- Graduate School of Global Environmental Studies, Kyoto University, Kyoto, Japan
With the exponential increase in the demand for water, energy, and food (WEF), WEF security is being threatened. To address this issue, the nexus approach, which explores interactions among different WEF sectors as an integrated system, can distinguish between different influencing indicators of WEF security. However, studies on the interactions between WEF sectors in South Korea are few, consequently challenging WEF security, and in the field of social science, WEF security nexus research using a quantitative approach is lacking. This study discusses the interactions composed of synergies and trade-offs between WEF sectors in South Korea through Spearman's rank correlation and network analyses using secondary data at the national level. The results show that the interaction between energy or energy-related sectors was highest; specifically, increasing the proportion of renewable energy utilization improved WEF security. In the water and food sectors, water infrastructure management and value-added management of agriculture showed the most interactions, respectively. The findings demonstrate that WEF security is an interconnected rather than an independent system, and WEF security improves efficiently when preferentially upgrading indicators with many interactions. The study provides important guidelines to prioritize policies to implement sustainable resource management systems.
Introduction
Water, energy, and food (WEF) are essential resources necessary for human survival (Adnan, 2013; Bizikova et al., 2013), and their demand is expected to increase worldwide by 80, 55, and 60%, respectively, in 2050 (Flammini et al., 2014) due to factors such as industrialization, urbanization, population explosion, and economic growth (Hoff, 2011). Consequently, the supply of corresponding resources can be disrupted, which in turn can diminish resource security (World Economic Forum, 2011). The concept of the WEF nexus, which is a holistic framework used to analyze the trade-offs and synergies between water, energy, and food, has emerged to address the problem of WEF security (Albrecht et al., 2018; Terrapon-Pfaff et al., 2018). As the three components of the nexus are inextricably linked, they should be considered integratively (Brears, 2018). For example, food production consumes ~70 and 30% of total global freshwater and energy worldwide, respectively (FAO, 2017). Moreover, bio-crops are not only food resources, but can also be used as a renewable bioenergy source (International Renewable Energy Agency, 2019). Furthermore, the water sector, involving wastewater transfer, treatment, re-use, desalination, distribution, and supply, consumes ~4% of the world's electricity, whereas primary energy production and power generation consume ~10% of the world's water resources (International Energy Agency, 2016).
The WEF nexus approach explores interconnections among different WEF sectors that can be generally regarded as synergies, wherein advances in one sector promote advances in another, and trade-offs, in which advances in one sector hinder advances in another (Putra et al., 2020). Synergies enhance WEF security, whereas trade-offs undermine WEF security (Cai et al., 2018). A cross-sectoral nexus approach to WEF sectors provides an opportunity to fulfill positive synergies and effectively manage trade-offs (Hoff, 2011). This approach could help to achieve the sustainable development goals (SDGs) of the United Nations because SDGs 2 (Zero hunger), 6 (Clean Water and Sanitation), and 7 (Affordable and Clean Energy) are closely related to water, energy, and food, respectively (Liu et al., 2018). Therefore, understanding the interactions among the WEF sectors, maximizing synergies, and minimizing trade-offs, could improve the overall WEF security while achieving SDGs (Karnib, 2018; Stephan et al., 2018; Terrapon-Pfaff et al., 2018).
Previous studies on the WEF security nexus have focused on global (Ringler et al., 2016), transboundary river basin (Amjath-Babu et al., 2019), local (Mroue et al., 2019), and regional (Mahlknecht et al., 2020; Saidmamatov et al., 2020) approaches. Several studies have been conducted on WEF security since the Bon 2011 Nexus Conference (Leck et al., 2015; Endo et al., 2017). However, according to Albrecht et al. (2018), quantitative research on the relationship among WEF sectors are limited at the national scale. Besides, in nexus-related research fields, the environmental science approach is dominant while the social science approach is insufficient (Newell et al., 2019).
South Korea does not have abundant natural resources and is thus facing difficulties to ensure WEF security. First, its renewable water resources per capita (1,453 m3) rank 129 among 153 countries (Ministry of Environment Korea Water Resources Corporation, 2020). Generally, managing water resources is easy and feasible if precipitation is uniformly distributed seasonally; however, in South Korea, precipitation is concentrated in summer. Second, because of the low abundance of natural resources, the country highly depends on imported natural resources for energy production that account for 94% (Korea Energy Economics Institute, 2019). Lastly, the food self-sufficiency rate in South Korea is only 23%, with major dependency on imports for food resources (Korea Rural Economic Institute, 2019a). Based on these factors, comprehensively investigating the WEF security nexus can help achieve sustainable resource management. Furthermore, understanding the interactions among the WEF indicators can not only provide a broader perspective of the relationship among the WEF sectors, but can also help to establish a priority implementation strategy to address WEF nexus security challenges (Huang et al., 2020). Wicaksono and Kang (2019) assessed the feedback analysis results and calculated the reliability index of resources resulting from energy policy changes in South Korea by examining the interlinkages between WEF sectors. Moreover, Wicaksono et al. (2019) proposed an optimization approach to maximize the reliability index of WEF security under plausible drought scenarios, and Lee et al. (2020) analyzed the food- related interconnections in the WEF nexus under different scenarios of climate change and changes in irrigation management. Despite numerous studies and unpredictable resource security, there are still few study cases of the WEF nexus targeting South Korea; additionally, explorations of the interactions between WEF security nexus indicators in South Korea are absent.
To fill these research gaps, this study aimed to perform a quantitative analysis of synergies and trade-offs identified between the WEF security indicators in South Korea using Spearman's rank correlation analysis and network analysis. The specific objectives were: (1) to identify the interactions in WEF security indicators for South Korea, (2) to analyze the most influential indicators in the WEF security nexus, and (3) to provide policy priorities for an effective resource management. The results can facilitate the identification of indicators, whose improvements need to be prioritized by sector, to ensure WEF security. The systematic framework on the assessment of WEF interactions proposed in this study makes a fundamental contribution to policy implementation, which can ensure the effective management of water, energy, and food resources.
Materials and methods
Materials
To identify the indicators influencing the WEF security nexus, the indicators in this study were selected based on the criteria defined in previous studies. Simpson et al. (2020) developed a composite indicator that can effectively measure the WEF nexus using a method developed by the European Commission. Flammini et al. (2014) proposed comprehensive indices for determining the interactions among WEF sectors. Moreover, Bizikova et al. (2013) defined utilization, access, and availability as core indicators of WEF security and further categorized them. For SDGs indicators, SDGs 2 (Zero hunger), 6 (Clean Water and Sanitation), and 7 (Affordable and Clean Energy) were considered by Liu et al. (2018) and Stephan et al. (2018). Referring to these studies, 48 indicators, including 16 indicators each for water, energy, and food, were selected in the present study (Table 1). These indicators considered the availability, accessibility, affordability, and productivity factors of WEF security in South Korea. Data for the selected 48 indicators were obtained for 2004–2018 from the SDG database (UN, 2021), International Energy Agency (2021), FAOSTAT (2021), World Bank (2021), Organization for Economic Cooperation and Development (2021), and the statistics databases of the South Korean government (Korea Energy Economics Institute, 2019; Ministry of Agriculture, Food and Rural Affair of South Korea, 2020; Ministry of Environment of South Korea, 2020, 2021; GIMS, 2021; Korean Statistical Information Service, 2021). The research approach followed in this study was similar to that of Putra et al. (2020), who analyzed the interactions among the WEF sectors from five South Asian countries; however, in the present study, comparatively more indicators were considered with the focus only on one country, that is, South Korea.
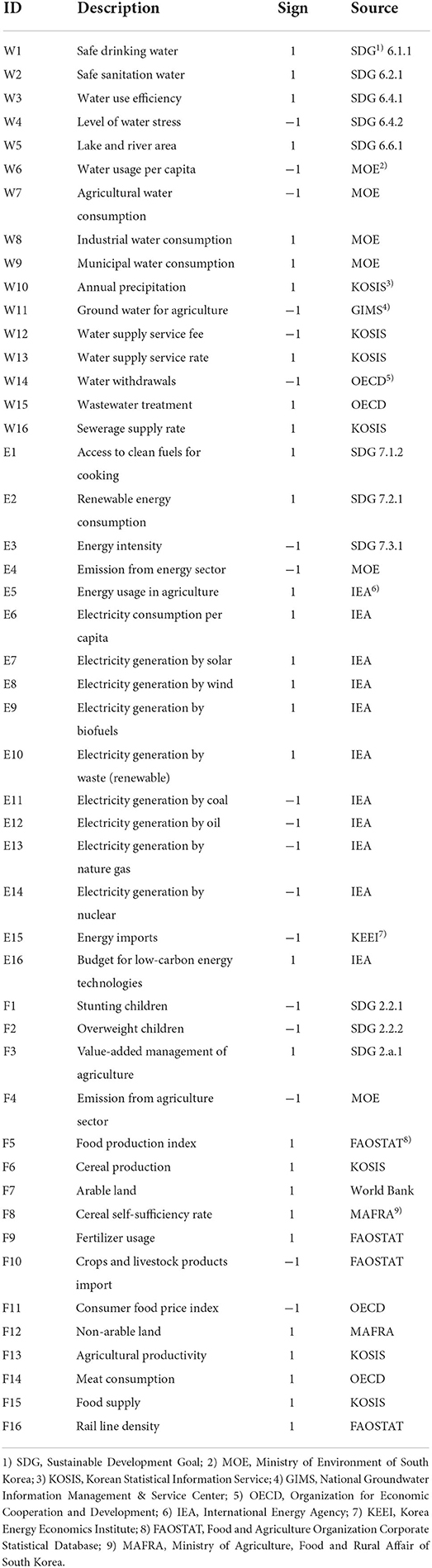
Table 1. Indicators selected to investigate the water, energy, and food (WEF) security nexus of South Korea.
Investigation of interactions
This study investigated the synergies and trade-offs among the WEF sectors mathematically, following the methodological approach suggested by Pradhan et al. (2017), who analyzed the interactions among SDGs by analyzing the official SDG indicator data of 227 countries using Spearman's rank correlation. This approach was also used by Putra et al. (2020) and Hao et al. (2022) to investigate interactions among different WEF security indicators. The author analyzed the correlations among the WEF security indicators as synergies (positive) and trade-offs (negative) based on Pradhan et al. (2017). All indicator values were re-coded consistently to advance WEF security and avoid false correlations. A positive sign was assigned to indicators that improved the WEF security when the indicator value increased, and a negative sign was assigned to indicators that reduced the WEF security when the indicator value increased. For example, a positive sign was assigned to “W3 (water use efficiency)” because an increase in the indicator improved water security; moreover, a negative sign was assigned to “F12 (non-arable land)” because an increase in the indicator decreased food security.
Spearman's rank correlation analysis, for which the coefficient is derived using the ranks of two values instead of the actual data values (Spearman, 1904), was used to identify the correlations between paired indicator values. The correlation coefficient was calculated using the following equation:
where rs is Spearman's rank correlation coefficient, d is the difference in ranks between the paired items, and n is the number of pairs of observations.
Similar to Pearson's correlation coefficient, the correlation coefficient ranges from −1 to 1, with a value of 1 indicating a strong positive correlation, −1 indicating a strong negative correlation, and 0 indicating no correlation (Myers et al.,, 2013). However, unlike Pearson's correlation analysis, Spearman's rank correlation can identify the correlations for non-linear relationships and can be applied to discrete and ordered data if the data can be ranked (Hauke and Kossowski, 2011). Moreover, Spearman's rank correlation is used as an alternative to the Pearson correlation coefficient since it is less sensitive to outliers due to the utilization of ranks rather than actual data values in the calculation and can capture the strength of monotonic relationships (Conover, 1999).
According to previous studies (Pradhan et al., 2017; Kroll et al., 2019; Putra et al., 2020; Ronzon and Sanjuán, 2020; Hao et al., 2022), a Spearman's rank correlation coefficient value of 0.6 or higher indicated a “synergy,” whereas a value of −0.6 or lower indicated a “trade-off.” Moreover, if the value ranged between −0.6 and 0.6, the correlation was interpreted as “unclassified” to avoid over-analysis (Hauke and Kossowski, 2011). Furthermore, only statistically significant correlations, that is, with p < 0.05, were considered. The open-source software Jamovi was used to perform Spearman's rank correlation analysis (The Jamovi Project, 2021).
Network analysis
To discern the most influential indicators of the WEF security nexus for South Korea, network analysis was used, which has been utilized previously to analyze the most influential indicators and interactions between objects (Stein et al., 2014; Kurian et al., 2018; Weitz et al., 2018; Yeh et al., 2019; Mahjabin et al., 2020; Putra et al., 2020; An et al., 2021; Swain and Ranganathan, 2021). Network analysis is a well-developed methodology that provides various tools to analyze the relationships between objects and the patterns and interpretations of such relationships (Wasserman and Faust, 1994). The network generally comprises nodes representing objects and edges that interconnect the pairs of nodes. In this study, the selected 48 indicators were interpreted as nodes, and the interactions between each pair of indicators, analyzed through Spearman's rank correlation analysis, were interpreted as edges. Thus, the interactions between WEF security indicators could be visualized. The open-source software Gephi was used to visualize the network (Bastian et al., 2009).
Results
Interactions within WEF security indicators
Figures 1, 2 show the results of analyzing the interaction between WEF security using Spearman's rank correlation analysis. The colors represent synergy (green), trade-off (orange), and unclassified (apricot). Gray indicates statistically insignificant values with a p > 0.05, which were therefore excluded from the analysis. The proportion of synergies and trade-offs was the same (both 38%) within the water sector, whereas that of synergies (49–56%) was higher than that of trade-offs (43–44%) within the energy and food sectors (Figures 1, 3; Table 2). These interactions indicated that improving an indicator in each sector could improve the other indicator and vice versa. In general, as the water fee and usage increased, water stress could aggravate, consequently exhibiting a negative impact on the overall water security (Waughray, 2011; Lankford et al., 2013). Thus, water usage (W6) and water fee (W12) were positively correlated with water stress (W4), whereas W4, W6, and W12 were negatively correlated with safe water (W1 and W2), water efficiency (W3), and water supply services (W13, W15, and W16). Moreover, W1-W2-W3 and W13-W15-W16 were positively correlated. Agricultural water consumption (W7) and water withdrawal (W14) showed synergies because South Korea uses ~60% of its water resources annually for agriculture (World Bank, 2021).
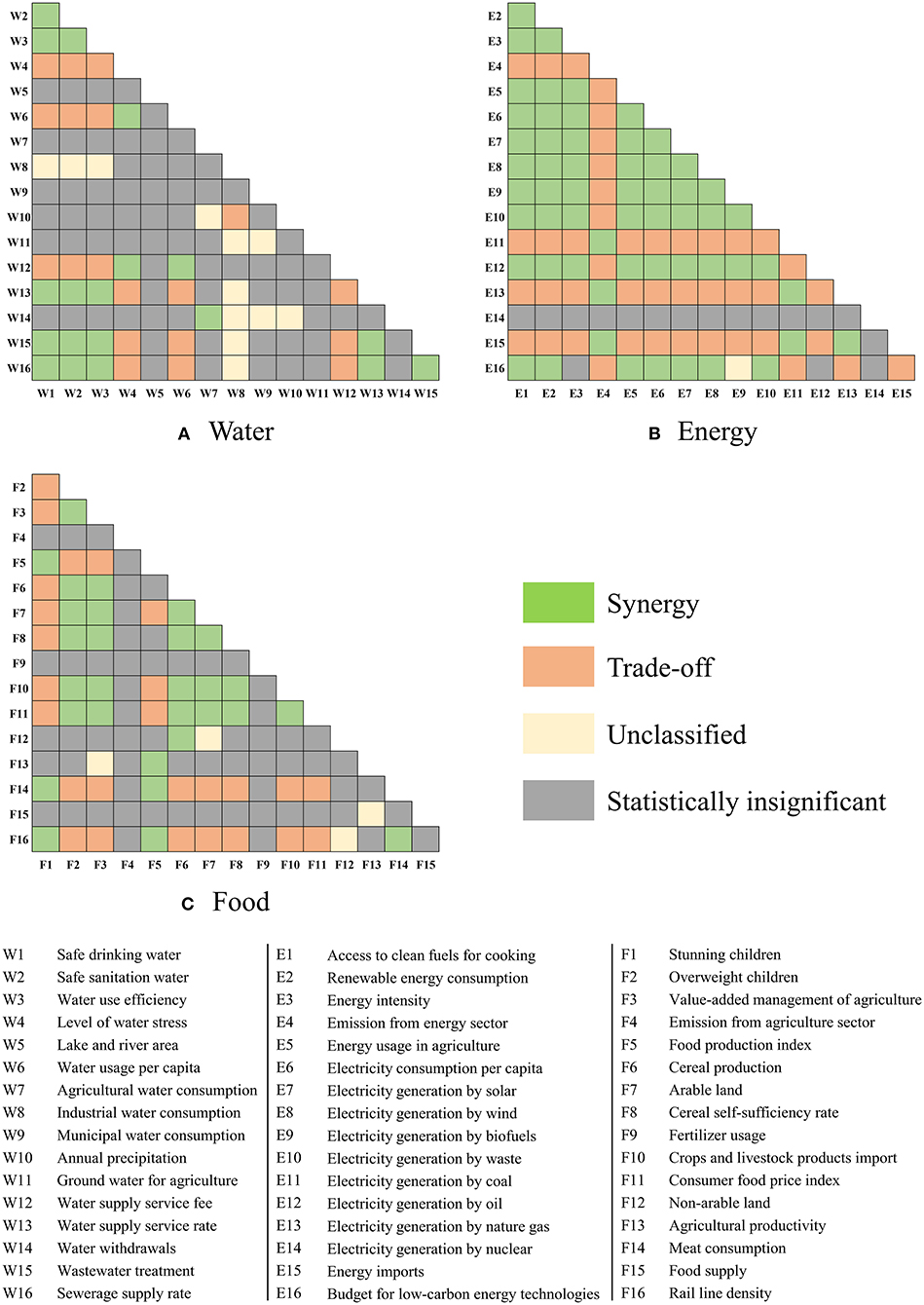
Figure 1. Interactions within water, energy, and food (WEF) security indicators in South Korea: (A) Interactions within water security indicators, (B) interactions within energy security indicators, and (C) interactions within food security indicators.
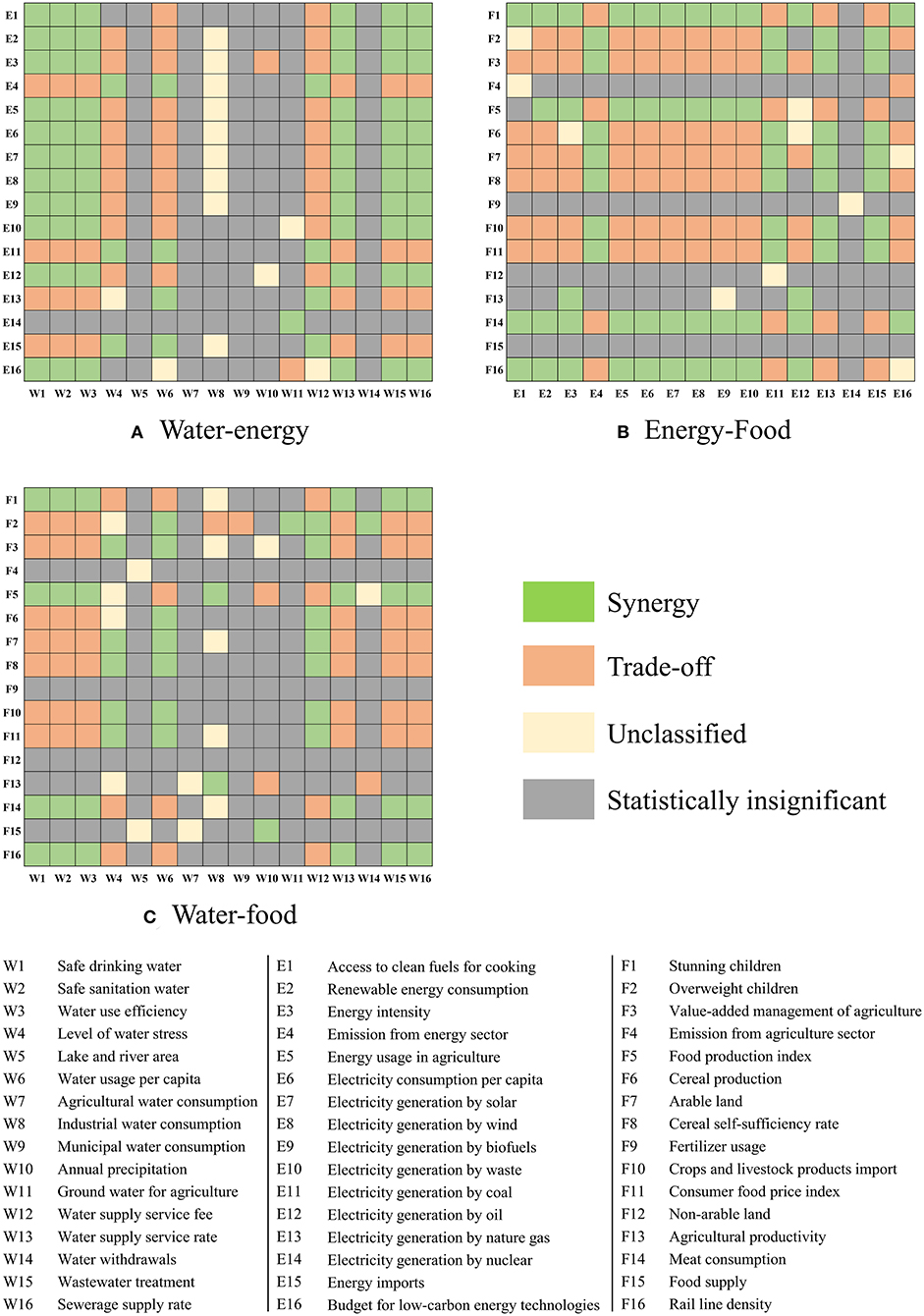
Figure 2. Interactions among water, energy, and food (WEF) security indicators in South Korea: (A) Interactions among water-energy security indicators, (B) interactions among energy-food security indicators, and (C) interactions among water-food security indicators.
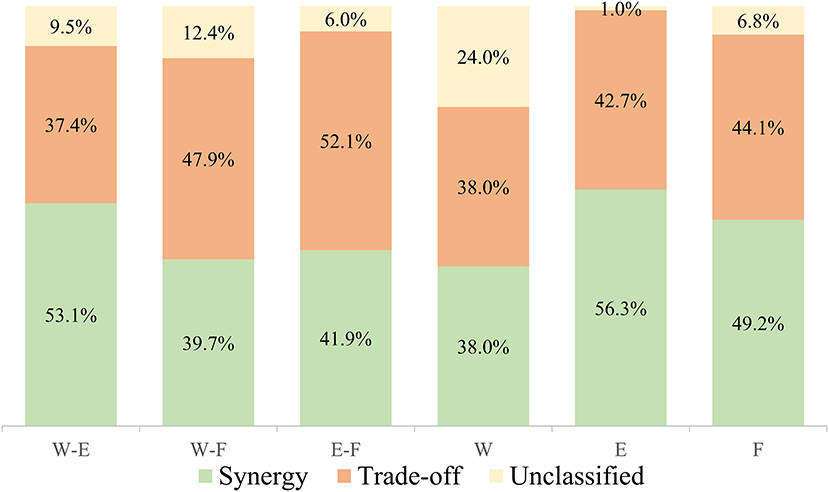
Figure 3. Interactions between WEF security indicators in South Korea indicated by the proportions of synergies, trade-offs, and unclassified items based on Figures 1, 2.
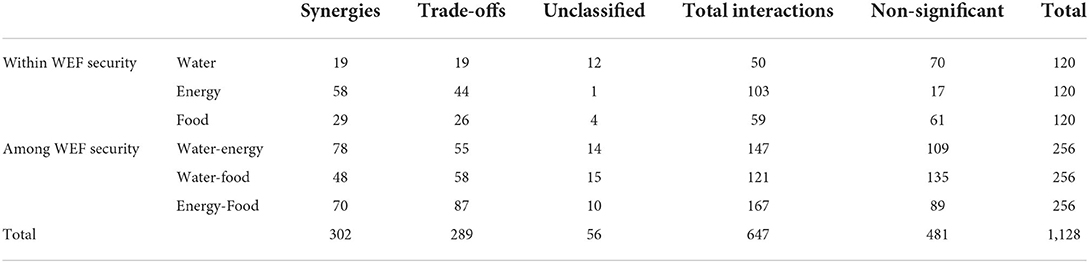
Table 2. Number of WEF interactions in Figure 1.
In the power generation field, renewable energies are environmentally friendly because fuel consumption and carbon emissions are significantly lower than those for non-renewable energies. Therefore, renewable energy indicators (solar: E7, wind: E8, biofuel: E9, and waste: E10) were negatively correlated with the carbon emissions in the energy field (E4), and non-renewable energy indicators (coal: E11 and nature gas: E13) were positively correlated with E4. Furthermore, because renewable energies have lower energy efficiency than non-renewable energies, E7, E8, E9, and E10 were positively correlated with energy intensity (E3), whereas an opposite trend was observed for E11 and E13. Contrastingly, oil-based electricity generation (E12) showed opposite correlations with E3 and E4, unlike other non-renewable energy indicators. This was probably because the operation of oil-fired plants has steadily decreased since 1995, presently accounting for approximately only 1% of the total energy mix (International Energy Agency, 2021). Energy imports (E15) were negatively correlated with the budgets for low-carbon energy technologies (E16). These interactions were similar to those observed in previous studies, which reported that renewable energies could enhance energy security (Valentine, 2011; Hinrichs-Rahlwes, 2013; Gökgöz and Güvercin, 2018).
Furthermore, a well-developed food supply chain can reduce food prices (Bunte, 2006; Armendariz et al., 2015). Hence, the increase in rail line density (F16) and the consumer food price index (F11) were negatively correlated. Additionally, a negative correlation was observed between arable land (F7) and value-added management of agriculture (F3) and between F7 and F3 and the proportion of starving children (F1). Arable land and the value-added management of agriculture in South Korea are continuously decreasing (UN, 2021; World Bank, 2021); thus, improving these problems could solve the hunger problem. As a decrease in domestic food production can be replaced by the corresponding food imports (Sandström et al., 2018), the food production index (F5) and the imports of crop and livestock products (F10) were negatively correlated. Moreover, F10 and F11 were positively correlated, highlighting the dependence on imports for food.
Interactions among WEF security indicators
This study captured interactions in which synergies (40–53%) and trade-offs (37–52%) showed similar proportions among the WEF sectors and discovered possibilities for improving WEF security (Figures 2, 3; Table 2). Many water indicators in the water and energy fields (safe: W1 and W2, efficiency: W3, and supply service: W13, W15, and W16) showed positive correlations with renewable energy-related indicators (E2: renewable consumption, solar: E7, wind: E8, biofuel: E9, and waste: E10) and negative correlations with non-renewable energy-related indicators (coal: E11 and natural gas: E13). Furthermore, indicators related to the water stress level (W4) and water usage per capita (W6) showed negative correlations with renewable energy-related indicators (E7, E8, E9, and E10), possibly because renewable energy consumes much less water than non-renewable energy (Larsen and Drews, 2019), which uses more water for cooling (Macknick et al., 2012). The water industry is highly energy-intensive because of its strong dependency on energy (Kenway et al., 2011; Plappally and Lienhard, 2012). Thus, the water supply service indicators (W13, W15, and W16) and emissions from the energy sector (E4) were negatively correlated.
Regarding water and food fields, as arable land (F7) and cereal self-sufficiency rate (F8) increased, W4 and W6 increased because agriculture is water-intensive and sensitive to water stress (FAO, 2017). For similar reasons, W4 and the consumer food price index (F11) were positively correlated. Moreover, annual precipitation (W10) and food supply (F15) were positively correlated because the volume of available water for food production depends on precipitation (Achite et al., 2017). The water supply service fee (W12) was positively correlated with crop and livestock product imports (F10) because as water prices increase, domestic food prices increase, making people more dependent on cheaper imported foods (Johansson, 2000). In the livestock industry, meat has a high water footprint, which includes the amount of water consumed to obtain products, including feed (Hoekstra and Chapagain, 2011). According to Mekonnen and Hoekstra (2012), for crops, the water footprint for 1 kg of potato, beans, and rice is 900, 1,800, and 4,300 L, respectively, whereas for meat, it is 15,500, 4,800, and 3,900 L for 1 kg of beef, pork, and chicken, respectively. Therefore, when W4 increased, meat consumption (F14) decreased, showing a negative correlation. Further, the indicators related to water supply services (W13, W15, and W16) showed positive correlations with the food production index (F5), indicating that food production becomes more efficient when the water supply system is well-developed (Bhagwat, 2019).
Regarding energy and food fields, the indicators related to agricultural productivity and availability (value-added management of agriculture: F3, cereal production: F6, and cereal self-sufficiency rate: F8) showed negative correlations with renewable energy indicators (E2, E7, E8, E9, and E10) and positive correlations with non-renewable energy indicators (E11 and E13). This is because the levelized cost of electricity from renewable energies in South Korea is higher than that from non-renewable energies (Hong et al., 2019). However, renewable energies are expected to achieve grid parity owing to improvements in efficiency and technology development (Breyer and Gerlach, 2013; International Renewable Energy Agency, 2021), but such correlations could be reversed in the near future. The food industry is an energy intensive industry that consumes ~30% of the total global energy (FAO, 2017). Accordingly, F3, F6, and F8 showed synergies with energy imports (E15), whereas crop and livestock product imports (F10) were negatively correlated with energy consumption in agriculture (E5). Fluctuations in the oil price significantly affect food prices (Esmaeili and Shokoohi, 2011) because food production depends heavily on non-renewable energy resources (Pelletier et al., 2011). Therefore, diversifying energy consumption in the food sector to renewable energy could stabilize food prices (Taghizadeh-Hesary et al., 2019).
Networks of interactions
Figure 4 shows the interaction networks within and among the WEF sectors in South Korea. The diagram on the left shows synergy interactions, whereas the diagram on the right shows trade-off interactions. Furthermore, each node indicates one of the 48 WEF indicators, and edges represent interactions between two indicators on the basis of Figures 1, 2. The higher the number of connected edges, the larger the node. Interactions are expressed in each network by distinguishing between synergies and trade-offs. The indicators of the energy field had the highest influence across all synergy and trade-off networks. Nonetheless, the water and food fields also contributed largely to the networks. The number of edges between the nodes (interactions) ranged from 1 to 21 per indicator. Furthermore, among the top 10 indicators that had the largest number of connected edges, the energy field had the largest influence (Table 3). Table 3 shows the most influential indicators with 20 or more connected edges, implying that these indicators further influenced 20 or more other indicators.
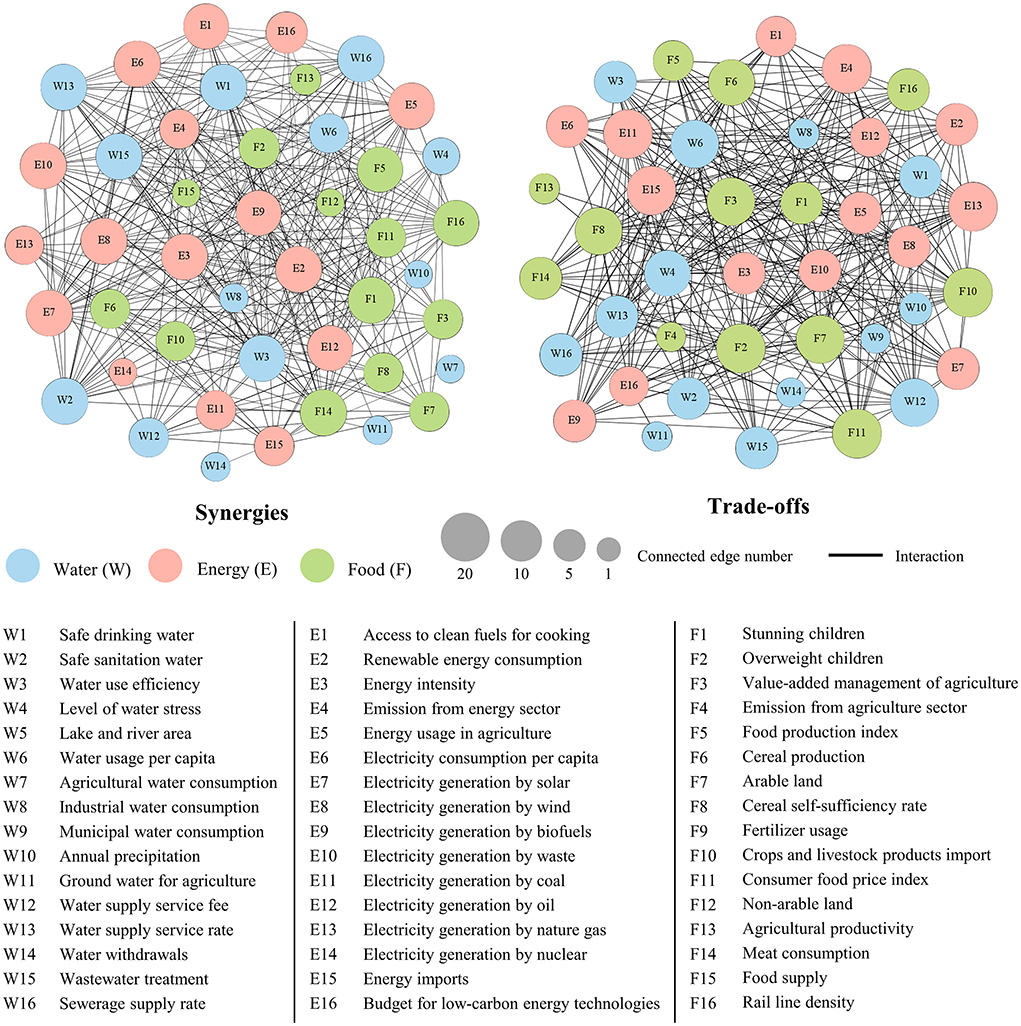
Figure 4. Visualization of the interactions between WEF security indicators in South Korea through network analysis.
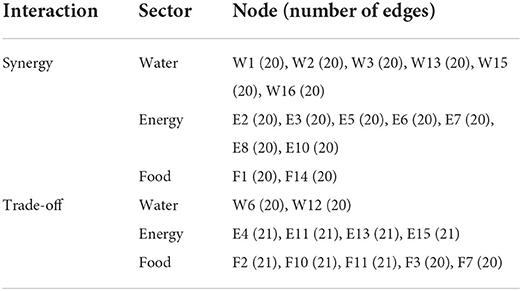
Table 3. Top 10 indicators with nodes having 20 or more edges in Figure 4.
Among the energy-related indicators in the synergy networks, the indicators related to renewable energies (E2, E7, E8, and E10) were the most influential, indicating that increasing the proportion of renewable energies can improve the WEF security (Wicaksono and Kang, 2019; Putra et al., 2020) and positively influence the other 20 indicators. Energy intensity (E3) and energy consumption in agriculture (E5) also showed similar effects. This is because higher energy intensity corresponds to lower water consumption during energy generation, and less food resources; moreover, the proportion of renewable energies for power consumption in South Korea is steadily increasing (International Energy Agency, 2021). Since food and energy industries are water intensive (FAO, 2017; Bhagwat, 2019), the indicators related to water infrastructure, safe water (W1 and W2), water efficiency (W3), and water supply services (W13, W15, and W16) in the water field had the largest impact. Water infrastructure refers to water-related facilities, such as dams, reservoirs, water supply systems, and sewage facilities, including the above-mentioned water-related indicators (Monsma et al., 2009). The current water infrastructure in South Korea is severely deteriorating (Kang, 2019). Thus, it needs major improvements to ensure the sustainability of these indicators. Since meat production has a high water footprint (Hoekstra and Chapagain, 2011), meat consumption (F14) showed many interactions with other indicators. Observations of the food consumption patterns of South Korea indicated that meat consumption has been increasing, whereas cereal consumption has been decreasing since 1980 (Korea Rural Economic Institute, 2019b), suggesting that switching to a cereal-based diet can promote water, energy, and food security.
The energy-related indicators showed contrasting patterns in the trade-off and synergy networks. The indicators related to non-renewable energies (electricity generation by coal and natural gas: E11 and E13, emission from energy sector: E4, and energy imports: E15) had the highest influence in the energy field. The emissions from the energy industry of South Korea are extremely high because the industry largely depends on thermal power for energy and on imports of non-renewable energy sources (International Energy Agency, 2021; Ministry of Environment of South Korea, 2021). Moreover, the thermal power industry is water intensive and can sometimes disrupt the water supply to surrounding villages during a drought (Zhang et al., 2017). Because water is an important resource for food and energy industries, water usage per capita (W6) and the water supply service fee (W12) could compromise WEF security. Interestingly, interactions with precipitation (W10), which is closely related to water availability, were few, implying that even if sufficient water is available, the impacts of W6 and W12 on water security are low if water infrastructure management and use are inefficient. Arable land (F7) and the value-added management of agriculture (F3) had the most negative impact on WEF security because the food industry is directly and indirectly associated with water and energy sectors (Daher and Mohtar, 2015; Vandone et al., 2018). Similarly, the nodes, namely, crop and livestock product imports (F10) and consumer food price index (F11), which are F3 elements, had the largest impact in the trade-off network (Lu and Dudensing, 2015).
Discussion
The current research presents two main findings. First, the interactions of indicators associated with WEF security were quantified, thereby realizing the first objective of the study. The results confirm that WEF sectors, which are inter-linked, interact with each other. Among all interactions, the proportion of synergies and trade-offs was higher, while the proportion of unclassified correlations was lower than those observed in previous studies in South Asian (Putra et al., 2020) and Central Asian (Hao et al., 2022) countries. These findings suggest that the WEF sectors in South Korea are complexly interconnected and that WEF security can be improved through the nexus approach (Organization for Economic Cooperation and Development, 2018). Using the approach outlined here, the author could discuss different ways of maximizing synergies while minimizing trade-offs, which is especially important in countries with WEF insecurity.
Second, the most influential indicators and policy priorities for an effective resource management in the WEF security nexus were analyzed, thus achieving the second and third research objectives. The corresponding results can help identify resource management policies that should be prioritized to ensure efficient WEF security (Flammini et al., 2014; Mahlknecht et al., 2020). For example, renewable energy-related and non-renewable energy-related indicators showed mostly positive and negative influences on WEF security, respectively, in the energy sector. If the proportion of renewable energies is increased in the energy mix, WEF security could be improved by maximizing synergies and minimizing trade-offs (Wicaksono and Kang, 2019; Putra et al., 2020). In the water sector, improving the water infrastructure and efficiency was the most effective strategy. Currently, the water infrastructure in South Korea is deteriorating rapidly, and thus, investments and repairs to maintain WEF security are necessary (Kang, 2019). Simultaneously, controlling water consumption and prices, which negatively influence WEF security, is important. In the food sector, the value-added management of agriculture was the most important. Notably, meat consumption had the most positive influence on WEF security. South Korea relies heavily on imports for meat products, with the import trend increasing overtime (Korea Rural Economic Institute, 2019a). The meat industry is both water and energy intensive (Mekonnen and Hoekstra, 2012), and thus, importing meat products would reduce the consumption of energy and water resources, which is why meat industry was the most influential indicator.
Overall, this analysis indicates that efforts in one area alone cannot improve WEF security as a whole. For example, even if the proportion of renewable energy and food production increases rapidly if the water supply is impaired, WEF security will deteriorate in general. WEF security could be ensured effectively when improving indicators in each field that have the highest influence on other fields.
However, a limitation of this study was that the selected indicators only covered a part of resource security. Owing to the nature of the applied method, which requires pairs of sorted data, the availability of data largely affected the selection of indicators. It is expected that more interactions could be analyzed in the future if more indicators related to WEF security are considered. Moreover, because linear relationships were applied to compare the pairs of data points, the relationships among the three resources (WEF) could not be considered concurrently, but individual relationships between two resources were analyzed by pairing them thrice (W-E, W-F, and E-F). The effects of the three resources could be examined simultaneously if a simulation model, which can simultaneously explore linear and non-linear relationships, is utilized. The implications of this study provide substantial reference to conduct such future studies. Furthermore, for its active utilization, the nexus system should be combined with decision-making fields such that policies can respond to the future demands of WEF resources. This can be achieved by applying various WEF food resource scenarios.
Conclusions
In this study, a systematic framework was proposed to determine the interactions between WEF security in South Korea using two quantitative approaches, namely, Spearman's rank correlation and network analysis to better understand WEF security on a national scale. The results indicated that WEF sectors are closely interconnected, and thus, WEF security could be ensured if synergies are maximized and trade-offs are minimized regarding the interactions in WEF security. Furthermore, the interaction between energy or energy-related sectors was found to be the highest, indicating that managing energy security is the most effective area to improve WEF security. Specifically, reducing the proportion of non-renewable energies and increasing the proportion of renewable energies could contribute significantly to WEF security, followed by the water infrastructure and value-added management of agriculture. An integrated approach via the WEF security nexus will provide a basis for sustainable resource management, and mutual feedback will enable a more efficient use of each resource. In addition, a sustainable and effective resource management could be achieved through policies prioritized for the most interactive indicators.
Data availability statement
The datasets presented in this study can be found in online repositories. The names of the repository/repositories and accession number(s) can be found in the article/Supplementary material.
Author contributions
This research was developed and completed by DA. All methodologies, analyses, and writing were conducted by DA.
Funding
This work was supported by JST SPRING, Grant Number JPMJSP2110.
Acknowledgments
The author would like to heartily thank Professor Makoto Usami from the Graduate School of Global Environmental Studies, Kyoto University, for his careful guidance and valuable advice.
Conflict of interest
The author declares that the research was conducted in the absence of any commercial or financial relationships that could be construed as a potential conflict of interest.
Publisher's note
All claims expressed in this article are solely those of the authors and do not necessarily represent those of their affiliated organizations, or those of the publisher, the editors and the reviewers. Any product that may be evaluated in this article, or claim that may be made by its manufacturer, is not guaranteed or endorsed by the publisher.
Supplementary material
The Supplementary Material for this article can be found online at: https://www.frontiersin.org/articles/10.3389/frwa.2022.943053/full#supplementary-material
References
Achite, M., Buttafuoco, G., Toubal, K. A., and Luca, F. (2017). Precipitation spatial variability and dry areas temporal stability for different elevation classes in the Macta basin (Algeria). Environ. Earth Sci. 76, 1–13. doi: 10.1007/s12665-017-6794-3
Albrecht, T. R., Crootof, A., and Scott, C. A. (2018). The water-energy-food nexus: a systematic review of methods for nexus assessment. Environ. Res. Lett. 13:043002. doi: 10.1088/1748-9326/aaa9c6
Amjath-Babu, T. S., Sharma, B., Brouwer, R., Rasul, G., Wahid, S. M., Neupane, N., et al. (2019). Integrated modelling of the impacts of hydropower projects on the water-food-energy nexus in a transboundary Himalayan river basin. Appl. Energy 239, 494–503. doi: 10.1016/j.apenergy.2019.01.147
An, R., Liu, P., Cheng, L., Yao, M., Li, H., and Wang, Y. (2021). Network analysis of the food–energy–water nexus in China's Yangtze River Economic Belt from a synergetic perspective. Environ. Res. Lett. 16:054001. doi: 10.1088/1748-9326/abe25e
Armendariz, V., Armenia, S., and Atzori, A. S. (2015). “Understanding the dynamics of food supply and distribution systems (FSDS),” in Proceedings of the 33rd International Conference of the System Dynamics Society (Cambridge, MA).
Bastian, M., Heymann, S., and Jacomy, M. (2009). “Gephi: an open source software for exploring and manipulating networks,” in: International AAAI Conference on Weblogs and Social Media.
Bhagwat, V. R. (2019). “Safety of water used in food production,” in Food Safety and Human Health (Academic Press), 219–247. doi: 10.1016/B978-0-12-816333-7.00009-6
Bizikova, L., Roy, D., Swanson, D., Venema, H. D., and McCandless, M. (2013). The Water-Energy-Food Security Nexus: Towards a Practical Planning and Decision-Support Framework for Landscape Investment and Risk Management. Winnipeg: International Institute for Sustainable Development.
Brears, R. C. (2018). “The Green Economy and the Water-Energy-Food Nexus,” in The Green Economy and the Water-Energy-Food Nexus. London: Palgrave Macmillan. doi: 10.1057/978-1-137-58365-9_2
Breyer, C., and Gerlach, A. (2013). Global overview on grid-parity. Prog. Photovolt. Res. App. 21, 121–136. doi: 10.1002/pip.1254
Bunte, F. H. J. (2006). “Pricing and performance in agri-food supply chains,” in Quantifying the Agri-Food Supply Chain: Proceedings of the Frontis Workshop on Quantifying the Agri-Food Supply Chain (Wageningen: Springer).
Cai, X., Wallington, K., Shafiee-Jood, M., and Marston, L. (2018). Understanding and managing the food-energy-water nexus–opportunities for water resources research. Adv. Water Resour. 111, 259–273. doi: 10.1016/j.advwatres.2017.11.014
Daher, B. T., and Mohtar, R. H. (2015). Water–energy–food (WEF) nexus tool 2.0: guiding integrative resource planning and decision-making. Water Int. 40, 748–771. doi: 10.1080/02508060.2015.1074148
Endo, A., Tsurita, I., Burnett, K., and Orencio, P. M. (2017). A review of the current state of research on the water, energy, and food nexus. J. Hydrol. Region. Stud. 11, 20–30. doi: 10.1016/j.ejrh.2015.11.010
Esmaeili, A., and Shokoohi, Z. (2011). Assessing the effect of oil price on world food prices: Application of principal component analysis. Energy Policy 39, 1022–1025. doi: 10.1016/j.enpol.2010.11.004
FAO (2017). The future of food and agriculture: trends and challenges. Ann. Rep. 296, 1–180. Available online at: https://www.fao.org/3/i6583e/i6583e.pdf (accessed November 19, 2020).
FAOSTAT (2021). FAOSTAT data. Food and Agriculture Organization Corporate Statistical Database. Available online at: https://www.fao.org/faostat/en/#data (accessed January 10, 2022).
Flammini, A., Puri, M., Pluschke, L., and Dubois, O. (2014). Walking the Nexus Talk: Assessing the Water-energy-food Nexus in the Context of the Sustainable Energy for All Initiative. Available online at: http://www.fao.org/3/a-i3959e.pdf (accessed September 18, 2020).
GIMS (2021). Statistical yearbook. National Groundwater Information Management & Service Center. Available online at: http://www.gims.go.kr/statistics.do?s_value=statistics_004#this (accessed January 8, 2022).
Gökgöz, F., and Güvercin, M. T. (2018). Energy security and renewable energy efficiency in EU. Renew. Sustain. Energy Rev. 96, 226–239. doi: 10.1016/j.rser.2018.07.046
Hao, L., Wang, P., Yu, J., and Ruan, H. (2022). An integrative analytical framework of water-energy-food security for sustainable development at the country scale: A case study of five Central Asian countries. J. Hydrol. 607:127530. doi: 10.1016/j.jhydrol.2022.127530
Hauke, J., and Kossowski, T. (2011). Comparison of values of Pearson's and Spearman's correlation coefficients on the same sets of data. Quaest. Geogr. 30, 87–93. doi: 10.2478/v10117-011-0021-1
Hinrichs-Rahlwes, R. (2013). Renewable energy: Paving the way towards sustainable energy security: lessons learnt from Germany. Renew. Energy 49, 10–14. doi: 10.1016/j.renene.2012.01.076
Hoekstra, A. Y., and Chapagain, A. K. (2011). Globalization of Water: Sharing the Planet's Freshwater Resources. New York, NY: John Wiley & Sons.
Hoff, H. (2011). Understanding the Nexus. Background Paper for the Bonn 2011 Nexus Conference: The Water, Energy and Food Security Nexus. Stockholm: Stockholm Environment Institute.
Hong, J. H., Kim, J., Son, W., Shin, H., Kim, N., Lee, W. K., et al. (2019). Long-term energy strategy scenarios for South Korea: transition to a sustainable energy system. Energy Policy 127, 425–437. doi: 10.1016/j.enpol.2018.11.055
Huang, D., Li, G., Sun, C., and Liu, Q. (2020). Exploring interactions in the local water-energy-food nexus (WEF-Nexus) using a simultaneous equations model. Sci. Total Environ. 703:135034. doi: 10.1016/j.scitotenv.2019.135034
International Energy Agency (2016). Water Energy Nexus: Excerpt From the World Energy Outlook 2016. Available online at: https://webstore.iea.org/download/direct/303?fileName=WorldEnergyOutlook2016ExcerptWaterEnergyNexus.pdf (accessed February 19, 2021).
International Energy Agency (2021). Data and Statistics. International Energy Agency. Available online at: https://www.iea.org/data-and-statistics (accessed March 2, 2022).
International Renewable Energy Agency (2019). Advanced Biofuels. What Holds Them Back?. Abu Dhabi: International Renewable Energy Agency.
International Renewable Energy Agency (2021). Renewable Power Generation Costs in 2020. Abu Dhabi: International Renewable Energy Agency.
Johansson, R. (2000). Pricing Irrigation Water: A Survey. Policy Research Working Paper No. 2449. Washington, DC: The World Bank.
Kang, H. (2019). Challenges for water infrastructure asset management in South Korea. Water Policy 21, 934–944. doi: 10.2166/wp.2019.005
Karnib, A. (2018). Bridging science and policy in water-energy-food nexus: using the Q-Nexus model for informing policy making. Water Resour. Manag. 32, 4895–4909. doi: 10.1007/s11269-018-2059-5
Kenway, S. J., Lant, P. A., Priestley, A., and Daniels, P. (2011). The connection between water and energy in cities: a review. Water Sci. Technol. 63, 1983–1990. doi: 10.2166/wst.2011.070
Korea Energy Economics Institute (2019). Yearbook of Energy Statistics (Korean). Available online at: http://www.keei.re.kr/main.nsf/index_en.html?open&p=%2Fweb_keei%2Fen_publish.nsf%2Fby_yearbook_energy_stat%2F2d9c8d93dfc8d58049257839004b5173&s=%3FOpenDocument%26menucode%3DES167%26category%3DYearbook%2520of%2520Energy%2520Statistics%26Click%3D (accessed June 20, 2020).
Korea Rural Economic Institute (2019a). Agricultural Outlook 2019. Available online at: http://library.krei.re.kr/pyxis-api/1/digital-files/d4b1612f-1649-44b8-b92e-ab0a1bb878e9 (In Korean) (accessed May 29, 2019).
Korean Statistical Information Service (2021). Database Statistics. Available online at: https://kosis.kr/index/index.do (accessed January 15, 2022).
Kroll, C., Warchold, A., and Pradhan, P. (2019). Sustainable development goals (SDGs): are we successful in turning trade-offs into synergies? Palgrave Commun. 5, 1–11. doi: 10.1057/s41599-019-0335-5
Kurian, M., Portney, K. E., Rappold, G., Hannibal, B., and Gebrechorkos, S. H. (2018). Governance of Water-Energy-Food Nexus: A Social Network Analysis Approach to Understanding Agency Behavior in Managing Water, Soil and Waste Resources to Achieve Sustainable Development Goals. Cham: Springer. doi: 10.1007/978-3-319-75163-4_6
Lankford, B., Bakker, K., Zeitoun, M., and Conway, D. (2013). Water Security: Principles, Perspectives and Practices. New York, NY: Earthscan; Routledge. doi: 10.4324/9780203113202
Larsen, M. A. D., and Drews, M. (2019). Water use in electricity generation for water-energy nexus analyses: the European case. Sci. Total Environ. 651, 2044–2058. doi: 10.1016/j.scitotenv.2018.10.045
Leck, H., Conway, D., Bradshaw, M., and Rees, J. (2015). Tracing the water–energy–food nexus: description, theory and practice. Geogr. Compass 9, 445–460. doi: 10.1111/gec3.12222
Lee, S. H., Choi, J. Y., Hur, S. O., Taniguchi, M., Masuhara, N., Kim, K. S., et al. (2020). Food-centric interlinkages in agricultural food-energy-water nexus under climate change and irrigation management. Resour. Conserv. Recycl. 163:105099. doi: 10.1016/j.resconrec.2020.105099
Liu, J., Hull, V., Godfray, H. C. J., Tilman, D., Gleick, P., Hoff, H., et al. (2018). Nexus approaches to global sustainable development. Nat. Sustainability 1, 466–476. doi: 10.1038/s41893-018-0135-8
Macknick, J., Newmark, R., Heath, G., and Hallett, K. C. (2012). Operational water consumption and withdrawal factors for electricity generating technologies: a review of existing literature. Environ. Res. Lett. 7:045802. doi: 10.1088/1748-9326/7/4/045802
Mahjabin, T., Mejia, A., Blumsack, S., and Grady, C. (2020). Integrating embedded resources and network analysis to understand food-energy-water nexus in the US. Sci. Total Environ. 709:136153. doi: 10.1016/j.scitotenv.2019.136153
Mahlknecht, J., González-Bravo, R., and Loge, F. J. (2020). Water-energy-food security: a Nexus perspective of the current situation in Latin America and the Caribbean. Energy 194:116824. doi: 10.1016/j.energy.2019.116824
Mekonnen, M. M., and Hoekstra, A. Y. (2012). A global assessment of the water footprint of farm animal products. Ecosystems 15, 401–415. doi: 10.1007/s10021-011-9517-8
Ministry of Environment Korea Water Resources Corporation (2020). Water and Future. Available online at: https://www.kwater.or.kr/news/sub03/sub01/landDownload.do?downloadSeq=502 (In Korean) (accessed May 29, 2021).
Monsma, D., Nelson, R., and Bolger, R. (2009). Sustainable Water Systems: Step One - Redefining the Nation's Infrastructure Challenge. Washington, DC: The Aspen Institute. Available online at: http://www.aspeninstitute.org/sites/default/files/content/docs/pubs/water_infra_final.pdf (accessed August 5, 2020).
Mroue, A. M., Mohtar, R. H., Pistikopoulos, E. N., and Holtzapple, M. T. (2019). Energy portfolio assessment tool (EPAT): sustainable energy planning using the WEF nexus approach–Texas case. Sci. Total Environ. 648, 1649–1664. doi: 10.1016/j.scitotenv.2018.08.135
Myers, J. L., Well, A. D., and Lorch Jr, R. F. (2013). Research Design and Statistical Analysis, 3rd Edn. New York, NY: Routledge. doi: 10.4324/9780203726631
Newell, J. P., Goldstein, B., and Foster, A. (2019). A 40-year review of food–energy–water nexus literature and its application to the urban scale. Environ. Res. Lett. 14:073003. doi: 10.1088/1748-9326/ab0767
Organization for Economic Cooperation and Development (2018). Managing the water-energy-land-food nexus in Korea: policies and Governance Options, OECD Studies on Water. Paris: OECD Publishing.
Organization for Economic Cooperation Development (2021). OECD Data. Available online at: https://data.oecd.org/ (accessed January 25, 2022).
Pelletier, N., Audsley, E., Brodt, S., Garnett, T., Henriksson, P., Kendall, A., et al. (2011). Energy intensity of agriculture and food systems. Annu. Rev. Environ. Resour. 36, 223–246. doi: 10.1146/annurev-environ-081710-161014
Plappally, A. K., and Lienhard, J. H. V. (2012). Energy requirements for water production, treatment, end use, reclamation, and disposal. Renew. Sustain. Energy Rev. 16:4818. doi: 10.1016/j.rser.2012.05.022
Pradhan, P., Costa, L., Rybski, D., Lucht, W., and Kropp, J. P. (2017). A systematic study of sustainable development goal (SDG) interactions. Earth's Future 5, 1169–1179. doi: 10.1002/2017EF000632
Putra, M. P. I. F., Pradhan, P., and Kropp, J. P. (2020). A systematic analysis of water-energy-food security nexus: a South Asian case study. Sci. Total Environ. 728:138451. doi: 10.1016/j.scitotenv.2020.138451
Ringler, C., Willenbockel, D., Perez, N., Rosegrant, M., Zhu, T., and Matthews, N. (2016). Global linkages among energy, food and water: an economic assessment. J. Environ. Stud. Sci. 6, 161–171. doi: 10.1007/s13412-016-0386-5
Ronzon, T., and Sanjuán, A. I. (2020). Friends or foes? A compatibility assessment of bioeconomy-related sustainable development goals for European policy coherence. J. Clean. Prod. 254:119832. doi: 10.1016/j.jclepro.2019.119832
Saidmamatov, O., Rudenko, I., Pfister, S., and Koziel, J. (2020). Water–energy–food nexus framework for promoting regional integration in Central Asia. Water 12:1896. doi: 10.3390/w12071896
Sandström, V., Lehikoinen, E., and Peltonen-Sainio, P. (2018). Replacing imports of crop based commodities by domestic production in Finland: potential to reduce virtual water imports. Front. Sustain. Food Syst. 2:67. doi: 10.3389/fsufs.2018.00067
Simpson, G. B., Jewitt, G. P. W., Becker, W., Badenhorst, J., Neves, A. R., Rovira, P., et al. (2020). The Water-Energy-Food Nexus Index. Jones & Wagener. Available online at: https://www.wefnexusindex.org (accessed July 19, 2021). doi: 10.31219/osf.io/tdhw5
Spearman, C. (1904). The proof and measurement of association between two things. Am. J. Psychol. 15, 72–101. doi: 10.2307/1412159
Stein, C., Barron, J., Nigussie, L., Gedif, B., Amsalu, T., and Langan, S. J. (2014). Advancing the Water-Energy-Food Nexus: Social Networks and Institutional Interplay in the Blue Nile. Water, Land and Ecosystems (WLE) Research for Development (R4D) Learning Series. doi: 10.5337/2014.223
Stephan, R. M., Mohtar, R. H., Daher, B., EmbidIrujo, A., Hillers, A., Ganter, J. C., et al. (2018). Water–energy–food nexus: a platform for implementing the Sustainable Development Goals. Water Int. 43:472. doi: 10.1080/02508060.2018.1446581
Swain, R. B., and Ranganathan, S. (2021). Modeling interlinkages between sustainable development goals using network analysis. World Dev. 138:105136. doi: 10.1016/j.worlddev.2020.105136
Taghizadeh-Hesary, F., Rasoulinezhad, E., and Yoshino, N. (2019). Energy and food security: Linkages through price volatility. Energy Policy 128, 796–806. doi: 10.1016/j.enpol.2018.12.043
Terrapon-Pfaff, J., Ortiz, W., Dienst, C., and Gröne, M. C. (2018). Energizing the WEF nexus to enhance sustainable development at local level. J. Environ. Manage. 223, 409–416. doi: 10.1016/j.jenvman.2018.06.037
The Jamovi Project (2021). Jamovi. Available online at: https://www.jamovi.org.
UN (2021). SDG Indicators Global Database. Available online at: https://unstats.un.org/sdgs/UNSDG/IndDatabasePage.
Valentine, S. V. (2011). Emerging symbiosis: renewable energy and energy security. Renew. Sustain. Energy Rev. 15, 4572–4578. doi: 10.1016/j.rser.2011.07.095
Vandone, D., Peri, M., Baldi, L., and Tanda, A. (2018). The impact of energy and agriculture prices on the stock performance of the water industry. Water Resour. Econ. 23, 14–27. doi: 10.1016/j.wre.2018.02.002
Wasserman, S., and Faust, K. (1994). Social Network Analysis: Methods and Applications. Cambridge: Cambridge University Press. doi: 10.1017/CBO9780511815478
Waughray, D. (2011). Water Security: The Water-Food-Energy-Climate Nexus. The World Economic Forum Water Initiative. Washington, DC: Island Press.
Weitz, N., Carlsen, H., Nilsson, M., and Skånberg, K. (2018). Towards systemic and contextual priority setting for implementing the 2030 Agenda. Sustain. Sci. 13, 531–548. doi: 10.1007/s11625-017-0470-0
Wicaksono, A., Jeong, G., and Kang, D. (2019). Water–energy–food nexus simulation: an optimization approach for resource security. Water 11:667. doi: 10.3390/w11040667
Wicaksono, A., and Kang, D. (2019). Nationwide simulation of water, energy, and food nexus: case study in South Korea and Indonesia. J. Hydro-Environ. Res. 22, 70–87. doi: 10.1016/j.jher.2018.10.003
World Bank (2021). World BankOpen Data. Available online at: https://data.worldbank.org.
World Economic Forum (2011). Global risks 2011. 6th Edition. Available online at: http://reports.weforum.org/wp-content/blogs.dir/1/mp/uploads/pages/files/global-risks-2011.pdf (accessed September 23, 2020).
Yeh, S. C., Chiou, H. J., Wu, A. W., Lee, H. C., and Wu, H. C. (2019). Diverged preferences towards sustainable development goals? A comparison between academia and the communication industry. Int. J. Environ. Res. Public Health 16:4577. doi: 10.3390/ijerph16224577
Keywords: water-energy-food nexus, synergies, trade-offs, resource management, water security, energy security, food security, WEF security
Citation: An D (2022) Interactions in water-energy-food security nexus: A case study of South Korea. Front. Water 4:943053. doi: 10.3389/frwa.2022.943053
Received: 13 May 2022; Accepted: 11 July 2022;
Published: 02 August 2022.
Edited by:
Reza Kerachian, University of Tehran, IranReviewed by:
Abbas Afshar, Iran University of Science and Technology, IranSteve Pueppke, Michigan State University, United States
Copyright © 2022 An. This is an open-access article distributed under the terms of the Creative Commons Attribution License (CC BY). The use, distribution or reproduction in other forums is permitted, provided the original author(s) and the copyright owner(s) are credited and that the original publication in this journal is cited, in accordance with accepted academic practice. No use, distribution or reproduction is permitted which does not comply with these terms.
*Correspondence: Daehan An, YW4uZGFlaGFuLjgzYSYjeDAwMDQwO3N0Lmt5b3RvLXUuYWMuanA=