- 1Institute for Water Research, Rhodes University, Makhanda, South Africa
- 2Freshwater Research Centre South Africa, Cape Town, South Africa
The Ecological Reserve (environmental flows) as defined under the South African National Water Act 36 of 1998 was designed to equitably manage water for river sustainability while maximizing economic and social welfare. We investigated the climate change impacts on the Ecological Reserve targets for a seasonal river in an agricultural catchment in the Western Cape region using the Habitat Flow Stressor Response method (which integrates hydrology, hydraulics, water quality, and ecological data) under projected climate scenarios (2041–2070). Current Ecological Reserve model outcomes for 3 sites on the Doring River were compared with the future hydrology using Global Circulation Models associated with four Representative Concentration Pathways (RCP 2.6–8.5). Climate predictions reflected reduced future flows, but the uncertainty band of predicted future flows overlapped with present day flows. Flood flashiness following heavy rains and the increased length of dry periods that are predicted would both contribute to increased erosion and geomorphological degradation, and further compromise biodiversity including the endangered fish populations and threaten both freshwater and estuarine ecosystems downstream. Salinity variation is predicted to increase in future leading to increased seasonal salinity stress and reduced use of abstracted water. We interrogate various options for mitigating the impacts including augmenting dry season flows, developing on-farm, catchment-scale, and strategic water resources management, and removing alien vegetation.
Introduction
Achieving the United Nations Sustainable Development Goals (SDG) 6.4 and 6.6 relating to stress on freshwater resources requires evaluating and enhancing the adaptive capacity of riverine ecosystems while meeting the demands for water withdrawal for food security. The Ecological Reserve (environmental flows) as defined under the South African National Water Act (no. 36 of 1998) provides a means of managing water in such a way as to maximize economic and social welfare in an equitable manner without compromising the sustainability of ecosystems and the services they provide (Palmer, 1999; van Wyk et al., 2006). Climate change, however, poses a significant potential threat to this allocation of water and hence the sustainability of aquatic ecosystems and ecosystem services to society (Kundzewicz et al., 2007; Palmer et al., 2009). This is largely due to substantial uncertainty in terms of rainfall scenarios i.e., how it will differ across South Africa and what the subsequent long-term hydrological and water quality changes will be, together with the implications for the Ecological Reserve in different parts of the country. The changes in the quantity of water in these ecosystems are due to changes in runoff patterns, frequency and intensity of extreme events (e.g., droughts and flooding) and groundwater recharge rates (Dallas and Rivers-Moore, 2014; Leigh et al., 2015).
A change in the hydrological character and regime of aquatic ecosystems due to climate change can trigger a chain of cascading effects with subsequent intrinsic changes that will be observed in the different components of these ecosystems (water quality, instream and riparian habitat and instream biological communities), and overall in its functioning (Palmer et al., 2009; Dallas and Rivers-Moore, 2014; Leigh et al., 2015). Managing and meeting the Ecological Reserve within the current complexities and constraints posed by climate change, is of utmost importance in ensuring the long-term sustainable management of these resources and to contain their widespread degradation. Our research targets the knowledge gap of the impacts of climate change on Ecological Reserve targets in the Doring River, a seasonal river with several indigenous fish and significant farming activity in the Western Cape region of South Africa under projected climate scenarios (2041–2070).
Water is central to socio-economic development, agricultural production and food security in this catchment. Given a likely reduction in rainfall and streamflow, some impacts are likely, both on the riverine biota and on farming and other activities. Currently, the river system is fully allocated and under stress, resulting in the Ecological Reserve not always being met. This stress is impacting the resilience of the river. Further system stress in the form of altered hydrology due to climate change will result in current catchment activities becoming untenable. Thus, this research aimed to reduce risks, promote principles of sustainability and address emerging issues with regards to climate change in a water-stressed region.
The paper has three main objectives:
a. Assess the resulting impacts of the increased variability on river hydrology.
b. Determine the impact of climate change on the Ecological Reserve for the Doring River.
c. Evaluate adaptive responses to balance the river sustainability with demand for water abstraction for agriculture.
Materials and methods
The Doring, or Doorn, River is located in the Western Cape Province, South Africa (Figure 1A) and forms part of the Olifants-Doring river system, being the main tributary of the Olifants River. It rises in the south and flows in a northerly direction, draining the eastern slopes of the Cedarberg, the Swartruggens and the western Roggeveld Mountains. The Doring catchment is situated in a winter rainfall area, which is naturally arid as a significant proportion of the catchment receives <200 mm rain y−1 (Brown et al., 2006; Figure 1C). It is a seasonal river whose flow varies considerably, with high flows in winter, whereas in summer the river is reduced to a chain of pools. It receives 2.5 million m3 y−1 of water in the upper reaches via the Inverdoorn Canal from the Breede River Catchment [Department of Water Affairs and Forestry (DWAF), 2004].
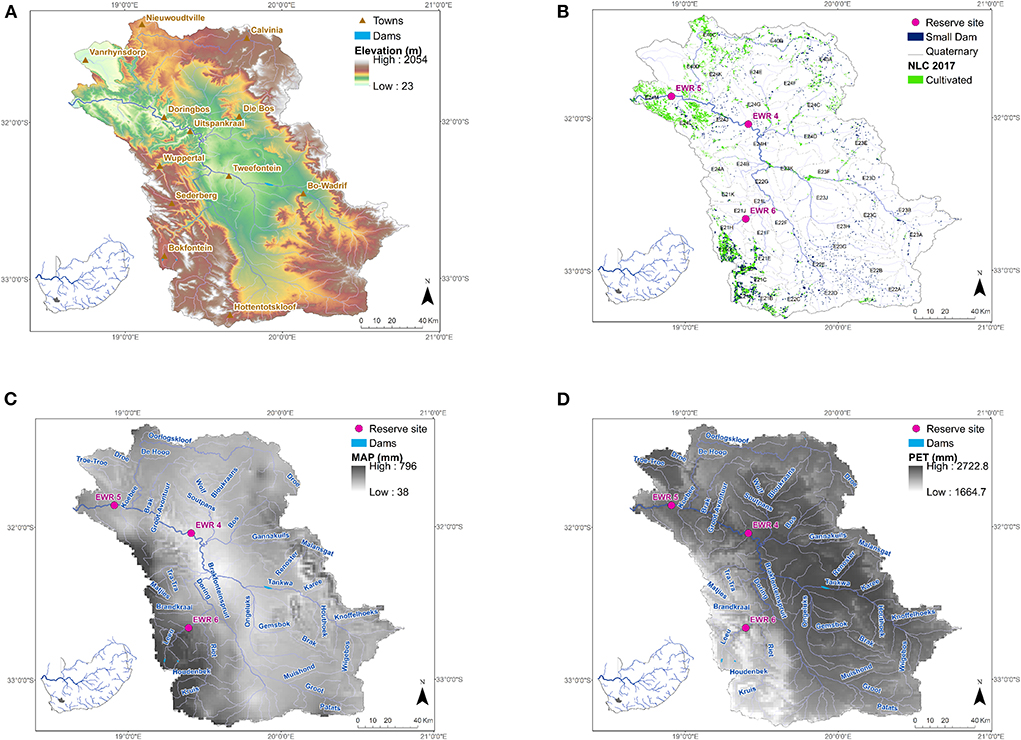
Figure 1. Doring River catchment showing (A) the elevation and location of rivers and towns, and (B) the location of cultivated areas [obtained from 2017 National Land Cover; Department of Environmental Affairs (2018)], the quaternary catchments (fourth-order primary management areas) and Ecological Reserve sites. (C) Mean Annual Precipitation (MAP) (D) and Potential Evapotranspiration (PET) for the catchment from the South African Atlas of Climatology and Agrohydrology (Schulze, 2007).
The study area supports eleven indigenous fish species, nine of which are endemic to the catchment. In recent decades, a decline in the abundances and contraction in the geographical ranges of these species have been attributed to the introduction and translocation of five alien and four extralimital fish species into the Olifants-Doring River system over the last century. Together with the over-abstraction of water from rivers to support agriculture, predation by and competition from the introduced species has led to all the endemic species being listed by the IUCN as threatened (Paxton et al., 2002). Currently, of the nine endemic species, four are considered Near Threatened (NT), three Endangered (EN) and two are Critically Endangered (CR) (Ellender et al., 2017). The area also supports an unusual macroinvertebrate fauna, with several local endemics, not all of which have been described as species (de Moor, 2011). The land use is largely agricultural (Figure 1B), with cultivation of citrus and deciduous fruits where water is plentiful, and livestock farming in the drier parts of the catchment. The potential evaporation is more than an order of magnitude higher than the rainfall over most of the catchment (Figures 1C,D). The rainfall is variable across the catchment with high rainfall areas primarily in the south-west of the catchment (Figure 1C). Water use is completely dominated by the irrigation sector. Abstraction for agriculture results in a delayed onset and shorter duration of the wet season and significantly reduced baseflows, impacting endangered fish populations and threatening both freshwater and estuarine ecosystems downstream. A significant increase in the wetland plant Palmiet (Prionium serratum) in recent years is linked to reduced river flows allowing the palmiet plant to propagate more successfully. Currently, the river water quality is suitable for agriculture and domestic water supplies. However, at the end of summer, water quality deteriorates and high salinity linked to the geology and return flows from irrigation results in some farmers curtailing irrigation.
For this study we used the South African Revised Desktop Reserve Model (RDRM) (Hughes et al., 2014) based on the Habitat Flow Stressor Response (Hughes and Louw, 2010) method, which integrates hydrology, hydraulics (limited), water quality and ecological data for determining the Ecological Reserve and evaluating different flow management options, considering that low flows are of particular concern (Nilsson and Renöfält, 2008; Opperman et al., 2018). The RDRM is designated a Level 1 flow assessment method in a review of methods to determine Environmental flows by Opperman et al. (2018). A level 1 assessment is defined as a desktop analysis based on existing data usually undertaken by one or a few scientists. The model was set up for three ecological water requirement (EWR) sites (sites 4, 5 and 6; Figure 1C) in the catchment using the natural hydrology. Site 6 lies at Mount Cedar on the Groot River in the Kouebokkeveld in the upper catchment of the Doring River. Ecological Reserve site 4 lies at Uitspankraal on the Doring River at the confluence with the Biedou River in the middle catchment of the Doring River. The region is arid, and the Doring River at this point has received saline inputs from the Tankwa Karroo, primarily via the Tankwa and Wolf rivers. Ecological Reserve site 5 is at Oudrif on the Doring River, in the Lower Doring River catchment ~30 km upstream of the confluence with the Olifants River.
Each site was assigned an ecological integrity score using the South African Ecostatus models (Kleynhans and Louw, 2008) which describes an ecosystem state in terms of the principal drivers of aquatic ecosystem change (hydrology, geomorphology, and physico-chemical properties) and response variables (fish, aquatic invertebrates, and riparian vegetation), with the Ecological Category (A) signifying an unmodified or natural state and category (D) signifying a critically or extremely modified condition (Table 1). Categories E and F were in use historically but are not currently used in South Africa, with category D used to represent the lower limit of an extremely modified condition. The current state for the sites is B for sites 4 and 5 and B/C for 6 (upper most).
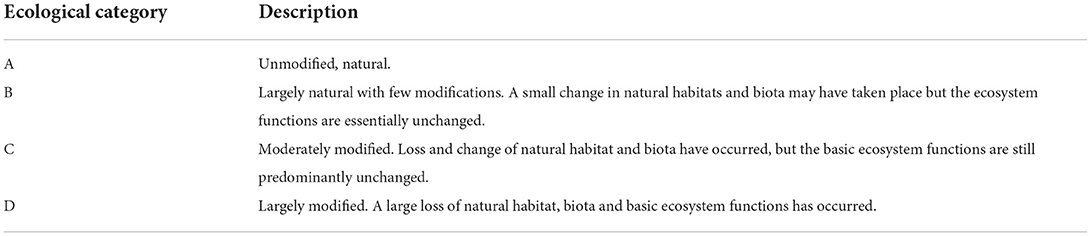
Table 1. Ecological categories as defined by the South African Ecostatus suite of models Kleynhans and Louw (2008).
Figure 2 shows the modeling framework that was developed (briefly described below) and adopted by the study to evaluate the future climate data and uncertainty for generating hydrological outputs, described in detail in a South African Water Research Commission funded report titled “Impacts of climate change in determining the ecological Reserve” (Tanner et al., 2020).
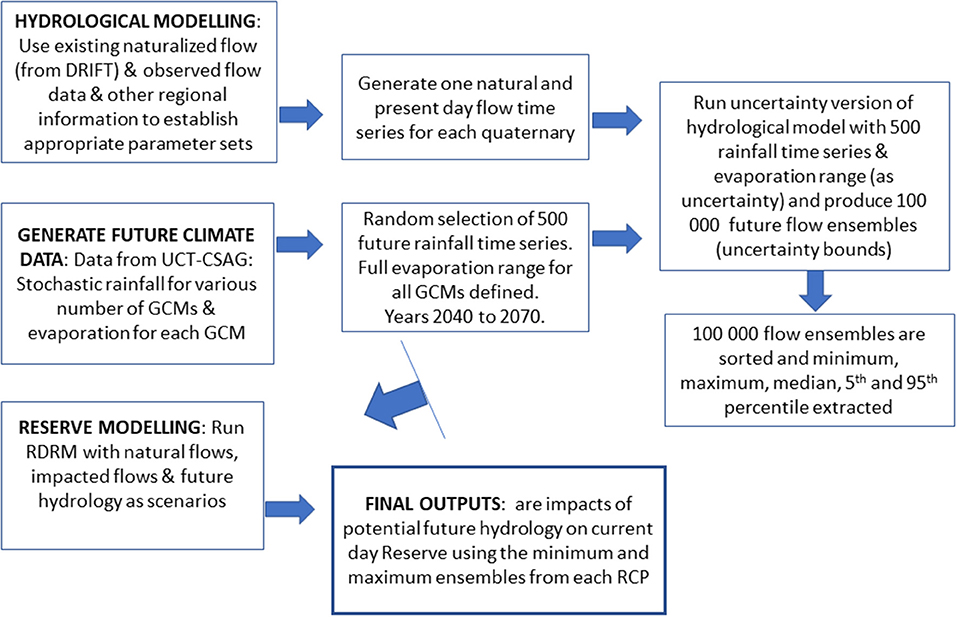
Figure 2. Modeling framework showing the steps to incorporate climate data into the global options uncertain framework.
The modified Pitman rainfall-runoff model (Hughes, 2013) was used to derive hydrological flows for both natural, present day and future hydrology. The hydrological model is a version of the Pitman monthly time step, semi-distributed, rainfall-runoff model (Pitman, 1973), that has been updated on a regular basis and has seen wide use within the country over the last several decades (summarized in Hughes, 2013). The version of the model used in this study is currently implemented as a flexible uncertainty model within a more general water resources modeling framework. The full details of the model are available in recent publications (Hughes, 2013).
The Ecological Reserve was determined for the Doring River in 2006 [Department of Water Affairs and Forestry (DWAF), 2006; Department of Water Affairs (DWA), 2014] using the Downstream Response to Imposed Flow Transformations (DRIFT; Arthington et al., 2003) which is defined as a Level 2 Flow assessment by Opperman et al. (2018). The definition of level 2 is similar to level 1 in that it is mostly reliant on existing information, but it does include input from a multidisciplinary set of experts, usually brought together in a facilitated workshop to use both the existing knowledge and professional judgment to develop flow recommendations and fill data gaps.
Since this project used the Revised Desktop Reserve Model (RDRM) of Hughes et al. (2014), a Level 1 Flow assessment (Opperman et al., 2018), it was calibrated against information used and/or generated using the DRIFT Level 2 Reserve method. The hydrological modeling used to drive the RDRM was calibrated against the natural hydrology used in the original DRIFT assessment to ensure a close match with the original more detailed assessment. The modeling included calibrating the natural hydrology to closely represent the natural hydrology used in the DRIFT assessment, calibrating the present day flows against observed flow gauge data (incorporating limited water use information and small farm dams), and finally using future climate ensembles (year 2040–2070), together with the present day Pitman Model setup under uncertainty to generate 100,000 potential flow time series using the range of future climate ensembles.
The projected climate data for various Global Circulation Models (GCMs) associated with four Representative Concentration Pathways (RCP 2.6–8.5 for January 2041–December 2070; generated by Dr. Piotr Wolski of the Climate System Analysis Group, University of Cape Town) was processed and entered into the hydrological model to produce 100,000 flow ensembles. The data included stochastically downscaled stationary rainfall time series (between 4,700 and 10,500 with 47–105 GCMs per RCP) for each quaternary catchment for the period of January 2041 to December 2070 (with base data from the South African Water Resources database, WR2012 https://waterresourceswr2012.co.za/about/, for the period of 1981–2010 used for downscaling), and a monthly potential evapotranspiration value associated with each GCM for each quaternary. Next, the RDRM was set up with natural and present day hydrology (with anthropogenic impacts), and with future hydrology as scenarios. The outputs generated are minimum and maximum future hydrology data which incorporate the impacts of climate change.
The Water Quality Systems Assessment Model (Slaughter et al., 2017), which has been developed for South African conditions, was used for modeling total dissolved salts (TDS) on a monthly time step. The outputs of the water quality (TDS) modeling (using future hydrology) are the expected changes in the water quality (TDS conditions) under future climatic conditions. The available water quality data are limited and thus, the calibration to observed data could not represent the spikes in TDS. Thus, we have not presented the water quality results here but they can be referred to in the report by Tanner et al. (2020).
Five South African specialists in the fields of water quality, fish, macroinvertebrates, fluvial geomorphology, and riparian vegetation were then approached to assess the impacts of the hydrological and water quality outputs on their specialty group and to additionally identify some adaptive response options. The expected impacts on these catchment characteristics and communities that are referred to in this manuscript are based on the experience of these specialists. In this paper, we highlight the impacts on freshwater fishes provided by Dr. Paxton.
Results
Hydrological and water quality modeling results
The simulated current day hydrology at the outlet of the entire catchment (quaternary catchment E24M; Figure 1B) compared to the observed data is shown in Figure 3 (time series and flow duration curve comparisons). There is significant non-stationarity, which resulted in an under-simulation of flow before 1950, therefore the present day flow was calibrated against the observed flow for the years after 1950 (Figure 3B). The coefficient of efficiency for the flows at the outlet of the catchment was 0.747 and for log values 0.700.
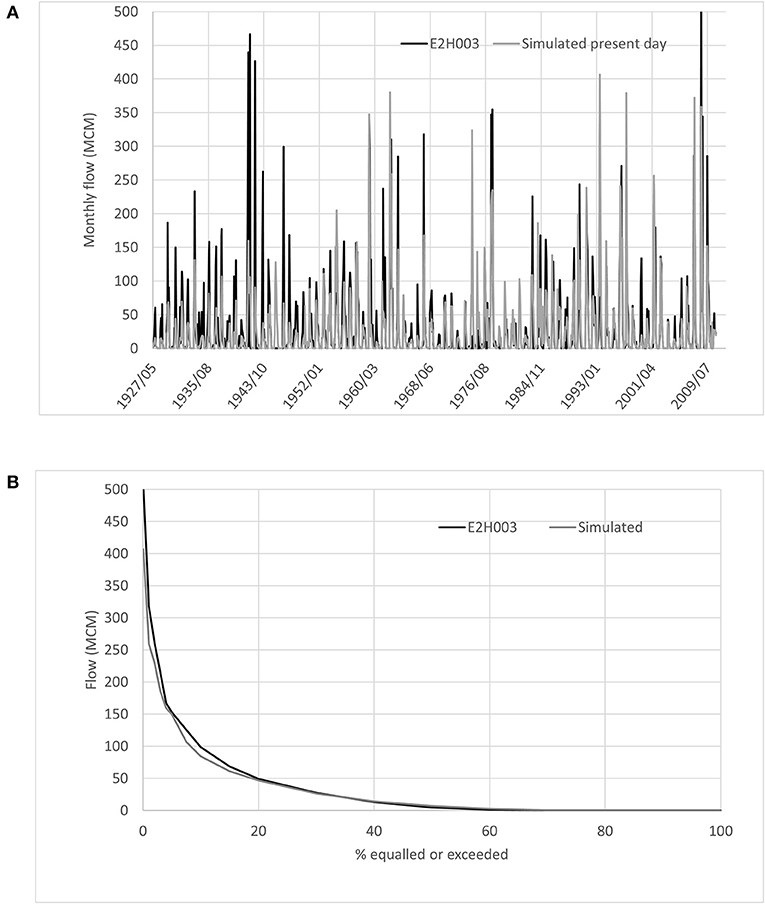
Figure 3. (A) Hydrograph comparing observed flow from flow gauge (E2H003), and simulated present day flow using the Pitman rainfall-runoff model. (B) Flow duration curve comparing observed data with simulated data (1959–2010) for quaternary catchment where Ecological Reserve site 5 (lower catchment) is located.
Table 2 provides the objective functions for the four observed flow gauges. The objective functions were considered acceptable in most of the comparisons due to a complex impacted hydrology which was difficult to represent in the hydrological model. The complexities are related to intensive agricultural abstraction from the rivers as well as from numerous small to medium sized farm dams, as well as numerous private water transfer networks set up between the farm dams.

Table 2. Objective functions for the comparisons of flow (post 1950) for four quaternary catchments with flow gauges in the study area.
Statistics of the future rainfall (minimum and maximum) relative to current climate indicate greater percent time with zero monthly rainfall under all RCPs (Table 3) and maximum monthly rainfall under minimum and maximum future scenarios straddling current maximum rainfall (Table 4). The Mean Annual Evaporation (mm) under all RCPs for the quaternaries straddles current day evaporation and ranges between 99.8 and 115.3% of current values.
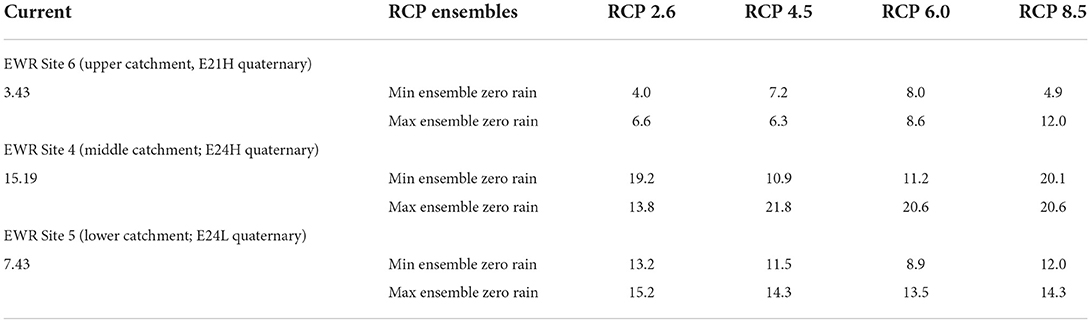
Table 3. Percent time zero monthly rainfall received (%) under current climate vs. that for the minimum and maximum ensembles under the four RCPs for the three quaternary catchments corresponding to the three Ecological Reserve sites.
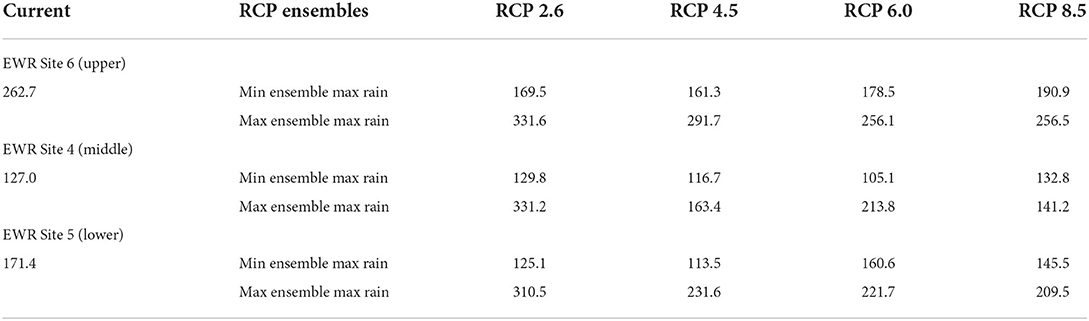
Table 4. Maximum monthly rainfall (mm) under current climate vs. that for the minimum and maximum ensembles under the four RCPs for the three quaternary catchments corresponding to the three Ecological Reserve sites.
The simulated hydrology under natural conditions, under present day conditions and for future climate (minimum and maximum ensemble) under RCP 2.6 and RCP 8.5 for the Ecological Reserve sites are shown in Figure 4. These are actual simulated time series/ensembles of flow which are selected based on the average mean over the 29 years simulated flow. The derived statistics from these data are shown in Tables 5, 6. These two extreme climate scenarios resulted in increased time periods for zero flows in general, with RCP 8.5 being worse (Table 5). The range of uncertainty for the two RCPs straddles the present day percent zero flow time periods, in general. Note that since the minimum and maximum ensembles were selected based on the minimum and maximum values for the mean over the 29 years of modeled hydrology, the relationship between current climate vs. RCP 2.6 or 8.5 scenarios is not always linear. In terms of maximum monthly flow (Table 6), the range of uncertainty is large (particularly for RCP 2.6). The upstream catchment Ecological Reserve site 6 is projected to have reduced maximum flows under both RCPs compared to both natural and present day. For the lower two Ecological Reserve sites (4 and 5) the uncertainty range for maximum monthly flows straddles both natural and present day maximum values but these flows may be significantly higher (Table 6).
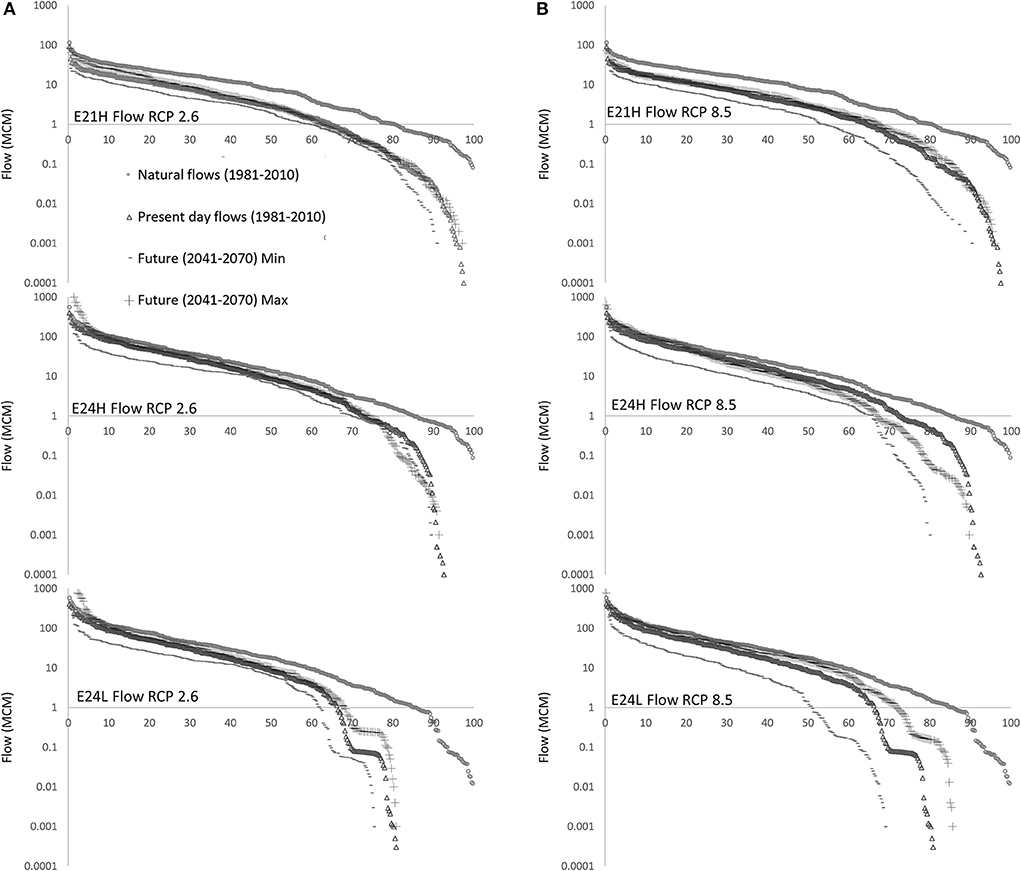
Figure 4. Uncertainty (minimum and maximum scenarios) in future flow (MCM; shown on a log scale) relative to natural and present day simulated data for EWR site 6 (E21H quaternary; row 1), site 4 (E24H quaternary; row 2 figures) and 5 (E24L quaternary; row 3 figures) under RCPs (A) 2.6 and (B) 8.5.
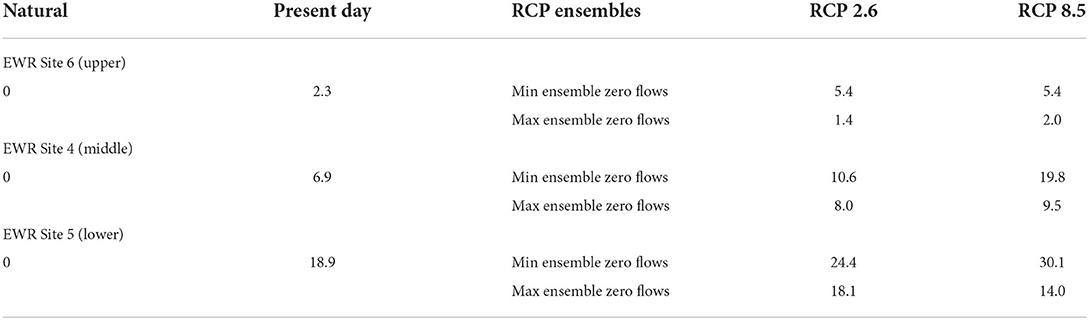
Table 5. Percent time zero monthly flow (%) under current climate (natural and present day hydrology; 1981–2010) vs. that for the minimum and maximum ensembles under RCP 2.6 and 8.5 (2041–2070) for the three quaternary catchments corresponding to the three Ecological Reserve sites.
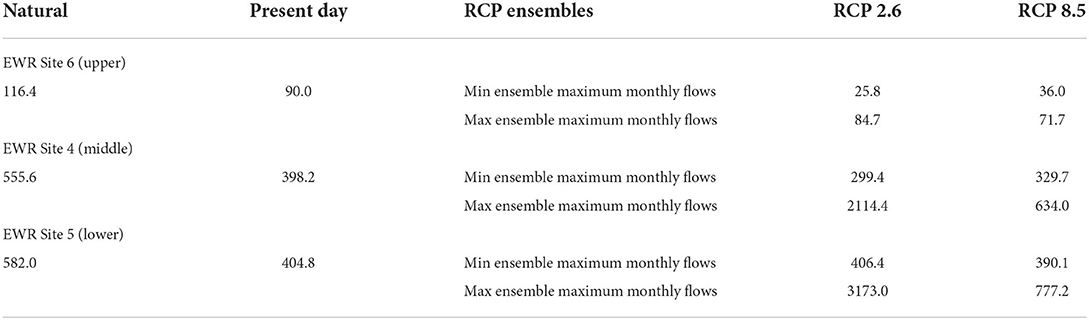
Table 6. Maximum monthly flow (MCM) under current climate (natural and present day hydrology; 1981–2010) vs. that for the minimum and maximum ensembles under RCP 2.6 and 8.5 (2041–2070) for the three quaternary catchments corresponding to the three Ecological Reserve sites.
The future water quality (TDS) under RCP 2.6 for the middle (Ecological Reserve site 4) and the lower catchments (site 5), is projected to shift considerably upwards in relation to the current situation, with simulated maximum TDS under climate change being ~2–3-fold higher, respectively, compared to the current situation (refer to Chapter 4 of Tanner et al., 2020 for details). The frequency distribution of TDS under RCP 8.5 at both sites showed only slight differences to that under RCP 2.6.
Ecological Reserve model results
The outputs of the RDRM model for the upper catchment (site 6) are shown in Figure 5. The RDRM specifies habitat stress values between 0 and 10 for different discharges. A stress of 0 is associated with an upper discharge limit (optimal conditions) and a stress of 10 is associated with zero flow conditions (non-optimal). A stress frequency relationship is determined for the site, before curves for ecological protection categories (A-D) are estimated by shifting the stress frequency curve upwards from the natural curve. Figures 5C,D show the stress-frequency curves for the critical wet and dry season months (determined via the hydrology) for ecological protection categories A-D relative to the climate change minimum and maximum ensembles for RCP 2.6, respectively. The band of uncertainty under RCP 2.6 approximately corresponds to a B/C Ecological Category during the wet season for site 6, while the range of uncertainty for the dry season is well below the flows required to meet even a D category. The results were similar for RCP 8.5 (figures not shown).
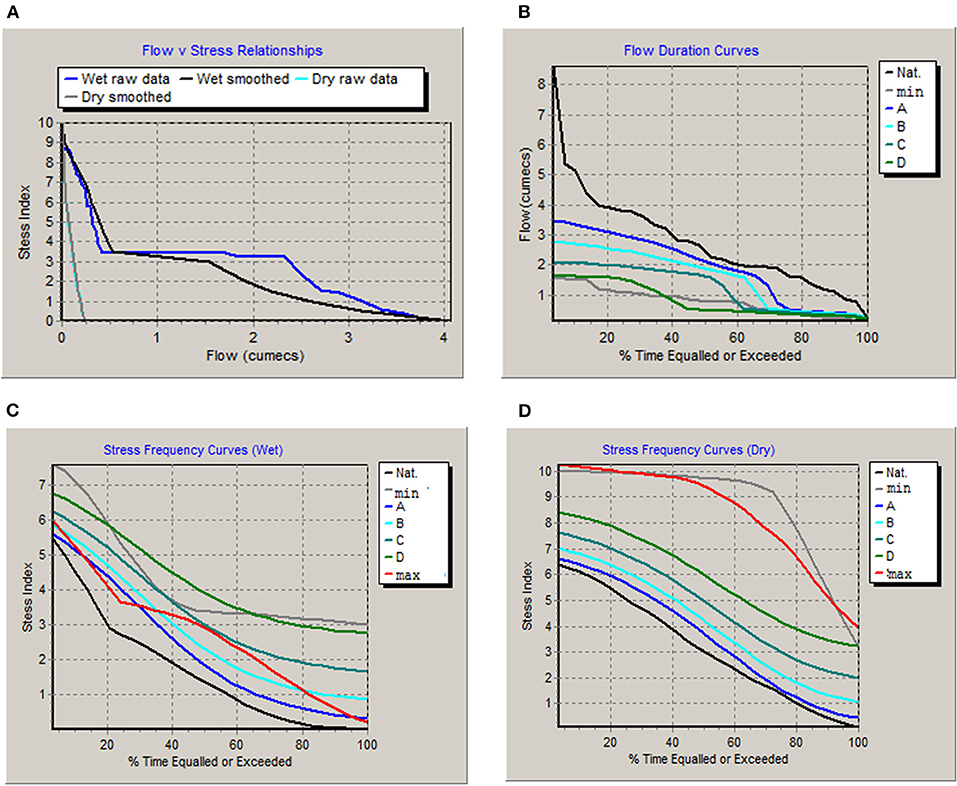
Figure 5. Outputs of the ecology sub-model with RCP 2.6 minimum and maximum ensembles for upper Ecological Reserve site 6 (Ecological Category B/C) (A) flow vs. stress relationships and (B) flow duration curves for natural, minimum ensemble and the four Ecological Categories (C) stress frequency curves for wet and (D) dry seasons.
The results for middle catchment Ecological Reserve site 4 (Ecological Category B) showed a similar pattern (results for RCP 8.5 shown in Figure 6) with stress frequency curves exceeding the dry season stress index for both RCPs 2.6 and 8.5 with stress values above 7 or 8 for the dry season. The wet season band of uncertainty is smaller for RCP 2.6 vs. 8.5. In the lowermost catchment, the results for Ecological Reserve site 5 (Ecological Category B) were similar to site 4 but the stress index values during the dry season are not as extreme throughout the season.
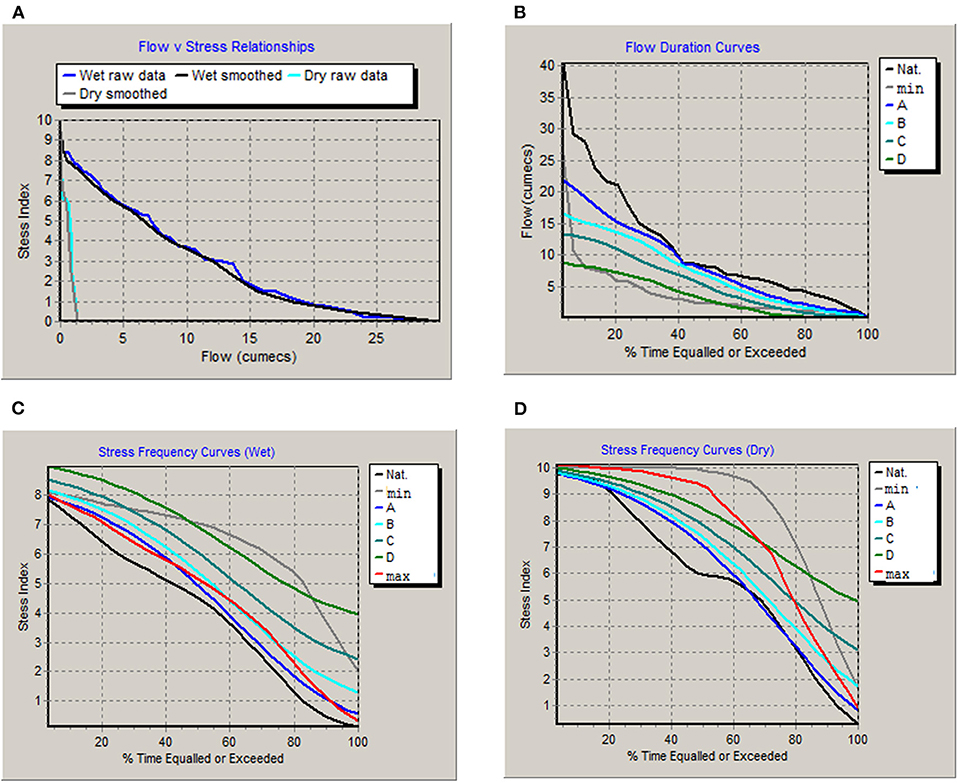
Figure 6. Outputs of the ecology sub-model with RCP 8.5 minimum and maximum ensembles for upper Ecological Reserve site 5 (Ecological Category B) (A) flow vs. stress relationships and (B) flow duration curves for natural, minimum ensemble and the four Ecological Categories (C) stress frequency curves for wet and (D) dry seasons.
Discussion
Growth and development need to occur in the context of long-term sustainability of freshwater systems, which requires the conservation of riverine ecosystems and the associated ecosystem services (Brauman, 2015) and appropriate management through implementation of tools such as the legislated Ecological Reserve in South Africa. The motivation behind the current assessment therefore was evaluation of the near future and long-term impacts of climate change on the adaptive capacity of the riverine ecosystems for suggesting ways to promote future sustainability.
Climate predictions for the three study sites in an arid region, which is important both from biodiversity and economic aspects, indicate that it would likely become more arid under modeled climate change. The maximum flows uncertainty indicates that there is possibility of much higher maximum flows for the middle and lower Ecological Reserve sites. Flood flashiness following heavy rains and the increased length of dry periods that are predicted would both contribute to increased erosion and geomorphological degradation, and further compromise downstream biota and ecosystems. One of the limitations of the modeling is that assessing the maximum and minimum modeled flow rates in effect only assesses the extremes, and that flow rates are likely to fluctuate between these extremes. Additionally, the difference between maximum and minimum flows is large and in many cases straddles present day flow regime characteristics. This contributes to uncertainty in identifying the impacts of flow change. While the majority of future flow ensembles (for all RCPs) predicted an increase in extremes and increased periods of zero flow, there are of course significant uncertainties associated with these modeled results. These include GCM structural uncertainties and climate data downscaling methods which reduce confidence in the predicted future climate data. For this reason, the study included a high number of GCMs (47 GCMs for RCP 2.6; 105 GCMs for RCP 4.5; 47 GCMs for RCP 6.0, and 78 GCMs for RCP 8.5) in order to explore differences in the outputs from the GCMs.
In terms of the hydrological modeling, 500 of the future climate outputs per RCP were randomly selected for use. This was due to the hydrology model being able to incorporate a maximum of 500 rainfall datasets per model run. While the data were checked for consistency, the random selection of the 500 rainfall ensembles meant a completely representative range of climate data might not be necessarily selected for use in the hydrological model. In addition, parameter uncertainty linked to both structural model uncertainty (simplification of the representation of some hydrological processes) and more significantly, water use uncertainty associated with the large amount of unreported water use, meant that hydrological model uncertainties were noteworthy.
Lastly, uncertainties in the Reserve modeling were introduced largely due to the lack of calibration of the category curves by ecological experts. The Ecological Reserve model was set up to represent the original more detailed DRIFT Reserve analysis as closely as possible but due to large differences in the two model structures, the RDRM category curves could not be calibrated using outputs from DRIFT. Ecological specialist time on the project was focused on interpretation of the model outputs and how the potential climate change impacts would affect the system, rather than calibration of the Reserve model.
The expected changes in salinity, geomorphology, and biotic change under climate change scenarios that were generated by the specialists generally indicated a reduced Ecological Category regardless of the climate change scenario assessed. It should be noted that these expected changes are based on modeled flow and habitat quality and availability, and do not include consideration of greater silt transport expected under all scenarios, and the greater salinity for the lower two sites (Ecological Reserve sites 4 and 5). These may act to further impact on biotic responses. Salinity variation is predicted to increase in the future leading to increased seasonal salinity stress and reduced use of abstracted water. Notably, the estimates of TDS under climate change as presented in the report by Tanner et al. (2020) should be interpreted with some caution as the analysis suffered from several sources of uncertainty, including working at a monthly time step and availability of few observed TDS data. Below we discuss the site specific impacts at the three Ecological Reserve sites that are detailed in Chapter 5 of Tanner et al. (2020).
Site specific impacts on salinity, geomorphology, riparian vegetation, and aquatic biota
The salinity at site 6, which is downstream of the most heavily irrigated part of the Doring River catchment, is predicted to decrease regardless of the climate change scenario. In comparison, the salinity at site 4 is predicted to increase significantly under all climate change scenarios. Similarly, the salinity at site 5, which already limits irrigation in late summer, is predicted to increase significantly under all climate change scenarios making it the most saline Ecological Reserve site in the catchment. The specialist report by Dr. Neil Griffin (Institute for Water Research, Rhodes University) noted that summer salinities lead farmers on the lower Doring to curtail irrigation owing to increased salt levels (Belcher et al., 2011). The increased salinities predicted under climate change will further limit water use in the mid- and lower catchment for irrigation. For example, a salinity of 500 mg.l−1, which is approximately the median salinity at site 4 under RCP 2.6 and RCP 8.5 maximum levels, and slightly more than median salinity at site 5 under RCP 2.6 and 8.5 minimum levels, would lead to minor crop losses and would require extra water for leaching of salts from soils [Department of Water Affairs and Forestry (DWAF), 1996]. Under the worst predicted scenario at EWR 5 with salinities of 3,000 mg.l−1, crop yield reductions of even salt tolerant crops would be greater, leaching fractions would be larger, and high frequency irrigation would be required, and livestock production would be decreased to some extent.
The geomorphology Ecological Category should stay the same or decrease slightly for site 6 depending on whether high flows increase or decrease. The geomorphological category is expected to decrease for both sites 4 and 5, particularly under a scenario of decreased high flows. Shifts to a drier climate is expected to reduce vegetation cover and increase soil erosion and sediment supply to the river channels (Xu, 2003). These changes are likely to occur on hill slopes that will decrease soil stability, vegetative cover and increase runoff intensity, all supporting soil erosion and sediment transport to the channel (Lavee et al., 1998). Increases in aridity may increase flood magnitude variability, thus more large floods are expected in relation to small magnitude floods (Molnar, 2001). This is translated to accelerated long-term (geological time) landscape incision that results in increased hill slope and channel erosion and sedimentation, despite reductions in discharge in rivers. Dr. Benjamin van der Waal (Department of Geography, Rhodes University) suggested that there could be increased capacity to transport the larger sediment load, but due to the flashiness of the system it is likely to decrease habitat diversity due to infilling of pools and deeper channels, in addition to poor sorting of materials to form sand bars and benches. An increase in large flood frequency will result in more frequent resetting of the habitat template. These more frequent scour and fill disruptions will lead to a less stable river channel with more variable vegetation dynamics.
The catchment vegetation is dominated by Karoo and Karroid type, consisting of scrub, bushes and some grasses. The majority of the infested area in the focal catchment is in riparian zones and the top ten genera of invasive alien plants are Acacias, Pines, Syringa, Eucalyptus, and Prosopis [Department of Water Affairs and Forestry (DWAF), 2005]. Mr. James MacKenzie, a vegetation specialist at MacKenzie Ecological & Development Services, South Africa, noted that the dry season base flows (summer months) and the duration of time that the river experiences zero flows is important for the determination of species composition (e.g., the presence of sedges and grasses that can endure seasonal drying as opposed to those that require year-round wetness) and the perenniality of pools that importantly support aquatic vegetation and surrounding phreatophytes. Increased periodicity of zero flows will reduce pool perenniality and have dramatic influence on surrounding vegetation. The riparian vegetation for site 6 is expected to be largely unchanged under high flow scenarios, or to degrade somewhat under low flow scenarios. For site 4, riparian vegetation is expected to degrade under all flow scenarios, but particularly RCP 8.5 (minimum). Riparian vegetation at site 5 is anticipated to degrade by one category under all scenarios except under RCP 8.5 (maximum), where less degradation is expected.
Dr. Nelson Odume of the Institute for Water Research (Rhodes University, South Africa) noted that the aquatic macroinvertebrate populations at site 6 are expected to degrade slightly, except under RCP 2.6 (maximum), for which they do not change. Aquatic macroinvertebrates communities at site 4 are suggested to degrade somewhat, particularly under RCP 2.6 scenarios. Similarly, at site 5 some degradation in macroinvertebrate community structure is expected under all climate change scenarios except for RCP 2.6 (minimum). These impacts will be due to serious effects of decreased flow and the increased periods of zero flow on macroinvertebrate instream habitats and water quality state including the predicted increased salinities noted above. The reduced flow would impact on habitat diversity and quality. Significant loss of riffles/runs habitats would be observed, impacting seriously on macroinvertebrates that have a natural preference for riffles/runs and stones in current habitats.
The impacts on freshwater fishes were evaluated by Dr. Paxton and a full section is dedicated to them below due to their endemicity, sensitivity and threat (IUCN listed).
Assessing the effects of climate change on freshwater fishes
Climate change is likely to have a significant effect on the hydrological regimes of rivers, freshwater-dependent ecosystems and the species that inhabit them, particularly in terms of the, quality, quantity, and timing of water delivered to them (Aldous et al., 2011). The freshwater fishes of the Cape Fold Ecoregion (CFR) are especially sensitive to what is predicted to become a hotter and drier climate in the Western Cape under future climate change scenarios. A recent study showed that under these scenarios, indigenous fishes will suffer significant range restrictions from east to west and north to south and into increasingly higher-altitude habitats (Dallas et al., 2019). The anticipated changes and reduced availability of surface waters will be exacerbated by higher demand for water for agricultural, domestic and industrial use. Rivers like the Doring River in the north of the CFR are particularly prone to reduced flows, longer dry seasons and more frequent drought years. The site specific impacts suggest that the fish populations at site 6 will be stressed by extension of the low flow period, and suitable floods will be required to maintain habitat quality. Increased zero flow periods for site 4 will stress fish populations as spawning and migration patterns are disturbed. Fish populations at site 5 will be impacted by extended low flow periods and a reduction in Fast habitats.
The endemic fish species of the Doring are threatened by a combination of predation and competition from introduced and translocated alien fishes together and over-abstraction of water for agriculture. All of the endemic fishes depend to a greater or lesser extent on river flow for habitat throughout the year (e.g., the rock catlets Austroglanis barnardi, EN and A. gilli NT), or for spawning during the southern hemisphere spring (Sep/Oct/Nov) – these include the larger-bodied cyprinids, i.e., the Clanwilliam yellowfish (Labeobarbus seeberi NT), the Clanwilliam sawfin (Pseudobarbus serra NT) and the Clanwilliam sandfish (Labeo seeberi EN) (Paxton and King, 2009). A broad outline of some of the changes that can be expected is provided below.
Spawning growth and development: Clanwilliam yellowfish are non-guarding, open substratum, lithophilic spawners (Balon, 1975; Cambray et al., 1997). They are repeat-spawners over several days and multiple-spawners throughout reproductive season (Cambray et al., 1997). Spawning takes place in high-velocity riffles (all “Fast” depth classes; Table 7) over a cobble-boulder substratum between October and December when temperatures are 19°C and stable or rising. Similar spawning conditions are required by sawfin (Paxton and King, 2009) and likely by sandfish as well. Yellowfish free embryos are photophobic and spend 9–10 days on the spawning beds before swim up occurs. Once they emerge they are carried out of high-flow riffle habitat and into backwaters and slackwaters where they would commence feeding and develop into larval fish (Cambray et al., 1997).
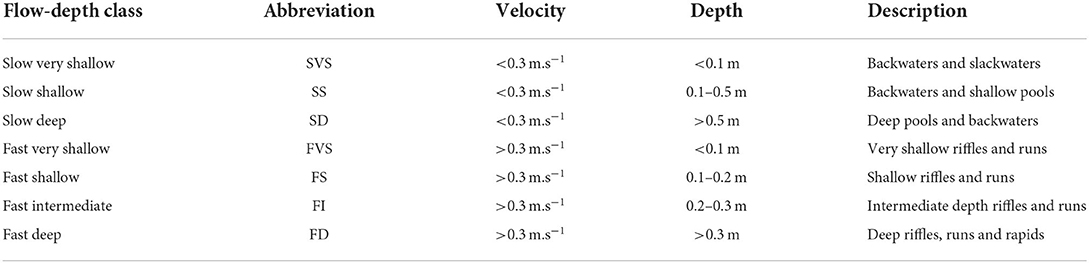
Table 7. Flow-depth classes for fish (Kleynhans et al., 2008).
The absence particularly of sufficient fast flow-depth habitat classes over the reproductive season (October to December) is likely to result in yellowfish skipping spawning (Paxton and King, 2009), or if they do spawn, the growth and development of embryos may be compromised since velocities in the riffles are likely to be insufficient to provide oxygen to the eggs, or wash away metabolites. High egg mortality is likely to arise in these instances due to reduced rainfall and curtailed flows are likely to adversely affect recruitment. Reduced velocities in the riffles is also likely to affect invertebrate productivity and food availability in and adjacent to riffles and rapids for both adults and juveniles. Floods of sufficient magnitude and duration are also required to maintain the spawning habitat integrity, i.e., flush fines from cobble-bed riffles. A reduced frequency and magnitude of flooding is therefore likely to degrade spawning habitats.
Migration: Permanent pools provide over-summering habitat for the indigenous fishes. Seasonal movement between winter, summer and spawning habitat is required. Migration cues may depend on temperature and/or flow-related migration cues for coordinating spawning aggregations (August). Of importance in these reaches is the maintenance of over-summering pools, as well as riffle depths and velocities over the spawning season (October, November and December) when fish are most active and migrating to spawning beds.
Over-summering habitat: Under current-day scenarios, the Doring River ceases flowing between December and June/July. Fish over-summer in deep pools over this period. During this time, the indigenous fishes are particularly susceptible to predation by introduced North American smallmouth bass (Micropterus dolomieu) and bluegill sunfish (Lepomis macrochirus) in the ever-shrinking pools. Also, these alien invasive fish species are less flow-dependent than the indigenous species and a prolonged dry season, or reduced flood intensity or frequency, would favor their proliferation. The climate scenarios examined in this study suggest an increase in the duration (% time) of zero flows, with RCP 8.5 being higher than RCP 2.6. A prolonged period of zero flow would also reduce survival in pools as a result of deteriorating water quality conditions, which, combined with higher temperatures would expose fish populations to significant stress.
Proposed adaptation and mitigation options
Specialists contributing to assessments of expected biotic change under climate change indicated several trends that may have a negative impact on biota. A common negative prediction related to extended periods of zero flow and potential lack of available and suitable pools during low or zero flow events. The habitat suitability will also be impacted by expected greater erosion and seasonal salinity levels, particularly during low flows (Nilsson and Renöfält, 2008). Below are some of the proposed adaptation and mitigation options suggested by the specialists.
One solution to augmenting dry season flows would be controlled releases from upstream impoundments, should management of upstream impoundments be possible. Notably, van Loon's et al. (2022) review noted that although dam releases can alleviate droughts in the dry season, they can also result in deficits during the wet season due to changes in flow seasonality. Perhaps the largest impoundment that might improve dry season flows in the mid and lower catchment is the Oudebaaskraal Dam on the Tankwa River. The suitability of this impoundment for controlled releases is not known, and no data are available on water quality in this impoundment. Given that the water held here drains from the Tankwa Karroo, salinity levels may not be suitable and this would need prior investigation. Furthermore, between 2015 and 2019, the Western Cape experienced the most severe drought on record and the dam dried up. Dam levels have remained extremely low subsequent to the drought, precluding its suitability for releases during drought periods. This aligns with a recent review paper analyzing contrasting case studies across the world which found that streamflow drought is being aggravated, as indicated by drought characteristics determined consistently across studies, due to abstraction for human use (van Loon et al., 2022).
The demand for water in the upstream Kouebokkeveld parts of the catchment is significant and the number of small farm dams is high. The potential of maintaining or supplementing flow in the lower Doring River (and potentially reducing salinity there) might therefore be addressed by managing water use in the Kouebokkeveld. Unfortunately, it was also noted that conflict between land users over water had been recorded, and that the potential for cooperative water management in this region was low. Recent attempts at monitoring and implementing the Ecological Reserve in the Kouebokkeveld, however, are starting to yield positive results (Paxton et al., 2016).
Another potential source of water to supplement flows might be via the transfer scheme from the Breede River catchment, but as this area is liable to be subject to the same or similar climatic changes as the Doring River catchment, availability of water from this source is liable to be curtailed. This would also introduce biota from the Breede River system into the Doring, further placing the endemic fish and invertebrates at risk.
A potential means of ameliorating reduced flow patterns could also relate to management of alien vegetation in the catchment. Water losses and reduced yields owing to alien plants have been found to be significant in South Africa, and particularly for the Western Cape (Le Maitre et al., 2016, 2019). The presence of alien plant Nerium oleander and other invasive species in the riparian zone of the Doring River has been established. In a similar light, the maintenance of buffer zones along rivers in the catchment may act to ameliorate impacts. Protection of high-yield sub-catchments will act to improve water availability in the catchment.
Amelioration of the impacts identified here will not be straightforward, and many of the proposed steps toward amelioration may be contested by other water users, particularly in the parts of the catchments where abstracted water, taken from surface or ground water, underlies economic activity. As climate change predictions indicate, the region will be under greater water stress in the future, and a reduction in water availability will force further adaptive measures on the catchment's water users.
Conclusion
We have investigated the impacts of climate change on the sustainability of water resources in an agricultural catchment, in terms of anticipated impacts on environmental flows and fish biodiversity. The 2019 IPCC Special Report on Climate Change and Land [Intergovernmental Panel on Climate Change (IPCC), 2019] also highlights the potential socio-economic impacts due to the interactions between desertification and climate change that are expected to affect the achievement of various SDGs including access to water and sanitation (SDG 6). Gosling and Arnell (2016) global assessment of climate change impact on water scarcity suggests that an increased exposure to water scarcity is likely for the majority of the world. Thus, adaptation and mitigation responses need to consider adoption of approaches such as sustainable land management and support of nature-based solutions (WWAP/UN-Water, 2018). The South African National Biodiversity Institute's (2014) Framework for Investing in Ecological Infrastructure in South Africa and other research from South African catchments (Mander et al., 2017; Jewitt et al., 2020) are building a case for the protection and restoration of ecological infrastructure, that not only support future water security, but also provide gains in terms of ecosystem functioning (Hughes et al., 2018a,b; South African National Biodiversity Institute, 2019).
Data availability statement
The raw data supporting the conclusions of this article will be made available by the authors, without undue reservation.
Author contributions
JT, SM, and AS: conceptualization, funding acquisition, formal analysis, and writing—original draft preparation. JT, DH, and SM: methodology. DH, AS, and BP: writing—review and editing. All authors have read and agreed to the published version of the manuscript.
Funding
This research was funded by the Water Research Commission of South Africa, Grant No. WRC K5/2834.
Acknowledgments
Climate data was provided by Dr. Piotr Wolski of Climate System Analysis Group (CSAG), University of Cape Town. Technical/software support provided by Mr. David Forsyth. Expert input on specific aspects of the modeling outputs and impacts was provided by BP, Dr. Neil Griffin, AS, Dr. Nelson Odume, Dr. Ben Van der Waal, and Mr. James MacKenzie.
Conflict of interest
The authors declare that the research was conducted in the absence of any commercial or financial relationships that could be construed as a potential conflict of interest.
Publisher's note
All claims expressed in this article are solely those of the authors and do not necessarily represent those of their affiliated organizations, or those of the publisher, the editors and the reviewers. Any product that may be evaluated in this article, or claim that may be made by its manufacturer, is not guaranteed or endorsed by the publisher.
References
Aldous, A., Fitzsimons, J., Richter, B., and Bach, L. (2011). Droughts, floods and freshwater ecosystems: evaluating climate change impacts and developing adaptation strategies. Marine Freshw. Res. 62, 223–231. doi: 10.1071/MF09285
Arthington, A. H., Rall, J. L., Kennard, M. J., and Pusey, B. J. (2003). Environmental flow requirements of fish in Lesotho rivers using the DRIFT methodology. River Res. Appl. 19, 641–666. doi: 10.1002/rra.728
Balon, E. K. (1975). Reproductive guilds of fishes: a proposal and definition. J. Fish. Res. Board Can. 32, 821–864. doi: 10.1139/f75-110
Belcher, A., Grobler, D., Barbour, T., Conrad, J., Dobinson, L., Jonker, V., et al. (2011). Integrated Socio-Economic and Ecological Scenario Specialist Report for the Classification of significant water resources in the Olifants-Doorn WMA. Department of Water Affairs, Pretoria.
Brauman, K. A. (2015). Hydrologic ecosystem services: linking ecohydrologic processes to human well-being in water research and watershed management. Wiley Interdiscipl. Rev. Water 2, 345–358. doi: 10.1002/wat2.1081
Brown, C., Pemberton, C., Birkhead, A., Bok, A., Boucher, C., Dollar, E. D., et al. (2006). In support of water-resource planning – highlighting key management issues using DRIFT: a case study. Water SA 32, 181–192. doi: 10.4314/wsa.v32i2.5258
Cambray, J. A., King, J. M., and Bruwer, C. (1997). Spawning behaviour and early development of the Clanwilliam yellowfish (Barbus capensis; Cyprinidae), linked to experimental dam releases in the Olifants River, South Africa. Regulated Rivers Res. Manag. 13, 579–602. doi: 10.1002/(SICI)1099-1646(199711/12)13:6<579::AID-RRR486>3.0.CO;2-F
Dallas, H. F., and Rivers-Moore, N. (2014). Ecological consequences of global climate change for freshwater ecosystems in South Africa. South Afr. J. Sci. 110, 1–11. doi: 10.1590/sajs.2014/20130274
Dallas, H. F., Shelton, J. M., Paxton, B. R., Weyl, O. L. F., Reizenberg, J., Bloy, L., et al. (2019). Assessing the effect of climate change on native and non-native freshwater fishes of the Cape Fold Ecoregion, South Africa. WRC Report No K5/2337. Water Resarch Commission, Pretoria, South Africa.
de Moor, F. C. (2011). A survey of Trichoptera from the Tributaries of the Doring and mainstream Olifants Rivers, Cedarberg, South Africa with implications for conservation. Zoosymposia 5, 350–359. doi: 10.11646/zoosymposia.5.1.27
Department of Environmental Affairs (2018). 2017-2018 South African National Land-Cover Dataset. Generated by Land Resources International (South Africa).
Department of Water Affairs (DWA). (2014). Determination of Resource Quality Objectives for the Olifants Doorn Water Management Area - Report No. 3 - RQO Determination Report. Department of Water Affairs, Pretoria, South Africa. Available online at: https://www.dws.gov.za/RDM/WRCS/doc/Olifants%20_WMA4_%20RQO%20Report%203%20-%20RU%20Delineation%20Report%20FINAL.pdf
Department of Water Affairs and Forestry (DWAF) (1996). South African Water Quality Guidelines. Volume 4: Agricultural use: Irrigation. Department of Water Affairs and Forestry, Pretoria, South Africa.
Department of Water Affairs and Forestry (DWAF) (2004). Breede WMA: Internal Strategic Perspective. Pretoria, South Africa.
Department of Water Affairs Forestry (DWAF) (2005). Olifants/Doorn Water Management Area: Internal Strategic Perspective. Version 1. DWAF Report No.: PWMA 17/000/00/0305. Report prepared by Ninham Shand Consulting Services. Available at https://www.dws.gov.za/Documents/Other/WMA/17/OlifantsDoornISPFeb05full.pdf.
Department of Water Affairs and Forestry (DWAF) (2006). Olifants/Doring Catchment Ecological Water Requirements Study. Final Summary Report. RDM/E000/MSR/01/CON/0606. DWAF, South Africa.
Ellender, B. R., Wasserman, R. J., Chakona, A., Skelton, P. H., and Weyl, O. L. (2017). A review of the biology and status of Cape Fold Ecoregion freshwater fishes. Aquat. Conserv. Mar. Freshwater Ecosyst. 27, 867–879. doi: 10.1002/aqc.2730
Gosling, S. N., and Arnell, N. W. (2016). A global assessment of the impact of climate change on water scarcity. Clim. Change 134, 371–385. doi: 10.1007/s10584-013-0853-x
Hughes, C. J., de Winnaar, G., Schulze, R. E., Mander, M., and Jewitt, G. (2018a). Mapping of water-related ecosystem services in the uMngeni catchment using a daily time-step hydrological model for prioritisation of ecological infrastructure investment – Part 2: outputs. Water SA 44, 590–600. doi: 10.4314/wsa.v44i4.08
Hughes, C. J., De Winnaar, G., Schulze, R. E., Mander, M., and Jewitt, G. P. W. (2018b). Mapping of water-related ecosystem services in the uMngeni catchment using a daily time-step hydrological model for prioritisation of ecological infrastructure investment - Part 1: context and modelling approach. Water SA 44, 577–589. doi: 10.4314/wsa.v44i4.07
Hughes, D. A. (2013). A review of 40 years of hydrological science and practice in Southern Africa using the Pitman rainfall-runoff model. J. Hydrol. 501, 111–124. doi: 10.1016/j.jhydrol.2013.07.043
Hughes, D. A., Desai, A. Y., Birkhead, A. L., and Louw, D. (2014). A new approach to rapid, desktop-level, environmental flow assessments for rivers in South Africa. Hydrol. Sci. J. 59, 673–687. doi: 10.1080/02626667.2013.818220
Hughes, D. A., and Louw, D. (2010). Integrating hydrology, hydraulics and ecological response into a flexible approach to the determination of environmental water requirements for rivers. Environ. Model. Softw. 25, 910–918. doi: 10.1016/j.envsoft.2010.03.004
Intergovernmental Panel on Climate Change [IPCC] (2019). Climate Change and Land: An IPCC Special Report on Climate Change, Desertification, Land Degradation, Sustainable Land Management, Food Security, and Greenhouse Gas Fluxes in Terrestrial Ecosystems.
Jewitt, G., Sutherland, C., Browne, M., Stuart-Hill, S., Risko, S., Martel, P., et al. (2020). Enhancing Water Security Through Restoration and Maintenance of Ecological Infrastructure: Lessons From the Umngeni River Catchment, South Africa. Report No. TT 815/20. Water Research Commission, Pretoria. doi: 10.5194/egusphere-egu21-11537
Kleynhans, C., Birkhead, A., and Louw, M. D. (2008). Principles of a Process to Estimate and/or Extrapolate Environmental Flow Requirements. WRC Report KV 210/08. Water Research Commission, Pretoria, South Africa.
Kleynhans, C., and Louw, M. D. (2008). Module A: EcoClassification and EcoStatus determination in River EcoClassification: Manual for EcoStatus Determination (Version 2). WRC and DWAF report TT 329/08.
Kundzewicz, Z. W., Mata, L. J., Arnell, N. W., Döll, P., Kabat, P., Jiménez, B., et al. (2007). Freshwater resources and their management. Climate Change 2007: Impacts, Adaptation and Vulnerability. Contribution of Working Group II to the Fourth Assessment Report of the Intergovernmental Panel on Climate Change. M.L. Parry, O.F. Canziani, J.P. Palut. Cambridge, UK.
Lavee, H., Imeson, A. C., and Sarah, P. (1998). The impact of climate change on geomorphology and desertification along a Mediterranean-arid transect. Land Degradation Dev. 9, 407–422. doi: 10.1002/(SICI)1099-145X(199809/10)9:5<407::AID-LDR302>3.0.CO;2-6
Le Maitre, D. C., Forsyth, G. G., Dzikiti, S., and Gush, M. B. (2016). Estimates of the impacts of invasive alien plants on water flows in South Africa. Water SA 42, 659–672. doi: 10.4314/wsa.v42i4.17
Le Maitre, D. C., Görgens, A. H. M., Howard, G., and Walker, N. (2019). Impacts of alien plant invasions on water resources and yields from the Western Cape Water Supply System (WCWSS). Water SA 45, 568–579. doi: 10.17159/wsa/2019.v45.i4.7538
Leigh, C., Bush, A., Harrison, E. T., Ho, S. S., Luke, L., Rolls, R. J., et al. (2015). Ecological effects of extreme climatic events on riverine ecosystems: insights from Australia. Freshw. Biol. 60, 2620–2638. doi: 10.1111/fwb.12515
Mander, M., Jewitt, G., Dini, J., Glenday, J., Blignaut, J., Hughes, C., et al. (2017). Modelling potential hydrological returns from investing in ecological infrastructure: case studies from the Baviaanskloof-Tsitsikamma and uMngeni catchments, South Africa. Ecosyst. Serv. 27, 261–271. doi: 10.1016/j.ecoser.2017.03.003
Molnar, P. (2001). Climate change, flooding in arid environments, and erosion rates. Geology 29, 1071–1074. doi: 10.1130/0091-7613(2001)029<1071:CCFIAE>2.0.CO;2
Nilsson, C., and Renöfält, B. M. (2008). Linking flow regime and water quality in rivers: a challenge to adaptive catchment management. Ecol. Soc. 13, 18. doi: 10.5751/ES-02588-130218
Opperman, J. J., Kendy, E., Tharme, R. E., Warner, A. T., Barrios, E., and Richter, B. D. (2018). A three-level framework for assessing and implementing environmental flows. Front. Environ. Sci. 6, 76. doi: 10.3389/fenvs.2018.00076
Palmer, C. G. (1999). Application of ecological research to the development of a New South African Water Law. J. North Am. Benthological Soc. 18, 132–142. doi: 10.2307/1468013
Palmer, M. A., Lettenmaier, D. P., Poff, N. L., Postel, S. L., Richter, B., and Warner, R. (2009). Climate change and river ecosystems: protection and adaptation options. Environ. Manag. 44, 1053–68. doi: 10.1007/s00267-009-9329-1
Paxton, B. R., Clark, B. M., and Brown, C. A. (2002). An assessment of the effects of habitat degradation and exotic fish species invasions on the distribution of three endemic cyprinids: Barbus capensis, Barbus serra and Labeo seeberi in the Olifants and Doring Rivers, Western Cape. Prepared for the Department of Water Affairs and Forestry and Department of Agriculture by Southern Waters Ecological Research and Consulting.
Paxton, B. R., Dobinson, L., Kleynhans, M., and Howard, G. (2016). Developing an elementary tool for Ecological Reserve monitoring in South Africa's Freshwater Ecosystem Priority Areas (FEPAs): a pilot study in the Kouebokkeveld. Water Research Commission, Pretoria, South Africa.
Paxton, B. R., and King, J. M. (2009). The Influence of Hydraulics, Hydrology and Temperature on the Distribution, Habitat Use and Recruitment of Threatened Cyprinids in a Western Cape river, South Africa. WRC Report No. 1483/1/09. Water Research Commission, Pretoria, South Africa.
Pitman, W. V. (1973). A mathematical model for generating monthly river flows from meteorological data in South Africa. Report No. 2/73, Hydrological Research Unit, University of the Witwatersand, Johannesburg, South Africa.
Schulze, R. E. (2007). South African Atlas of Climatology and Agrohydrology. Water Research Commission report 1489/1/06. Pretoria, South Africa.
Slaughter, A. R. R., Hughes, D. A. A., Retief, D. C. H. C.H., and Mantel, S. K. K. (2017). A management-oriented water quality model for data scarce catchments. Environ. Model. Softw. 97, 93–111. doi: 10.1016/j.envsoft.2017.07.015
South African National Biodiversity Institute (2014). A Framework for Investing in Ecological Infrastructure in South Africa. Lead contributors Tracey Cumming, Amanda Driver, Mark Botha, Jeffery Manuel, John Dini, Anthea Stephens. Pretoria, South Africa.
South African National Biodiversity Institute (2019). South African National Biodiversity Assessment 2018: Compendium of Benefits of Biodiversity. Pretoria, South Africa.
Tanner, J., Griffin, N., Slaughter, A., Mantel, S., Dubula, P., Hughes, D., et al. (2020). Impacts of Climate Change in Determining the Ecological Reserve. Final report for WRC Project K5/2834/1and2. Water Research Commission, Pretoria, South Africa. Available online at: https://www.ru.ac.za/iwr/research/climatechange.
van Loon, A. F., Rangecroft, S., Coxon, G., Werner, M., Wanders, N., di Baldassarre, G., et al. (2022). Streamflow droughts aggravated by human activities despite management. Environ. Res. Lett. 17, 044059. doi: 10.1088/1748-9326/ac5def
van Wyk, E., Breen, C. M., Roux, D. J., Rogers, K. H., Sherwill, T., and van Wilgen, B. W. (2006). The ecological reserve: towards a common understanding for river management in South Africa. Water SA 32, 403–409. doi: 10.4314/wsa.v32i3.5266
WWAP/UN-Water (2018). The United Nations World Water Development Report 2018: Nature-Based Solutions for Water. United Nations World Water Assessment Programme, UNESCO, Paris.
Keywords: climate change, environmental flows (e-flows), hydrological modeling, water availability, biodiversity protection
Citation: Tanner J, Mantel S, Paxton B, Slaughter A and Hughes D (2022) Impacts of climate change on rivers and biodiversity in a water-scarce semi-arid region of the Western Cape, South Africa. Front. Water 4:949901. doi: 10.3389/frwa.2022.949901
Received: 21 May 2022; Accepted: 15 August 2022;
Published: 23 September 2022.
Edited by:
Alessia Flammini, University of Perugia, ItalyReviewed by:
Luz Adriana Cuartas, Centro Nacional de Monitoramento e Alertas de Desastres Naturais (CEMADEN), BrazilJaime Gaona, University of Salamanca, Spain
Copyright © 2022 Tanner, Mantel, Paxton, Slaughter and Hughes. This is an open-access article distributed under the terms of the Creative Commons Attribution License (CC BY). The use, distribution or reproduction in other forums is permitted, provided the original author(s) and the copyright owner(s) are credited and that the original publication in this journal is cited, in accordance with accepted academic practice. No use, distribution or reproduction is permitted which does not comply with these terms.
*Correspondence: Jane Tanner, j.tanner@ru.ac.za