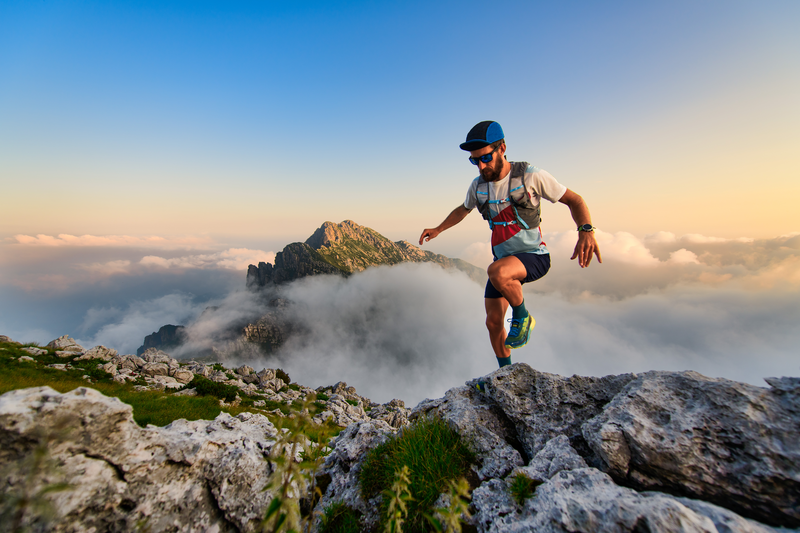
95% of researchers rate our articles as excellent or good
Learn more about the work of our research integrity team to safeguard the quality of each article we publish.
Find out more
ORIGINAL RESEARCH article
Front. Water , 29 April 2022
Sec. Water and Climate
Volume 4 - 2022 | https://doi.org/10.3389/frwa.2022.879001
This article is part of the Research Topic Hydrosphere-Cryosphere Interactions in the Himalayas under Climate Change View all 6 articles
Rambiara River basin, a sub-basin of the Upper Indus, is dotted with fluvial and glacial geomorphic landforms. The presence of large number of glacial landforms like moraines, cirques, aretes, U-shaped valleys, etc. reflects the enormous erosive power of the past glaciers. During the Last Glacial Maximum (LGM), glaciers on average were 200 m thick and extended more than 10 km from the present-day cirque marks. Rock glaciers outnumber the clean glaciers that cover an area of 0.22 and 1.25 km2, respectively, in the basin. Glacial lakes are the prominent features in the higher reaches of the basin and occupy an area ranging from 0.01 to 0.70 km2. Downstream, the river is characterized by large channel width, anomalous sinuosity, braided pattern, and the presence of unpaired fluvial terraces. The large channel width depicts the enormous discharge from the glacial- and snow-melt during the Late Pleistocene when glaciers covered most of the Pir Panjal. After LGM, the glacier cover and the river discharge significantly decreased as highlighted by a large number of braided bars and the narrow stream to which the river is reduced. The warming trends observed across the Himalayas encompassing the Pir Panjal Range since the last century has further contributed significantly to the glacier recession in the basin.
The Quaternary was a period of major climatic changes all over the world with glacial and interglacial phases (Past Interglacials Working Group of PAGES, 2016; Dar and Zeeden, 2020). The fluctuating climate and the resultant advance and retreat of the glaciers- particularly mountain glaciers- considerably altered the geomorphic architecture and the rate of uplift of most of the mountain ranges (Molnar and England, 1990; Dar et al., 2017; Joya et al., 2021). The climatic oscillations also had a great influence on the production and deposition of mineral dust and the formation of soils (Dar and Zeeden, 2020; Zeeden et al., 2021). For example, during cold glacial periods, loess deposition took place, and during interglacial warm periods, deep nature soils developed with dense forest vegetation (Dar et al., 2015a,b). Besides, the dynamics of the mountain glaciers and the concurrent climatic fluctuations have also directly or indirectly influenced the geomorphic response of fluvial systems and consequently changed the hydrological regimes from small streams to large river basins. In addition to their impact on the hydrological regimes of the river basins, the glaciers along with the melting of the seasonal snow cover form a major component of the surface water resource (Kumar et al., 2022) and also form a significant component of the moisture that will be available for runoff in the future (Dar and Ramshoo, 2013; Młyński et al., 2021). Various studies have highlighted that the cryosphere in the Himalayas is thinning and melting at a faster pace than the global average (Wang and Zhang, 2013; Dixit et al., 2021). A very high recession rate has been reported from the glaciers in the Kashmir Himalayas (Murtaza and Romshoo, 2017; Romshoo et al., 2020). The accelerated melting of the cryosphere in the region has already reached a threshold limit resulting in a significant decrease in the discharge of Himalayan rivers (Marazi and Romshoo, 2018; Młyński et al., 2021; Kumar et al., 2022).
Therefore, understanding the interaction between the melting cryosphere and the fluvial system is imperative because glaciers affect river behavior significantly, especially during interglacial periods. During these periods meltwater is greatly increased in the fluvial systems, which increases the sediment supply and influences geomorphic work, aggradation, and degradation, of the rivers. However, the river geomorphic processes may vary spatially and temporally in accordance with the thickness and extent of the melting cryosphere, sediment supply, gradient of the rivers, and the tectonics of the area (Antoine et al., 2000).
The Pir Panjal Range (PPR) in the NW Himalaya was heavily glaciated during the Quaternary period (Paul et al., 2021). The glaciers advanced much below the present-day cirques as revealed by huge moraine ridges, deeply carved glacial troughs and ubiquitous cirques across the strike of the mountain range, and the presence of thick fluvioglacial sediments downstream of the mountain aprons (Dar et al., 2014; Paul et al., 2021). The meltwater from the seasonal snow and the cryosphere maintains the flow of numerous rivers, like Rambiara, Vishav, Romushi, Doodhganga, Ningli, etc. that originate from range. However, melting of the glaciers in response to the atmospheric warming all through the Holocene, especially during the recent decades (Romshoo et al., 2017), increased the discharge of river systems and modified the geomorphic setup of the region.
Although much research focused on the river response to active tectonics in the Kashmir Himalaya (Dar et al., 2017; Romshoo et al., 2017), the studies on the relationship between climate change, glacial fluctuations, and river response are sparse. This encouraged us to study the response of the fluvial system to the melting cryosphere and the main focus of paper is to examine the extent and the geomorphic work of the glaciers and how the fluvial system has responded to the melting glaciers in the PPR. Pertinently, PPR is one of the rapidly rising mountain ranges of the Himalaya and has witnessed multiple glacial advances during the Cenozoic period (Agrawal et al., 1989; Paul et al., 2022). The glaciers have been under recession as revealed by deglaciated cirques and troughs of the region (Paul et al., 2021). In the first section, we focus on the mapping of the glacial extent and the glacial geomorphic landforms in the catchment. In the second section, we examine the complex pattern of fluvial response as glaciers can influence both the sediment supply and water discharge. We carried out quantitative measurements and calculated the geomorphic indices that are useful for identifying a particular characteristic of the fluvial system and that have been tested also elsewhere (Bull, 1984).
The Rambiara River basin lies between latitudes 33° 33 and 33° 54 N and longitudes 74° 30 and 75° 00 E, toward the south of the Kashmir Valley, India covering an area of 660 km2 (Figure 1). Originating from the snow and glacier resources of the Pir Panjal Range, the Rambiara has a course of 60 km and joins the trunk stream, River Jhelum in the axial part of the Kashmir Valley, near Nyaiyun. The Rambiara River is a high gradient river and drops vertically at an average of 41 m per km (Ahmad et al., 2013). In the upper reaches, the river is a bedrock rock-river traversing through the hard rock lithology and downstream it turns into an alluvial river. Based on the geomorphological observations, the fluvial aggradation might have occurred during the glacial advances (eroding and transporting the sediments downstream) and during periods of deglaciation (when the meltwater eroded and mobilized the glacial sediments). Geologically, the upper reaches of the basin are dominated by the Panjal Volcanics and Salkhala series of rocks (Figure 2). The lower reaches are dominated by fluvial-glacial-lacustrine sediments, loess-paleosol sequences, and recent alluvium (Dar et al., 2015c; Stojanovic et al., 2016). The fluvial-glacial-lacustrine sediments derived from the surrounding glacial-covered mountain ranges, known as Karewa Group were deposited in a lake (Karewa Lake), which previously occupied the whole valley. These sediments of Early Pliocene to Late Pleistocene age, range in thickness from a few meters to more than 500 m along the river cut banks. The Karewa deposits are thick along the Pir Panjal flank and decrease in thickness toward the Greater Himalayan flank of the valley (Dar et al., 2015c). The Karewas are divided into Hirpur Formation, Nagum Formation, and Dilpur Formation (Bhatt, 1982). The Hirpur Formation is further subdivided into Dubjan, Rambiara, and Methowian Members. Rambiara Member, named after the Rambiara River, comprises a thick sequence of glacial-fluvial sediments with thickness reaching up to 200 m resting over the very old Early Pliocene Dubjan Member (Bhatt, 1982). The Hirpur Formation is in turn overlain by the Nagum Formation, which comprises glacial wash and morainic sediments of 3rd and 4th glaciations (Wadia, 1942; Paul et al., 2021) representing major deglaciation periods. The four main climatic oscillations reported from the glacial deposits of the Karewa Group are also confirmed from moraine deposits of different glacial advances (Paul et al., 2022) and loess-paleosol sequences (Bronger et al., 1987).
Figure 1. Relief map of the Kashmir valley and the adjoining areas showing the location of the study area (Rambiara Basin).
Figure 2. Geological map of the Rambiara Basin and adjoining area showing Karewa deposits and major rock formations. The faults/lineaments present in and around the basin are also shown in the map.
Numerous small-scale lineaments/faults along with the Balapora thrust fault cut across the basin (Figure 2). These faults follow the general trend of the major faults (Main Boundary Thrust, Main Central Thrust, etc.) bounding the Kashmir Valley (Paul et al., 2022). The climate of the Kashmir Valley encompassing the Rambiara basin is dominated by winter precipitation, mostly in the form of snow. The effect of Western Disturbances is prevalent during the winter and spring seasons and ISM cause the summer rainfall (Dar et al., 2015a). The average annual precipitation and average annual temperatures of the Kashmir Valley encompassing the Rambiara basin are 710 mm and 13.5°C, respectively (Dar et al., 2013).
In this study, we used Google Earth Imagery, Arc GIS, Advanced Spaceborne Thermal Emission and Reflection Radiometer (ASTER), Global Digital Elevation Model (GDEM). The methodology follows the identification of glacial and river geomorphic features from Google Earth Imagery and Digital Elevation Model (DEM). Field validation and photographic documentation of the glacial and river geomorphic features was carried out, wherever possible. Measurements and quantification of the glacial geomorphic features were carried out in terms of the extent and thickness of the past glaciers. Measurement of river-width variations, braid bars, former river terraces, and the calculation of geomorphic indices and other parameters was carried out in Arc GIS using Google Earth imagery and DEM. This was followed by the interpretation of the river geomorphic features and geomorphic indices in terms of river response to changing fluvial conditions. The detailed methodology adopted for glacier mapping, understanding the effect of deglaciation, and the consequent increase in sediment load on fluvial system behavior is discussed below.
Multispectral Landsat satellite data of Landsat Thematic Mapper (TM) (1992) and Landsat Operational Land Imager (OLI) from the year 2020 were used to assess the current status as well as the spatio-temporal variations of the glaciers in the Rambiara basin. For the selection of imageries, care was taken to choose the snow and cloud-free imageries for the delineation of the glacier boundaries. Visual interpretation in FCC (False-color composite) was used to identify glacier boundaries and differentiate between glaciated and non-glaciated regions (Paul et al., 2002; Romshoo et al., 2022). The origin of the glacier melt stream immediately at the tongue of the glacier, also known as the snout, was used to mark the snouts of the glaciers. The glacier boundaries were delineated manually using the on-screen digitization in the GIS environment. The mapping and interpretation of the glacier boundaries were further complimented by the use of high-resolution Google Earth, Bing Maps, Planet images, and ASTER GDEM wherever available.
To highlight the irregularities in the channel path, the parameter used is the Sinuosity index, a measure of the deviation of a river from its ideal path of movement, which was calculated for various reaches of the Rambiara River. Mathematically, it is calculated by the formula: SI = CL/SL, where CL = Actual Channel Length between two points (source and mouth), SL = Straight line length between the same two points. SI is 1.0 if there is no shifting of the channel at all and more than 1.0 in the case of river shifting. Meandering channels have higher SI values (Wolman and Miller, 1960). Practically, the straight-line path is not possible because the river is usually affected by a number of factors, like sediment deposition, river discharge, debris load, etc., which causes it to deviate from the straight-line path. Straight streams possess essentially straight banks with less sinuosity, but the river affected by varied geomorphic processes exhibits variable sinuosity in different reaches. We calculated the Braided index for the river to measure the degree of braiding. It is calculated as; Bi = 2 (Total bar length)/Reach Length. A value less than one represents a single channel, whereas values greater than five reflect intensely braided rivers. The Bi values <1 occur for a single channel, whereas a value > 5 reflects intensely braided rivers (>65% degree of braiding). The intermediate values (1–5) reflect 5–65% degree of braiding.
The Lateral Entrenchment ratio (ER) was also calculated to determine the overall impact of channel sinuosity on channel erosion and channel shifting. It is the ratio between lengths of the two banks of a river and is expressed as; ER = LL/LR, where LL is the length of the left bank, LR is the length of the right bank. The ER ratio is used to identify the amount of bank shifting of a river. If the value of the entrenchment ratio is 1, it means no shifting of banks. If the value is >1, it means the channel has shifted toward the left bank and if the value is <1, it indicates the channel has shifted toward the right bank within a given reach of the river. Besides, stream length gradient index was used for quantitative characterization of stream gradient conditions and the evaluation of the relationship between rock resistance, topography, and length of the stream (Keller and Pinter, 2002; Zhang et al., 2011). In landscape evolution, it is assumed that stream profiles adjust quite rapidly to the rock resistance. Commonly high SL index values are present where rivers cross hard rock and low values where softer rocks are present (Hack, 1973; Keller and Pinter, 2002). Stream length gradient index is calculated using the following formula; SL = (ΔH/ΔL)*L where SL is the stream length gradient index, L is the total channel length from the midpoint of the reach upstream where the index is calculated to the highest on the channel, ΔH/ΔL is the channel slope or gradient of the reach, and ΔH represents the change in elevation for a particular channel of the reach with respect to ΔL that symbolizes the length of the reach.
Besides, the mountain front sinuosity index (Smf) was used to highlight the post deglaciation rebound of the mountain range. It represents the battle between erosional forces that tend to produce an irregular mountain front and tectonic/isostatic forces that tend to produce a straight mountain front (Kaewmuangmoon et al., 2008; Jaan et al., 2015). It is expressed as; Smf = Lmf Ls, where Lmf is the length along the edge of the mountain-piedmont junction, Ls is the overall length of the mountain front.
The river terraces preserved on both the banks were analyzed to ascertain the former extents of the river and also to evaluate the vertical incision after deglaciation. The combination of the techniques used in this study will provide a basic podium for understanding the fluvial-glacial interaction in the region. This is because the fluvial-glacial landscape is shaped by the advance and retreat of glaciers, flow of meltwater, ice, sediments, and also by the vertical uplift post the glacial recession (Cordier et al., 2017).
Landsat satellite data revealed a total of 13 small glaciers in the Rambiara basin. These glaciers are characterized by their relatively small size ranging from 3.40 to 27 ha with an average size of 10.75 ha. The glaciers of the basin are further characterized by their high altitudinal snout, situated between 4,000 to 4,320 m a.s.l. The dominant aspect of the glaciers in the basin is N and NE facing slopes, whereas the slope of the glaciers ranges between 17 to 28° with an average slope of 21°. The analysis of satellite data showed that the areal extent of glaciers in the basin has receded significantly from 1992 to 2020. The total area of the glaciers in 1992 was 139.78 ha which shrunk to 125.10 ha by 2020. Therefore, the basin has witnessed deglaciation of about 15 ha or 10.50% during the last 28 years indicating an area loss at a rate of 0.38% a−1. The mean length change of all analyzed glaciers between 1992 and 2020 was 65 m (2.32 m a−1).
The prominent erosional and depositional landforms present in the basin include moraines, trimlines, U-shaped valleys, outwash plains, erratic boulders, cirques, tarns, horsn, aretse, kettle lakes, glacial lakes etc. Such features are the direct piece of evidence of glaciation/deglaciations observed across the basin (Figure 3). There are about 40 cirques present in the basin located near the drainage divide, with the area ranging from 0.05 to 3.60 km2 with a total area of 38.40 km2. The presence of cirques suggests extensive alpine-style glaciation observed in the Kashmir Himalaya during the Quaternary. The majority of the deglaciated cirques harbor a tarn lake and 4 well-developed horns are also observed in the basin. Glacial lakes are the prominent feature in the basin ranging in size from 0.01 to 0.70 km2 with an average area of 0.175 km2. These lakes are observed in the cirques and in the valleys carved by glaciers (Figure 4). A few kettle lakes are also present in the glacial outwash with the size ranging from 164 to 2,003.5 m2. The glacial troughs down the snout position of the rock glaciers are predominantly U-shaped with varying depth and width. The development of a U-shaped valley is controlled by the weight and velocity of the overlying ice and the bedrock lithology (Fu and Harbor, 2011). The depth of the U-shaped valleys observed in the area suggests that the glaciers in the range have been more than 200 m thick that enabled them to carve deep into the valleys. The mechanism of rock scouring and excavation by glacier action has paved way for the development of overdeepenings, a manifestation of which is observed in the form of a few over-deepened troughs and cirque lakes. A few tributary valleys with their heads marked by the deglaciated cirques, “hang” above the main glacial trough. Trimlines, representing the most recent high-ice stands along the walls of glacial valleys, are observed in the basin. Besides, 40 arêtes associated with present cirque/rock- and paleo- glaciers are observed in the basin, suggesting that after the fragmentation into valley glaciers due to climate change, the glaciers scoured and eroded their respective valleys. Most of the arête ridges are smooth reflecting that glaciers modified these ridges during the LGM.
Figure 3. (A) Fluvio-glacial geomorphic map of the Rambiara basin showing the river course and the glacial geomorphic features in the upper reaches, (B) the zoomed-in map of the glaciated terrain showing the main- glacial geomorphic features.
Figure 4. Google Earth image (a) shows lateral moraines (LM) of different glacial stages (4 stages) and the glacial trough, (b) shows the glacial sculptured landscape. Some of the prominent features are labeled are cirques (C), rock glacier (RG), tarn (T), overdeepening (OD), lateral moraines (LM), arete ridges (AR), trim line (TL), glacial trough.
Lateral moraines of varying size and height are the prominent depositional landforms preserved across the basin. Pertinently moraine ridges formed during the glacier movement, if well preserved, provide vital pieces of evidence of the past extent and the thickness of the glaciers (Huggett, 2007; Mehta et al., 2011; Sheinkman, 2016; Dar et al., 2021; Murtaza et al., 2021). Some of the well-preserved lateral moraines are preserved much downstream from the current snout position of the cirque and rock glaciers. Length of lateral moraines ranges in size between 123 and 1,153 m and the elevation of the lateral moraines measured from the bottom of the U-shaped valleys ranges between 132 and 1,173 m with an average elevation of about 515.6 m. Lots of recent rock glaciers exist in the deglaciated cirques and valleys of the range. The total number of glaciers and rock glaciers present are 13 and 59, respectively, with the average area of glaciers about 0.10 km2 and rock glaciers having an average area of about 0.22 km2. Besides, a few paleo-rock glaciers are also found. Some well-developed debris cones, alluvial fans, and outwash plains were also mapped in the area. Twenty-six alluvial fans were observed in the upper reaches of the basin. The size of the alluvial fans ranges from 0.2 ha to more than 150 ha. Outwash plains are dotted with kettle holes reflecting continuous deglaciation owing to the current global warming. Loess—a product of glacial environment- intercalated with paleosol profiles are also observed downstream of the basin. Pertinently, loess represents cold and arid glacial climate and the paleosols represent warm humid interglacial climate (Dar and Zeeden, 2020). The effect of glaciotectonics is also visible from vertical incisions and various knickpoints observed in the longitudinal river profile (Figure 5).
Figure 5. (a) showing the longitudinal profile of the Rambiara river and the seven reaches into which the river was divided for calculating various geomorphic indices. Note the location of Balapur fault and the presence of other convexities in the longitudinal profile, the braid bars along a small reach are also shown, (b) the braid bar formed along the trace of the Balapur fault. The blue arrow shows the direction of river flow and (c) the vertical incision observed along the banks of the river near the Hirpur Village.
The braided index (Bi) measures the degree to which bars and/or islands separate multiple flow paths (Table 1). It is evident from the Bi, that the river is highly braided in downstream reaches, 4, 5, and 6. The Rambiara River has responded to the slight changes in discharge and sediment load, the signatures of which are present in the form of unstable banks (Figure 6), unpaired terraces, river bank shifting, entrenchment, and river sinuosity. The ER values obtained suggest that in reaches 1, 3, 4, and 7 the river has slightly shifted toward the left bank (Table 1). ER values of 2 and 6 reach indicate that there is no bank shifting and the value of reach 7 shows that the river has shifted toward the right bank. The shifting of the river course has resulted in the sinuous nature of the river.
Figure 6. (a) showing the braided pattern of the Rambiara river near Shopian town, (b) the Google Earth image showing the unpaired terraces near the Hirpur Village, (c) river terrace and (d) the loess deposits blanketing the river terraces near Shopian town.
The longitudinal profile of the Rambiara River deviates significantly from a simple concave-up shape. The most remarkable feature of the profile is numerous convexities (knickpoints), especially in the upstream reaches of the river (Figure 5a). While it is obvious that most of these convexities are associated with the braid bars, few are associated with the faults that cut across the river (Figure 2). The stream length gradient index of the river calculated at 15 locations ranges from 36.1 to 2,256.2. The higher SL indicates either hard rock base or relatively higher tectonic activity (Figure 5). The stream length gradient values decrease as we move upstream or downstream from the knickpoints.
The sinuosity index suggests that the river is more sinuous in the lower reaches (6–7 reach). The river flows over the loose unconsolidated fluvio-glacial-lacustrine sediments downstream with the result even subtle modifications in the slope manifest the changes in its morphology. The sinuous behavior and differential entrenchment downstream are attributed to the abundant sediment supply from the erosion of the glacial-borne deposits, moraines, of the range. However, the river is less sinuous in the upstream area (1–4 reach), which was occupied by glaciers during the Pleistocene and the glaciers carved deep valleys presently occupied by the river, hence less sinuosity.
Climate change is a change in temperature, rainfall, humidity, wind speed, snowfall pattern, runoff, etc. (Verhoog, 1987). The change in the climate affects the earth's surface processes, which brings changes in the earth's surface by eroding material from one place and depositing this material to other places. Likewise, climate change affects glaciers and glacier related processes which can influence the fluvial system in various ways; by influencing river course and valley orientation (hydrographical), by creating new settings for sediments transport and deposition (hydro-sedimentary), and by vertical motions that are mainly significant during glacial and deglacial periods, i.e., isostasy (Cordier et al., 2017). However, unraveling the impact of glacial melting and river behavior is challenging, especially today, when the glacier cover has drastically reduced, like in the Rambiara River basin, and the direct volume of sediments and water is difficult to calculate. However, mapping the extent and thickness of the past glaciers and the calculation of various fluvial parameters, (e.g., braided index, sinuosity index, entrenchment ratio), and analysis of river terraces provides a basis for the relationship between the glacial melting and fluvial response.
The Cenozoic uplift of the PPR has played an important role in changing the climate of the Kashmir Valley from tropical to temperate type (Dar et al., 2015a). The uplift has probably contributed to cooling and the onset of glaciation in the range (Dar and Zeeden, 2020). Recently, the nature and extent of glaciation of the range have been investigated and few maps have been published on the glacial geomorphology of the area (Paul et al., 2021). However, the relationship between the melting cryosphere, climate change, and the fluvial response has remained unexplored. It is agreed that valley scouring and moraine formation is linked to major glacial phases. For example, during the Early Pleistocene glacial periods, the glaciers advanced much below the mountain ridges, and a large number of moraines (lateral, medial, ground, and terminal) formed in the PPR (Paul et al., 2021) (Figures 3, 4). During the Late Pleistocene glacial phases, for example during LGM, glaciers did not advance much below 10 km from the present-day cirque and rock glaciers (Paul et al., 2021). On the basis of the analysis of the transverse profile of the glacial troughs and the maximum height of the lateral moraines and trimlines, it is evident that the ice was on an average ~200 m thick during the maximum of the glaciation. The presence of numerous recessional (terminal) moraines, small outwash plains, and kettles, advocate continuous deglaciation of the range and the constant decrease in glacial extents (Murtaza and Romshoo, 2021). The presence of the recent rock glaciers and outwash plains up in the glacial troughs indicate the continuity of the deglaciation in the area (Figure 3). During warm interglacial periods, meltwater and sediments from the glaciers and moraines were channeled through numerous small valleys downstream. Since the river discharge has considerably decreased, as observed during the field study, owing to the continuous decrease in the glacial cover (current glacier cover ~1.25 km2) and also due to changes in precipitation pattern (Dar et al., 2013, 2017; Romshoo et al., 2017, 2020), much of the transported sediments have remained preserved especially along the steep valley walls and along the river bed. Terraces preserved along the banks of the Rambiara River contain a thick sedimentary sequence with intervening gravel beds, sand, and, clay lenses (Figure 5b). The coarser sediments/boulder, which makes up the larger proportion of these terraces are composed of the lithologies (basalt, limestone) occurring in the glaciated part of the range. Some of the gravel beds preserved at the top of the river terraces are dated more than 350 kyr old (Bronger et al., 1987). The estimated maximum thickness of these gravel beds is 135 m and is regarded as fluvial-glacial in origin (De Terra, 1939).
Similarly, the occurrence of a large number of alluvial fans at the apron of the confined (hanging) valleys, suggest continuous glacial recession of the PPR. The fan sequence indicates high sediment flux associated with the fluctuating incision and aggradation rates. The distal part of most of these fans is eroded by the streams emanating from the snow-glacier meltwater and precipitation. Morphologically these fans are correlated with the recent deglaciation; however, no chronological data is available. Similar glacio-fluvial fans might have formed in relation to the former-recession episodes. However, owing to the various (four?) glacial periods these fans might have been eroded away during the deglaciation phases.
In glaciated terrains, sediments and surfaces get exposed during the deglacial phases (cold to warm transition) due to receding glaciers and provide a vast source of erodible material that is eroded, transported, and deposited downstream (Cordier et al., 2017). The sediments deposited in the Karewa lake (Hirpur and Nagum Formations) were subsequently exposed due to the desiccation and the draining out of the waters that resulted in base-level change after which the fluvial incision ensued. The alluvial fill incision can be seen from the vertical incision through thick alluvial deposits and river terraces along the course of the Rambiara River. The vertical incision is also influenced by glacio-isostasy; however, this assumption needs further research in the PPR range which is also affected by Himalayan tectonics. Besides, the glacial cycles of the Kashmir Himalaya have been very complex owing to the complex interplay of mountain building, climatic, and hydrographic changes (Brozovic et al., 1997). Therefore, fluvial incision observed in the Rambiara basin is a combination of glacial dynamics, base-level change, glacio-isostasy (typical of glaciated mountain ranges), and the regional tectonic uplift.
The glacial and aeolian system connectivity is important in the production and preservation of windblown dust (loess). Besides, loess deposits are generally closely related to glacial processes and changes in global and regional climate change (Újvári et al., 2016). The downstream area of the basin is characterized by the presence of loess deposits, belonging to the Dilpur Formation of the Karewa Group. At few places, former river terraces are covered with thick loess deposits (Figure 6) and show the presence of interbedded paleosols. Overall, the loess deposits of Kashmir are intercalated with 10–12 paleosol profiles and range in thickness from a few meters to more than 20 m (Dar et al., 2015a,b). The available chronology of these deposits suggests that loess deposition dates back to 350 kyr (Bronger et al., 1987). Since four major glacial periods have been reported from the moraine of the Pir Panjal Range (De Terra, 1939; Holmes, 1989), the rock floor produced during the movement of the glaciers might have provided the basic mineral dust for the deposition of the loess deposits. Pertinently four glacial and interglacial periods have also been recognized in these (loess) deposits (Bronger et al., 1987). A recent study has suggested that the mineral dust for the deposition of the Kashmir Loess was local (Zeeden et al., 2021). Therefore, the loess contains a detailed picture of the changing Quaternary climates and the glacial dynamics of the region.
Rambiara is a braided river having multiple wide and shallow channels, reflecting high sediment load and the non-cohesive nature of bank materials. The melting cryosphere and sediment deposition have profoundly affected the morphology of the Rambiara River as revealed by the thick fluvial-glacial river bank sediments, channel width variations, sinuosity, braided pattern, bank entrenchment, etc. Since the channel morphology is closely related to the volume of sediments deposited, which in turn depends on the water discharge and on the climate change. If owing to climate change, the water discharge increases (due to glacial melting or increase in rainfall), the sediment volume, in the long run, will become exhausted. This will lead to an increase in channel depth, decrease in gradient, and increase in sinuosity. According to Woolderink et al. (2021), a river changes to a sinuous one to maintain a constant channel gradient. Therefore, sinuosity acts as a proxy for the water discharge, sediment flux, and channel migration; the former two factors are determined by the climate (Limaye et al., 2021). The Rambiara River shows sinuous nature and a high braided index in its lower reaches (4–7) where the river gradient is very low (Table 1). Rivers change to the braided patterns as a result of changing gradient, changes in hydrology, sediment load as well as active tectonics. In case of greater in-channel sediment storage, the greater would be the degree of braiding as the sediment inputs modify the channel morphology by altering sediment mobility (Hassan et al., 2007, 2008). In the upper reaches the river is less sinuous and the braided index is also low. In these reaches (1–3) the high gradient and the partly confined settings result in a straight river course and also leads to downstream movement of the sediment load. The sinuosity can also be attributed to the vertical motion, as the range is uplifting at a rate of 10 mm per year (Burbank and Johnson, 1983) owing to the tectonics and glaciotectonics. This is evident from the presence of unpaired terraces and the asymmetry factor of (61.5). Studies carried out on major rivers of the world reflect that these rivers are significantly affected by slight changes in the valley slope even though it seems that the high discharge of these rivers can enable them to maintain a straight course (Schumm, 1979). Therefore, it is believed that the course and pattern of the Rambiara River are affected by the high sedimentation rate brought from the erosion of the unconsolidated glacial deposits, moraines.
Channel widening and braiding can increase if the river course is cut across by geological structures like faults and lineaments (Woolderink et al., 2021). This is observed in the Rambiara River, especially in its reach 4. Here the river is cut across by the Balapur fault (Ahmad et al., 2015) and the river is widest owing to the deformation of the bed and banks associated with the fault activity. This fault is visible on the longitudinal river profile in the form of a knickpoint and also by the abrupt increase in the braided index in this reach (Table 1). The river gradient changes abruptly after crossing this knickzone, with the result deposition of the bed load has resulted in the formation of a large island bar (Figure 5b). The analysis of the terraces reveals that the river might have attained a width of 1,000 m at a few locations during the former glacial periods. The wide nature of the river has resulted in the development of many inland braided bars, especially in the reaches (4–7). The braided index suggested a greater amount of sediment storage within the channel in these reaches. Braiding decreases as the sediment flux decrease or the river are confined in a narrow setting or a fault runs along the river course (Holbrook and Schumm, 1999). The asymmetry factor (AF) indicates that the Rambiara river has shifted downstream to the left of the basin. The basin tilt goes well with the preservation of terraces along the right bank and erosion of left bank terraces (Figure 6b) as well as the entrenchment ratio of 0.9 in the reach 5 of the river.
Glaciers can also influence the fluvial system through glacio-isostasy. However, unlike impacts of glacial activity, the effects of glaciotectonics are difficult to identify especially in the upper reaches of the mountains. Besides, isolating the glaciotectonic signal from the tectonic uplift is challenging especially in the PPR which rapidly rises at an average rate of 10 mm per year (Burbank and Johnson, 1983). Nevertheless, the deglaciation of the range in combination with the tectonic uplift might have led to the vertical exhumation of the range as is evident from the straight mountain fronts and vertical valley walls and few knickpoints which are associated with the faults observed in the Kashmir Valley (Dar et al., 2014). The deglaciation and the consequent isostatic uplift are confirmed from the mountain front sinuosity values obtained across the basin (Table 2). The impacts of glaciation on the fluvial dynamics are superimposed on the long-term tectonic characteristics of the range. The mountain front sinuosity index measured along 42 front segments range from 1.01 to 1.56 indicating active mountain fronts (Table 2). Besides, the presence of numerous triangular facets and a few well-defined lineaments/faults in the basin gives credence to this inference.
Table 2. Mountain front sinuosity values calculated from mountain fronts in the upper reached of the Rambiara basin.
This paper is a first attempt to present the relationship between glacial and fluvial systems in the Rambiara Basin of the Kashmir Himalaya. The analysis reveals that the glacial-fluvial connectivity is complex owing to multiple glacial and interglacial cycles witnessed in the range. This is well substantiated by the presence of glacial geomorphic features and moraine deposits of different glacial stages and diversity in the grain size of the river terraces. The fluvial system has responded to the changing sediment and water discharge through changes in sinuosity, braiding, entrenchment, and through changes in the stream gradient. The study suggests that detailed mapping of glacial landforms and analysis of fluvial systems is key for understanding the spatial and temporal relationship between glacial and fluvial systems. Since the methodology applied is believed to be among the first attempts to study glacial dynamics and fluvial response in the Kashmir Himalaya, the literature discussing this method was scarce and quite limited. In addition, the lack of age constraints on glacial and fluvial landforms, the exact timing and the nature of deglaciation and fluvial response though informative remains indicative only. Therefore, detailed high-resolution mapping of fluvial-glacial landforms supported with absolute chronology should form the future course of the work on the subject.
The original contributions presented in the study are included in the article/supplementary material, further inquiries can be directed to the corresponding author/s.
RD conceived the idea and took lead in writing the manuscript with support from KM, FD, and AN. RM, NA, and OP provided critical feedback, helped in fieldwork, prepared figures, and carried out the geomorphic analysis of fluvial-glacial-geomorphic features. All authors contributed and approved the submitted manuscript.
The authors declare that the research was conducted in the absence of any commercial or financial relationships that could be construed as a potential conflict of interest.
All claims expressed in this article are solely those of the authors and do not necessarily represent those of their affiliated organizations, or those of the publisher, the editors and the reviewers. Any product that may be evaluated in this article, or claim that may be made by its manufacturer, is not guaranteed or endorsed by the publisher.
Agrawal, D. P., Dodia, R., Kotlia, B. S., Razdan, H., and Sahni, A. (1989). The Plio-Pleistocene geologic and climatic record of the Kashmir valley, India: a review and new data. Palaeogeogr. Palaeoclimatol. Palaeoecol. 73, 267–286. doi: 10.1016/0031-0182(89)90008-4
Ahmad, S., Alam, A., Ahmad, B., Bhat, M. I., and Bhat, M. S. (2015). Geomorphic evidence of unrecognized Balapur fault segment in the southwest Kashmir basin of northwest Himalayas. Geomorphology 250, 159–172. doi: 10.1016/j.geomorph.2015.09.006
Ahmad, S., Bhat, M. I., Madden, C., and Bali, B. S. (2013). Geomorphic analysis reveals active tectonic deformation on the eastern flank of the Pir Panjal Range, Kashmir Valley India. Arab. J. Geosci. 7, 2225–2235. doi: 10.1007/s12517-013-0900-y
Antoine, P., Lautridou, J.-P., and Laurent, M. (2000). Long-term fluvial archives in NW France: response of the Seine and Somme rivers to tectonic movements, climatic variations and sea level changes. Geomorphology 33, 183–207. doi: 10.1016/S0169-555X(99)00122-1
Bhatt, D. K. (1982). A review of the stratigraphy of the Karewa Group (Pliocene/Quaternary), Kashmir. Man Environ. 6, 46–55.
Bronger, A., Pant, R. K., and Singhvi, A. K. (1987). Pleistocene climatic changes and landscape evolution in the Kashmir Basin, India: paleopedologic and chronostratigraphic studies. Quaternary Res. 27, 167–181. doi: 10.1016/0033-5894(87)90075-5
Brozovic, N., Burbank, D. W., and Meigs, A. J. (1997). Climatic limits on landscape development in the northwestern Himalaya. Science 276, 571–574. doi: 10.1126/science.276.5312.571
Bull, W. B. (1984). Tectonic geomorphology. J. Geol. Educ. 32, 310–324. doi: 10.5408/0022-1368-32.5.310
Burbank, D. W., and Johnson, G. D. (1983). The late Cenozoic chronologic and stratigraphic development of the Kashmir intermontane basin, northwestern Himalaya. Palaeogeogr. Palaeoclimatol. Palaeoecol. 43, 205–235. doi: 10.1016/0031-0182(83)90012-3
Cordier, S., Adamson, K., Delmas, M., Calvet, M., and Harmand, D. (2017). Of ice and water: quaternary fluvial response to glacial forcing. Quaternary Sci. Rev. 166, 57–73. doi: 10.1016/j.quascirev.2017.02.006
Dar, R. A., Chandra, R., and Romshoo, S. A. (2013). Morphotectonic and lithostratigraphic analysis of intermontane Karewa basin of Kashmir Himalayas, India. J. Mountain Sci. 10, 1–15. doi: 10.1007/s11629-013-2494-y
Dar, R. A., Chandra, R., Romshoo, S. A., and Kowser, N. (2015c). Micromorphological investigations of the late quaternary loess–paleosol sequences of the Kashmir Valley, India. J. Asian Earth Sci. 111, 328–338. doi: 10.1016/j.jseaes.2015.07.004
Dar, R. A., Chandra, R., Romshoo, S. A., Lone, M. A., and Ahmad, S. M. (2015a). Isotopic and micromorphological studies of Late Quaternary loess–paleosol sequences of the Karewa Group: inferences for palaeoclimate of Kashmir Valley. Quaternary Int. 371, 122–134. doi: 10.1016/j.quaint.2014.10.060
Dar, R. A., Chandra, R., Romshoo, S. A., Lone, M. A., and Ahmad, S. M. (2015b). Reply to the comment by Shah on “Isotopic and micromorphological studies of Late Quaternary loess-paleosol sequences of the Karewa Group: inferences for palaeoclimate of Kashmir Valley”. Quaternary Int. 374, 200–202. doi: 10.1016/j.quaint.2015.03.029
Dar, R. A., Jaan, O., Murtaza, K. O., and Romshoo, S. A. (2017). Glacial-geomorphic study of the Thajwas glacier valley, Kashmir Himalayas, India. Quaternary Int. 444, 157–171. doi: 10.1016/j.quaint.2017.05.021
Dar, R. A., and Ramshoo, S. A. (2013). Estimating daily stream flow in the glacierized Mountainous Kashmir Himalayan Basin. J. Res. Dev. 12. 113–130.
Dar, R. A., Romshoo, S. A., Chandra, R., and Ahmad, I. (2014). Tectono-geomorphic study of the Karewa Basin of Kashmir Valley. J. Asian Earth Sci. 92, 143–156. doi: 10.1016/j.jseaes.2014.06.018
Dar, R. A., and Zeeden, C. (2020). Loess-palaeosol sequences in the Kashmir Valley, NW Himalayas: a review. Front. Earth Sci. 8, 113. doi: 10.3389/feart.2020.00113
Dar, R. A., Paul, O. J., Murtaza, K. O., and Romshoo, S. A. (2021). “Late Quaternary glacial geomorphology of Kashmir Valley, NW Himalayas: a case study of the Sind basin,” in: Water, Cryosphere, and Climate Change in the Himalayas, eds A. K. Taloor, B. S. Kotlia, and K. Kumar (Cham: Springer), 145–157.
De Terra, H. (1939). Studies on the Ice-Age in India and associated human cultures. Publ. Carnegie Inst 493, 354.
Dixit, A., Sahany, S., and Kulkarni, A. V. (2021). Glacial changes over the Himalayan Beas basin under global warming. J. Environ. Manage. 295, 113101. doi: 10.1016/j.jenvman.2021.113101
Fu, P., and Harbor, J. (2011). “Glacial erosion,” in Encyclopedia of Snow, Ice and Glaciers, eds V. P. Singh, P. Singh, and U. K. Haritashya (Dordrecht: Springer Netherlands), 332–341.
Hack, J. T. (1973). Stream-profile analysis and stream-gradient index. J. Res. US Geol. Survey 1, 421–429.
Hassan, M. A., Gottesfeld, A. S., Montgomery, D. R., Tunnicliffe, J. F., Clarke, G. K., Wynn, G., et al. (2008). Salmon-driven bed load transport and bed morphology in mountain streams. Geophys. Res. Lett. 35. 1–6. doi: 10.1029/2007GL032997
Hassan, M. A., Smith, B. J., Hogan, D. L., Luzi, D. S., Zimmermann, A. E., and Eaton, B. C. (2007). 18 Sediment storage and transport in coarse bed streams: scale considerations. Dev. Earth Surface Processes 11, 473–496. doi: 10.1016/S0928-2025(07)11137-8
Holbrook, J., and Schumm, S. A. (1999). Geomorphic and sedimentary response of rivers to tectonic deformation: a brief review and critique of a tool for recognizing subtle epeirogenic deformation in modern and ancient settings. Tectonophysics 305, 287–306. doi: 10.1016/S0040-1951(99)00011-6
Holmes, J. A. (1989). The Quaternary glacial history of Kashmir, north-west Himalaya: a revision of de Terra and Paterson's Sequence. Z. Geomorph. NF, Suppl. Bd. 76, 195–212.
Huggett, R. (2007). A history of the systems approach in geomorphology. Géomorphol. Relief Processus Environ. 13, 145–158. doi: 10.4000/geomorphologie.1031
Jaan, O., Lone, S., Malik, R., Lone, A., and Wasim, M. (2015). Morphotectonic and morphometric analysis of Vishav basin left bank tributary of Jhelum River SW Kashmir Valley India. Int. J. Econ. Environ. Geol. 17–26. doi: 10.46660/ijeeg.Vol0.Iss0.0.101
Joya, E., Bromand, M. T., Murtaza, K. O., and Dar, R. A. (2021). Current glacier status and ELA changes since the Late Pleistocene in the Hindu Kush Mountains of Afghanistan. J. Asian Earth Sci. 219, 104897. doi: 10.1016/j.jseaes.2021.104897
Kaewmuangmoon, S., Thipyopass, S., Kosuwan, S., Daorerk, V., and Charusiri, P. (2008). “Investigations on tectonic geomorphology along the Khlong Marui Fault, Kao Phanom area, Southern Thailand: application of ArcGIS approach,” in Proceedings of the International Symposia on Geoscience Resources and Environments of Asian Terranes (GREAT 2008), 4th IGCP. Vol. 516, 24–26.
Keller, E. A., and Pinter, N. (2002). Active Tectonics, Earthquakes, Uplift and Landscape. 2nd Edn. Prentice Hall, NJ: Upper Saddle River. p. 362.
Kumar, R., Manzoor, S., Vishwakarma, D. K., Al-Ansari, N., Kushwaha, N. L., Elbeltagi, A., et al. (2022). Assessment of climate change impact on snowmelt runoff in Himalayan region. Sustainability 14, 1150. doi: 10.3390/su14031150
Limaye, A. B., Lazarus, E. D., Li, Y., and Schwenk, J. (2021). River sinuosity describes a continuum between randomness and ordered growth. Geology 49, 1506–1510. doi: 10.1130/G49153.1
Marazi, A., and Romshoo, S. A. (2018). Streamflow response to shrinking glaciers under changing climate in the Lidder Valley, Kashmir Himalayas. J. Mountain Sci. 15, 1241–1253. doi: 10.1007/s11629-017-4474-0
Mehta, M., Dobhal, D. P., and Bisht, M. P. S. (2011). Avalanche morphometry and hazard potential assessment in Laxman Ganga catchment Garhwal Himalaya. Himalayan Geol. 32, 159–167.
Młyński, D., Wałega, A., and Kuriqi, A. (2021). Influence of meteorological drought on environmental flows in mountain catchments. Ecol. Indicat. 133, 108460. doi: 10.1016/j.ecolind.2021.108460
Molnar, P., and England, P. (1990). Late Cenozoic uplift of mountain ranges and global climate change: chicken or egg? Nature 346, 29–34. doi: 10.1038/346029a0
Murtaza, K. O., Dar, R. A., Paul, O. J., Bhat, N. A., and Romshoo, S. A. (2021). Glacial geomorphology and recent glacial recession of the Harmukh Range, NW Himalaya. Quaternary Int. 575, 236–248. doi: 10.1016/j.quaint.2020.08.044
Murtaza, K. O., and Romshoo, S. A. (2017). Recent glacier changes in the Kashmir alpine Himalayas, India. Geocarto Int. 32, 188–205.
Murtaza, K. O., and Romshoo, S. A. (2021). Applications of glacial geomorphological and lichenometric studies for reconstructing the Late Holocene glacial history of the Hoksar valley, Kashmir Himalaya, India. Geografiska Annaler Series A Phys. Geogr. 103, 51–68. doi: 10.1080/04353676.2020.1822001
Past Interglacials Working Group of PAGES (2016). Interglacials of the last 800,000 years. Rev. Geophys. 54, 162–219. doi: 10.1002/2015RG000482
Paul, F., Kääb, A., Maisch, M., Kellenberger, T., and Haeberli, W. (2002). The new remote-sensing-derived Swiss glacier inventory: I. Methods. Ann. Glaciol. 34, 355–361. doi: 10.3189/172756402781817941
Paul, O. J., Dar, R. A., and Romshoo, S. A. (2021). Paleo-glacial and paleo-equilibrium line altitude reconstruction from the Late Quaternary glacier features in the Pir Panjal Range, NW Himalayas. Quaternary Int. doi: 10.1016/j.quaint.2021.03.005
Paul, O. J., Dar, R. A., and Romshoo, S. A. (2022). Cirque Development in the Pir Panjal Range of North Western Himalaya. India. CATENA 213, 106179. doi: 10.1016/j.catena.2022.106179
Romshoo, S. A., Dar, R. A., Murtaza, K. O., Rashid, I., and Dar, F. A. (2017). Hydrochemical characterization and pollution assessment of groundwater in Jammu Siwaliks, India. Environ. Monit. Assessment 189, 1–19. doi: 10.1007/s10661-017-5860-3
Romshoo, S. A., Fayaz, M., Meraj, G., and Bahuguna, I. M. (2020). Satellite-observed glacier recession in the Kashmir Himalaya, India, from 1980 to 2018. Environ. Monit. Assessment 192, 1–17. doi: 10.1007/s10661-020-08554-1
Romshoo, S. A., Murtaza, K. O., Shah, W., Ramzan, T., Ameen, U., and Bhat, M. H. (2022). Anthropogenic climate change drives melting of glaciers in the Himalaya. Environ. Sci. Pollut. Res. 1–20. doi: 10.1007/s11356-022-19524-0
Schumm, S. A. (1979). Geomorphic thresholds: the concept and its applications. Trans. Inst. Br. Geogr. 485–515. doi: 10.2307/622211
Sheinkman, V. (2016). Quaternary glaciation in North-Western Siberia–New evidence and interpretation. Quaternary Int. 420, 15–23. doi: 10.1016/j.quaint.2015.11.147
Stojanovic, D., Aitchison, J. C., Ali, J. R., Ahmad, T., and Dar, R. A. (2016). Paleomagnetic investigation of the Early Permian Panjal Traps of NW India; regional tectonic implications. J. Asian Earth Sci. 115, 114–123. doi: 10.1016/j.jseaes.2015.09.028
Újvári, G., Kok, J. F., Varga, G., and Kovács, J. (2016). The physics of windblown loess: implications for grain size proxy interpretations in Quaternary paleoclimate studies. Earth Sci. Rev. 154, 247–278. doi: 10.1016/j.earscirev.2016.01.006
Verhoog, F. H. (1987). “Impact of climate change on the morphology of river basins,” in Proceedings of the Vancouver Symposium, IAHS, Publ (No. 168).
Wadia, D. N. (1942). The sources of the rivers Indus, Sutlej, Ganges and Brahmaputra. Curr. Sci. 11, 351–353.
Wang, S. J., and Zhang, T. (2013). Glacial lakes change and current status in the central Chinese Himalayas from 1990 to 2010. J. Appl. Remote Sens. 7, 073459. doi: 10.1117/1.JRS.7.073459
Wolman, M. G., and Miller, J. P. (1960). Magnitude and frequency of forces in geomorphic processes. J. Geol. 68, 54–74. doi: 10.1086/626637
Woolderink, H. A. G., Cohen, K. M., Kasse, C., Kleinhans, M. G., and Van Balen, R. T. (2021). Patterns in river channel sinuosity of the Meuse, Roer and Rhine rivers in the Lower Rhine Embayment rift-system, are they tectonically forced? Geomorphology 375, 107550. doi: 10.1016/j.geomorph.2020.107550
Zeeden, C., Mir, J. A., Vinnepand, M., Laag, C., Rolf, C., and Dar, R. A. (2021). Local mineral dust transported by varying wind intensities forms the main substrate for loess in Kashmir. EG Quaternary Sci. J. 70, 191–195. doi: 10.5194/egqsj-70-191-2021
Keywords: cryosphere, river morphology, Kashmir Himalaya, LGM, climate change, late qauternary, glacio-isostasy
Citation: Dar RA, Murtaza KO, Paul OJ, Nisa AU, Akhter N, Dar FA and Mir RA (2022) River Response to Melting Cryosphere Since Late Quaternary in the Pir Panjal Range of NW Himalaya. Front. Water 4:879001. doi: 10.3389/frwa.2022.879001
Received: 18 February 2022; Accepted: 11 April 2022;
Published: 29 April 2022.
Edited by:
Lahoucine Hanich, Cadi Ayyad University, MoroccoReviewed by:
Luigi Bruno, University of Modena and Reggio Emilia, ItalyCopyright © 2022 Dar, Murtaza, Paul, Nisa, Akhter, Dar and Mir. This is an open-access article distributed under the terms of the Creative Commons Attribution License (CC BY). The use, distribution or reproduction in other forums is permitted, provided the original author(s) and the copyright owner(s) are credited and that the original publication in this journal is cited, in accordance with accepted academic practice. No use, distribution or reproduction is permitted which does not comply with these terms.
*Correspondence: Reyaz Ahmad Dar, cmV5YXpkYXJAa2FzaG1pcnVuaXZlcnNpdHkuYWMuaW4=
Disclaimer: All claims expressed in this article are solely those of the authors and do not necessarily represent those of their affiliated organizations, or those of the publisher, the editors and the reviewers. Any product that may be evaluated in this article or claim that may be made by its manufacturer is not guaranteed or endorsed by the publisher.
Research integrity at Frontiers
Learn more about the work of our research integrity team to safeguard the quality of each article we publish.