- Stable Isotopes for Biosphere Science Laboratory, Department of Ecology and Conservation Biology, Texas A&M University, College Station, TX, United States
Across the karst landscape of Quintana Roo, Mexico, plant access to nutrients and water appears limited by generally shallow soil. However, underlying this surface are heterogenous pockets in bedrock and deeper, stable groundwater, suggesting the potential for specialization by species in accessing soil resources. If species differentially access rock resources, divisions by functional groups may also be expected. In this study, shallow caves provided an opportunity to assess resource use strategies by direct, species-specific root observations coupled with traditional above ground measurements. Utilizing stable isotopes from stems and leaves (δ18O and δ13C), we investigated water access and water use efficiency of trees during the dry season to uncover relationships between rooting habit, tree size, and pre-determined functional groups based on leaf habit and wood density. Functional group membership did not predict measured stable isotope ratios, indicating that functional groups were poor predictors of resource use. We did find evidence for deep water use by select species and larger individuals. Interestingly, as trees became larger, δ13C increased to a threshold but then declined, suggesting increasing vulnerability to water limitation as trees increase in size, consistent with other seasonally dry tropical forests. Our work demonstrates that, although shallow soils likely drive strong resource limitations, co-occurring trees in karst ecosystems employ diverse resource acquisition strategies, suggesting important consequences for community composition and ecosystem function in the face of environmental change.
Introduction
In seasonally dry habitats, plants often face a fundamental tradeoff between maintaining functional water status and sufficient C uptake (McDowell et al., 2008). Several plant traits have been identified as adaptive in these environments. These include high stomatal sensitivity (Henry et al., 2019), low leaf mass per area (Givnishn, 2002; Wright et al., 2004), high cavitation resistance (Steudle, 2001; Sperry, 2003; Chave et al., 2009; Trueba et al., 2017), deciduousness (Álvarez-Yépiz et al., 2017), and deep roots for better access to reliable water (Doody and Benyon, 2011; Nardini et al., 2016; Pierret et al., 2016). Given high species diversity, particularly in tropical habitats, classification strategies, like resource conservative vs. acquisitive have been derived primarily from leaf traits (Aerts, 1995; Reich, 2014). Researchers have further attempted to link leaf traits with root traits to establish whole plant functional groups (Freschet et al., 2015; McCormack et al., 2015). However, several studies have demonstrated apparent disconnects between the above and below ground function and a coherent whole plant approach remains elusive (Roumet et al., 2016; Weemstra et al., 2016; Kleinschmidt et al., 2020). Thus, a gap in our understanding of how root traits interact with better characterized leaf and wood traits persists (Grew, 1692; Yamauchi et al., 2021; Zhou et al., 2022).
Seasonally dry tropical forests are compelling ecosystems to investigate resource use strategies due to apparently obvious challenges associated with water availability (Santiago et al., 2004). Several studies report niche separation based on functional groups (Kitajima and Poorter, 2008; Markesteijn et al., 2011; Álvarez-Yépiz et al., 2017), with evergreens having advantages over deciduous species due to conservative strategies expected to be associated with longer leaf lifespans and persistence of carbon gain into dry periods (Mitchell et al., 2008; Hasselquist et al., 2010; Ellsworth and Sternberg, 2015). Wood density is also associated with water use strategies, following observations of species with low wood density and high hydraulic efficiency employing a “boom-and-bust” style while species with high wood density tend to align with conservative traits (Méndez-Alonzo et al., 2012; Reich, 2014). Contrarily, others have noted that functional groups were not useful in predicting differences in water-use strategies in dry tropical forests (Powers and Tiffin, 2010; Werden et al., 2018). Significant intra- and interspecific variation among traits and resource use strategies observed in seasonally dry tropical forests add complexity to functional group organizational schemes, potentially weakening their utility in these systems (Hulshof and Swenson, 2010; Xu et al., 2016; Santiago et al., 2017; Derroire et al., 2018). However, continued research to understand resource acquisition strategies and underlying drivers is crucial for conservation efforts and predictions for plant responses to climate change. In addition, seasonally dry tropical forests are among the most threatened of global tropical forests, often impacted by deforestation for agriculture and urbanization (Arturo Sánchez-Azofeifa et al., 2005), despite the fact that they are crucial landscapes for carbon sequestration and storage (Becknell et al., 2012) and home to notably high phylogenetic and functional diversity (Eamus, 1999; Cavender-Bares et al., 2016; DRYFLOR, 2016).
We studied a seasonally dry tropical forest in the eastern Yucatán Peninsula, which provided an ideal setting to assess relationships between water use efficiency, plant function, and apparent rooting strategies. Semi-evergreen tropical forests dominate in the eastern state of Quintana Roo (Ibarra-Manríquez et al., 2002; Durán-García et al., 2016) where there is a dry season from November to May, in which 20% of the annual precipitation falls. The peninsula is a vast karst landscape, which is expected to exacerbate this limitation by limiting surface or soil water availability (Geekiyanage et al., 2019). The region is generally characterized by roughly 10 centimeter deep soils atop limestone bedrock where water content and storage vary with the heterogeneous distribution of cavities in the rock (Querejeta et al., 2007; Estrada-Medina et al., 2013a; Fragoso-Servón et al., 2020). This surface dynamism is contrasted by the karst aquifer, a reliable water source, that underlies the entire peninsula (Bauer-Gottwein et al., 2011). In our study area, the distance to the surface of the freshwater lens varies from 4 to 5 m deep, but may be between 9 and 20 meters deep near Mérida in the northwest (Hasselquist et al., 2010; Estrada-Medina et al., 2013a; Durán-García et al., 2016). The increase in abundance of evergreen species as precipitation increases and depth to groundwater decreases from west to east prompts questions about functional group dependence on groundwater and how this relates to other plant traits (Nepstad et al., 1994; Bleby et al., 2010; Swaffer et al., 2014; Nardini et al., 2016).
Below ground, extensive cave systems have formed by dissolution of the limestone bedrock (Smart et al., 2006; van Hengstum et al., 2010). In these caves, it is common to see tree roots emerging from conduits in the ceiling, walls, and speleothems (Adams et al., 2020b). Observations of roots in contact with groundwater in many areas of the eastern peninsula provide evidence that some trees are indeed accessing and utilizing deep water. Although commonly observed in these caves, very little is known about the role deep water access plays in water stress tolerance for these species and how rooting habits relate to other characteristics of these trees.
We report here a field-based effort to characterize relationships between access to groundwater and inferred water stress and whether these were consistent with expectations from functional group theory. We made three specific predictions: (1) evergreen species would have relatively high water use efficiency, but be more reliant on groundwater than deciduous species, with more abundant roots in caves than deciduous species, (2) trees with lower wood density (irrespective of leaf habit) would have lower water use efficiency and be more reliant on deep water with abundant roots in caves than high wood density species, and (3) species with larger individuals aboveground would also be dominant in the caves with consistent deep-water access.
Materials and Methods
Site Description and Plot Establishment
Our field sites were in the eastern state of Quintana Roo, Mexico. This portion of the Yucatán peninsula receives between 1200 to 1500 mm of precipitation annually, with a dry period from November to May (Bauer-Gottwein et al., 2011; Durán-García et al., 2016). Forests are classified as semi-evergreen, with approximately 25% of the trees losing leaves in the dry season (Sánchez-Sánchez and Islebe, 2002). This work was conducted at four sites along the Caribbean coast of Quintana Roo. The sites, Cueva Culebron, Jaguar Maw, Nohoch Aktun, and Ruta de los Guerreros, were located between the cities of Paamul and Akumal. Each site was associated with a cave network, allowing direct access to roots and groundwater. Because an important aim was to directly connect the surface trees to their subterranean roots, detailed cave maps were required for each (Trimmis, 2018). Using cave survey data, surface plots (100 m2) were randomly established at each site directly above cave passages and paired with below ground plots used to assess root biodiversity and abundance (Adams, 2020). Using survey data, GPS locations on the surface mirroring the location of georeferenced survey stations below ground were gathered before arriving at the sites (see the Supplementary Materials for details). Two plots were randomly selected from these candidates at each site.
Vegetation Surveys
Field work was conducted between December 28, 2017 and January 8, 2018, during the dry season to capture differences in water use strategies during a time of limited water availability. To assess above ground community composition, all individuals were identified to species either in the field or via subsequent DNA barcoding (Adams, 2020). All trees with a diameter at breast height (DBH) ≥ 5 cm were recorded. Basal area was calculated for each tree and compiled by species and site. Using the cave at each site, we collected root samples in the paired below ground plots and later identified species reaching into the caves to groundwater with DNA barcoding (Adams, 2020). Based on these observations, species were organized into three rooting habit groups: above ground only (roots never observed in caves), rare below ground (<5% relative abundance), and common below ground (>5% relative abundance). For a full summary of the above and below ground community composition, (see Supplementary Table 1).
Stems and leaves of all individuals measured were collected for stem water (δ18O and δ2H) and leaf C (δ13C) and N (δ15N) stable isotope analysis. Stems were stripped of bark in the field and quickly placed in glass scintillation vials with lined screw caps (12 mL, Wheaton-DWK Life Sciences, Millville, NJ, USA). Caps were wrapped with Parafilm to prevent loosening. Vials were stored on ice in the field and then frozen until transport to the United States. Leaves for C and N isotope analysis were placed in Whirlpak bags (Nasco, Fort Aktinson, WI, USA) filled with silica gel (VWR International, LLC, Radnor, PA, USA) to rapidly dry the leaves. Silica gel included indicator and was replaced as necessary in the bags as the leaves dried.
Soil samples were collected in the middle of the north half of each plot. These samples were later used to characterize the isotopic composition of soil water. Soil was collected at 5 and 10 cm from the surface after removing the litter layer. Scintillation vials were filled three quarters full, sealed, stored, and transported as above for stems. When samples arrived at Texas A&M University, stem and soil samples were stored in a freezer (0°C) for later processing. Leaves were stored in the lab with added silica gel to ensure samples remained dry until processing.
Water was also sampled from pools in the caves for a synoptic assessment of groundwater isotope ratios. For collection, glass vials (20 mL, VWR International, LLC, Radnor, PA, USA) were filled completely to minimize headspace and capped and sealed as above. Once at Texas A&M University, water samples were stored in a refrigerator (4°C) until stable isotope analysis.
Categorizing Functional Groups
Tree species were organized into functional groups based on leaf habit and wood density. Associated information was gathered from existing literature from the Yucatán Peninsula and other tropical forests (Supplementary Table 1). Distinction between lightwoods, softwoods, and hardwoods followed Borchert (1994), with wood density values of <0.05, 0.5–0.8, and >0.8 g cm−3, respectively.
Sample Processing
Water was extracted from stem and soil samples via cryogenic vacuum distillation. Extractions were performed at 100°C on a closed vacuum extraction line (West et al., 2006). We recognize that extraction temperature and other variables like soil texture and mineralogy potentially interact to affect the isotopic composition of extracted water (Adams et al., 2020a). We chose to perform extractions at this temperature to be consistent with other published work and with our objective of comparing among individuals with access to soils with similar clay content (Orlowski et al., 2016). Extractions were considered complete when no further visible condensation was observed in the cold finger. Two stem samples were outliers with evaporative losses from the extraction process and were removed from subsequent analyses.
Water samples were transferred into vials (0.3 mL, Wheaton-DWK Life Sciences, Millville, NJ, USA) for stable isotope analysis. Analyses were performed using a Delta V Advantage isotope ratio mass spectrometer (IRMS) linked to a High Temperature Conversion/Elemental Analyzer (TC/EA) via a Conflo IV (Thermo Fisher Scientific, Waltham, MA, USA). Calibration standards were: SIBS-wA (δ18O = −50.10‰, δ2H = −390.8‰), SIBS-wP (δ18O = −4.61‰, δ2H = −34.1‰) and a QC check with SIBS-wU (δ18O = −15.97‰, δ2H = −120.2‰).
Leaves for C and N isotope analysis were ground to powder using a Ball Mill (MM 400; Retsch GmbH, Haan, Germany), placed in tin capsules, and weighed prior to isotope analysis (EA-IRMS; Costech Analytical Technologies Inc., Valencia, CA, USA). Calibration standards were: USGS 40, δ13C = −26.39‰, δ15N = −4.52‰ and USGS 41, δ13C = 36.55‰, δ15N = 47.55‰. All sample processing and stable isotope analysis was performed at the Stable Isotopes for Biosphere Science (SIBS) Laboratory (https://sibs.tamu.edu) at Texas A&M University.
Leaves from 6 individuals that spanned the range of found tree size were analyzed for Δ14C to determine if root-derived bicarbonate could be a source of CO2 for leaf photosynthesis, potentially increasing with tree size (Berry, 1989; Hang and Wu, 2016; Rao and Wu, 2017). Analyses were conducted at the W.M. Keck-Carbon Cycle Accelerator Mass Spectrometry (KCCAMS) facility at University of California-Irvine (https://sites.uci.edu/keckams/). Radiocarbon concentrations were given as fractionation-corrected fraction modern (F14C), Δ14C, and conventional radiocarbon age, following Stuiver and Polach (1977). All samples were classified as “modern”. We found no relationship between F14C and δ13C (R2 = −0.25, p = 0.96), allowing us to interpret δ13C solely as a function of leaf physiology (Supplementary Table 3).
Stable Isotope Analysis
Isotopic composition of stem water from individual trees was compared to soil water and cave water collected from each site. This allowed for evaluation of an individual tree's water source, given the assumption that stem water is a weighted average of the water acquired by the tree and there is no fractionation during water uptake (Ehleringer and Dawson, 1992). Trees were identified as utilizing shallow water if it resembled the heavy isotope-enriched soil water value or deep water if it resembled the heavy isotope-depleted cave water value. We did not attempt to quantify a quantitative fraction of groundwater use, as we were interested in relative differences among individuals.
Statistical Evaluation
Data analysis was carried out with the statistical software R (R Core Team, 2019) run in RStudio (RStudio Team, 2019). Model residuals were checked for normality using the Shapiro-Wilk normality test and checked for equal variance with the Brown-Forsythe test. The δ2H residuals were not normal and were transformed before analysis. Effect of site, species, rooting habit, basal area, and functional group on δ18O, δ2H, δ13C, and δ15N values were evaluated using one-way ANOVAs. Welch's tests were used when residuals violated ANOVA assumptions. A summary of these analyses can be found in Supplementary Table 4. TukeyHSD mean comparisons and Games-Howell post hoc tests provided insight into the differences between groups. Linear regressions were used to assess the overall relationship between δ18O and δ13C. Separate linear regressions were performed for each rooting habit group to isolate potential patterns in water use and efficiency among these individuals. Similarly, linear regressions were used to assess the overall relationship between δ18O and δ15N as well as separate analyses for non-legumes and legumes. This analysis was performed to determine if patterns in nitrogen availability in tropical karst systems complimented rooting depth and water use observations. A summary of these analyses can be found in Supplementary Table 5. Independent, two sample t-tests were employed to determine if there were differences in soil water isotopes from different depths and cave water collected from different seasons.
Results
Water Stable Isotopes
Cave water was collected during the dry season from Cueva Culebron and Ruta de los Guerreros and during the wet season (August 2017) at Ruta de los Guerreros and Nohoch Aktun. No cave water samples were collected at Jaguar Maw as there was no exposed water in this system. Independent, two sample t-tests showed that water collected in the dry season did not differ from water collected during the wet season for both δ18O and δ2H (t14 = 1.89, p = 0.08 and t14 = −0.15, p = 0.88, respectively). The average isotopic composition of cave water was δ18O: −4.48 ± 0.25‰; n = 11, δ2H: −27.1 ± 1.6‰. Soil water from 5 and 10 cm in the dry season was δ18O: −1.4 ± 0.69‰; n = 12, δ2H: −14.1 ± 4.2‰. There was not a significant difference between depths for either δ18O or δ2H (t10 = −1.24, p = 0.24 and t10 = −1.05, p = 0.32, respectively).
Stem water δ18O values across all samples ranged from −4.93 to −1.57‰, with an average of −3.21 ± 0.79‰. δ2H values ranged from −37.7 to −11.5‰ with an average of −22.8 ± 6.1‰. δ18O varied significantly across sites (F3, 67.5 = 6.81, p < 0.01). Trees from Cueva Culebron had stem water that was heavy isotope-depleted compared to Jaguar Maw (t64.9 = 4.43, p < 0.01; Table 1). δ2H values from stem water also significantly varied by site (F3, 70.7 = 16.3, p < 0.01), with all sites being different from each other, except Nohoch Aktun overlapped with Ruta de los Guerreros (t52.7 = 0.33, p = 0.99; Table 1). Jaguar Maw was had the highest mean δ2H, matching the pattern observed for δ18O.
Leaf Stable Isotopes
Across sites, leaf δ13C ranged from −35.3 to −27.4‰, with an average of −31.1 ± 1.5‰. There was a significant difference in δ13C values among sites (F3, 68.6 = 2.90, p = 0.04), with the difference between Ruta de los Guerreros and Jaguar Maw appearing to drive this site effect (t74.6 = 2.62, p = 0.05; Table 1).
Leaf δ15N varied from −1.9 to 4.8‰, with an average of 1.2 ± 1.2‰. As expected, legumes had δ15N values that were closer to 0‰, compared to non-nitrogen fixing species (F1, 142 = 17.1, p < 0.01). δ15N increased with increasing δ18O values among individual trees (R2 = 0.11, p < 0.01), with legumes and non-legumes both having significantly positive relationships (R2 = 0.26, p = 0.01 and R2 = 0.07, p < 0.01, respectively; Figure 1A). There was a significant difference in δ15N values by site (F3, 68.9 = 23.8, p < 0.01), with sites being different from each other except for Cueva Culebron and Jaguar Maw (t73.3 = 1.71, p = 0.32; Table 1). Nohoch Aktun had the highest δ15N values and was associated with dominance by O. lanceolata, a shallow rooted species. Ruta de los Guerreros had the lowest δ15N values, consistent with S. cubensis, a legume, being the most common tree at this site.
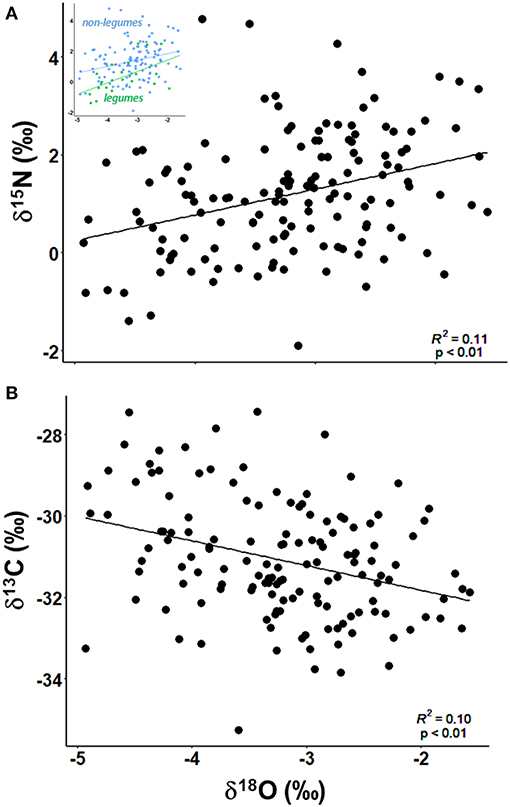
Figure 1. Relationships between δ18O from stem water and δ15N [(A); R2 = 0.11, p < 0.01] and δ13C [(B); R2 = 0.10, p < 0.01] from leaves among individual trees. The inset in (A) depicts the linear regressions for non-legumes (R2 = 0.07, p < 0.01) and legumes (R2 = 0.26, p = 0.01).
Water and Carbon Isotopes Based on Rooting Habit
Three genera/species were identified as common below ground, all evergreens: Ficus spp. Pouteria spp. and Leucaena leucocephala (Lam.) de Wit (Ficus and Pouteria could not be identified lower than genus level). Of the 29 tree species with roots exposed in the caves, 17 were evergreen and 12 were deciduous. The rare below ground group was fairly equally represented by evergreen (47%) and deciduous (53%) species. Overall, 49% of evergreens observed above ground had roots in caves compared to 63% of deciduous species.
δ18O and δ2H from extracted stem water did not differ between rooting habit groups (F2, 139 = 0.19, p = 0.83 and F2, 139 = 2.71, p = 0.07, respectively; Table 2). Likewise, leaf δ13C and δ15N did not differ between rooting habit groups (F2, 140 = 0.75, p = 0.47 and F2, 141 = 0.75, p = 0.48, respectively; Table 2). The relationship with δ15N did not change when legumes were excluded from the analysis (F2, 118 = 1.89, p = 0.16).
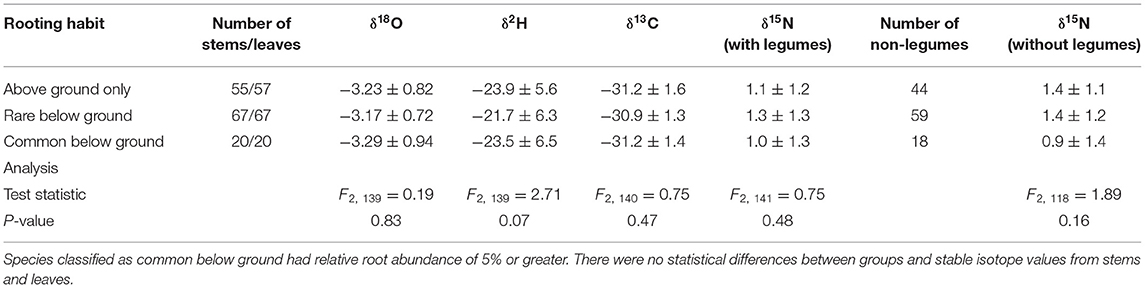
Table 2. Mean and standard deviation (1σ) of stem water δ18O and δ2H as well as leaf δ13C and δ15N by rooting habit groups.
We observed a negative correlation across all individuals between δ13C and δ18O (R2 = 0.10, p < 0.01; Figure 1B). Separating individuals into their respective rooting habit groups based on root abundance revealed differences in the relationship between C and O isotopes (Figure 2). Individuals in the rare below ground and common below ground groups both have significant relationships between δ13C and δ18O values (R2 = 0.09, p < 0.01 and R2 = 0.55, p < 0.01, respectively), while individuals in the above group only group do not (R2 = 0.02, p = 0.13).
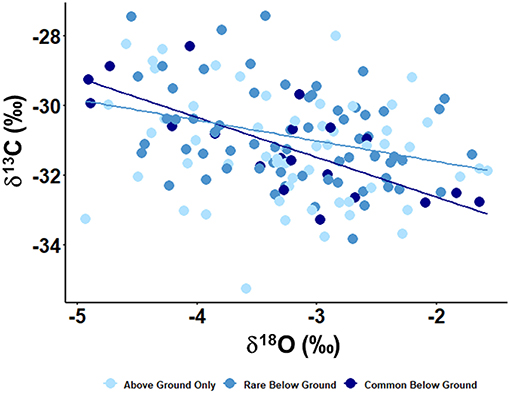
Figure 2. Leaf δ13C by stem water δ18O and the relationships among rooting habit groups. The linear regression for the above ground only group (light blue) was not significant (R2 = 0.02, p = 0.13), while the regressions for the rare below ground (medium blue) and common below ground (dark blue) groups were significant (R2 = 0.09, p < 0.01 and R2 = 0.55, p < 0.01, respectively).
Water Use Strategies Among Functional Groups
There was no statistical effect of leaf habit (evergreen vs. deciduous) for δ18O, δ2H, or δ13C (F1, 140 = 1.05, p = 0.31, F1, 140 = 0.03, p = 0.87, and F1, 141 = 2.4, p = 0.12, respectively). Leaf δ15N among evergreens was significantly more heavy-isotope enriched compared to deciduous species when evaluated with and without legumes (F1, 142 = 8.87, p < 0.01 and F1, 119 = 6.89, p < 0.01, respectively).
Stem water and leaf C isotopes did not differ among wood density groups (F2, 132 = 1.32, p = 0.27, F2, 132 = 2.61, p = 0.08, and F2, 133 = 0.37, p = 0.69, respectively). Leaf δ15N did not differ by wood density group when analyzed with legumes (F2, 134 = 0.45, p = 0.64), but when legumes were excluded, wood density groups differed (F2, 111 = 3.94, p = 0.02). In this case, hardwoods were significantly 15N-enriched compared to softwoods (p = 0.02).
Functional groups based on leaf habit and wood density together did not yield significant differences in δ18O, δ2H, or δ13C values (F5, 129 = 0.92, p = 0.47, F5, 129 = 1.31, p = 0.27, and F5, 130 = 1.33, p = 0.26, respectively; Table 3). Leaf δ15N differed by functional group for the complete dataset (F5, 25.8 = 2.64, p = 0.05; Table 3) and when legumes were removed (F4, 109 = 2.65, p = 0.04), with evergreen hardwoods being heavy-isotope enriched compared to deciduous softwoods (p = 0.03).
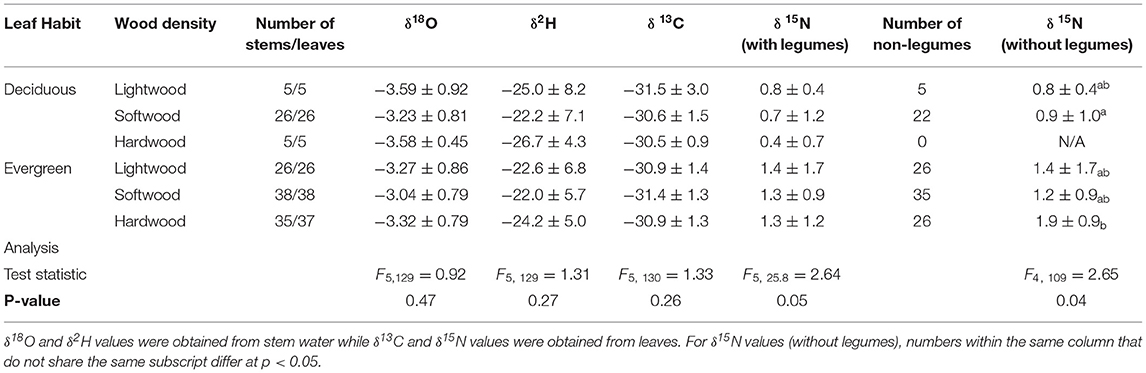
Table 3. Mean and standard deviation (1σ) of δ18O, δ2H, δ13C, and δ15N by functional group based on leaf habit and wood density.
Water and Carbon Isotopes Based on Tree Size
δ18O values from extracted stem water were significantly different among size classes (F5, 136 = 3.35, p < 0.01); Figure 3A). The two largest size classes based on DBH (25 to 29.9 cm and 30 to 34.9 cm) had a relatively narrow range of heavy isotope-depleted values, while the other four size classes (5 to 9.9 cm, 10 to 14.9 cm, 15 to 19.9 cm, and 20 to 24.9 cm) had a wide range of δ18O, with relatively heavy isotope-enriched means for each group. However, δ2H did not vary with DBH (F5, 136 = 2.10, p = 0.07; Supplementary Table 6).
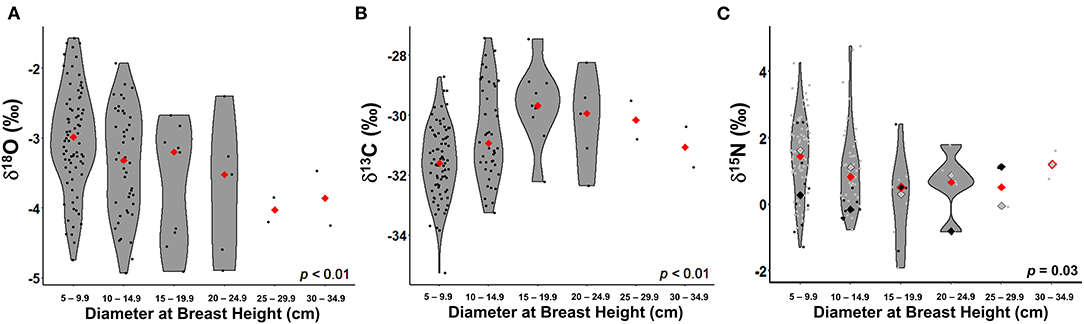
Figure 3. δ18O from stem water (A), leaf δ13C (B), and leaf δ15N (C) by diameter at breast height, ranging from 5 to 35 cm, in 5-cm increments. The overall mean (red diamond) is shown for each size class. Legumes are included in the δ15N analysis. Legumes and the associated mean for each size class are denoted as black circles and black diamonds, respectively, while non-legumes and the associated mean are denoted as gray circles and diamonds, respectively.
With regards to leaf δ13C, as DBH increased, δ13C values became increasingly heavy isotope-enriched yet became heavy isotope-depleted after trees reached a certain size, seemingly around 20 cm in diameter (F5, 137 = 5.70, p < 0.01; Figure 3B). Leaf δ15N, evaluated with and without legumes, varied with DBH (F5, 138 = 2.61, p = 0.03 and F5, 115 = 2.90, p = 0.02, respectively; Figure 3C). δ15N had a similar, though inverted, non-linear pattern as δ13C.
Species-Level Differences
Species-level effects were significant for all isotope ratios measured (δ18O: F44, 97 = 1.57, p = 0.03; δ2H: F44, 97 = 1.99, p < 0.01; δ13C: F44, 98 = 3.68, p < 0.01; δ15N: F44, 97 = 6.54, p < 0.01). Focusing specifically on δ18O and δ13C, we evaluated species differences in water use and water use efficiency (Figure 4). Despite differing root habit and functional groups, species with shallow water access (less negative δ18O values) and deep water access (more negative δ18O values) in general had overlapping δ13C [e.g., D. lateriflora, Cordia gerascanthus L., and Pouteria reticulata (Engl.) Eyma]. Variation within species appeared to be attributed to size. For example, N. psychotrioides, V. gaumeri and F. obtusifolia individuals ranged in DBH from 7.3 to 13.1 cm, 7.4 to 14.4 cm, and 8.8 to 19.2 cm, respectively. All three species showed a comparably wide range of δ18O and δ13C. While some species express expected patterns in water use and water use efficiency based on leaf habit, wood density, rooting habit, tree size, and life history (e.g., M. zapota was represented in the largest DBH size class, had roots present in caves, had low δ18O stem water and relatively low δ13C), many do not follow expected patterns (e.g., although S. cubensis is an evergreen species observed above ground only, individuals had low mean δ18O and relatively high δ13C).
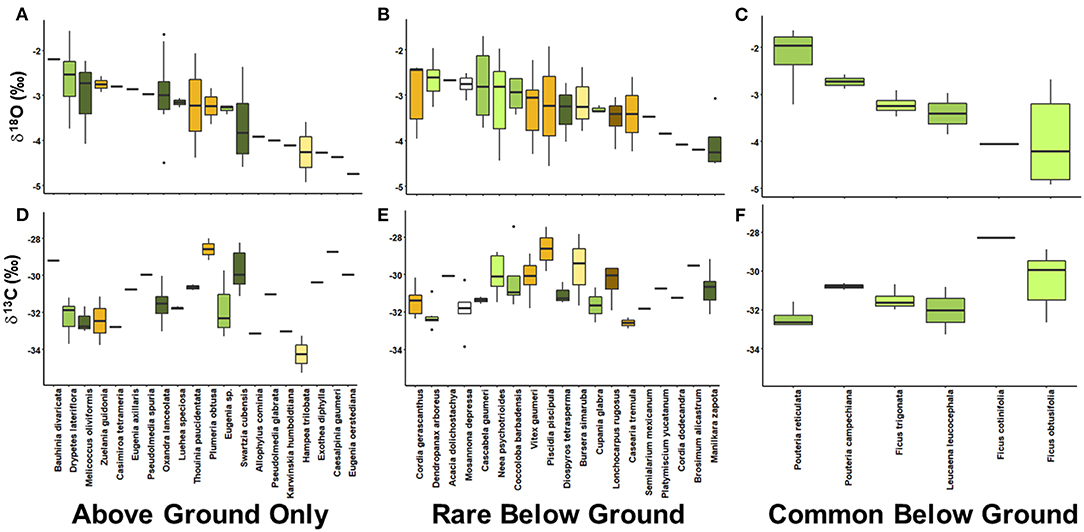
Figure 4. Patterns in by stem water δ18O and leaf δ13C among species in the above ground only (A,D), rare below ground (B,E), and common below ground (C,F) groups. Colors reflect functional groups based on leaf habit (brown shades denote deciduous and green shades denote evergreen) and wood density (light to dark shades denote low to high wood density). Across all species, both δ18O and δ13C are significantly different (δ18O: F44, 97 = 1.57, p = 0.03; δ13C: F44, 98 = 3.68, p < 0.01).
Discussion
The results of our study showed that water utilization and water use efficiency among co-occurring species in Quintana Roo was generally not predicted by rooting habit or functional groups based on leaf habit or wood density, implying that generalizations about rooting depth and resource use strategies based on functional groups may be difficult in this system. Tree size was important, with larger trees in general utilizing deeper water sources than smaller trees. However, deep water use did not correlate with lower water use efficiency. Interestingly, smaller trees and those with shallow water access generally expressed the lowest water use efficiency, contrary to our expectation that these trees would face the greatest water limitation. Our assessment of multiple species allowed us to capture the inter- and intra-specific variation in water access in this karst landscape.
Evergreen and Deciduous Species Have Overlapping Root Zones
Through direct access to roots in caves, we tested expectations about rooting depth and deep water access based on leaf habit (evergreen vs. deciduous). We identified 29 tree species in the caves, consistent with our expectation that many species have access to groundwater via deep roots in this seasonally dry, shallow soil ecosystem (Adams, 2020). Yet, there was tremendous variation among species in this access. Three evergreen species: Ficus spp., Pouteria spp., and L. leucocephala were the most common below ground. While this is consistent with our prediction that evergreens would be most common below ground, a large majority (15 of 22) evergreen species observed above ground were never observed in the caves compared, to only 5 of 15 deciduous species. Therefore, our observations do not support deep rooting specialization by evergreens in general. Rather, our results suggest that evergreen and deciduous species have overlapping root zones with select evergreen species strongly specializing in deep rooting and others (a majority) specializing in shallow rooting. This finding supports observations from other parts of the peninsula that showed no significant division in water sources among deciduous and evergreen species (Querejeta et al., 2007; Hasselquist et al., 2010). A related study on root density in Yucatán found that B. simaruba, a deciduous species, had 25% of its roots in the lowest layers of the bedrock (Estrada-Medina et al., 2013a). Studies on tropical tree seedlings from Bolivia found that the majority of deciduous species had taproots compared to evergreen species (Poorter and Markesteijn, 2008). Further, an investigation of root growth into bedrock under irrigation treatments found that deciduous species responded to drought by increasing root growth into rock fractures (Nie et al., 2017). While these observations were on seedlings in greenhouses, they suggest that deciduous species may have aggressive root foraging strategies, which appear to persist as trees age. These findings combined with our observations suggest that deciduous species are capable of rooting deeply, alongside evergreens in Quintana Roo.
Our findings contrast those from other tropical forests in Venezuela and Panama, which found that evergreens had evidence of deeper water use from water potential measurements and stable isotope assessments (Sobrado, 1986; Jackson et al., 1995; Meinzer et al., 1999). Research on root biomass in Bolivia found that drought-delaying species with conservative traits had deeper root systems compared to acquisitive species (Markesteijn and Poorter, 2009). A potential explanation for this discrepancy may relate to deeper soils in these forests, providing more opportunities for vertical partitioning compared to a karst system, which limits root exploration to pre-existing conduits (Schwinning, 2010; Gao et al., 2016). Therefore, our findings challenge assumptions about rooting depth made from expectations based on leaf habit (Eamus, 1999). Overall, these findings show that multiple species, across leaf habit groups, have potential to access deep water sources in Quintana Roo. Further work on traits that may favor deep rooting specialization in these two groups is warranted.
Tree Size Influences Water Access and Use
Our results showed that tree size was related to water use and water use efficiency. A negative correlation between δ18O values of extracted stem water and tree size confirmed that larger individuals utilized deep water more than smaller individuals. The δ2H of stem water exhibited the same patterns, but the correlation was only marginally significant (see Supplementary Table 6). This may be explained by relatively small differences in sensitivity to soil conditions or extraction methodologies between δ18O and δ2H (Barbeta et al., 2019, 2020; Chen et al., 2020; Allen and Kirchner, 2021; Miguez-Macho and Fan, 2021; Nehemy et al., 2022), although more work is needed in this area. Individuals in the two largest size classes were represented by M. zapota, F. trigonata, B. alicastrum, and L. leucocephala, which all had roots present in the caves, with Ficus spp. and L. leucocephala being common below ground. While both are evergreen, Ficus cotinifolia Kunth was found to have shallow water use in a previous study (Querejeta et al., 2007) and L. leucocephala has been noted as having a more isohydric water use strategy, which might suggest conservative water use and shallower rooting (Liang and Zhang, 1999). M. zapota and B. alicastrum are notable species in mature forests (García-Frapolli et al., 2007) and important to Maya agroforestry, suggesting deep water access may contribute to their persistence during dry periods (Querejeta et al., 2006; Sánchez-Sánchez et al., 2015); however, limited studies have been conducted on water use strategies of these key species (Benjamin et al., 2001; Querejeta et al., 2006). Our findings agree with studies that show, in general, below ground biomass positively correlates with above ground biomass (Schenk and Jackson, 2002; Casper et al., 2003), leading to deeper water use compared to smaller trees with shallower root penetration (Sayer and Newbery, 2003; Hasselquist et al., 2010; Phillips et al., 2010).
Our results further suggest that leaf N isotopes provide insight into rooting depth and compliment tree water source information from water isotope results in this system. In seasonally dry systems, with increased denitrification in surface layers and leaching with high mean annual precipitation in tropical systems (Pataki et al., 2008; Craine et al., 2015; Roa-Fuentes et al., 2015; Campo, 2016), shallow soils may be expected to have available N with higher δ15N values. In addition, in karst soils, N can bind to organic matter in the presence of Ca from the calcium carbonate bedrock (Clarholm et al., 2015; Pan et al., 2016). This decreases plant available N, which may be exacerbated with increasing depth and decreasing soil within bedrock conduits from the surface. Therefore, the smallest trees appear to be accessing shallow sources, as evidenced by relatively heavy-isotope enriched δ15N and δ18O values. Observed depletion of δ15N from the 5 to 9.9 cm DBH group to the 15 to 19.9 cm DBH group may be the result of reduced N availability from leaching and stabilization in soil organic matter at depth. It is crucial to note that the legumes are not driving the depletion among the 15 to 19.9 cm DBH group, as evidenced by a comparable mean δ15N among legumes and non-legumes in this DBH class, even though this size class had the highest fraction of legumes represented. Overall, leaf δ15N appears to indicate rooting depth, providing further support for water access and utilization among individuals of different sizes in our study.
Interestingly, this relationship with tree size and water use does not correspond to other studies conducted in the Yucatán Peninsula. Similar investigations utilizing stable isotopes report no relationship between water source depth and tree size (Querejeta et al., 2006, 2007; Hasselquist et al., 2010; Estrada-Medina et al., 2013b). Rather, it was previously noted that deep water use by small individuals, particularly deciduous species, may be a critical water use strategy during early life stages (Meinzer et al., 1999; Stratton et al., 2000; Hasselquist et al., 2010). Establishing deep roots with reliable water access early on has been suggested to increase survival in seasonally dry environments (Paz et al., 2015). Individuals in the two smallest size classes in our study expressed the full range of δ18O values observed, suggesting more complexity in water use and access, not easily explained by simple variables like tree size. At the same time, our results do suggest that with increasing tree size, variation decreases, with the largest trees relying more heavily on deeper water as a group during the dry season.
Deep Water Use Does Not Equate to Lower Water Use Efficiency
Comparing δ13C to cross-sectional area of individual trees showed an increase in δ13C values with tree size until a threshold, after which δ13C then appeared to decline. This suggests that water use efficiency increases with tree size (suggesting increasing water limitation) until trees reach a certain size, seemingly around 20 cm in diameter in our system, after which efficiency declines (suggesting a concomitant decline in water limitation). As previously discussed, we interpreted the water isotope results as indicating deeper water use by larger trees. We would expect, then, that deeper water access would result in a more stable water source and that would yield lower water use efficiency with increasing tree size. However, this is not the pattern observed. This suggests that while deep water use increases with tree size, complimentary decrease in water use efficiency is not observed until the tree reaches a certain size, suggesting that water limitation in fact increases for all but the very largest trees. We noted that the corollary to this is that smallest trees, without deep water use, had comparable δ13C values to the largest trees. This seems surprising as previous work showed that thin top soil and heterogenous conduits in the bedrock affect the spatial distribution of water in these karstic systems (Estrada-Medina et al., 2013a). Studies from the Edwards Plateau in Texas, USA have shown that evapotranspiration and soil moisture tended to be dynamic near the surface, suggesting short-term and readily depleted soil water storage (McCole and Stern, 2007; Heilman et al., 2014). Yet, the smallest trees (5–9.9 cm DBH) in our system utilizing shallow water appear to withstand these fluctuations in water availability, potentially because the lower water demands by small trees are being met by the soil-filled voids in the limestone (Schwartz et al., 2013; Ding et al., 2018, 2021). On the other hand, medium sized trees (15–19.9 cm DBH and 20–24.9 cm DBH) display high water use efficiency and potential water stress, as their relatively higher water demands are apparently not being satisfied by often low and variable water in the pores. The observed patterns in water use and water use efficiency highlights the range of strategies and suggests that tree size is important to consider when evaluating water use and strategies among co-occurring species. Furthermore, there may be implications for how size distribution among species and with communities may impact responses to disturbance and climate change.
Functional Groups Mask Relevant Species-Specific Water Use Strategies
We predicted that functional groups would inform water use strategies among species in these semi-evergreen tropical forests. Yet, the functions we observed inferred from stable isotopes and direct root observations were not well-predicted by existing groups for these dry tropical forest species. While the functional group framework has been useful for predicting vegetation responses to environmental change (Lavorel and Garnier, 2002; Poorter and Bongers, 2006; Sterck et al., 2006; Maire et al., 2015), some studies in seasonal tropical systems have found that organizing species based on a few leaf or wood traits does not explain variation in responses to water limitation. For example, a study from a tropical dry forest in Costa Rica showed that canopy position and leaf toughness explained variation in leaf δ13C while leaf habit did not (Leffler and Enquist, 2002). In our study, significant variation within groups resulted in a lack of variation between groups, similarly observed by Powers and Tiffin (2010). Therefore, evaluating water use strategies by functional groups masks relevant species variation that could impact community composition and ecosystem functioning, particularly in diverse tropical regions.
Conclusions
The karst topography of the Quintana Roo provided the unique opportunity to evaluate water use strategies among co-occurring species and functional groups through above and below ground observations. Through direct root observation in caves, we showed that rooting depth does not match predictions based solely on functional groups. Evergreen and deciduous species were observed rooting together with overlapping root zones and in fact, a higher fraction of deciduous species appeared to access groundwater than evergreen, although all the species dominant in the caves were evergreens. This finding implies that there is competition for resources and potentially space in the limestone in Quintana Roo and karst landscapes in general. Future work should work to characterize the bedrock matrix as well as soil water content and associated stable isotope values to better describe water availability, water use, and competition within the epikarst. Rooting depth and root abundance did not predict water use efficiency, suggesting that some species can manage resources effectively, even without deep water access. Differences in water use and water use efficiency was better explained by tree size. Progressively larger trees were utilizing deep water, but the trees in the smallest DBH size class had overlapping δ13C values with trees in the largest DBH size class, suggesting water demands of the small individuals were being met by water in shallow soils and bedrock while large trees were successfully accessing groundwater to increase plant performance. This relates to differences among successional stages, with implications for how size distribution among species and within communities may impact responses to disturbance and climate change. Further studies within the region will not only increase sample size but include a wider distribution of tree sizes and species to capture broader community-level patterns. Finally, functional groups did not accurately depict water use strategies among co-occurring species. This framework appears to mask relevant species variation, as observed among the abundant species, that could impact community composition and ecosystem functioning in this seasonally dry region. Therefore, research on species-specific resource acquisition strategies and ecophysiological responses to water availability needs to be on the forefront to properly assess the trajectories of these and other seasonally dry tropical forests in future climate scenarios.
Data Availability Statement
The original contributions presented in the study are included in the article/Supplementary Material, further inquiries can be directed to the corresponding author/s.
Author Contributions
RA and JW designed the research, conducted fieldwork, and wrote the manuscript. RA performed experiments and analyzed data. Both authors contributed to the article and approved the submitted version.
Funding
Research and travel were supported by the Sid Kyle Graduate Merit Assistantship from the Department of Ecology and Conservation Biology (ECCB) at Texas A&M University (TAMU), National Geographic Society Early Career Grant (CP-090ER-17), and the MSC L.T. Jordan Institute for International Awareness at TAMU.
Conflict of Interest
The authors declare that the research was conducted in the absence of any commercial or financial relationships that could be construed as a potential conflict of interest.
Publisher's Note
All claims expressed in this article are solely those of the authors and do not necessarily represent those of their affiliated organizations, or those of the publisher, the editors and the reviewers. Any product that may be evaluated in this article, or claim that may be made by its manufacturer, is not guaranteed or endorsed by the publisher.
Acknowledgments
We greatly appreciate Peter Sprouse of Zara Environmental, LLC for his guidance and hospitality as well as maps and survey data for all sites. We would like to thank the landowners for providing us access to their property to conduct this work. A conversation with Dr. Daniel Breecker prompted us to conduct the 14C analyses. We thank Dr. John Southon from the KCCAMS facility at University of California-Irvine for performing those analyses.
Supplementary Material
The Supplementary Material for this article can be found online at: https://www.frontiersin.org/articles/10.3389/frwa.2022.950346/full#supplementary-material
References
Adams, R. E. (2020). Landscape controls on and physiological benefits of deep rooting by trees in Quintana Roo, Mexico (Dissertation thesis). Texas, USA: Texas A&M University.
Adams, R. E., Hyodo, A., SantaMaria, T., Wright, C.L., Boutton, T.W., and West, J.B. (2020a). Bound and mobile soil water isotope ratios are affected by soil texture and mineralogy, whereas extraction method influences their measurement. Hydrol. Process. 34, 991–1003. doi: 10.1002/hyp.13633
Adams, R. E., Iliffe, T. M., and West, J. B. (2020b). Identifying tree roots in the caves of Quintana Roo, Mexico as a step toward ecological insights and improved conservation. Plants People Planet. 2, 133–139. doi: 10.1002/ppp3.10079
Aerts, R. (1995). The advantages of being evergreen. Trends. Ecol. E10, 402–407. doi: 10.1016/S0169-5347(00)89156-9
Allen, S. T., and Kirchner, J. W. (2021). Potential effects of cryogenic extraction biases on inferences drawn from xylem water deuterium isotope ratios: case studies using stable isotopes to infer plant water sources. Hydrol. Earth Syst. Sci. Discuss. Discuss. 19, 1–5. doi: 10.5194/hess-2020-683
Álvarez-Yépiz, J. C., Búrquez, A., Martínez-Yrízar, A., Teece, M., Yépez, E. A., Dovciak, M., et al. (2017). Resource partitioning by evergreen and deciduous species in a tropical dry forest. Oecologia. 183, 607–618. doi: 10.1007/s00442-016-3790-3
Arturo Sánchez-Azofeifa, G., Quesada, M., Rodriguez, J. P., Nassar, J. M., Stoner, K. E., Castillo, A., et al. (2005). Research priorities for neotropical dry forests. Biotropica. 37, 477–485. doi: 10.1046/j.0950-091x.2001.00153.x-i1
Barbeta, A., Jones, S. P., Clavé, L., Wingate, L., Gimeno, T. E., Fréjaville, B., et al. (2019). Unexplained hydrogen isotope offsets complicate the identification and quantification of tree water sources in a riparian forest. Hydrol. Earth. Syst. Sc. 23, 2129–2146. doi: 10.5194/hess-23-2129-2019
Barbeta, A., Gimeno, T. E., Clavé, L., Fréjaville, B., Jones, S. P., Wingate, L., et al. (2020). An explanation for the isotopic offset between soil and stem water in temperate tree species. New. Phytol. 227, 766–779. doi: 10.1111/nph.16564
Bauer-Gottwein, P., Gondwe, B. R. N. N., Charvet, G., Marín, L. E., Rebolledo-Vieyra, M., Merediz-Alonso, G., et al. (2011). Review: The Yucatán Peninsula karst aquifer, Mexico. Hydrogeol. J. 19, 507–524. doi: 10.1007/s10040-010-0699-5
Becknell, J. M., Kucek, L. K., and Powers, J. S. (2012). Aboveground biomass in mature and secondary seasonally dry tropical forests: A literature review and global synthesis. Forest. Ecol. Manag. 276, 88–95. doi: 10.1016/j.foreco.2012.03.033
Benjamin, T. J., Montanez, P. I., Jimenez, J. J. M., and Gillespie, A. R. (2001). Carbon, water and nutrient flux in Maya homegardens in the Yucatán Peninsula of Mexico. Agroforest. Syst. 53, 103–111. doi: 10.1023/A:1013312217471
Berry, J. A. (1989). “Studies of mechanisms affecting the fractionation of carbon isotopes in photosynthesis”. in Stable Isotopes in Ecological Research, eds P. W. Rundel, J. R. Ehleringer, K. A. Nagy (New York, NY: Springer New York), 82–94.
Bleby, T. M., McElrone, A. J., and Jackson, R. B. (2010). Water uptake and hydraulic redistribution across large woody root systems to 20 m depth. Plant. Cell. Environ. 33, 2132–2148. doi: 10.1111/j.1365-3040.2010.02212.x
Borchert, R. (1994). Soil and stem water storage determine phenology and distribution of tropical dry forest trees. Ecology. 75, 1437–1449. doi: 10.2307/1937467
Campo, J. (2016). Shift from ecosystem P to N limitation at precipitation gradient in tropical dry forests at Yucatán, Mexico. Environ. Res. Lett. 11, 095006. doi: 10.1088/1748-9326/11/9/095006
Casper, B. B., Schenk, H. J., and Jackson, R. B. (2003). Defining a plant's belowground zone of influence. Ecology. 84, 2313–2321. doi: 10.1890/02-0287
Cavender-Bares, J., Ackerly, D. D., Hobbie, S. E., and Townsend, P. A. (2016). Evolutionary legacy effects on ecosystems: biogeographic origins, plant traits, and implications for management in the era of global change. Annu. Rev. Ecol. Evol. Syst. 47, 433–462. doi: 10.1146/annurev-ecolsys-121415-032229
Chave, J., Coomes, D., Jansen, S., Lewis, S. L., Swenson, N. G., Zanne, A. E., et al. (2009). Towards a worldwide wood economics spectrum. Ecol. Lett. 12, 351–366. doi: 10.1111/j.1461-0248.2009.01285.x
Chen, Y., Helliker, B. R., Tang, X., Li, F., Zhou, Y., Song, X., et al. (2020). Stem water cyogenic extraction biases estimation in deuterium isotope composition of plant source water. PNAS. 117, 33345–33350. doi: 10.1073/pnas.2014422117
Clarholm, M., Skyllberg, U., and Rosling, A. (2015). Organic acid induced release of nutrients from metal-stabilized soil organic matter—The unbutton model. Soil. Biol. Biochem. 84, 168–176. doi: 10.1016/j.soilbio.2015.02.019
Craine, J. M., Brookshire, E. N. J., Cramer, M. D., Hasselquist, N. J., Koba, K., Marin-Spiotta, E., et al. (2015). Ecological interpretations of nitrogen isotope ratios of terrestrial plants and soils. Plant. Soil. 396, 1–26. doi: 10.1007/s11104-015-2542-1
Derroire, G., Powers, J. S., Hulshof, C. M., Cárdenas Varela, L. E., and Healey, J. R. (2018). Contrasting patterns of leaf trait variation among and within species during tropical dry forest succession in Costa Rica. Sci. Rep-UK. 8, 1–11. doi: 10.1038/s41598-017-18525-1
Ding, Y., Nie, Y., Chen, H., Wang, K., and Querejeta, J. I. (2021). Water uptake depth is coordinated with leaf water potential, water use efficiency and drought vulnerability in karst vegetation. New. Phytol. 229, 1339–1353. doi: 10.1111/nph.16971
Ding, Y., Nie, Y., Schwinning, S., Chen, H., Yang, J., Zhang, W., et al. (2018). A novel approach for estimating groundwater use by plants in rock-dominated habitats. J. Hydrol. 565, 760–769. doi: 10.1016/j.jhydrol.2018.08.033
Doody, T. M., and Benyon, R. G. (2011). Direct measurement of groundwater uptake through tree roots in a cave. Ecohydrology. 4, 644–649. doi: 10.1002/eco.152
DRYFLOR (2016). Plant diversity patterns in neotropical dry forests and their conservation implications. Science. 353, 1383–1387. doi: 10.1126/science.aaf5080
Durán-García, R., Méndez-Gonzalez, M., and Larqué-Saavedra, A. (2016). The biodiversity of the Yucatán Peninsula: a natural laboratory. Prog. Bot. 78, 237–258. doi: 10.1007/124_2016_8
Eamus, D. (1999). Ecophysiological traits of deciduous and evergreen woody species in the seasonally dry tropics. Trends. Ecol. E14, 11–16. doi: 10.1016/S0169-5347(98)01532-8
Ehleringer, J. R., and Dawson, T. E. (1992). Water uptake by plants: perspectives from stable isotope composition. Plant. Cell. Environ. 15, 1073–1082. doi: 10.1111/j.1365-3040.1992.tb01657.x
Ellsworth, P. Z., and Sternberg, L. S. L. (2015). Seasonal water use by deciduous and evergreen woody species in a scrub community is based on water availability and root distribution. Ecohydrology. 8, 538–551. doi: 10.1002/eco.1523
Estrada-Medina, H., Graham, R. C., Allen, M. F., Jiménez-Osornio, J. J., and Robles-Casolco, S. (2013a). The importance of limestone bedrock and dissolution karst features on tree root distribution in northern Yucatán, México. Plant. Soil. 362, 37–50. doi: 10.1007/s11104-012-1175-x
Estrada-Medina, H., Santiago, L. S., Graham, R. C., Allen, M. F., and Jiménez-Osornio, J. J. (2013b). Source water, phenology and growth of two tropical dry forest tree species growing on shallow karst soils. Trees. 27, 1297–1307. doi: 10.1007/s00468-013-0878-9
Fragoso-Servón, P., Pereira Corona, A., Bautista Zúñiga, F., Prezas Hernández, B., and Reyes, N. A. (2020). Soils in extreme conditions: the case of the catenas karst-marsh-coastline in the Mexican Caribbean. Bol. Soc. Geol. Mex. 72, 00011. doi: 10.18268/BSGM2020v72n2a040619
Freschet, G. T., Swart, E. M., and Cornelissen, J. H. C. (2015). Integrated plant phenotypic responses to contrasting above- and below-ground resources: key roles of specific leaf area and root mass fraction. New. Phytol. 206, 1247–1260. doi: 10.1111/nph.13352
Gao, W., Hodgkinson, L., Jin, K., Watts, C. W., Ashton, R. W., Shen, J., et al. (2016). Deep roots and soil structure. Plant. Cell. Environ. 39, 1662–1668. doi: 10.1111/pce.12684
García-Frapolli, E., Ayala-Orozco, B., Bonilla-Moheno, M., Espadas-Manrique, C., and Ramos-Fernández, G. (2007). Biodiversity conservation, traditional agriculture and ecotourism: Land cover/land use change projections for a natural protected area in the northeastern Yucatán Peninsula, Mexico. Landscape. Urban. Plan. 83, 137–153. doi: 10.1016/j.landurbplan.2007.03.007
Geekiyanage, N., Goodale, U. M., Cao, K., and Kitajima, K. (2019). Plant ecology of tropical and subtropical karst ecosystems. Biotropica 51, 626–640. doi: 10.1111/btp.12696
Givnishn, T. (2002). Adaptive significance of evergreen vs. deciduous leaves: solving the triple paradox. Silva. Fenn. 36, 703–743. doi: 10.14214/sf.535
Hang, H., and Wu, Y. (2016). Quantification of photosynthetic inorganic carbon utilisation via a bidirectional stable carbon isotope tracer. Acta. Geochim. 35, 130–137. doi: 10.1007/s11631-015-0088-9
Hasselquist, N. J., Allen, M. F., and Santiago, L. S. (2010). Water relations of evergreen and drought-deciduous trees along a seasonally dry tropical forest chronosequence. Oecologia. 164, 881–890. doi: 10.1007/s00442-010-1725-y
Heilman, J. L., Litvak, M. E., Mcinnes, K. J., Kjelgaard, J. F., Kamps, R. H., Schwinning, S., et al. (2014). Water-storage capacity controls energy partitioning and water use in karst ecosystems on the Edwards Plateau, Texas. Ecohydrology. 7, 127–138. doi: 10.1002/eco.1327
Henry, C., John, G. P., Pan, R., Bartlett, M. K., Fletcher, L. R., Scoffoni, C., et al. (2019). A stomatal safety-efficiency trade-off constrains responses to leaf dehydration. Nat. Commun. 10, 3398. doi: 10.1038/s41467-019-11006-1
Hulshof, C. M., and Swenson, N. G. (2010). Variation in leaf functional trait values within and across individuals and species: an example from a Costa Rican dry forest. Funct. Ecol. 24, 217–223. doi: 10.1111/j.1365-2435.2009.01614.x
Ibarra-Manríquez, G., Villaseñor, J. L., Durán, R., and Meave, J. (2002). Biogeographical analysis of the tree flora of the Yucatán Peninsula. J. Biogeogr. 29, 17–29. doi: 10.1046/j.1365-2699.2002.00648.x
Jackson, P. C., Cavelier, J., Goldstein, G., Meinzer, F. C., and Holbrook, N. M. (1995). Partitioning of water resources among plants of a lowland tropical forest. Oecologia. 101, 197–203. doi: 10.1007/BF00317284
Kitajima, K., and Poorter, L. (2008). “Functional basis for resource niche partitioning by tropical trees,” in Tropical Forest Community Ecology, eds W. P. Carson, S.A. Schnitzer. (Hoboken, NJ: Wiley), 160–181.
Kleinschmidt, S., Wanek, W., Kreinecker, F., Hackl, D., Jenking, D., Weissenhofer, A., et al. (2020). Successional habitat filtering of rainforest trees is explained by potential growth more than by functional traits. Funct. Ecol. 34, 1438–1447. doi: 10.1111/1365-2435.13571
Lavorel, S., and Garnier, E. (2002). Predicting changes in community composition and ecosystem functioning from plant traits: revisiting the Holy Grail. Funct. Ecol. 16, 545–556. doi: 10.1046/j.1365-2435.2002.00664.x
Leffler, A. J., and Enquist, B. J. (2002). Carbon isotope composition of tree leaves from Guanacaste, Costa Rica: comparison across tropical forests and tree life history. J. Trop. Ecol. 18, 151–159. doi: 10.1017/S0266467402002109
Liang, J., and Zhang, J. (1999). The relations of stomatal closure and reopening to xylem ABA concentration and leaf water potential during soil drying and rewatering. Plant. Growth. Regul. 29, 77–86. doi: 10.1023/A:1006207900619
Maire, V., Wright, L. J., Prentice, I. C., Batjes, N. H., Bhaskar, R., van Bodegom, P. M., et al. (2015). Global effects of soil and climate on leaf photosynthetic traits and rates: Effects of soil and climate on photosynthetic traits. Global Ecol. Biogeogr. 24, 706–717. doi: 10.1111/geb.12296
Markesteijn, L., and Poorter, L. (2009). Seedling root morphology and biomass allocation of 62 tropical tree species in relation to drought- and shade-tolerance. J. Ecol. 97, 311–325. doi: 10.1111/j.1365-2745.2008.01466.x
Markesteijn, L., Poorter, L., Bongers, F., Paz, H., and Sack, L. (2011). Hydraulics and life history of tropical dry forest tree species: coordination of species' drought and shade tolerance. New. Phytol. 191, 480–495. doi: 10.1111/j.1469-8137.2011.03708.x
McCole, A. A., and Stern, L. A. (2007). Seasonal water use patterns of Juniperus ashei on the Edwards Plateau, Texas, based on stable isotopes in water. J. Hydrol. 342, 238–248. doi: 10.1016/j.jhydrol.2007.05.024
McCormack, M. L., Dickie, I. A., Eissenstat, D. M., Fahey, T. J., Fernandez, C. W., Guo, D., et al. (2015). Redefining fine roots improves understanding of below-ground contributions to terrestrial biosphere processes. New. Phytol. 207, 505–518. doi: 10.1111/nph.13363
McDowell, N., Pockman, W. T., Allen, C. D., Breshears, D. D., Cobb, N., Kolb, T., et al. (2008). Mechanisms of plant survival and mortality during drought: why do some plants survive while others succumb to drought? New. Phytol. 178, 719–739. doi: 10.1111/j.1469-8137.2008.02436.x
Meinzer, C. F., Andrade, L. J., Goldstein, G., Holbrook, M. N., Cavelier, J., Wright, J. S., et al. (1999). Partitioning of soil water among canopy trees in a seasonally dry tropical forest. Oecologia. 121, 293–301. doi: 10.1007/s004420050931
Méndez-Alonzo, R., Paz, H., Zuluaga, R. C., Rosell, J. A., and Olson, M. E. (2012). Coordinated evolution of leaf and stem economics in tropical dry forest trees. Ecology. 93, 2397–2406. doi: 10.1890/11-1213.1
Miguez-Macho, G., and Fan, Y. (2021). Spatiotemporal origin of soil water taken up by vegetation. Nature. 598, 624–628. doi: 10.1038/s41586-021-03958-6
Mitchell, P. J., Veneklaas, E. J., Lambers, H., and Burgess, S. S. O. (2008). Leaf water relations during summer water deficit: Differential responses in turgor maintenance and variation in leaf structure among different plant communities in south-western Australia. Plant. Cell. Environ. 31, 1791–1802. doi: 10.1111/j.1365-3040.2008.01882.x
Nardini, A., Casolo, V., Dal Borgo, A., Savi, T., Stenni, B., Bertoncin, P., et al. (2016). Rooting depth, water relations and non-structural carbohydrate dynamics in three woody angiosperms differentially affected by an extreme summer drought. Plant. Cell. Environ. 39, 618–627. doi: 10.1111/pce.12646
Nehemy, M. F., Benettin, P., Allen, S. T., Steppe, K., Rinaldo, A., Lehmann, M. M., et al. (2022). Phloem water isotopically different to xylem water: potential causes and implications for ecohydrological tracing. Ecohydrology. 15, e2417. doi: 10.1002/eco.2417
Nepstad, D. C., de Carvalho, C. R., Davidson, E. A., Jipp, P. H., Lefebvre, P. A., Negroiros, G. A., et al. (1994). The role of deep roots in the hydrological cycles of Amazonian forest and pastures. Nature. 372, 666–669. doi: 10.1038/372666a0
Nie, Y., Chen, H., Ding, Y., Yang, J., and Wang, K. (2017). Comparison of rooting strategies to explore rock fractures for shallow soil-adapted tree species with contrasting aboveground growth rates: a greenhouse microcosm experiment. Front. Plant. Sci. 8, 1651. doi: 10.3389/fpls.2017.01651
Orlowski, N., Breuer, L., and McDonnell, J. J. (2016). Critical issues with cryogenic extraction of soil water for stable isotope analysis: issues with cryogenic soil water extraction. Ecohydrology. 9, 3–10. doi: 10.1002/eco.1722
Pan, F., Liang, Y., Zhang, W., Zhao, J., and Wang, K. (2016). Enhanced nitrogen availability in karst ecosystems by oxalic acid release in the rhizosphere. Front. Plant. Sci. 7, 687. doi: 10.3389/fpls.2016.00687
Pataki, D. E., Billings, S. A., Naumburg, E., and Goedhart, C. M. (2008). Water sources and nitrogen relations of grasses and shrubs in phreatophytic communities of the Great Basin Desert. J. Arid. Environ. 72, 1581–1593. doi: 10.1016/j.jaridenv.2008.03.011
Paz, H., Pineda-García, F., and Pinzón-Pérez, L. F. (2015). Root depth and morphology in response to soil drought: comparing ecological groups along the secondary succession in a tropical dry forest. Oecologia. 179, 551–561. doi: 10.1007/s00442-015-3359-6
Phillips, O. L., van der Heijden, G., Lewis, S. L., López-González, G., Aragão, L. E. C. O., Lloyd, J., et al. (2010). Drought-mortality relationships for tropical forests. New. Phytol. 187, 631–646. doi: 10.1111/j.1469-8137.2010.03359.x
Pierret, A., Maeght, J. L., Clément, C., Montoroi, J. P., Hartmann, C., Gonkhamdee, S., et al. (2016). Understanding deep roots and their functions in ecosystems: an advocacy for more unconventional research. Ann. Bot.-London. 118, 621–635. doi: 10.1093/aob/mcw130
Poorter, L., and Bongers, F. (2006). Leaf traits are good predictors of plant performance across 53 rain forest species. Ecology. 87, 1733–1743. doi: 10.1890/0012-9658(2006)87(1733:LTAGPO)2.0.CO;2
Poorter, L., and Markesteijn, L. (2008). Seedling traits determine drought tolerance of tropical tree species. Biotropica. 40, 321–331. doi: 10.1111/j.1744-7429.2007.00380.x
Powers, J. S., and Tiffin, P. (2010). Plant functional type classifications in tropical dry forests in Costa Rica: leaf habit vs. taxonomic approaches: leaf habit and functional traits of dry forest trees. Funct. Ecol. 24, 927–936. doi: 10.1111/j.1365-2435.2010.01701.x
Querejeta, J. I., Estrada-Medina, H., Allen, M. F., and Jiménez-Osornio, J. J. (2007). Water source partitioning among trees growing on shallow karst soils in a seasonally dry tropical climate. Oecologia. 152, 26–36. doi: 10.1007/s00442-006-0629-3
Querejeta, J. I., Estrada-Medina, H., Allen, M. F., Jiménez-Osornio, J. J., and Ruenes, R. (2006). Utilization of bedrock water by Brosimum alicastrum trees growing on shallow soil atop limestone in a dry tropical climate. Plant. Soil. 287, 187–197. doi: 10.1007/s11104-006-9065-8
R Core Team. (2019). R: A language and environment for statistical computing. Vienna: R Foundation for Statistical Computing. Available online at: https://www.R-project.org/
Rao, S., and Wu, Y. (2017). Root-derived bicarbonate assimilation in response to variable water deficit in Camptotheca acuminate seedlings. Photosynth. Res. 134, 59–70. doi: 10.1007/s11120-017-0414-7
Reich, P. B. (2014). The world-wide ‘fast-slow’ plant economics spectrum: a traits manifesto. J. Ecol. 102, 275–301. doi: 10.1111/1365-2745.12211
Roa-Fuentes, L. L., Templer, P. H., and Campo, J. (2015). Effects of precipitation regime and soil nitrogen on leaf traits in seasonally dry tropical forests of the Yucatán Peninsula, Mexico. Oecologia. 179, 585–597. doi: 10.1007/s00442-015-3354-y
Roumet, C., Birouste, M., Picon-Cochard, C., Ghestem, M., Osman, N., Vrignon-Brenas, S., et al. (2016). Root structure-function relationships in 74 species: evidence of a root economics spectrum related to carbon economy. New. Phytol. 210, 815–826. doi: 10.1111/nph.13828
RStudio Team. (2019). RStudio: Integrated Development for R. RStudio, Inc., Boston, MA. Available online at: http://www.rstudio.com/
Sánchez-Sánchez, O., and Islebe, G. A. (2002). Tropical forest communities in Southeastern Mexico. Plant. Ecol. 158, 183–200. doi: 10.1023/A:1015509832734
Sánchez-Sánchez, O., Islebe, G. A., Ramírez-Barajas, P. J., and Torrescano-Valle, N. (2015). “Natural and human induced disturbance in vegetation,” in Biodiversity and Conservation of the Yucatán Peninsula, ed G. A. Islebe, S. Calmé, J. L. León-Cortés, and B. Schmook. (Cham, Switzerland: Springer International Publishing), 153–167.
Santiago, L. S., Kitajima, K., Wright, S. J., and Mulkey, S. S. (2004). Coordinated changes in photosynthesis, water relations and leaf nutritional traits of canopy trees along a precipitation gradient in lowland tropical forest. Oecologia. 139, 495–502. doi: 10.1007/s00442-004-1542-2
Santiago, L. S., Silvera, K., Andrade, J. L., and Dawson, T. E. (2017). Functional strategies of tropical dry forest plants in relation to growth form and isotopic composition. Environ. Res. Lett. 12, 115006. doi: 10.1088/1748-9326/aa8959
Sayer, E. J., and Newbery, D. M. (2003). The role of tree size in the leafing phenology of a seasonally dry tropical forest in Belize, Central America. J. Trop. Ecol. 19, 539–548. doi: 10.1017/S0266467403003596
Schenk, H. J., and Jackson, R. B. (2002). Rooting depths, lateral root spreads and belowground aboveground allometries of plants in water limited ecosystems. J. Ecol. 90, 480–494. doi: 10.1046/j.1365-2745.2002.00682.x
Schwartz, B. F., Schwinning, S., Gerard, B., Kukowski, K. R., Stinson, C. L., Dammeyer, H. C., et al. (2013). Using hydrogeochemical and ecohydrologic responses to understand epikarst process in semi-arid systems, Edwards Plateau, Texas, USA. Acta. Carsologica. 42, 315–325. doi: 10.3986/ac.v42i2-3.670
Schwinning, S. (2010). The ecohydrology of roots in rocks. Ecohydrology. 3, 238–245. doi: 10.1002/eco.134
Smart, L. P., Beddows, P. A., Coke, J., Doerr, S., Smith, S., Whitaker, F. F., et al. (2006). “Cave development on the Caribbean coast of the Yucatán Peninsula, Quintana Roo, Mexico,” in Perspectives on Karst Geomorphology, Hydrology, and Geochemistry – A Tribute Volume to Derek C. Ford and William B. White, eds. R.S. Harmon and C.M. Wicks (Boulder, CO: Geological Society of America), 105–128.
Sobrado, M. A. (1986). Aspects of tissue water relations and seasonal changes of leaf water potential components of evergreen and deciduous species coexisting in tropical dry forests. Oecologia. 68, 413–416. doi: 10.1007/BF01036748
Sperry, J. S. (2003). The evolution of water transport and xylem structure. Int. J. Plant Sci. 164, S115–S127. doi: 10.1086/368398
Sterck, F. J., Poorter, L., and Schieving, F. (2006). Leaf traits determine the growth-survival trade-off across rain forest tree species. Am. Nat. 167, 758–765. doi: 10.1086/503056
Steudle, E. (2001). The cohesion-tension mechanism and the acquisition of water by plant roots. Annu. Rev. Plant Biol. 58, 847–875. doi: 10.1146/annurev.arplant.52.1.847
Stratton, L. C., Goldstein, G., and Meinzer, F. C. (2000). Temporal and spatial partitioning of water resources among eight woody species in a Hawaiian dry forest. Oecologia. 124, 309–317. doi: 10.1007/s004420000384
Stuiver, M., and Polach, H. A. (1977). Reporting of 14C data. Radiocarbon. 19, 355–363. doi: 10.1017/S0033822200003672
Swaffer, B. A., Holland, K. L., Doody, T. M., Li, C., and Hutson, J. (2014). Water use strategies of two co-occurring tree species in a semi-arid karst environment. Hydrol. Process. 28, 2003–2017. doi: 10.1002/hyp.9739
Trimmis, K. P. (2018). Paperless mapping and cave archaeology: a review on the application of DistoX survey method in archaeological cave sites. J. Archaeol. Sci. 18, 399–407. doi: 10.1016/j.jasrep.2018.01.022
Trueba, S., Pouteau, R., Lens, F., Field, T. S., Isnard, S., Olson, M. E., et al. (2017). Vulnerability to xylem embolism as a major correlate of the environmental distribution of rain forest species on a tropical island. Plant. Cell. Environ. 40, 277–289. doi: 10.1111/pce.12859
van Hengstum, P. J., Reinhardt, E. G., Beddows, P. A., and Gabriel, J. J. (2010). Linkages between Holocene paleoclimate and paleohydrogeology preserved in a Yucatán underwater cave. Quaternary Sci. Rev. 29, 2788–2798. doi: 10.1016/j.quascirev.2010.06.034
Weemstra, M., Mommer, L., Visser, E. J. W., van Ruijven, J., Kuyper, T. W., Mohren, G. M. J., et al. (2016). Towards a multidimensional root trait framework: a tree root review. New. Phytol. 211, 1159–1169. doi: 10.1111/nph.14003
Werden, L. K., Waring, B. G., Smith-Martin, C. M., and Powers, J. S. (2018). Tropical dry forest trees and lianas differ in leaf economic spectrum traits but have overlapping water-use strategies. Tree. Physiol. 38, 517–530. doi: 10.1093/treephys/tpx135
West, A. G., Patrickson, S. J., and Ehleringer, J. R. (2006). Water extraction times for plant and soil materials used in stable isotope analysis. Rapid Commun. Mass. Sp. 20, 1317–1321. doi: 10.1002/rcm.2456
Wright, I. J., Reich, P. B., Westoby, M., Ackerly, D. D., Baruch, Z., Bongers, F., et al. (2004). The worldwide leaf economics spectrum. Nature. 428, 821–827. doi: 10.1038/nature02403
Xu, X., Medvigy, D., Powers, J. S., Becknell, J. M., and Guan, K. (2016). Diversity in plant hydraulic traits explains seasonal and inter-annual variations of vegetation dynamics in seasonally dry tropical forests. New. Phytol. 212, 80–95. doi: 10.1111/nph.14009
Yamauchi, T., Pedersen, O., Nakazono, M., and Tsutsumi, N. (2021). Key root traits of Poaceae for adaptation to soil water gradients. New. Phytol. 229, 3133–3140. doi: 10.1111/nph.17093
Keywords: seasonally dry tropical forest, water use strategies, functional groups, stable isotopes, ecophysiology
Citation: Adams RE and West JB (2022) Functional Groups Mask Inter- and Intraspecific Variation in Water Use Strategies in a Seasonally Dry Tropical Forest. Front. Water 4:950346. doi: 10.3389/frwa.2022.950346
Received: 22 May 2022; Accepted: 20 June 2022;
Published: 07 July 2022.
Edited by:
Yunpeng Nie, Institute of Subtropical Agriculture (CAS), ChinaReviewed by:
Wenjie Liu, Xishuangbanna Tropical Botanical Garden (CAS), ChinaJing Yang, Guizhou University, China
Copyright © 2022 Adams and West. This is an open-access article distributed under the terms of the Creative Commons Attribution License (CC BY). The use, distribution or reproduction in other forums is permitted, provided the original author(s) and the copyright owner(s) are credited and that the original publication in this journal is cited, in accordance with accepted academic practice. No use, distribution or reproduction is permitted which does not comply with these terms.
*Correspondence: Rachel E. Adams, cmVyYWRhbXNAZ21haWwuY29t
†These authors have contributed equally to this work and share first authorship