- 1Institute of Infrastructure and Environment, University of Edinburgh, Edinburgh, United Kingdom
- 2Department of Hydroinformatics and Socio-Technical Innovation, IHE Delft Institute for Water Education, Delft, Netherlands
- 3Department of Civil Engineering, University of Bristol, Bristol, United Kingdom
- 4School of Engineering, University of Birmingham, Birmingham, United Kingdom
Hydrological hazards (“hydro-hazards”) are defined as extreme events associated with the occurrence, movement and distribution of water, specifically resulting in floods and droughts. As a result of global climate change these hazards are expected to change in the future, with areas of the globe becoming “hotspots” for the intensification of these extremes. This paper is the first global review of the state- of-the-art research on hotspots for floods and drought. The work follows a systematic literature review of published research, and analyses and categorizes the results of 122 published papers after a methodical screening process. The analysis highlighted the geographical areas where increasing hazards are anticipated (e.g., Europe for both floods and droughts), and those areas of the globe where no significant research has been published (e.g., Russia). The methods used to undertake the research are analyzed and new trends identified. Potential avenues for future research are highlighted, including the incorporation of uncertainty analyses into hydro-hazard assessments, the consideration of multi-hazards and their interconnections, and finally the consideration of dynamic vulnerability and exposure in conjunction with changing hydro-hazards to understanding future risk.
Introduction
Hydrological hazards (“hydro-hazards”) are defined as extreme events associated with the occurrence, movement and distribution of water, specifically resulting in floods and droughts. Flood hazards are the result of water excess from one or multiple sources (e.g., coastal, fluvial, or surface/sub-surface water), while drought hazards arise from a deficit of river flow or precipitation over a prolonged period. Exposure to these natural hazards affects people and infrastructure, and the severity of impacts experienced depends on their underlying vulnerability. Impacts can range from tangible, e.g., loss of life, injury, property damage, loss of livelihoods and services, social and economic disruption, or environmental damage through to intangible impacts, such as detrimental effects to mental health. For example, recent events in Europe in July 2021 caused widespread devastation across a large region and resulted in over 200 deaths. In 48 h, a month worth of rain fell onto saturated soils in Germany causing flooding on the Meuse and Rhine rivers across Germany, Belgium and the Netherlands. The floods led to building collapse, relocation of populations and widespread destruction to travel and transport links (UN, 2022).
Hydrological hazards are influenced by both climatic (e.g., temperature, rainfall patterns and intensity) and hydrological factors (e.g., land use, soil and bedrock). With the impact of climate change on global and regional weather, there is growing evidence that there will be changes in the severity and frequency of weather and climate related natural hazards (Collet et al., 2018; Visser-Quinn et al., 2019) arising from warmer and wetter winters, hotter and drier summers (with an increased intensity of summer rainfall) and continued sea-level rise. These changes will lead to more frequent, intense pluvial, fluvial and coastal flood hazards (Alfieri et al., 2017), leading to an increase in flood exposure (Arnell et al., 2019), and similarly to effects on drought frequency, severity (Prudhomme et al., 2014) and exposure globally (Arnell et al., 2019). However, to what extent these hazards will increase is subject to large uncertainties, associated with climate projections, global mitigation implementation success, local land use and hydrology, as well as the ability to project the changes to river flows accurately.
Research to understand the change signal arising from climate change influences on hydrological extremes at a regional scale has been a topic of growing interest across the globe in recent years (Wilby, 2019). Changes to flood magnitude and frequency have been studied at local levels and across river basins (e.g., Lompi et al., 2021), whilst drought signals have generally been explored on a larger spatial scale and over longer timescales (e.g., Carrão et al., 2018; Lu et al., 2019). This work tends to be driven by researcher's interests and often also their location, and hence the spatial coverage of the knowledge associated with changing extremes is piecemeal and variable. Considering in addition the developing science of climate modeling, the evolution of different climate forcing assumptions and emission scenarios in recent years [e.g., between AR4 (IPCC, 2007) and AR5 (IPCC, 2014)], it is clear that scientific understanding of the changes to hydrological extremes is incomplete. Consequently, there is a need to review existing works, to understand the current knowledge base and globally synthesize the gaps in data.
This study aims to review existing literature that focuses on climate change impacts on water resources (flooding and droughts), with particular emphasis on hydrological extremes. This review assesses the state-of-the-art of knowledge, regional understanding of both extremes and identifies cross-cutting challenges as potential research directions of future studies. Though systematic review is specific to health domains, other domains, such as water process engineering (Bautista Quispe et al., 2022), or remote sensing (Wellmann et al., 2020). The scope of the present review is in line with previous two examples, to report on the state-of-the-art, and not to collect data.
Method
The materials used to conduct this review were retrieved from several search engines, namely Scopus, Web of Science and the Directory of Open Access Journals (DOAJ). Papers were limited to journal or conference articles written in English, with no limit on publication date. The initial literature search was conducted in June 2021 and the subsequent analysis between July and December 2021.
The full research methodology is summarized in Figure 1. Search queries were as follows: TITLE-ABS-KEY (“FLOOD” OR “DROUGHT” OR “HYDRO-HAZARD”) AND TITLE-ABS-KEY (“CLIMATE CHANGE PROJECTIONS” OR “FUTURE UNCERTAINTIES” OR “FUTURE HOTSPOT”). In the search, all forms of flooding were included, and no date restriction was set.
The initial search in the three databases returned a total of 486 papers, which was reduced to 462 papers after duplicates were removed. These were then carried forward to the abstract screening phase. Strict inclusion and exclusion criteria were established to keep the papers within the scope of this review. Papers were included if:
(i) their abstracts were about drought or flood hazard projections for the future as a result of climate model projections, and if
(ii) these studies were at a regional or catchment scale.
Papers were excluded if:
(i) the work was discursive, or if
(ii) it was on a small geographical unit (i.e. a local study <5km2), or if
(iii) the work was reporting current flood and drought hazards, without any quantification of future changes.
During the abstract-screening stage, the 462 papers were manually examined to determine whether they met the pre-defined eligibility criteria for the qualitative analysis. The abstract screening process removed 313 papers, leaving 149 papers for the full-text screening stage. The full paper analysis phase disregarded a further 27 papers, leaving 122 papers which were considered for this review. Reasons for removal included: non-English paper (following an English abstract), descriptive text with no new analysis, or qualitative research. When fully reviewing the paper, the analysis process was standardized to interrogate the papers by themes. For each study the hazard type was recorded, the geographic scope of the research, alongside the metrics used to classify and monitor the hazard into the future (Table 1). Method and metric analysis was included in order to explore the diversity (or otherwise) in the approach. The modeling method and the emission scenario was recorded, this included the climate modeling chain that was used to drive the hazard models. Here it was important to explore the evolution of the climate forcing assumption, and the breadth of climate models considered. As part of this analysis, the review highlighted the consideration (or not) of uncertainty and the similarity of the results within regions. Each study was analyzed to collect the information illustrated in Table 1, and cataloged into a full database (Appendix A) whose headings are the listed parameters of Table 1.
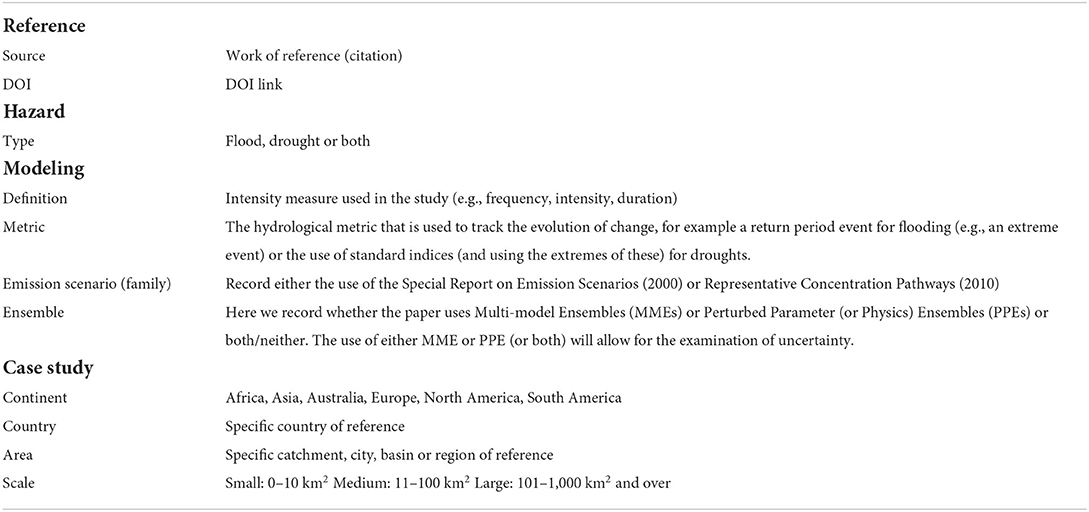
Table 1. List of parameters considered when reviewing the literature (which appear as Table headings for Appendix A).
In addition to collected data, the review team used systematic scientometric software (VosViewer) in order to look for trends in the data in a quantitative manner. Systematic scientometric reviews are used to identify the most representative publications in a field (Blümel and Schniedermann, 2020). For example, the software was used to analyse where the studies were conducted (i.e., location) to explore overlaps and areas of under-representation, and the connectedness of the academics undertaking the work in order to explore areas of research strength. The software completes this analysis in a systematic, repeatable and robust manner.
Analysis
An overview of all case studies presented in the analyzed papers (n = 122) with their location is shown in Figure 2. It is clear from this figure that there is a good geographical spread of analysis, but with areas of significant sparsity (e.g., Russia, New Zealand, the Philippines and large swathes of the African continent). The full database of papers and theme identification is included in Appendix A for interrogation.
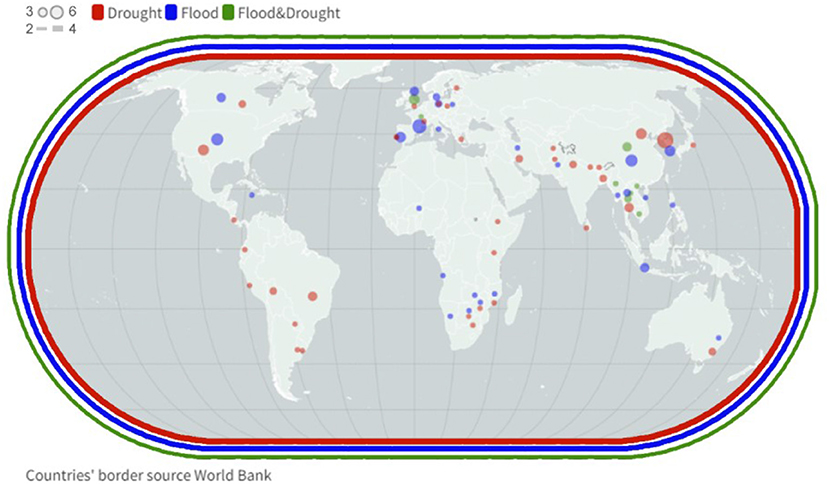
Figure 2. Location of the analyzed research (dots for geographically-located works, circle for global works). The representation of the above map is available interactively at: https://public.flourish.studio/visualisation/8137783/. Details of papers presenting all of the above case studies are available interactively at: https://public.flourish.studio/visualisation/8168904/. The above public links were built using flourish studio visualization tool (flourish.studio).
Scientometric analysis
The systematic scientometric review was used to identify the most representative publications in the study (Blümel and Schniedermann, 2020). In the analysis presented herein, visual analytic approaches from scientometry were used to determine the timeliness of the reviewed papers and the remaining gaps in research of the hydro-hazards hot-spots. The VosViewer tool, developed by Leiden University (Van Eck and Waltman, 2011), was used to map paper outcomes. Figure 3 shows the frequency of most used terms in the reviewed papers, using a threshold of 10 or more occurrences. This figure is the output of a two-stage process which uses a refined second round to further categorize papers. This further round uses a user-defined thesaurus of similar terms, in order to screen for similarity and avoid double counting. As expected, the most frequent terms are floods, droughts, climate change and climate modeling.
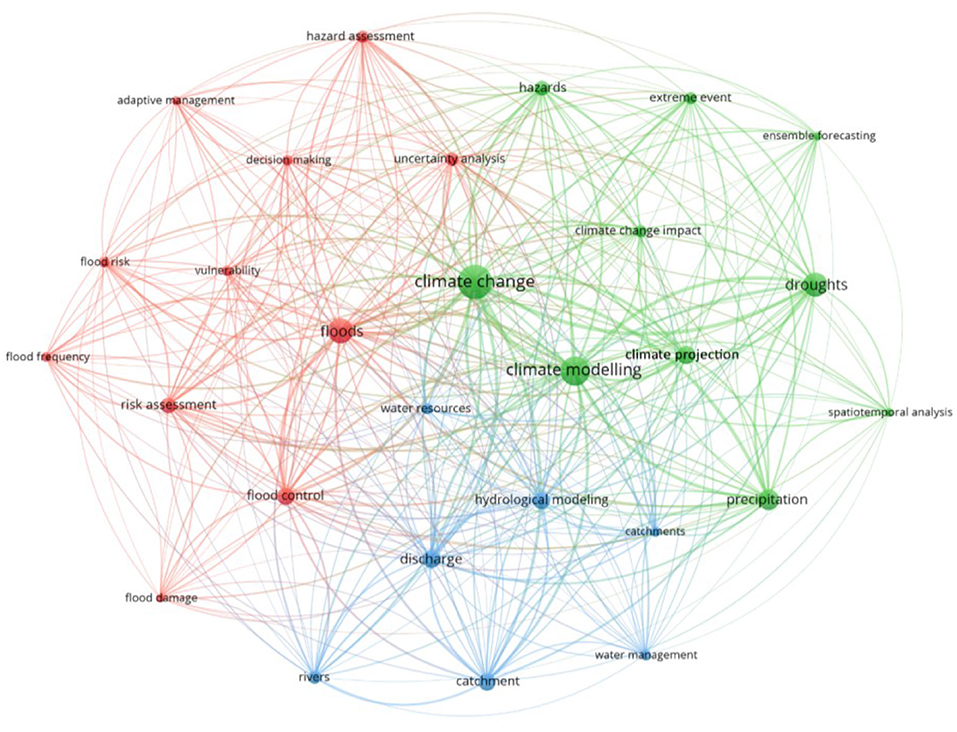
Figure 3. Systematic frequency analysis using Vosviewer tool (vosviwer.com).
The tool offers the possibility of showing the relationship of one term (i.e. a “concept” such as floods, droughts, climate change), with other analyzed terms. This analysis suggests that studies of droughts are more often linked with climate modeling, while studies of floods are more closely linked to uncertainty analysis and risk assessment (see Figures 4A,B). This insight is interesting and may relate to the longer history of flood prediction modeling that has occurred. Additional reasons may lie in the predominant use of precipitation and temperature for drought analyses which are direct products from climate models and do not need further model chains unlike flooding which often uses hydrological models to further downscale projections.
Database analysis
The authors analyzed the papers in a consistent manner to extract the data detailed in Table 1. This consistency is required due to the diversity of terms used to mean a similar item or concept: for example, “area” and “spatial extent” could be used interchangeably depending on the context, or hazard “intensity” could also be referred to as hazard “severity or magnitude”. The resultant database can be found in the Appendix A, and a searchable copy is held here (DOI will be available if published). Preliminary analysis of the database suggests that there is good global coverage of research (Figures 2, 5), with prevalence of case studies in Asia (48 studies), followed by Europe (36), North (16) and South (5) America, and Africa (5). Eight studies had a global focus. However, there are large areas which remain un-researched, which is reflected in Figure 2. It is clear that large areas of Eastern Europe, Northern Asia and the Middle East remain un-studied, alongside swathes of Africa.
Most of the studies (100 of 122) covered an area at “large scale” (101–1000 km2 and over), studies at medium (13; 11–100 km2) and small (2; 0–10 km2) scale are limited; 7 studies did not specify the scale. The analysis of the hazard type highlighted that there is a longer history of flood modeling in association with climate change. Figure 6 indicates that since 2010 (the earliest paper in our analysis), flood hazard assessment in the context of climate change has been a reasonably consistent research topic. The topic of droughts and climate change on the other hand has grown in popularity since 2014. Studies which investigate both extremes consistently are much more poorly represented in the literature. The first occurrence is in 2012, with only sporadic output since then. Around 46% of the analyzed studies are about floods (56), 42% of the works focus on drought (50), while only 15 works (12%) address both hazards (Figure 6). Despite setting no limit on the date of the search, the results were limited to the preceding 11 years, consequently it is clear that this research area is novel and evolving.
Emerging themes
As the database was analyzed, the authors identified a number of emerging themes.
Difference between flood and drought studies
Our analysis shows that flood and droughts research is almost equally distributed. However, their occurrence is not equally balanced spatially. For example, in South America and Australia only droughts are analyzed, whilst in North America and Europe floods are more commonly researched.
In the case of droughts, the majority of studies use direct outputs of climate models (e.g., precipitation and temperature) to analyze future changes. In order to do this analysis, standardized indices are used such as SPEI or the Palmer Drought Index. Different temporal and spatial scales are used for the analysis and these are not consistent across studies, as the chosen scale depends heavily on the focus of the specific study.
The tools used for analyzing floods generally require a further set of models in the climate model chain. These models are mainly hydrological models, which translate precipitation and temperature time series into a times series hydrograph at the outlet of the catchment. This data can be analyzed raw (for example picking out annual maximum flows) or through further statistical analysis such as extreme value analysis (for flood frequency). Hydrodynamic models (which translate flows into detailed flood inundation, depths, extents and velocities) are seldom used, and when they are used this is mainly to determine adaptation measures under climate change conditions, in cases where the management of the river and corresponding catchment is well-known. In this review, the estimates of all of the papers which included flood damage were based on hydrological models rather than hydrodynamic models. Each subsequent model which is added to the climate modeling chain introduces further uncertainty into the analysis process (see discussion in Section 3.3.3).
Spatial coverage
On further analysis of the database, the research suggests that most authors and their teams research their local/regional area. The majority of studies by European authors for example focus on the countries in which the authors themselves are located. Using VosViewer we undertook analysis on the links and collaboration between authors (Figure 7). Six clusters are evident, where there are interactions (i.e. cross-authorship) between research groups. These groups are highly correlated to different spatial or geographical regions (Figure 8). Figure 8 details how authors are clustered and linked for a few clusters in Europe. Further investigation shows that the authorship for the majority of these studies overlaps. For example, a detailed case study in the Rhine and Danube basins links several authors, whilst UK based studies (small but geographically diverse river basins) link other authors. Pan-European studies which look across western Europe link these authors together. Other clusters are also linked by geography, for example clusters in North America (Gaur et al., 2018, 2019) or Asia (e.g., the Korean peninsula or China).
Analysis of uncertainty
There is an increasing trend for the consideration of uncertainty when discussing future changes and trends in flood or drought. There are 54 papers (of 122) which mentioned or explored the uncertainty in future climate results. Further there is evidence of research either mentioning or exploring uncertainty research in each year considered in the review. Uncertainty analyses tend to use either multi-model ensemble projections or perturbed physics ensembles and to explore the sources of uncertainty in climate modeling. In terms of the contribution, climate models are generally considered to be a significant source of uncertainty in projected hydrological change (e.g., Collet et al., 2018; Visser-Quinn et al., 2019).
The uncertainty for future impact assessments arise from several sources (Rojas et al., 2012; Cloke et al., 2013; Prudhomme et al., 2014): (1) climate model including emission scenario, climate model structure and parametrisation (e.g., Lung et al., 2013; Lu et al., 2019); (2) hydrological and topographical observations including scarcity of ground-truthing data of adequate quality and quantity (e.g., Delgado et al., 2014); (3) the bias correction/downscaling process for the climate model (e.g., Didovets et al., 2019; Helaire et al., 2020); and (4) hydrological modeling uncertainty (e.g., Dankers et al., 2014; Prudhomme et al., 2014). However, their opinions vary by scale and focus. Forzieri et al. (2014) focused on uncertainty in low-flow projections including climate uncertainty; extreme value fitting uncertainty and uncertainty in projections of streamflow droughts. This study holds a view that climate model configuration remains the main source of uncertainty and extreme value fitting is the secondary source in climate projections, especially for European precipitation (Déqué et al., 2012). However, at the global scale, Prudhomme et al. (2014) concluded that the main source of uncertainty comes from the hydrological models, with climate models contributing to a substantial but still smaller, amount of uncertainty. This observation is corroborated by Visser-Quinn et al. (2019) at a smaller scale for the UK.
In the context of the models included in the Climate Model Intercomparison Project (CMIP) 5 and CMIP6, some researchers summarized that the (climate) model uncertainty represents the largest source of uncertainty in the 21st century and is associated with three sources of uncertainty: internal variability, model uncertainty, and scenario uncertainty (Lu et al., 2019; Mondal et al., 2021). The reason for the uncertainty has been demonstrated by several researchers. For example, (1) in large-scale studies, global hydrological models are usually applied without any calibration/validation at the catchment scale (Zubieta et al., 2021); (2) climate model-based projections indicate a likely increase in frequency of heavy rainfall or severe drought conditions (Arnell et al., 2019); (3) the uncertainty in regional precipitation over the study region is predominantly related to spatial shifts in convection and convergence (Tan et al., 2020).
Research has used uncertainty analysis to quantify model accuracy; however, it is also used to understand the confidence in future projections. On the catchment scale, uncertainty can be assessed using the Generalized Likelihood Uncertainty Estimation (Samantaray et al., 2020) and the Reliability Ensemble Averaging (REA) technique (Abdulai and Chung, 2019). For frequency analysis, bootstrap resampling can be used to measure uncertainty of the sample estimates. Some measures are employed to reduce model uncertainty such as: statistical calibration of hydrological model; a real option analysis (ROA) and Monte Carlo simulations. Also other methods can be used to quantify the sources of uncertainty in the modeling chain (e.g., quasi-ergodic analysis of variance QE-ANNOVA (Visser-Quinn et al., 2019).
Emerging research on multi-hazards
Floods and droughts are significant natural hazards, and together contribute to the majority of natural disasters globally every year (e.g., in the year 2020, floods caused around two thirds of all natural disasters globally (CRED and UNDRR, 2021). These hazards have traditionally been researched in isolation, but more recently (as evidenced by this analysis) this approach has begun to change. However, such hazards do not act in isolation and significant flood periods can occur during, or subsequent to a period of prolonged drought, thus amplifying the effects of any single event (de Ruiter et al., 2020). Multi-hazard research has developed considerably in recent years, and this approach investigates related events and the different mechanisms which can intensify impacts. Considering hazards singularly, without considering their interactions, significantly underestimates their effects (Gill and Malamud, 2016), and considering hazards without the associated vulnerability of those exposed to hazards, and how that vulnerability changes over time (dynamic vulnerability), drastically underestimates risk to those impacted. Future research should look across the scientific community to improve our understanding of multi-hazards, including hydro-hazards, and to quantify the interaction of the processes that drive them.
Discussion
Current gaps and future hotspots of change
The data collected from 122 papers revealed that there is good coverage at global level, with eight global studies and a prevalence of case studies in Asia (48 studies), followed by Europe (36), North (16) and South (5) America, and Africa (5). However, large areas of Eastern Europe, Northern Asia, Middle East and Africa remain un-studied. On further analysis of the database, results suggested that most works focus on their local/regional area. Around 46% of the analyzed studies are about floods, 42% focus on drought, while only 15 works (12%) address both hazards. Different temporal and spatial scales are used for the analysis and these are not consistent across studies, as the methods depend heavily on the focus of the specific study. There is an increasing trend for the consideration of uncertainty, when discussing future changes and trends on floods or droughts.
Detailed studies of the distribution of intensifying hydrological extremes within local regions are available (as identified in Appendix A). These studies can be used to understand spatio-temporal aspects of the projections and whether regional trends emerge. For example, a number of studies have explored the national picture for the UK (Lane and Kay, 2021 (this issue); Collet et al., 2018; Visser-Quinn et al., 2019, 2021; Arnell et al., 2021 amongst others), investigating potential patterns, as well as examining the trends in seasonality of hazard occurrence. What is clear from the work explored in this paper is that a significant amount of information exists on potential hazard trends, and that in the future much of the global population will be at a greater risk of exposure from either or both floods and droughts than they currently are. Thus, the more information that exists on these trends, the better the potential for adaptation. Several of the studies (Prudhomme et al., 2014; Alfieri et al., 2017; Carrão et al., 2018; Lu et al., 2019; Coppola et al., 2021) explored the broader, global scale of analysis and this analysis can assist in identifying consistent areas which are future hotspots of change. Whilst a global analysis is necessarily large scale, and lacks the detail that is often needed for detailed adaptation plans, the strength of this approach is that it highlights those areas which are at most risk of change in a comparable manner. Looking across these studies, it is important to explore whether there are consistent messages arising. Of the studies, one focussed on floods (Alfieri et al., 2017), two focussed on both hydrological extremes (Arnell et al., 2019; Coppola et al., 2021) and three covered drought indicators only (Prudhomme et al., 2014; Carrão et al., 2018; Lu et al., 2019).
Consistent messages arise around floods from Alfieri et al. (2017) and Coppola et al. (2021) which identify intensifying flood risk (more frequent and significant increases) are unevenly distributed. Large changes are anticipated with the largest increases in Asia, and Europe. Arnell et al. (2019) corroborate this finding in their study too. Coppola et al. (2021) additionally identify the La Plata basin, Central Africa as well as Northern Australia, and China. While Alfieri et al. (2017) identify North America in addition. The global projections of drought also deliver reasonably consistent messages. Areas under increasing risk (more frequent and severe) are Southern and Central America, and the Caribbean, Europe and specifically the Mediterranean region (Prudhomme et al., 2014; Carrão et al., 2018; Lu et al., 2019). However, there are less consistent messages for India (identified by Coppola et al., 2021), Africa (identified by all studies but with different regions highlighted (e.g., Central Africa - Prudhomme et al., 2014; Arnell et al., 2019; Southern Africa - Lu et al., 2019; Coppola et al., 2021). Prudhomme et al. (2014) and Coppola et al. (2021) highlight Australia and New Zealand as hotspots whilst Lu et al. (2019) additionally identifies Eastern North America. Based on the above insights, it would seem that Africa and much of Asia need particular focused analysis as future hotspots, and further detailed studies are needed for Europe as a hotspot for both droughts and floods.
Novel datasets and analytics
The understanding of hydrological hazards has benefitted greatly from the development of remotely-sensed data over recent decades. This is true for droughts (AghaKouchak et al., 2015) and particularly for flooding (Bates, 2022). Early remotely-sensed digital terrain data represented a significant improvement on paper-based mapping and has developed from resolutions of tens of meters to <1 meter. Further developments have led to datasets with global coverage such as the NASA Shuttle Radar Topography Mission (SRTM) in 2000 (Farr et al., 2007) which has a resolution of approximately 90 m at the Equator. While further developments at a global scale promise greater resolution and accuracy, there is a lack of data on aspects beyond terrain heights. For example, despite much promise there is a lack of high resolution, accurate data for river channel bathymetry and discharge. Developments on these aspects are arguably more important for the future than greater resolution in terrain data given that current terrain data is at the limits of what most inundation models can use, meaning that deficiencies in bathymetry and discharge data represent the main uncertainty in these models. Gauge data, that is denser both spatially and temporally, would bring significant benefits to both flood and drought hydro-hazard identification.
The increasing impact of the changing climate means that past records can no longer be relied upon to give an indication of future events (Milly et al., 2008), particularly given the evidence that, even if mean precipitation or temperature does not change significantly, there will be an increase in extremes (Easterling et al., 2000). This phenomenon will consequently lead to an increase in both floods and droughts. If historical data collected over many decades can no longer be used, there is an even greater need for more dense and accurate data to support real-time and event-based predictions.
Given the complexity of natural systems, no analytic model can take into account all physical effects and all scales. The need to parameterise processes or to neglect them entirely means that there are inherent uncertainties in all models (Refsgaard et al., 2007). As discussed above it is now accepted that these uncertainties cannot be ignored when modeling natural systems (Pappenberger and Beven, 2006) and in turn this requires multiple substantiations of a particular model in order to cover the space of parameters (Hall et al., 2012). In view of this insight, there is a balance to be struck between model complexity and model run-time, meaning that in most cases a less complex model, that can be run multiple times in the time available, can generate greater understanding than one that contains more representation of physical phenomena. Bates (2022) sets this out as a modern version of Occam's Razor (Young et al., 1996; Forster, 2000) and also suggests that there is a bias amongst researchers to use models that are computationally feasible for comparison with laboratory studies or analytical solutions, but that are too computationally-intensive and over-engineered for full-scale problems. Overall, this points to the need for ensemble predications, which most of the papers in this review have not adopted, but which could be a future research direction.
Implications for decision-making
The majority of the reviewed papers state that the main aim of the study is to show a proof of concept for methods to support decision making. The main question for decision-makers is what advances are available to reduce the risk arising from future hydro-hazards, such that water managers are properly planning and adapting to the potential risk (Bates, 2022).
The reviewed papers advocate that modeling is one of the approaches to identify where hydro-hazards will happen and how these processes will develop. There are several challenges for the modeling extremes, perhaps the largest of which is data availability, because there are few historical recorded data on extreme events. Moreover, global and regional climate models have difficulties in properly accounting for precipitation, which can cascade through to the eventual projections. Additionally, the complexity of climate modeling chains is high, and uncertainty in inputs and model structure cascade through the chain. These factors can make outputs less routinely accessible by decision makers (Delgado et al., 2014), and can result in a reported lack of trust in modeling results by decision makers (Hall et al., 2012).
As discussed in this article, uncertainties are due to model parameter uncertainty, model definition and structure (Forster, 2000; Willis et al., 2019). In order to build trust in model outputs for decision-making purposes, approaches use ensemble predictions, which give a better range of models for both high flows and low flows, as seen in the case studies conducted by Cloke et al. (2013) and Coppola et al. (2021). All studies concluded that uncertainty of the input is unavoidable, and thus a better understanding of the uncertainty in modeling hazards and the uncertainty of their output can improve the trust of water managers in modeling approaches, and can lead to informed decisions. Of the analyzed papers herein, ~40% include uncertainty analysis, making reference to decision making. However, none of the papers show if such models were further used in practice by decision makers.
Multiple hazards
Any hydro-hazard analysis requires significance expertise and knowledge from the individuals involved, but “knowledge bias” (Gill and Malamud, 2014) can lead individuals to see their own area of focus as of paramount importance. This silo-based approach can lead in turn to a focus on just one hazard, rather than the full range of hazards such as floods, droughts, earthquake, extreme temperatures, pandemic, etc. However, many specialists do acknowledge the need for an integrated approach (De Angeli et al., 2022), but some can still see this as a hub-and-spoke system where the other systems need to integrate with their own as the central issue.
There are many links between hazards that can be taken into account and by way of example these include:
- Flooding and the water resource system;
- The impact of urban drainage systems on the transport system;
- Earthquake that leads to landslides and then lead to floods (De Angeli et al., 2022);
- The impact that flooding across vegetated areas has on the emission of CO2 (Richey et al., 2002);
- The impact that COVID lockdowns can have on emergency response to flooding (Simonovic et al., 2021).
The UN Sendai Framework for Disaster Risk Reduction (UNDRR, 2015) does state that multi-hazard approaches are needed for disaster risk reduction, but whilst much research has been carried out in recent decades, mature methods have not been set out or adopted (Wang et al., 2020). This remark is in part due to the complexity of the modeling on multiple hazards and the need to consider hazards that occur together spatially and temporally whilst not interacting, along with those that do interact and even where one hazard leads to another or at least increases the probability of another. Even once the hazards are assessed, there is then a need to work with a range of stakeholders in an integrated manner. Uncertainty, both aleatory and epistemic, is often considered in single hazard analysis (Rougier et al., 2013) but is more complex in the multi-hazard case and its treatment is not as mature.
Ultimately, society needs an approach that integrates many hazards, which requires clear leadership and compromise across specialists. Further, it has to be acknowledged that not all aspects can be included in an integrated approach and in each case the most significant hazards need to be identified and addressed. The latter requires detailed multi-disciplinary analysis that reflects the full range of interactions in the system that is impacted, such as that proposed at city level by McClymont et al. (2022). Such approaches need to include qualitative analysis based on case studies and experience, along with a quantitative approach based on analytical and AI (Artificial Intelligence) methods for identifying the key influences.
Global interconnections across impacts
Hydological hazards are interconnected, and so are the consequent impacts. Different sectors of society are affected in different ways. Individuals are at risk of death or injury during an event, and in the aftermath have to cope with: property damage and recovery costs; mental health challenges; interruptions to supply of goods and services; and potentially the need to migrate. Businesses also suffer damage to property along with: business interruption; loss of markets; and possible business failure (Coates et al., 2020). As well as their responsibility to citizens, governments have to face economic damage; loss of tax revenue; cost of recovery; and the challenges of migration. Investors face financial losses (Fiedler et al., 2021) and the cost of insurance claims. Across these impacts, it can be seen that there are many inter-connections and all these should be taken into account in risk analysis both to minimize the overall impact and to carry out comprehensive cost-benefit analyses.
Many of these impacts can go far beyond the locations where the hazard occurs. For example, supply chains can affect people on the other side of the globe (Haraguchi and Lall, 2015; Sheffi, 2020) and losses on investments and insurance are increasingly global. As the impact of a changing climate increases there will be more migration between regions causing further challenges for governments. This global aspect of flooding and droughts appears to be largely neglected in the literature reviewed here which found that most authors report impacts local to them. Whilst there is a strong focus on Europe (36% of the papers compared to 11% of the global population), there is proportionally less research on Asia (48% compared to 60% of the global population). The latter clearly has a significant and increasing impact on global trade.
This work looked into climatic hotspots: future research could also look at the exposure and vulnerability of those areas, focusing more on the actual potential impact on society and the ecosystem, moving from “hazard hotspots” to “risk hotspots”. In fact, a better definition of “hotspots” could be refined alongside a risk-based approach. The concept of risk encompasses hazard, exposure and vulnerability (Grossi and Kunreuther, 2005) and thus a risk-based approach seems sensible because in the future exposure and vulnerability are likely to change too (in addition to the hazards); for example, the world population will increase and with that the number and location of buildings in cities (change of exposure), or houses may follow a more robust design to hazard impact (change of vulnerability).
Future research needs
This work is the first global collection of identified hotspots for floods and droughts in the literature. This paper has highlighted the geographical areas where increasing hazards are anticipated. The climatic impact identified in the hotspots will require substantial and specific investments into mitigation and adaptation to develop both societal and ecological adaptive capacity (Szabo et al., 2016), which should be seen as interdependent and thus benefiting from integrated policy (Khan and Cundill, 2019). In these key hotspots, environmental indicators or frameworks (Rickels et al., 2016) could be applied for monitoring and tracking impact and implications of both climate and interventions (e.g., mitigation strategies). This work offers the “entry point” for further analysis and specific modeling to explore climatic consequences and resilience strategies, within cross-sectoral policy discussions; the database could be further enriched, by collecting more specific information (e.g., whether flood studies adopt hydrologic and/or hydrodynamic models). Its relevance is also in the possibility of cross-study learning and sharing that the global review underpins: for example, the climate community and networks may use this review for comparing methods and barriers at multiple levels (e.g., scale, location, etc.). However, this paper has highlighted that more research is needed in unrepresented areas of the world, such as Eastern Europe, Asia, the Middle East and Africa, as well as in the areas which are consistently being identified as future hotspots.
In this paper the analysis points toward three areas where future research should focus:
1. Uncertainty analyses and modeling: to understand the evolution of, and variability in, the hydro-hazards in the identified hotspots so far. For example, future studies could take an ensemble approach to future projections, thus specifically quantifying and communicating uncertainty across analyses and translating this into meaningful information for decision-makers.
2. Understanding of multi-hazard (compounding hydro-hazards in the remit of this paper), and identify potential hotspots associated with the interaction of such processes and the global interconnections of the associated impacts.
3. Defining the concept of “hotspots” alongside a risk-based approach: considering that the changes that will occur in the future to hazards will also be felt with changes to exposure and vulnerability.
Conclusion
This paper reviewed existing studies focusing on “hotspots” of hydro-hazards under global change; in the literature, the term “hotspot” generally refers to hazard only. Given this scope, all the studies focus on the hazard. However, the hazard itself is not an indication of the impact or negative consequences for the built environment, natural environment and human societies, since the consequences related to a hazard relate to the affected elements. The understanding of the hazard is the beginning of the assessment of impacts and the development of climate service activities.
The results of this paper suggest that while there has been significant progress over the last 11 years, more research is needed to understand the geographical spread of potential changes to hydro-hazard occurrence as a result of climate change. There are areas of the world which remain unstudied (e.g., Russia) and areas which consistently are highlighted as hotspots (e.g., Europe for both floods and droughts) that warrant further analysis. The papers analyzed indicated that new data sources, and methods which incorporate uncertainty offer opportunities for this developing research field. Similarly new research must look to understand multiple hazards, rather than one hazard in isolation, in the recognition that the interconnections between hazards can add complexity for planners and decision-makers. This requires work on joint probability of events. Finally, more analysis is needed on the combination of hazard, exposure and vulnerability and how these may all individually change in the future, and in combination lead to increasing risk.
Data availability statement
The original contributions presented in the study are publicly available. This data can be found here: https://doi.org/10.5523/bris.1b5yd1dy3deyj2ok2v5em94pac.
Author contributions
LB, IP, and MP conceptualized, designed the study, and undertook the screening. IP and LB undertook the search. YL, LB, and IP undertook the systematic analysis. IP and MP undertook the scientometric analysis and figure preparation. Final review and edits LB, NW, and MP. All authors undertook the analysis and draft paper write up.
Funding
LB was supported by the Engineering and Physical Sciences Research Council (ESPRC) LWEC (Living With Environmental Change) Fellowship (EP/N030419). MP was supported by the Engineering and Physical Sciences Research Council (ESPRC) LWEC (Living With Environmental Change) Fellowship (EP/R00742X/2). YL was supported by the China Scholarship Council-University of Bristol Joint PhD Scholarships Program (No. 201808420207). IP was funded by the European Union's Horizon 2020 Research and Innovation Programme EIFFEL project (No.101003518).
Conflict of interest
The authors declare that the research was conducted in the absence of any commercial or financial relationships that could be construed as a potential conflict of interest.
Publisher's note
All claims expressed in this article are solely those of the authors and do not necessarily represent those of their affiliated organizations, or those of the publisher, the editors and the reviewers. Any product that may be evaluated in this article, or claim that may be made by its manufacturer, is not guaranteed or endorsed by the publisher.
Supplementary material
The Supplementary Material for this article can be found online at: https://www.frontiersin.org/articles/10.3389/frwa.2022.879536/full#supplementary-material
References
Abdulai, P. J., and Chung, E.-S. (2019). Uncertainty assessment in drought severities for the Cheongmicheon watershed using multiple GCMs and the reliability ensemble averaging method. Sustainability, 11, 4283. doi: 10.3390/su11164283
AghaKouchak, A., Farahmand, A., Melton, F. S., Teixeira, J., Anderson, M. C., Wardlow, B. D., et al. (2015). Remote sensing of drought: Progress, challenges and opportunities. Rev. Geophy. 53, 452–480. doi: 10.1002/2014RG000456
Alfieri, L., Bisselink, B., Dottori, F., Naumann, G., de Roo, A., Salamon, P., et al. (2017). Global projections of river flood risk in a warmer world. Earths Fut. 5, 171–182. doi: 10.1002/2016EF000485
Arnell, N., Lowe, J. A., Bernie, D. J., Nicholls, R., Brown, S., Challinor, A., et al. (2019). The global and regional impacts of climate change under representative concentration pathway forcings and shared socioeconomic pathway socioeconomic scenarios. Environ. Res. Lett. 14, 84046. doi: 10.1088/1748-9326/ab35a6
Arnell, N. W., Kay, A. L., Freeman, A., Rudd, A. C., and Lowe, J. A. (2021). Changing climate risk in the UK: A multi-sectoral analysis using policy-relevant indicators. Clim. Risk Manag. 31. doi: 10.1016/j.crm.2020.100265
Bates, P. D. (2022). Flood Inundation Prediction. Annu. Rev. Fluid Mech. 54, 287–315. doi: 10.1146/annurev-fluid-030121-113138
Bautista Quispe, J,I, Campos, L. C., Masek, O., and Bogush, A. (2022). Use of biochar-based column filtration systems for greywater treatment: a systematic literature review. J. Water Proc. Eng. 28, 102908. doi: 10.1016/j.jwpe.2022.102908
Blümel, C., and Schniedermann, A. (2020). Studying review articles in scientometrics and beyond: a research agenda. Scientometrics 124, 711–728. doi: 10.1007/s11192-020-03431-7
Carrão, H., Naumann, G., and Barbosa, P. (2018). Global projections of drought hazard in a warming climate: a prime for disaster risk management. Clim. Dyn. 50, 2137–2155. doi: 10.1007/s00382-017-3740-8
Cloke, H. L., Wetterhall, F., He, Y., Freer, J. E., and Pappenberger, F. (2013). Modelling climate impact on floods with ensemble climate projections. Q. J. R. Meteorol. Soc. 139, 282–297. doi: 10.1002/qj.1998
Coates, G., Alharbi, M., Li, C., Ahilan, S., and Wright, N. (2020). Evaluating the operational resilience of small and medium-sized enterprises to flooding using a computational modelling and simulation approach: a case study of the 2007 flood in Tewkesbury. Philos. Trans. A. Math. Phys. Eng. Sci. 378, 20190210. doi: 10.1098/rsta.2019.0210
Collet, LHarrigan, S., Prudhomme, C., Formetta, G., and Beevers, L. (2018). Future hot-spots for hydro-hazards in Great Britain: a probabilistic assessment. Hydrol. Earth Syst. Sci. 22, 5387–5401. doi: 10.5194/hess-22-5387-2018
Coppola, E., Raffaele, F., Giorgi, F., et al. (2021). Climate hazard indices projections based on CORDEX-CORE, CMIP5 and CMIP6 ensemble. Clim. Dyn. 57, 1293–1383. doi: 10.1007/s00382-021-05640-z
CRED UNDRR (2021). 2020: The Non-Covid Year in Disasters. Brussels, CRED. Available online at: https://www.undrr.org/publication/2020-non-covid-year-disasters (accessed February 16, 22).
Dankers, R., Arnell, N., Clark, D., Falloon, P., Fekete, B., Gosling, S., et al. (2014). Changes in flood hazard in the ISI-MIP ensemble. Proc. Natl. Acad. Sci. (2014) 111, 3257–3261. doi: 10.1073/pnas.1302078110
De Angeli, S., Malamud, B., Rossi, L., Taylor, F., Trasforini, E., and Rudari, R. (2022). A multi-hazard framework for spatial-temporal impact analysis. Int. J. Dis. Risk Red. 73, 102829. doi: 10.1016/j.ijdrr.2022.102829
de Ruiter, M. C., Couasnon, A., van den Homberg, M. J. C., Daniell, J. E., Gill, J. C., and Ward, P. J. (2020). Why we can no longer ignore consecutive disasters. Earths Fut. 8, e2019EF001425. doi: 10.1029/2019EF001425
Delgado, J. M., Merz, B., and Apel, H. (2014). Projecting flood hazard under climate change: an alternative approach to model chains. Nat. Hazard. Earth Syst. Sci. 14, 1579–1589. doi: 10.5194/nhess-14-1579-2014
Déqué, M., Somot, S., Sanchez-Gomez, E., et al. (2012). The spread amongst ENSEMBLES regional scenarios: regional climate models, driving general circulation models and interannual variability. Clim Dyn. 38, 951–964. doi: 10.1007/s00382-011-1053-x
Didovets, I., Krysanovab, V., Bürgera, G., Snizhkoc, S., Balabukhd, V., Bronsterta, A., et al. (2019). Climate change impact on regional floods in the Carpathian region. J. Hydrol. Reg. Stud. 22, 100590. doi: 10.1016/j.ejrh.2019.01.002
Easterling, D. R., Evans, J. L., Groisman, P. Y., Karl, T. R., Kunkel, K. E., and Ambenje, P. (2000). Observed variability and trends in extreme climate events: a brief review. Bull. Am. Meteorol. Soc. 81, 417–426. doi: 10.1175/1520-0477(2000)081<0417:OVATIE>2.3.CO;2
Farr, T. G., Rosen, P. A., Caro, E., Crippen, R., Duren, R., Hensley, S., et al. (2007). The shuttle radar topography mission. Rev. Geophy. 45, RG2004. doi: 10.1029/2005RG000183
Fiedler, T., Pitman, A. J., Mackenzie, K., et al. (2021). Business risk and the emergence of climate analytics. Nat. Clim. Change. 11, 87–94. doi: 10.1038/s41558-020-00984
Forster, M. R. (2000). Key concepts in model selection: performance and generalizability. J. Math. Psychol. 44, 205–231. doi: 10.1006/jmps.1999.1284
Forzieri, G., Feyen, L., Rojas, R., Flörke, M., Wimmer, F. A., et al. (2014). Ensemble projections of future streamflow droughts in Europe. Hydrol. Earth Syst. Sci. 18, 85–108. doi: 10.5194/hess-18-85-2014
Gaur, A., Gaur, A., and Simonovic, S. (2018). Future changes in flood hazards across Canada under a changing climate. Water 10, 1441. doi: 10.3390/w10101441
Gaur, A., Gaur, A., Yamazaki, D., and Simonovic, S. (2019). Flooding related consequences of climate change on Canadian cities and flow regulation infrastructure. Water 11, 63. doi: 10.3390/w11010063
Gill, J. C., and Malamud, B. D. (2014). Reviewing and visualizing the interactions of natural hazards, Rev. Geophys., 52, 680–722, doi: 10.1002/2013RG000445
Gill, J. C., and Malamud, B. D. (2016). Hazard interactions and interaction networks (cascades) within multi-hazard methodologies. Earth Syst. Dyn. 7, 659–679. doi: 10.5194/esd-7-659-2016
Grossi, P., and Kunreuther, H. (2005). Catastrophe Modeling: A New Approach to Managing Risk. Huebner International Series on Risk, Insurance and Economic Security, Springer. Avialable online at: https://www.springer.com/gp/book/9780387230825 (accessed December 13, 2021).
Hall, J. W., Lempert, R. J., Keller, K, Hackbarth, A., Mijere, C., and McInerney, D.J. (2012). Robust climate policies under uncertainty: a comparison of robust decision making and info-gap methods. Risk Anal. 32, 1657–1672. doi: 10.1111/j.1539-6924.2012.01802.x
Haraguchi, M., and Lall, U. (2015). Flood risks and impacts: a case study of Thailand's floods in 2011 and research questions for supply chain decision making. Int. J. Disast. Risk Reduct. 14:256–272. doi: 10.1016/j.ijdrr.2014.09.005
Helaire, L. T., Talke, S. A., Jay, D. A., and Chang, H. (2020). Present and future flood hazard in the lower columbia river estuary: changing flood hazards in the portland-vancouver metropolitan area. J Geophy Res Oceans. 125, e2019JC015928. doi: 10.1029/2019jc015928
IPCC (2007). Climate change 2007: synthesis report. Core Writing Team, Pachauri, R.K., and Reisinger, A., editors. Contribution of Working Groups I, II and III to the Fourth Assessment Report of the Intergovernmental Panel on Climate Change. Geneva: IPCC. p. 104.
IPCC (2014). Climate change 2014: synthesis report. Core Writing Team, Pachauri, R.K., and Reisinger, A., editors. Contribution of Working Groups I, II and III to the Fifth Assessment Report of the Intergovernmental Panel on Climate Change. Geneva: IPCC. p. 151.
Khan, A. S., and Cundill, G. (2019). Hotspots 2.0: toward an integrated understanding of stressors and response options. Ambio 48, 639–648. doi: 10.1007/s13280-018-1120-1
Lane, R. A., and Kay, A. L. (2021). Climate change impact on the magnitude and timing of hydrological extremes across great Britain. Front. Water 3, 684982. doi: 10.3389/frwa.2021.684982
Lompi, M., Mediero, L., and Caporali, E. (2021). Future flood hazard assessment for the city of Pamplona (Spain) using an ensemble of climate change projections. Water 13, 792. doi: 10.3390/w130607921
Lu, J., Carbone, G. J., and Grego, J. M. (2019). Uncertainty and hotspots in 21st century projections of agricultural drought from CMIP5 models. Sci. Rep. 9, 4922. doi: 10.1038/s41598-019-41196-z
Lung, T., Dosio, A., Becker, W., Lavalle, C., and Bouwer, L. M. (2013). Assessing the influence of climate model uncertainty on EU-wide climate change impact indicators. Clim. Change 120, 211–227. doi: 10.1007/s10584-013-0825-1
McClymont, K., Bedinger, M., Beevers, L., Visser-Quinn, A., and Walker, G. (2022). Understanding urban resilience with the urban system abstraction hierarchy. Sustain. Cities Soc. 80, 103729. doi: 10.1016/j.scs.2022.103729
Milly, P. C. D., Betancourt, J., Falkenmark, M., Hirsch, R. H., Kundzewicz, Z. W., Lettenmaier, D. P., et al. (2008). Climate change: Stationarity is dead: Whither water management? Science 319, 573–574. doi: 10.1126/science.1151915
Mondal, S. K., Huang, J., Wang, Y., Su, B., Zhai, J., Tao, H., et al. (2021). Doubling of the population exposed to drought over South Asia: CMIP6 multi-model-based analysis. Sci. Total Environ. 771, 145186. doi: 10.1016/j.scitotenv.2021.145186
Pappenberger, F., and Beven, K. J. (2006). Ignorance is bliss: on seven reasons not to use uncertainty analysis. Water Resour. Res. 42, W05302. doi: 10.1029/2005WR004820
Prudhomme, C., Giuntoli, I., Robinson, E. L., Clark., D. B, Arnell, N. W., Dankers, R., et al. (2014). Hydrological droughts in the 21st century, hotspots and uncertainties from a global multimodel ensemble experiment. Proc. Nat. Acad. Sci. U S A 111, 3262–7. doi: 10.1073/pnas.1222473110
Refsgaard, J. C., van der Sluijs, J. P., Højberg, A. L., and Vanrolleghem, P. A. (2007). Uncertainty in the environmental modelling process – A framework and guidance. Environ. Model. Softw. 22, 1543–1556. doi: 10.1016/j.envsoft.2007.02.004
Richey, J. E., Melack, J., Aufdenkampe, A., Ballester, V. M., and Hess, L. L. (2002). Outgassing from Amazonian rivers and wetlands as a large tropical source of atmospheric CO2. Nature 416, 617–620. doi: 10.1038/416617a
Rickels, W., Dovern, J., Hoffmann, J., Quaas, M. F., Schmidt, J. O., and Visbeck, M. (2016). Indicators for monitoring sustainable development goals: an application to oceanic development in the European Union. Earths Fut. 4, 252–267. doi: 10.1002/2016EF000353
Rojas, R., Feyen, L., Bianchi, A., and Dosio, A. (2012). Assessment of future flood hazard in Europe using a large ensemble of bias-corrected regional climate simulations. J. Geophy. Res. Atmos. 117, D17109. doi: 10.1029/2012jD017461
Rougier, J., Sparks, S., and Hill, L. (2013). Risk and Uncertainty Assessment for Natural Hazards. In: Rougier, J., Sparks, S., and Hill L., editors. Risk and Uncertainty Assessment for Natural Hazards (p. Iii). Cambridge: Cambridge University Press.
Samantaray, A. K., Ramadas, M., and Panda, R. K. (2020). Assessment of impacts of potential climate change on meteorological drought characteristics at regional scales. Int. J. Climatol. 41, E319–E341. doi: 10.1002/joc.6687
Sheffi, Y. (2020). Who Gets What When Supply Chains are Disrupted. MIT Sloan Management Review. Available online at: https://sloanreview.mit.edu/article/who-gets-what-when-supply-chains-are-disrupted/ (accessed February, 16 2022).
Simonovic, S. P., Kundzewicz, Z. W., and Wright, N. (2021). Floods and the COVID-19 pandemic—A new double hazard problem. WIREs. Water 8, e1509. doi: 10.1002/wat2.1509
Szabo, S., Nicholls, R. J., Neumann, B., Renaud, F. G., Matthews, Z., Sebesvari, Z., et al. (2016). Making SDGs Work for Climate Change Hotspots. Environ. Sci. Pol. Sustain. Dev. 58, 24–33. doi: 10.1080/00139157.2016.1209016
Tan, G., Ayugi, B., Ngoma, H., and Ongoma, V. (2020). Projections of future meteorological drought events under representative concentration pathways (RCPs) of CMIP5 over Kenya, East Africa. Atmos. Res. 246, 105112. doi: 10.1016/j.atmosres.2020.105112
UN (2022). 2021 Floods: UN Researchers Aim to Better Prepare for Climate Risks (unric.org). Available online at: https://unric.org/en/2021-floods-un-researchers-aim-to-better-prepare-for-climate-risks/ (accessed August 3, 2022).
UNDRR (2015). Sendai framework for disaster risk reduction, in: World Conference on Disaster Risk Reduction, 14−18 March 2015, Sendai, Japan, United Nations Office for Disaster Risk Reduction, 2015. Available online at: https://www.preventionweb.net/files/43291_sendaiframeworkfordrren.pdf (accessed August 15, 2022).
Van Eck, N. J., and Waltman, L. (2011). Text mining and visualization using VOSviewer. ISSI Newsletter 7, 50–54. doi: 10.48550/arXiv.1109.2058
Visser-Quinn, A., Beevers, L., Collet, L., Formetta, G., Smith, K., Wanders, N., et al. (2019). Spatio-temporal analysis of compound hydro-hazard extremes across the UK. Adv. Water Res. 130, 77–90 doi: 10.1016/j.advwatres.2019.05.019
Visser-Quinn, A., Beevers, L., Lau, T., and Gosling, R. (2021). Mapping future water scarcity in a water abundant nation: near-term projections for Scotland. Clim Risk Manag. 32, 100302. doi: 10.1016/j.crm.2021.100302
Wang, J., He, Z., and Weng, W. (2020). A review of the research into the relations between hazards in multi-hazard risk analysis. Nat. Hazard. 104, 2003–2026. doi: 10.1007/s11069-020-04259-3
Wellmann, T., Lausch, A, Andersson, E., Knapp, S., Cortinovis, C, Jache, J., et al. (2020). Remote sensing in urban planning: contributions towards ecologically sound policies? Landsc. Urban Plan. 204, 103921. doi: 10.1016/j.landurbplan.2020.103921
Wilby, R. (2019). A global hydrology research agenda fit for the 2030s. Hydrol. Res. 50, 1464–1480. doi: 10.2166/nh.2019.100
Willis, T., Wright, N., and Sleigh, A. (2019). Systematic analysis of uncertainty in 2D flood inundation models. Environ. Model. Softw. 122:104520. doi: 10.1016/j.envsoft.2019.104520
Young, P., Parkinson, S., and Lees, M. (1996). Simplicity out of complexity in environmental modelling: Occam's razor revisited. J. Appl. Stat. 23, 165–210. doi: 10.1080/02664769624206
Keywords: floods, droughts, climate change, hotspots, hydro-hazard
Citation: Beevers L, Popescu I, Pregnolato M, Liu Y and Wright N (2022) Identifying hotspots of hydro-hazards under global change: A worldwide review. Front. Water 4:879536. doi: 10.3389/frwa.2022.879536
Received: 19 February 2022; Accepted: 17 August 2022;
Published: 14 September 2022.
Edited by:
Subimal Ghosh, Indian Institute of Technology Bombay, IndiaReviewed by:
Rishi Sahastrabuddhe, Indian Institute of Technology Bombay, IndiaAndrew Hursthouse, University of the West of Scotland, United Kingdom
Boulent Imam, University of Surrey, United Kingdom
Shrabani Sailaja Tripathy, Indian Institute of Technology Bombay, India
Copyright © 2022 Beevers, Popescu, Pregnolato, Liu and Wright. This is an open-access article distributed under the terms of the Creative Commons Attribution License (CC BY). The use, distribution or reproduction in other forums is permitted, provided the original author(s) and the copyright owner(s) are credited and that the original publication in this journal is cited, in accordance with accepted academic practice. No use, distribution or reproduction is permitted which does not comply with these terms.
*Correspondence: Lindsay Beevers, bC5iZWV2ZXJzQGVkLmFjLnVr
†ORCID: Lindsay Beevers orcid.org/0000-0002-1597-273X
Ioana Popescu orcid.org/0000-0002-1009-5424
Maria Pregnolato orcid.org/0000-0003-0796-9618
Yuexiao Liu orcid.org/0000-0002-9026-3108
Nigel Wright orcid.org/0000-0002-1289-2830