periods of no use (Figures 2A,B). This suggests that long periods of stagnation can increase bacterial detachment from biofilms present in distal devices. Additionally, a greater turnover of water through flushing (250 liters), as performed in the showerhead from one large grouped shower system (Supplementary Figure S1A), was more beneficial at reducing total and intact cell counts (Figure 2B) than profiled flushing only until the TMV (6 liters; Figure 2A).
The high positivity rates of L. pneumophila by culture-based method and qPCR results (Table 1) recovered in first draw and flushed samples indicate a broad and systemic contamination of the building hot and tempered water systems. Whereas some studies carried out during the pandemic reported either no detectable L. pneumophila (Hozalski et al., 2020) or low positivity and concentrations of L. pneumophila (Dowdell et al., 2022, submitted to Environmental Science and Technology), others measured quantifiable concentrations in similar ranges as those observed in this study for samples representative of points of use (101–104 MPN/L or CFU/L; Giglio et al., 2020; Rhoads et al., 2022).
Typically, starved monocultured Legionella bacteria show complete loss of culturability within a few weeks (Al-Bana et al., 2014; Schrammel et al., 2018). However, the detection of culture-based L. pneumophila in showerheads which had not been used for 9 months (3 out of 21; data not shown) at levels comparable to those in showerheads unused only during the 16-week building shutdown suggests that conditions remained favorable for growth despite the probable depletion of nutrients. The establishment of a suitable microcosm in which L. pneumophila can seek nutrients through protozoal infection (Fields et al., 2002) could have contributed to its extended culturability in spite of environmental stressors, such as stagnant conditions. These results bring evidence that L. pneumophila can remain culturable for a prolonged period of time in stagnant water at low temperatures (23–25°C) with nutrient limitations.
Alert and action thresholds for the detection of L. pneumophila almost all rely on culture-based methods as an epidemiological link has been established between culture-based concentrations and infection and disease (NASEM, 2019). Thresholds proposed vary significantly across control regulations and guidelines, and many are set to protect vulnerable people in the building in addition to considering the potential for aerosolization exposure. For public buildings serving a general population, a threshold of 10 000 CFU/L is suggested by the European Technical Guidelines for the prevention, control and investigation of infections caused by Legionella species (ESCMID, 2017). In contrast, for healthcare-associated settings, a lower action threshold of 1 000 CFU/L is recommended by the Veterans Health Administration (VHA) in the United States. More recently, the National Academies of Sciences, Engineering, and Medicine (NASEM) reviewed existing evidence and supported the definition of concentrations corresponding to an acceptable risk of infection and disease.
Using a reverse QMRA approach, Hamilton et al. (2019) calculated a risk-based concentration of 14.4 CFU/L and 1 400 CFU/L for a shower exposition, respectively for per exposure risk of 10−6 DALY and 10−4 annual infection benchmarks. Typically, the acceptable burden for both Legionellosis outcomes (Pontiac fever or Legionnaires' disease) strongly depends on which risk benchmark is selected. For example, in this study, per exposure risk values calculated for first draw samples collected after either the 16-week building closure or the 4-week distal water stagnation were on average above the common risk target of 10−4 for a Pontiac fever endpoint, and above the more stringent risk target of 10−6 DALY for a Legionnaires' disease endpoint (Supplementary Table S2). However, flushing these showerheads for 5 min did not result in sufficient risk reductions as per exposure mean risk values remained above the previous benchmarks.
According to the employees of the sports complex sampled, almost half of their clientele is composed of elderly, thus susceptible people to Legionella infection. Although a gap remains between observed health outcomes and results from environmental monitoring of L. pneumophila (culture-based or qPCR-oriented), the action limit for such building, and more particularly for showers which result in greater aerosolization, should be reconsidered. Hence, considering that one CFU is the near equivalent of one MPN in potable water samples according to previous studies (Sartory et al., 2017; Spies et al., 2018; Boczek et al., 2021), the detection of more than 30% of first draws above culture-based L. pneumophila concentrations of 1 000 MPN/L fully justifies the flushing recommissioning intervention.
The high prevalence in the showers sampled strongly suggests that L. pneumophila had already colonized the building plumbing system prior to the period of no water use, although the extent of the contamination cannot be established. Most of the showers had not been used for 16 weeks, but some of the showers sampled were only used seasonally and had not been used for at least 9 months.
To identify the cause of the high prevalence of L. pneumophila observed in this building, several investigations were conducted. As proposed by Bédard et al. (2015), this investigation should first establish whether the main hot water system composed of the water heaters, reservoirs and recirculation loops were adequately operated to control L. pneumophila during the pandemic period. If the main distribution system is not operated to control L. pneumophila reliably, it will seed the secondary piping and distal sites and lead to widespread occurrence of the pathogen in distal points of use such as showerheads. In this study, low levels of culture-based L. pneumophila (20–65 MPN/L) were detected in the hot recirculation line, confirming the presence of sub-optimal conditions to control the pathogen.
The characterization of the centralized hot water system through sampling and temperature monitoring revealed numerous design and operational issues that could contribute to the presence of L. pneumophila in the main hot water system. First, of the four water heaters in series, the penultimate from the hot water being supply to the building was turned off without first isolating it (e.g., through closure of isolation valves) from the others. The water heater was then operated at 30°C for months before, during, and after the study period, hence providing a water environment that could have acted as a L. pneumophila nursery, although not tested due to a lack of available sampling ports. Maintaining a temperature above 60°C, as recommended in the Construction Code of Quebec (Building Act, chapter B-1.1, r. 2) and elsewhere (NASEM, 2019), is a beneficial control measure to prevent the growth of L. pneumophila (Bédard et al., 2016a; Cazals et al., 2022). Secondly, the water heaters feed flow was modified recently to improve energy efficiency. To do so, part of the recirculated hot water returning to the first water heater of the series was rather blended with the hot water coming out from the last heater of the series. These modifications resulted in hot water supplied to the building at 53–55°C, despite the hot water leaving the last heater at a consistent 60°C. In turn, the temperature of the hot water returning to the series of heaters was lowered to 48–51°C, thus likely allowing the survival of L. pneumophila. Moreover, episodic drops in temperature down to 25°C were observed in a small plate heat exchanger which preheated a fraction of the hot water returning back to the first heater of the series. High surface area and favorable temperatures measured in the plate heat exchanger may have further promoted the growth and dissemination of L. pneumophila (Bédard et al., 2016b). It is interesting to note that a similar design of mixing contaminated recirculation water with cold water inflow to a heat exchanger was previously shown to cause a Legionnaire's disease outbreak in a hospital (Bédard et al., 2016b).
Thirdly, the general configuration of the hot water system consisted of a central hot water recirculation loop feeding secondary piping that provided water to locker rooms with showers across the building. Because of this configuration, unrecirculated secondary connecting piping constituted extensive dead water volumes of hot water between the central hot water recirculation loop and the TMV of the shower systems. This dead volume was estimated to reach up to 300 liters in some locker rooms. Typically, the time required for a given point of use to reach the hot water temperature of the recirculation line should be less than 60 s, and preferably under 30 s (Singh et al., 2022). However, in the most distant large grouped shower system from the hot water recirculation line, it took 30 min for the hot water to get to the TMV when using only one showerhead at a time. Such prolonged elapsed time was likely due to imbalanced hydraulics, or the long pipe lengths and large diameter (2 ½ inches) of the hot water pipe supplying the TMV, which was designed for simultaneous use of showerheads in the large grouped shower systems.
Finally, considerable volumes averaging 200–300 liters of mitigated water (25–40°C), were measured in the large grouped shower systems between the TMV and its supplied distal showerheads, thus providing favorable conditions for L. pneumophila growth (Sharaby et al., 2017). According to several thermostatic mixing valve manufacturers recommendations, this volume should be minimized so that a maximum volume of 3 liters or an elapsed time of 30 s are found between the TMV and the farthest outlet. In addition, manufacturers also recommend monitoring and disinfecting such grouped shower systems with hot water (60–70°C) on a routine basis.
When considering the operational and design weaknesses documented, it was not surprising to find the high prevalence of L. pneumophila in the shower systems investigated. Apart from operational issues with the water heaters and recirculation water mixing, the building studied reflected an older design with little or no consideration to the limitation of volumes of unrecirculated hot water and tempered water. These conditions are likely to be present in many legacy buildings. Standards and plumbing codes related to grouped shower systems should be carefully reviewed in order to prevent these conditions highly favorable to Legionella growth. Additional research is needed regarding preventive control measures in large grouped shower systems, such as the installation of auto-flush valves situated at their rear-end to allow more frequent renewal of water.
In the context of environmental routine monitoring, which is a central part of water safety management plans, the use of quick and accurate surrogate parameters to predict the presence of L. pneumophila in building plumbing systems is beneficial to identify more effectively at risk systems or conditions. Low cost indicators such as ATP have been used in building water quality monitoring to flag any changes in the water systems (Prest et al., 2016).
In this study, no strong correlations were observed between measured microbiological indicators and concentrations of L. pneumophila, even when considering first draw and flushed samples separately (Supplementary Figures S2A,B), in agreement with prior observations (Duda et al., 2015). However, comparatively to water samples with culture-based concentrations of L. pneumophila below 1 000 MPN/L, samples above 1 000 MPN/L tended to have significantly (p < 0.05) higher proportions of intact cells in both first draw (38 vs. 25%) and flushed samples (32 vs. 13%; Supplementary Figure S3). This suggests that higher proportions of viable bacterial cells were favorable to the detection of culture-based L. pneumophila, possibly due to beneficial ecological interactions with other microorganisms, or due to detachment of biofilm, which is an ecosystem known to harbor most live cells.
Culture-based and qPCR concentrations of L. pneumophila also correlated strongly and moderately together when modeled by a linear regression (Figure 4). The proportion of dead or viable-but-non-culturable (VBNC) cells is likely to account for the large discrepancies between culture-based and qPCR concentrations (differences ranging 0.2–3.8-log), especially in flushed samples which are representative of more unfavorable environmental conditions (e.g., higher temperatures). These discrepancies are in agreement with previous studies (Lee et al., 2011; Whiley and Taylor, 2014; Dowdell et al., 2022, submitted to Environmental Science and Technology).
In this study, flushing showerheads for 5 min with mitigated water produced clear and immediate benefits on a microbiological perspective. Overall, bacterial-ATP levels were lowered by 1.2–278-fold in flushed samples (Figure 1A), whereas total (Figure 1B) and intact (Figure 1C) bacterial cell counts were on average 1.1- and 0.7-log lower than in first draw samples. The proportion of intact cells was sightly lowered by about 14% (Figure 1D). The largest differences among paired samples were commonly observed in first draw samples with especially elevated bacterial-ATP (> 10 pg ATP/mL), total and intact cell counts over 106 and 105 count/mL, or percent of intact cells over 25%, which occurred mostly following the extended 16-week building closure. Reduced numbers and smaller reductions in measured bacterial indicators are artifacts of the upstream water quality, which is typically less vulnerable to microbial amplification due to the maintenance of a thermal barrier (in hot water) and measurable chlorine residuals (in cold water). Although culturability was evidently diminished with flushing, total cell counts almost 3-log higher than the incoming cold water entry (data not shown) suggest that a great deal of dead cells were nevertheless circulating in the building plumbing system. Observed decreases are comparable with previously reported observations in building plumbing systems and for similar flushing times in chlorinated and unchlorinated systems (Lautenschlager et al., 2010; Lipphaus et al., 2014; Bédard et al., 2018), although values observed in this study remained slightly higher.
Consistent trends were also observed for culture-based (Figure 3A) and qPCR concentrations of L. pneumophila (Figure 3B) as flushing lowered concentrations by 0.8- and 0.5-log on average, respectively. Although considerable reductions in culture-based L. pneumophila concentrations (over 1-log on average) were measured in flushed samples after the 16-week building shutdown and the monthly distal water stagnation period that followed the recommissioning flushing event, insufficient per exposure mean risk reductions (less than 1-log on average) were computed (Supplementary Table S2). This implies that a one log reduction in L. pneumophila culture-based concentrations might not be enough to reduce consequently the exposure risk to the pathogen in some instances. Moreover, considering each sampling event separately, the high proportions of flushed samples with detectable L. pneumophila by culture-based method (24–39%) or qPCR (86–100%) indicate that the contribution of biofilm during flow and upstream conditions allowed the persistence and the circulation of the bacteria across the building plumbing system, either in the VBNC form or dead.
The benefit of flushing some devices for 5 min after extended stagnation can be examined by excluding data collected 24 h after the whole recommissioning flushing conducted at every outlet. These data provide evidence of the benefits of partial distal flushing as a preventative measure. Flushing each outlet as recommended by recommissioning guidance is quite demanding and building managers may consider flushing selected points of use as an alternative method. Results show that 63 and 29% of paired samples (n = 41) underwent a minimum of 1-log reduction in culture-based and qPCR L. pneumophila loads after 5 min of device flushing. Although there is limited guidance for the interpretation of qPCR results of Legionella spp. or L. pneumophila, some showerheads reaching the 1-log reduction after a brief five-min flush still had concentrations over 103 gu/L, yet undetectable culture-based L. pneumophila. Therefore, longer flushing times may be required depending on the building plumbing system layouts to perform a water turnover equivalent to meeting upstream water quality.
It is noteworthy that flushing resulted in simultaneous increases of 0.1–1.8-log and 0.3- to 1.4-log in both culture-based and qPCR L. pneumophila concentrations in few showerheads. Such increases can be coincidental, due to odd biofilm detachment, or because of localized contamination. Although fixture-flushing is expected to reduce Legionella spp. levels (Wang et al., 2012; Hozalski et al., 2020; Richard and Boyer, 2021), it can lead to momentary detrimental effects. One study also demonstrated increases in L. pneumophila levels after flushing, which was associated to building-specific features and deleterious recommissioning flushing practices such as rapid depletion of boiler temperatures with water turnover in the hot distribution system (Rhoads et al., 2022).
Growth of Legionella bacteria and more particularly L. pneumophila were identified as a significant risk in most recommissioning flushing recommendations issued to address the safe reopening of building water systems during the COVID-19 pandemic. Yet, there is a scarcity of data regarding the short- and long-term benefits of such intervention because of the novelty of the prolonged building shutdowns that were observed during the COVID-19 pandemic.
Significant (p < 0.05) reductions in bacterial-ATP (Figure 1A), total (Figure 1B) and intact (Figure 1C) cell counts, viability ratios (Figure 1D), and culture-based L. pneumophila concentrations (Figure 3A) were observed in first draw samples collected shortly (24 h) after the targeted recommissioning flushing intervention. More importantly, culture-based L. pneumophila concentrations were lowered below the 1 000 MPN/L alert threshold (Table 1) in all first draw samples. However, despite small decreases, qPCR concentrations (Figure 3B) did not significantly (p = 0.063) change in first draw samples, likely due to its persistence in points of use and the great proportions of previously collected paired flushed samples that still had detectable L. pneumophila DNA through qPCR (Table 1).
Beneficial short-term impacts of targeted recommissioning flushing did not persist over the subsequent monthly distal water stagnation in shower systems. Indeed, bacterial indicators (Figure 1) and L. pneumophila loads (Figure 3) bounced back in first draws to more or less similar levels than those measured following the 16-week building closure. One study also demonstrated that Legionella species ssrA genes concentrations slightly rebounded in some taps during a follow-up period (2–4 days) thereafter flushing points of use for 45 min (Hozalski et al., 2020). These increases in distal parts of the building plumbing system could either reflect bacterial growth occurring under stagnant conditions (Lautenschlager et al., 2010; Zlatanović et al., 2017; Bédard et al., 2018; Peng et al., 2020; Rahmatika et al., 2022), or result from higher detachment rates of bacteria from the biofilm during stagnation and when flow resumes after prolonged periods of no use (Stoodley et al., 2001; Figure 2). It is likely that the water turnover resulting from the recommissioning flushing intervention repleted nutrients near points of use, as well as dead biomass already circulating in the hot water distribution system, supporting regrowth of bacteria and biofilm grazing hosts of Legionella, amoeba (Rhoads et al., 2022). The implementation of routine flushing regimes at points of use should therefore be explored by building managers to prevent stagnant conditions.
Two showerheads that were negative for the presence of culture-based L. pneumophila at the beginning of the study finally became positive at concentrations of 141 and 264 MPN/L. The detection of the pathogen with qPCR concentrations ranging 102–103 gu/L in their first draw samples after the 16-week building shutdown strongly suggests that the bacteria had already colonized the points of use but was mostly either in its VBNC form or dead. Aside from a single showerhead that only tested positive to culture-based L. pneumophila once, all others remained positive in both of sampling events characterized by prior distal water stagnation periods (first and last ones). This illustrates the persistence of the pathogen in distal areas, despite the targeted recommissioning flushing intervention. As flushing can only partially dislodge microorganisms found in the biofilm and stagnant water, its effect is likely to be short lived especially when followed by periods of no use.
Several authors have assessed the occurrence rate over time of the pathogen, and whereas one group found persistent contamination of Legionella species at all investigated taps throughout the year (Liu et al., 2018), others have mainly reported sporadic presence of L. pneumophila (Bédard et al., 2016a; Donohue et al., 2018; Buse et al., 2020). Generally, taps which were persistently positive for the presence of L. pneumophila tended to harbor higher concentrations of the bacteria (Donohue et al., 2018; Buse et al., 2020), similarly to what was observed in the present study. This suggests that when L. pneumophila is well established, its detection is likely to occur repeatedly regardless of the normal building water usage, although in dynamic concentration ranges.
The observations from this study raise a few important issues on how and when preventative and curative flushing should be carried out, as well as how its efficacy should be assessed. The present study was conducted at a single site and would benefit from additional flushing efficacy studies on a wider set of building plumbing systems. In this specific sports complex, partial recommissioning flushing was mostly effective at short term to lower planktonic L. pneumophila found in shower water, but building specifics at other sites could alter these trends.
Flushing as performed in this study was conducted at the shower distal points, which are considered to be the most at risk devices for aerosol formation. A more extensive and systematic turnover of water through system-wide recommissioning flushing programs in which all water outlets are individually flushed with both cold and hot water is highly recommended (AWWA, 2020; CSA, 2020; Government of Quebec, 2020; Proctor et al., 2020; CDC, 2021). It is more likely to result in lower Legionella positivity on a short- and possibly long-term perspective. Indeed, flushing with a high hot water temperature (> 55°C), then with a cold-water residual (> 0.5 mg Cl2/L) is doubtlessly more effective to control the growth of a broad range of microorganisms, and should therefore be prioritized when possible. However, without other evidence, recommissioning flushing should preferentially be carried out within 1 or 2 days from the building reopening. As bacterial indicators concentrations and Legionella loads rebounded during the monthly distal water stagnation event, the 2-week (re)commissioning threshold proposed in the ASHRAE Standard 188 may not be sufficient to maintain low Legionella levels, especially in contaminated building plumbing systems.
This study shows the wide variations (up to 3-log at times) of concentrations of L. pneumophila found in neighboring showers. This important variation raises the issue of the effective selection of devices to be sampled to monitor the efficacy of flushing. Additionally, sampling the very first draw is necessary to assess distal contamination of showerheads, comparatively to what is suggested as monitoring strategies for microbiological sampling in some guidance document (ISO, 2006). The large differences between concentrations in first draw and flushed samples (up to 3-log) strongly support collecting a first draw sample as the best indicator of the maximum recovery level of contamination to which the user may be exposed (Wang et al., 2017; Hirsh et al., 2020).
Finally, additional research is needed to identify the most suited preventive and remedial flushing regimes to limit the growth of Legionella that building managers could easily implement in water safety plans, such as automatic flushing devices that are now commonly installed in healthcare facilities. Most urgent is the production of actionable data to determine the frequency at which preventative, recommissioning and curative flushing should be conducted.
• The present study is the first to evidence the high prevalence and prolonged culturability (4–9 months) of L. pneumophila in distal parts of building plumbing systems.
• A prolonged building closure of 16-week revealed large amplification of bacterial-ATP (0.7–24 pg ATP/mL) and total and intact cell counts (104–105 count/mL) in first draw samples that exceeded previously published results for shorter stagnation times (less than 2 weeks). Mean values of L. pneumophila through culture-based and qPCR methods respectively reached 4 487 MPN/L and 63 822 gu/L, even in showerheads that were left unused for more than 9 months. These elevated loads were mostly attributable to detachment from the adjacent biofilm, which is thought to be more important after periods of prolonged stagnation.
• The widespread L. pneumophila contamination was presumably linked to poor design and operational considerations of the hot water system, including the use of TMV feeding a large (> 20) number of devices, sub-optimal temperature conditions in the hot water recirculation line due to water heaters feed flow modifications, drastic drops in temperatures observed in a small plate heat exchanger, and overall large dead water volumes in secondary piping across the building.
• Individual flushing of showerheads for 5 min resulted in significant (p < 0.05) decreases in all measured bacterial indicators. Culture-based and qPCR concentrations of L. pneumophila were also reduced by respectively 0.8- and 0.5-log on average. Profile sampling showed that prolonging flushing from 1 to 35 min provided additional reductions of concentrations of total and intact cell counts.
• Targeted recommissioning flushing was beneficial at short-term (24 h) to lower risks as all showerheads remained under the common alert threshold of 1 000 MPN/L for the monitoring of L. pneumophila in public buildings. However, recommissioning flushing was not sufficient to lower long-term (4-week) mean risks below benchmarks of 10−4 infections per exposure for a Pontiac fever endpoint and below 10−6 DALY per exposure for a Legionnaires' disease endpoint.
• The maximum stagnation period following commissioning should be reevaluated as significant positivity for L. pneumophila and rebound concentrations were observed during the monthly distal water stagnation after targeted recommissioning flushing.
• Legionella pneumophila positivity and concentrations by culture-based or qPCR methods could not be strongly predicted or correlated by any measured bacterial indicators in agreement with prior studies.
The datasets presented in this study can be found in online repositories. The names of the repository/repositories and accession number(s) can be found at: https://doi.org/10.6084/m9.figshare.19807282.v3.
MP and MG-C conceptualized the study research methodology. MG-C conducted the investigations, analyzed the data, and wrote the original draft of the manuscript. EDe, EDo, and MP revised and edited the manuscript. MP acquired funding for this study. All authors have read and agreed to the final version of the manuscript.
Funding was provided by the Natural Sciences and Engineering Research Council of Canada (NSERC) through the Alliance Grant (ALLRP 545363-19) and the Discovery research grant (RGPIN-2021-04341).
The authors thank the Industrial Chair in Drinking Water (Polytechnique Montreal) and the Centre for Research, Development and Validation of Water Treatment Technologies and Processes (CREDEAU) which benefit from the financial support of the Alliance and NSERC grants, the City of Montreal, Veolia Water Technologies Canada, the City of Laval, the City of Longueil, the City of Repentigny, the City of Joliette and the City of L'Assomption.
The authors declare that the research was conducted in the absence of any commercial or financial relationships that could be construed as a potential conflict of interest.
All claims expressed in this article are solely those of the authors and do not necessarily represent those of their affiliated organizations, or those of the publisher, the editors and the reviewers. Any product that may be evaluated in this article, or claim that may be made by its manufacturer, is not guaranteed or endorsed by the publisher.
The Supplementary Material for this article can be found online at: https://www.frontiersin.org/articles/10.3389/frwa.2022.959689/full#supplementary-material
Al-Bana, B. H., Haddad, M. T., and Garduño, R. A. (2014). Stationary phase and mature infectious forms of Legionella pneumophila produce distinct viable but non-culturable cells. Environ. Microbiol. 16, 382–395. doi: 10.1111/1462-2920.12219
Allegra, S., Leclerc, L., Massard, P. A., Girardot, F., Riffard, S., Pourchez, J., et al. (2016). Characterization of aerosols containing Legionella generated upon nebulization. Sci. Rep. 6, 33998. doi: 10.1038/srep33998
Arroyo, M. G., Ferreira, A. M., Frota, O. P., Rigotti, M. A., Andrade, D. E., Brizzotti, D., et al. (2017). Effectiveness of ATP bioluminescence assay for presumptive identification of microorganisms in hospital water sources. BMC Infec. Dis. 17,458. doi: 10.1186/s12879-017-2562-y
ASHRAE (2018). ANSI/ASHRAE Standard 188-2018, Legionellosis: Risk Management for Building Water Systems. Atlanta, GA, United States.
ASHRAE (2020). Guideline 12-2020, Managing the Risk of Legionellosis Associated With Building Water Systems. Atlanta, GA, United States.
Australian Government (2015). Guidelines for Legionella Control in the Operation and Maintenance of Water Distribution Systems in Health and Aged-Care Facilities. Adelaide, SA: SA Health.
AWWA (2020). Responding to Water Stagnation in Buildings With Reduced or No Water Use, A Framework for Building Managers. Denver, CO.
Bédard, E., Boppe, I., Kouamé, S., Martin, P., Pinsonneault, L., Valiquette, L., et al. (2016a). Combination of heat shock and enhanced thermal regime to control the growth of a persistent Legionella pneumophila strain. Pathogens. 5,35. doi: 10.3390/pathogens5020035
Bédard, E., Fey, S., Charron, D., Lalancette, C., Cantin, P., Dolcé, P., et al. (2015). Temperature diagnostic to identify high risk areas and optimize Legionella pneumophila surveillance in hot water distribution systems. Wat. Res. 71, 244–256. doi: 10.1016/j.watres.2015.01.006
Bédard, E., Laferrière, C., Déziel, E., and Prévost, M. (2018). Impact of stagnation and sampling volume on water microbial quality monitoring in large buildings. PLoS ONE. 13, e0199429. doi: 10.1371/journal.pone.0199429
Bédard, E., Lévesque, S., Martin, P., Pinsonneault, L., Paranjape, K., Lalancette, C., et al. (2016b). Energy conservation and the promotion of Legionella pneumophila growth: the probable role of heat exchangers in a nosocomial outbreak. Infect. Control. Hosp. Epidemiol. 37, 1475–1480. doi: 10.1017/ice.2016.205
Boczek, L. A., Tang, M., Formal, C., Lytle, D., and Ryu, H. (2021). Comparison of two culture methods for the enumeration of Legionella pneumophila from potable water samples. J. Water Health. 19, 468–477. doi: 10.2166/wh.2021.051
Buse, H. Y., Morris, B. J., Gomez-Alvarez, V., Szabo, J. G., and Hall, J. S. (2020). Legionella diversity and spatiotemporal variation in the occurrence of opportunistic pathogens within a large building water system. Pathogens 9, 567. doi: 10.3390/pathogens9070567
Cazals, M., Bédard, E., Doberva, M., Faucher, S., and Prévost, M. (2022). Compromised effectiveness of thermal inactivation of Legionella pneumophila in water heater sediments and water, and influence of the presence of Vermamoeba vermiformis. Microorganisms 10, 443. doi: 10.3390/microorganisms10020443
CDC (2021). Reopening buildings after prolonged shutdown or reduced operation. Available online at: https://www.cdc.gov/nceh/ehs/water/legionella/building-water-system.html (Accessed April 11, 2022).
CSA (2020). Workplaces and COVID-19: Occupational Health and Safety Considerations for Reopening and Operating During the Pandemic. Toronto, ON.
Dias, V., Durand, A. A., Constant, P., Prévost, M., and Bédard, E. (2019). Identification of factors affecting bacterial abundance and community structures in a full-scale chlorinated drinking water distribution system. Water 11, 627. doi: 10.3390/w11030627
Dias, V. C. F., Besner, M. C., and Prévost, M. (2017). Predicting water quality impact after district metered area implementation in a full-scale drinking water distribution system. J. AWWA. 109, E363–E380. doi: 10.5942/jawwa.2017.109.0099
Donohue, M. J., King, D., Pfaller, S., and Mistry, J. H. (2018). The sporadic nature of Legionella pneumophila, Legionella pneumophila sg1 and Mycobacterium avium occurrence within residences and office buildings across 36 states in the United States. J. Appl. Microbiol. 126, 1568–1579. doi: 10.1111/jam.14196
Dowdell, K. S., Greenwald, H. D., Joshi, S., Grimard-Conea, M., Pitell, S., Song, Y., et al. (2022). Legionella pneumophila occurrence in reduced-occupancy buildings in 11 cities during the COVID-19 pandemic. medRxiv. doi: 10.1101/2022.06.28.22277022
Duda, S., Baron, J. L., Wagener, M. M., Vidic, R. D., and Stout, J. E. (2015). Lack of correlation between Legionella colonization and microbial population quantification using heterotrophic plate count and adenosine triphosphate bioluminescence measurement. Environ. Monit. Assess. 187, 393. doi: 10.1007/s10661-015-4612-5
ESCMID (2017). European Technical Guidelines for the Prevention, Control and Investigation of Infections Caused by Legionella Species, Basel.
Fields, B. S., Benson, R. F., and Besser, R. E. (2002). Legionella and Legionnaires' disease: 25 years of investigation. Clin. Microbiol. Rev. 15, 506–526. doi: 10.1128/CMR.15.3.506-526.2002
Gatza, E., Hammes, F., and Prest, E. (2013). Assessing Water Quality With the BD AccuriTM C6 Flow Cytometer. Franklin Lakes, NJ: BD Biosciences.
Giglio, D. E., Diella, O., Lopuzzo, G., Triggiano, M., Calia, F., Pousis, C., et al. (2020). Impact of lockdown on the microbiological status of the hospital water network during COVID-19 pandemic. Environ. Res. 191, 110231. doi: 10.1016/j.envres.2020.110231
Government of Quebec (2020). Recommendations for Restoring Service To Water Distribution Systems In Vacant Buildings, Quebec.
Hamilton, K. A., Hamilton, M. T., Johnson, W., Jjemba, P., Bukhari, Z., LeChevallier, M., et al. (2019). Risk-based critical concentrations of Legionella pneumophila for indoor residential water uses. Environ. Sci. Technol. 53, 4528–4541. doi: 10.1021/acs.est.8b03000
Hammes, F., Berney, M., Wang, Y., Vital, M., Köster, O., Egli, T., et al. (2008). Flow-cytometric total bacterial cell counts as a descriptive microbiological parameter for drinking water treatment processes. Wat. Res. 42, 269–277. doi: 10.1016/j.watres.2007.07.009
Hammes, F., Goldschmidt, F., Vital, M., Wang, Y., and Egli, T. (2010). Measurement and interpretation of microbial adenosine tri-phosphate (ATP) in aquatic environments. Wat. Res. 44, 3915–3923. doi: 10.1016/j.watres.2010.04.015
Hirsh, M. B., Baron, J. L., Mietzner, S. M., Rihs, J. D., Yassin, M. H., Stout, J. E., et al. (2020). Evaluation of recommended water sample collection methods and the impact of holding time on Legionella recovery and variability from healthcare building water systems. Microorganisms 8, 1770. doi: 10.3390/microorganisms8111770
Hozalski, R. M., LaPara, T. M., Zhao, X., Kim, T., Waak, M. B., Burch, T., et al. (2020). Flushing of stagnant premise water systems after the COVID-19 shutdown can reduce infection risk by Legionella and Mycobacterium spp. Environ. Sci. Technol. 54, 15914–15924. doi: 10.1021/acs.est.0c06357
ISO (2006). ISO 19458, 2006. – Water Quality – Sampling for Microbiological Analysis. Geneva: International Organization for Standardization.
Lautenschlager, K., Boon, N., Wang, Y., Egli, T., and Hammes, F. (2010). Overnight stagnation of drinking water in household taps induces microbial growth and changes in community composition. Wat. Res. 44, 4868–4877. doi: 10.1016/j.watres.2010.07.032
Lee, J. V., Lai, S., Exner, M., Lenz, J., Gaia, V., Casati, S., et al. (2011). An international trial of quantitative PCR for monitoring Legionella in artificial water systems. J. Appl. Microbiol. 110, 1032–1044. doi: 10.1111/j.1365-2672.2011.04957.x
Lipphaus, P., Hammes, F., Kotzsch, S., Green, J., Gillespie, S., Nocker, A., et al. (2014). Microbiological tap water profile of a medium-sized building and effect of water stagnation. Environ. Technol. 35, 620–628. doi: 10.1080/09593330.2013.839748
Liu, L., Xing, X., Hu, C., and Wang, H. (2018). One-year survey of opportunistic premise plumbing pathogens and free-living amoebae in the tap-water of one northern city of China. J. Environ. Sci. 77, 20–31. doi: 10.1016/j.jes.2018.04.020
Manuel, C. M., Nunes, O. C., and Melo, L. F. (2010). Unsteady state flow and stagnation in distribution systems affect the biological stability of drinking water. Biofouling. 26, 129–139. doi: 10.1080/08927010903383448
Montagnino, E., Proctor, C. R., Ra, K., Ley, C., Noh, Y., Vigil, K., et al. (2022). Over the weekend: water stagnation and contaminant exceedances in a green office building. PLoS Water 1, e0000006. doi: 10.1371/journal.pwat.0000006
NASEM (2019). Management of Legionella in Water Systems. Washington, DC: The National Academies Press.
NHS England (2016). Health Technical Memorandum 04-01: Safe Water in Healthcare Premises, Part B: Operational Management, London.
Paul, E., Ochoa, J. C., Pechaud, Y., Liu, Y., and Liné, A. (2012). Effect of shear stress and growth conditions on detachment and physical properties of biofilms. Wat. Res. 46, 5499–5508. doi: 10.1016/j.watres.2012.07.029
Peng, H., Zhang, Y., Wang, R., Liu, J., and Liu, W. T. (2020). Assessing the contribution of biofilm to bacterial growth during stagnation in shower hoses. Water Supply. 20, 2564–2576. doi: 10.2166/ws.2020.161
Prest, E. I., Hammes, F., Van Loosdrecht, M. C. M., and Vrouwenvelder, J. S. (2016). Biological stability of drinking water: controlling factors, methods, and challenges. Front. Microbiol. 7, 45. doi: 10.3389/fmicb.2016.00045
Prévost, M., Abouelmakarim, A., and Bédard, E. (2019). Establishing the Prevalence of Legionella Pneumophila in Various Niches of Chlorinated Drinking Water Distribution System [Conference Presentation]. 20th Symposium on Health-Related Water Microbiology. Vienna: IWA.
Proctor, C., Rhoads, W. J., Keane, T., Salehi, M., Hamilton, K., Pieper, K. J., et al. (2020). Considerations for large building water quality after extended stagnation. AWWA Water Sci. 2, e1186. doi: 10.1002/aws2.1186
Rahmatika, I., Kurisu, F., Furumai, H., and Kasuga, I. (2022). Dynamics of the microbial community and opportunistic pathogens after water stagnation in the premise plumbing of a building. Microbes Environ. 37, ME21065. doi: 10.1264/jsme2.ME21065
Rhoads, W. J., Sindelar, M., Margot, C., Graf, N., and Hammes, F. (2022). Variable Legionella response to building occupancy patterns and precautionary flushing. Microorganisms. 10, 555. doi: 10.3390/microorganisms10030555
Richard, R., and Boyer, T. H. (2021). Pre- and post-flushing of three schools in Arizona due to COVID-19 shutdown. AWWA Water Sci. 3, e1239. doi: 10.1002/aws2.1239
Sartory, D. P., Spies, K., Lange, B., Schneider, S., and Langer, B. (2017). Evaluation of a most probable number method for the enumeration of Legionella pneumophila from potable and related water samples. Lett. Appl. Microbiol. 64, 271–275. doi: 10.1111/lam.12719
Schrammel, B., Cervero-Aragó, S., Dietersdorfer, E., Walochnik, J., Lück, C., Sommer, R., et al. (2018). Differential development of Legionella sub-populations during short- and long-term starvation. Wat. Res. 141, 417–427. doi: 10.1016/j.watres.2018.04.027
Sharaby, Y., Rodríguez-Martínez, S., Oks, O., Pecellin, M., Mizrahi, H., Peretz, A., et al. (2017). Temperature-dependent growth modeling of environmental and clinical Legionella pneumophila multilocus variable-number tandem-repeat analysis (MLVA) genotypes. Appl. Environ. Microbiol. 83, e03295. doi: 10.1128/AEM.03295-16
Siebel, E., Wang, Y., Egli, T., and Hammes, F. (2008). Correlations between total cell concentration, total adenosine tri-phosphate concentration and heterotrophic plate counts during microbial monitoring of drinking water. Drink. Water Eng. Sci. 1, 1–6. doi: 10.5194/dwes-1-1-2008
Singh, R., Chauhan, D., Fogarty, A., Rasheduzzaman, M., and Gurian, P. L. (2022). Practitioners' perspective on the prevalent water quality management practices for Legionella control in large buildings in the United States. Water. 14, 663. doi: 10.3390/w14040663
Spies, K., Pleischl, S., Lange, B., Langer, B., Hübner, I., Jurzik, L., et al. (2018). Comparison. of the Legiolert™/Quanti-Tray® MPN test for the enumeration of Legionella pneumophila from potable water samples with the German regulatory requirements methods ISO 11731-2 and ISO 11731. Inter. J. Hyg. Environ. Health. 221, 1047–1053. doi: 10.1016/j.ijheh.2018.07.006
Stoodley, P., Wilson, S., Hall-Stoodley, L., Boyle, J. D., Lappin-Scott, H. M., Costerton, J. W., et al. (2001). Growth and detachment of cell clusters from mature mixed-species biofilms. Appl. Environ. Microbiol. 67, 5608–5613. doi: 10.1128/AEM.67.12.5608-5613.2001
Van Lier, A., McDonald, S. A., Bouwknegt, M., Kretzschmar, M. E., Havelaar, A. H., Mangen, M. J. J., et al. (2016). Disease burden of 32 infectious diseases in the Netherlands, 2007–2011. PLoS ONE. 11, e0153106. doi: 10.1371/journal.pone.0153106
Van Nevel, S., Koetzsch, S., Proctor, C. R., Besmer, M. D., Prest, E. I., Vrouwenvelder, J. S., et al. (2017). Flow cytometric bacterial cell counts challenge conventional heterotrophic plate counts for routine microbiological drinking water monitoring. Wat. Res. 113, 191–206. doi: 10.1016/j.watres.2017.01.065
VHA (2021). VHA Directive 1061, Prevention of Health Care-Associated Legionella Disease and Scald Injury From Water Systems. Washington, DC.
Wang, H., Bédard, E., Prévost, M., Camper, A. K., Hill, V. R., Pruden, A., et al. (2017). Methodological approaches for monitoring opportunistic pathogens in premise plumbing: a review. Wat. Res. 117, 68–86. doi: 10.1016/j.watres.2017.03.046
Wang, H., Edwards, M., Falkinham, I. I. I., and Pruden, J. O. A. (2012). Molecular survey of the occurrence of Legionella spp., Mycobacterium spp., Pseudomonas aeruginosa, and Amoeba hosts in two chloraminated drinking water distribution systems. Appl. Environ. Microbiol. 78, 6285–6294. doi: 10.1128/AEM.01492-12
Whiley, H., and Taylor, M. (2014). Legionella detection by culture and qPCR: comparing apples and oranges. Crit. Rev. Micobiol. 42, 65–74. doi: 10.3109/1040841X.2014.885930
Wilkes, C. R., Mason, A. D., and Hern, S. C. (2005). Probability distributions for showering and bathing water-use behavior for various U.S. subpopulations. Risk Anal. 25, 317–337. doi: 10.1111/j.1539-6924.2005.00592.x
Yu, Z., and Mohn, W. (1999). Killing two birds with one stone: simultaneous extraction of DNA and RNA from activated sludge biomass. Can. J. Microbiol. 45, 269–272. doi: 10.1139/w98-211
Zhang, K., Pan, R., Zhang, T., Xu, J., Zhou, X., Yang, Y., et al. (2019). A novel method: using an adenosine triphosphate (ATP) luminescence-based assay to rapidly assess the biological stability of drinking water. Appl. Microbiol. Biotech. 103, 4269–4277. doi: 10.1007/s00253-019-09774-3
Keywords: legionella, flushing, recommissioning, stagnation, building plumbing, COVID-19
Citation: Grimard-Conea M, Deshommes E, Doré E and Prévost M (2022) Impact of recommissioning flushing on Legionella pneumophila in a large building during the COVID-19 pandemic. Front. Water 4:959689. doi: 10.3389/frwa.2022.959689
Received: 02 June 2022; Accepted: 28 September 2022;
Published: 13 October 2022.
Edited by:
Frederik Hammes, Swiss Federal Institute of Aquatic Science and Technology, SwitzerlandReviewed by:
Joseph Oliver Falkinham, Virginia Tech, United StatesCopyright © 2022 Grimard-Conea, Deshommes, Doré and Prévost. This is an open-access article distributed under the terms of the Creative Commons Attribution License (CC BY). The use, distribution or reproduction in other forums is permitted, provided the original author(s) and the copyright owner(s) are credited and that the original publication in this journal is cited, in accordance with accepted academic practice. No use, distribution or reproduction is permitted which does not comply with these terms.
*Correspondence: Marianne Grimard-Conea, bWFyaWFubmUtMi5ncmltYXJkLWNvbmVhQHBvbHltdGwuY2E=
Disclaimer: All claims expressed in this article are solely those of the authors and do not necessarily represent those of their affiliated organizations, or those of the publisher, the editors and the reviewers. Any product that may be evaluated in this article or claim that may be made by its manufacturer is not guaranteed or endorsed by the publisher.
Research integrity at Frontiers
Learn more about the work of our research integrity team to safeguard the quality of each article we publish.